Abstract
Purpose
Canadian Organization on Health Effects from Radiation Exposure (COHERE) is a government initiative to better understand biological and human health risks from ionizing radiation exposures relevant to occupational and environmental settings (<100 mGy, <6 mGy/h). It is currently a partnership between two federal agencies, Health Canada (HC) and the Canadian Nuclear Safety Commission (CNSC). COHERE’s vision is to contribute knowledge to reduce scientific uncertainties from low dose and dose-rate exposures. COHERE will advance our understanding by bridging the knowledge gap between human health risks and linkages to molecular- and cellular-level responses to radiation. Research focuses on identifying sensitive, early, and key molecular events of relevance to risk assessment.
Conclusions
The initiative will address questions of relevance to better apprize Canadians, including radiation workers and members of the public and Indigenous peoples, on health risks from low dose radiation exposure and inform radiation protection frameworks at a national and international level. Furthermore, it will support global efforts to conduct collaborative undertakings and better coordinate research. Here, we describe a historical overview of the research conducted, the strategic research agenda that outlines the scientific framework, stakeholders, opportunities to harmonize internationally, and how research outcomes will better inform communication of risk to Canadians.
Introduction
Background
The current radiation protection framework is practical and built to ensure the health and safety of workers, the members of the public and Indigenous peoples, and the environment. While the framework is prudent and conservative, there continues to be a need for low-dose radiation research (LDRR) to fill existing knowledge gaps. New knowledge can help address: (1) large statistical uncertainties in dose-response at low doses (<100 mGy), (2) lack of consensus amid an ongoing debate among scientific communities regarding the biological and health effects at a low dose, and (3) continued public concern and fear of radiation.
The Nuclear Energy Agency (NEA) has been seeking inputs from member states on the possibility of global coordination and collaboration of low dose radiation research to more effectively address the relevant questions (i.e. cost and research efficiency) to operate and manage such a program (National Academies of Sciences, Engineering, and Medicine et al. Citation2019). This will support a multidisciplinary approach to LDRR, allowing for harmonization that can contribute new knowledge to international radiation protection bodies (Repussard Citation2019). This initiative began following NEA’s recognition of the continued need for an LDRR program for government funding as well as the ongoing efforts to effectively collaborate and coordinate research. Part of the discussions involved tasks geared toward developing a harmonized risk communication strategy for more efficient and targeted risk communication.
Two agencies of the Government of Canada [Health Canada (HC) and the Canadian Nuclear Safety Commission (CNSC)] are members of the NEA initiative and play an important role in conducting LDRR. Both agencies strive to communicate their research findings and inform Canadians of the risks from radiation. The factors impacting effective risk communication are complex, and most agree that risk communication and radiation education need improvement in a society where information (and misinformation) is so readily accessible (Weiss and Yonekura Citation2019). To this end, there is a concrete effort toward the integration of social sciences and humanities in radiation protection (Perko et al. Citation2019).
Given that HC and CNSC are invested in improving research coordination and how research information is communicated, the establishment of a Canadian Organization on the Health Effects from Radiation Exposure (COHERE) program will be valuable. In this paper, a historical perspective of the research conducted by the Government of Canada is provided as well as an overview of COHERE and possibilities for expansion to the national level.
Historical perspective
The Canadian radiation protection framework is modeled on the international framework which to date are informed by human health risks from radiation exposure derived using large databases of cancer and non-cancer health outcomes from atomic bomb survivors, nuclear accidents, and occupational and medical exposure scenarios. Accumulating scientific knowledge emerging over the last decades clearly indicates that these models may not be accurate at the low doses and more research is needed to reduce this uncertainty. Globally, conversations around this topic have led to identifying priority areas for research, efforts to better coordinate projects (to prevent unnecessary duplication of work), and improved ways to inform stakeholders about risks. Canada is a leader in low dose research (Radiation Research Special Issue: Snolab Citation2017; Health Physics Special Issue: Canadian Radiation Protection and Research 2019). Alone and in collaboration, HC and CNSC have contributed significantly to the Canadian landscape of LDRR in areas that include dosimetry, radiobiology, and epidemiology, demonstrating the value of a more formalized approach to cooperation and coordination between the two government institutions.
Health Canada LDRR
Health Canada is responsible for helping Canadians maintain and improve their health. It also acts on requests from other federal departments and agencies on occupational and public health matters related to radiation safety. Two Bureaus within HC focus on radiation health effects: The Radiation Protection Bureau (RPB) and the Consumer and Clinical Radiation Protection Bureau (CCRPB). Together, these Bureaus evaluate, monitor, and assist in the management of risk associated with radiation exposure from devices, occupational, and public environments. In addition, they undertake research into the biological effects of radiation exposure to develop technical advice for responsible authorities. Some HC scientists have recently focused their research efforts on developing experimental studies to reduce uncertainties at low doses. This includes radiation effects related to chronic and acute exposures, low and high doses, and varied dose rates. The outcomes of this research contribute to the Addressing Air Pollution Horizontal Initiative (AAPHI), the Radiation Emitting Device Act, as well as the work of international scientific committees, such as the United Nations Scientific Committee on the Effects of Atomic Radiation (UNSCEAR). HC developed the relevant studies, as described below, jointly with partners across academia and industry.
Adverse outcome pathway integration into radiation risk assessment
The adverse outcome pathway (AOP) framework is a means to consolidate existing mechanistic and epidemiological studies. It assembles knowledge across levels of biological organization to formulate key events linked to adverse outcomes of relevance to regulatory decision-making (Ankley et al. Citation2010). A formalized AOP development program was launched by the Organization for Economic Co-operation and Development (OECD) in 2012. It has shown promise in informing on knowledge gaps, test method development, and predictive toxicology for risk assessment in chemical toxicity. This approach is gaining traction within the wider radiation research community and HC scientists have been instrumental in these efforts. This has included knowledge transfer through organizing international and national workshops (Chauhan, Said, et al. Citation2019) and illustrating the approach with a case example of lung cancer-relevant to stressors, such as radon gas (Chauhan, Sherman, et al. Citation2021). The work has highlighted that, despite decades of mechanistic research in the radiation field, high-quality empirical evidence data to support causal linkages to adverse outcomes remains limited. Likewise, considerable uncertainties/inconsistencies in the literature are prevalent, predominantly because of the lack of coordinated research across institutions (Chauhan, Stricklin, et al. Citation2021; Chauhan, Villeneuve, et al. Citation2021). Continued work in this area will demonstrate the value of the approach to broadly support many areas in the radiation field including a better understanding of low dose/low dose-rate effects.
Genomics and benchmark dose modeling
Genomics enables an analysis of molecular effects at earlier time points than most conventional tests that rely on observations of disease or overt health effects. Tracking genomic changes over time following exposure to an agent can provide insight into the underlying molecular changes to the development of pathology. In the context of radiation, genomics has been moving forward for decades in several high-profile areas, resulting in a large amount of data but with no significant translation to human health risk assessment. Recent developments in high-throughput benchmark dose modeling in chemical toxicity have shown promise in using transcriptional data to identify critical mechanistic pathways and associated safe dose thresholds for various chemical exposure scenarios (Yang et al. Citation2007; Thomas et al. Citation2013). HC has applied these approaches to genomic studies relevant to radiation exposures and shown the approach to be valuable in identifying pathway and gene-based point-of-departures, that can be used to compare radiation qualities, dose-rates and model systems (Chauhan et al. Citation2016; Qutob et al. Citation2018; Chauhan, Rowan-Carroll, et al. Citation2019; Chauhan, Adam, et al. Citation2021). More recently, HC secured funding through the Genomics Research and Development Initiative (https://grdi.canada.ca/en) for a five-year project aimed at reducing knowledge gaps and uncertainty in risk associated with low-dose radiation exposures, using transcriptional data and benchmark dose modeling. Three projects are underway. The first is reviewing existing genomic studies and applying benchmark dose modeling to identify the dose needed to begin observing changes in pathways and gene responses under a variety of exposure scenarios. In the second sub-project, human blood will be exposed to ionizing radiation under physiological conditions (i.e. optimal pH and temperature) at a broad dose range using varied dose rates and at levels that will allow for effective modeling of data in the low-dose range. Such ex vivo blood irradiated studies are cost-effective and as informative as in vivo studies. The third sub-project will identify doses that trigger gene/protein markers in animal models that have been exposed to very low doses of radiation from uranium.
Lens of the eye
The deterministic nature of cataract formation from radiation exposure is of interest. The eye lens is one of the most radiosensitive tissues in the human body (ICRP, Citation2007). Post-radiation exposure, the lens of the eye can undergo subtle changes, which can take many years to manifest as visible cataracts. HC has led a series of studies that have contributed to the advancement of knowledge on mechanisms of radiation-induced cataracts. State-of-art genomic, proteomic, and targeted metabolic technology in conjunction with Raman spectroscopy have provided data on pathways that are critical drivers in radiation-induced cataracts. The approaches have identified biological pathways most important to radiation-induced macro-molecular level effects. Together, this information alongside published literature has been valuable for guiding further studies in occupational exposures with a long-term vision of being developed into early prognostic predictors of eye disease (Allen et al. Citation2018; Bahia et al. Citation2018; Chauhan, Rowan-Carroll, et al. Citation2019).
Cytogenetics
As the lead national laboratory for biological dosimetry, HC researchers have focused on the use of cytogenetics and DNA damage and repair to measure biological damage to radiation doses down to 100 mGy. Dicentrics measured by the dicentric chromosome assay (DCA), translocations measured by fluorescence in situ hybridization (FISH), micronuclei in binucleated cells, and DNA double-strand break induction as measured by γH2AX foci formation, all provide mechanistic information that feeds into the radiation response pathways (Andrievski and Wilkins Citation2009; McNamee et al. Citation2009; Flegal et al. Citation2012; Beaton-Green et al. Citation2016). Based on this work, HC scientists have contributed greatly to the development of standards for these methods (ISO 17099 Citation2014; ISO 19238 Citation2014; ISO 20046 Citation2019) and a guidance document for the initial phase assessment of individual doses after acute exposure to ionizing radiation (ICRU Citation2019). Research is also being conducted on developing imaging flow cytometry-based methods for the measurement of radiation damage that might have applications in the low dose region (Rodrigues et al. Citation2016; Beaton-Green et al. Citation2017; Wilkins et al. Citation2017). Furthermore, the DCA, FISH, genomics (Chauhan et al. Citation2014) and γH2AX assays have been used to examine the radiation sensitivity of patients exposed to external beam radiotherapy for prostate cancer (Beaton et al. Citation2013; Beaton et al. Citation2013; Beaton et al. Citation2013).
Raman spectroscopy
Raman spectroscopy (RS) is a label-free technique that allows for the real-time acquisition of spectral data on vibrational changes of biologics in the specimen being analyzed (Baker et al. Citation2016). It measures the inelastic scattering of low-intensity, non-destructive laser light by a sample, providing information on its content. In the case of a biological sample, this would include nucleic acids, proteins, lipids, and fatty acids, etc. This process can require minimal sample preparation and is non-damaging to the specimen of interest (Bonifacio et al. Citation2015; Jahn et al. Citation2017). Sensitive portable Raman spectrometers are also available that contain a high-power excitation laser, low-fluorescence optics, and advanced software for in-field use and these systems have been shown to be useful for analyzing body fluids (e.g. blood, saliva, and urine) (Yan and Vo-Dinh Citation2007; Bonifacio et al. Citation2015). Published RS studies are extensive; studies have shown this technology to be successful in quantifying analytes in blood, such as C-reactive-protein, glucose, albumin, urea, cholesterol, and lactate despite their low concentrations (Berger et al. Citation1999; Qu et al. Citation1999; Bergholt and Hassing Citation2009). It has also been shown to be capable of detecting different blood types, metabolite concentration, and pH (Wu et al. Citation2010; Atkins et al. 2015, Citation2016) and to help understand the structure of heme and hemoglobin oxygenation in the blood (Strekas and Spiro Citation1972; Brunner and Sussner Citation1973; Brunner Citation1974; Spiro and Strekas Citation1974; Wood et al. Citation2005; Rusciano et al. Citation2008; Huang et al. Citation2011; Premasiri et al. Citation2012). HC researchers in collaboration with Carleton University and the University of Montreal are leading the design of studies directed to support a non-invasive, label-free, real-time molecular detection of biomolecules (e.g. lipids, proteins, and RNA) in cells, latent fingermarks, and blood using optical spectroscopic approaches. This innovative work has shown promise in identifying spectral molecular-level features indicative of radiation exposure at the single-cell level (Allen et al. Citation2018; Hansson et al. Citation2019).
Dosimetry and biological effects from exposure to uranium and radon
HC has a long history of studying the effects of environmental radiation on Canadians (Tracy et al. Citation1983; Letourneau Citation1985; Tracy et al. Citation1997; Thomas et al. Citation2001; Macdonald et al. Citation2007; Chen et al. Citation2009). In the late 1990s, Health Canada researchers conducted studies on the biological effects of chronic exposure to uranium in drinking water in a group of Canadians using private drinking water wells. The results indicated the loss of proximal tubular function of the kidney (Zamora et al. Citation1998; Zamora et al. Citation2009). More recently, HC, in collaboration with CNSC and the Canadian Nuclear Laboratories (CNL), has led the development of an AOP for kidney toxicity relevant to uranium exposure. To supplement the knowledge gap identified through this AOP development and to acquire a better understanding of the health effects of chronic exposure to uranium in drinking water, HC has launched a collaborative research project with CNL by undertaking an animal experiment on a rodent model.
Extensive efforts to characterize residential radon in Canada and the associated risk led to the establishment of Canada’s National Radon Program (McCullough et al. Citation1981; Levesque et al. Citation1997; Ayotte et al. Citation1998; Chen Citation2005a, Citation2005b; Tracy et al. Citation2006; Chen and Moir Citation2010; Chen Citation2019). More recently, HC has extended the scope of its radon calibration chamber from quality control for radon metrology to an exposure facility, to support studies of radon progeny concentration and indoor aerosol characteristics, thereby improving radon dosimetry. Development of an in-house computational modeling tool for radon dosimetry is also underway to help assess the contribution of indoor aerosol characteristics on the radiation dose to the lung from radon exposure. HC researchers, in collaboration with CNL, are also supporting the commissioning of a radon inhalation chamber for animal experiments (on rodent models) to investigate biomarkers of exposure for dose assessment and biomarkers of effects for risk assessment. HC researchers will use the radon chamber to conduct animal experiments to obtain mechanistic insights into lung carcinogenesis from co-exposure of radon and chemicals present in mainstream cigarette smoke. In addition, work is progressing in the area of identifying biomarkers of radiation exposure in radon cohorts. This pilot study will leverage the Canadian Partnership for Tomorrow’s Health Project (CanPath) that brings together data from over 330,000 Canadians linked to their health information (Awadalla et al. Citation2013; Sweeney et al. Citation2017; Dummer et al. Citation2018). The investigation will use ‘omic’ based technologies and classic cytogenetic techniques to identify biomarkers in the blood of individuals living in high radon areas.
Canadian Nuclear Safety Commission LDRR
As the Canadian nuclear regulator, the CNSC has been involved in LDRR for many years. Research drivers include the mandate (protect human health and the environment as well as disseminate objective scientific information), regulatory questions or changes (e.g. proposed changes to the lens of an eye dose limit), requests from the CNSC members (e.g. tritium toxicity project) and the broader international scientific community [e.g. International Commission on Radiological Protection (ICRP), UNSCEAR]. The CNSC recognizes the importance of research conducted both internally and externally to ensure that it remains a trusted nuclear regulator. A summary of projects supported under CNSC’s Research and Support Program is on the CNSC’s website (Canadian Nuclear Safety Commission 2020d).
Occupational exposures
Historically, much of the work has been epidemiology-based with significant contributions to the study of the health of nuclear energy workers, including uranium miners.
Canada has several cohorts of workers exposed to occupational radon with long-term follow-up of mortality and cancer incidence. These studies assess the radon-lung cancer risk relationship as well as other relationships:
Eldorado cohort (Port Radium uranium mine, Beaverlodge uranium mine, and the Port Hope Radium and Uranium Refining and Conversion Facility),
Ontario uranium miners (Elliott Lake, Bancroft, and Agnew areas),
Newfoundland Fluorspar miners.
Findings from these three cohorts (Villeneuve et al. Citation2007; Lane et al. Citation2010; Navaranjan et al. Citation2016) have contributed significantly to the international understanding of the health effects of occupational radon exposure (National Research Council Citation1999; United Nations Citation2009). The Eldorado and Ontario cohorts are currently part of the Pooled Uranium Miners Analysis (PUMA) (Rage et al. Citation2020) and the International Pooled Analysis of Uranium Workers (iPAUW). Currently, CNSC is leading a Canadian Uranium Workers Study (CANUWS) of ∼80,000 uranium workers (i.e. Eldorado and Ontario cohorts of uranium miners, Northern Saskatchewan uranium mine and mill workers, and Ontario uranium processing and fabrication workers) with long-term mortality and cancer incidence follow-up to 2017.
Studies of Canadian nuclear energy workers include studies of the historic Atomic Energy of Canada Limited workers (Gribbin et al. Citation1993), and all workers occupationally exposed to radiation, whose dose records are in HC’s National Dose Registry (NDR) (Ashmore et al. Citation1998; Sont et al. Citation2001; Zablotska et al. Citation2004; Zablotska et al. Citation2014). These studies are included in international pooled analyses (Cardis et al. Citation1995; Cardis et al. Citation2005; Cardis et al. Citation2007) and have contributed to international scientific consensus documents (United Nations Citation2008). Currently, HC and CNSC are collaborating to address data issues identified among pre-1965 Atomic Energy of Canada Limited (AECL) workers (Ashmore et al. Citation2010; Zablotska et al. Citation2014) and to create an updated linkage of the NDR to mortality and cancer incidence databases at Statistics Canada. These linkages will provide long-term mortality and cancer incidence follow-up of workers to 2017 and many opportunities for future research and collaboration.
Public exposures
The CNSC and HC have conducted many studies of environmental radiation exposures and the health of people who live near nuclear facilities. A series of studies assessed the health of the community due to historic waste disposal practices of the radium and uranium refining and processing industry in Port Hope, Ontario before modern regulation and oversight (Burtt et al. Citation2011; Chen et al. Citation2013). Similarly, a study assessed environmental radiation exposures and cancer incidence around Ontario nuclear power plants (Lane et al. Citation2013). The CNSC also conducted a series of studies on the health effects of tritium in the environment (Thompson Citation2011; Bundy Citation2012). Most recently, in response to the Fukushima accident, the CNSC investigated the consequences of a hypothetical large-scale nuclear power plant accident (Burtt et al. Citation2020).
Radiobiology and the radiation protection framework
The CNSC has recently increased its expertise and capacity in radiobiology. The CNSC, in collaboration with the ICRP, is conducting a systematic review on how sex differences modify ionizing-radiation-induced health effects. It is also building capacity in the development of AOPs. In addition to the work conducted under the auspices of the Research and Support Program, staff regularly contribute to radiation science through publishing and also providing technical support to the Commission on the topic of radiation health sciences (Burtt et al. Citation2016; Leblanc and Burtt Citation2019).
As part of a larger project requested by the Commission to improve the regulation of tritium releases in Canada, the CNSC has and continues to conduct research on tritium environmental behavior and health effects, in collaboration with several national and international partners. Specifically, the report on the Health Effects, Dosimetry and Radiological Protection of Tritium reviewed the scientific literature to assess the health risk from exposures to tritium, assessed Canadian and international dosimetry practices for tritium intakes, and reviewed the current approaches for limiting exposure to tritium (Canadian Nuclear Safety Commission Citation2010; Bundy Citation2012).
Outreach, education, and trust
This research informs not only the radiation protection framework but also the communications to stakeholders. CNSC contributes to informing the members of the public, Indigenous peoples, and other stakeholders by regularly participating in outreach (schools, community events, open houses, etc.) and by creating communication products (information documents, various educational resources, content for social media, etc.) (Canadian Nuclear Safety Commission 2020c). CNSC staff engage with academia, where they supervise graduate student research and provide guest lectures. These activities build capacity and trust, a CNSC strategic priority, and trust-building is further strengthened by valuing stakeholder input and feedback. One example of many is when staff engages with stakeholders outside the context of Commission hearings or meetings through the Independent Environmental Monitoring Program. Stakeholder input is considered for the development of the sampling plan, but also for assessing other communication needs (i.e. concerns needed to be addressed). CNSC also acknowledges the importance of including stakeholders in research working groups to share knowledge and experiences, engagement, and communication. Lastly, CNSC staff are working with their federal partners to document a roadmap that will outline the tools necessary to address the perceived risks of members of the public and Indigenous peoples as they broadly relate to the nuclear industry.
History of federal collaboration
HC and the CNSC have a memorandum of understanding (MOU) which provides a formal framework to coordinate aspects of assessing and managing health risks to Canadians exposed to nuclear substances and radiation-emitting devices. With the CNSC’s regulatory authority, under the Nuclear Safety and Control Act (NSCA), and HC’s responsibilities under the AAPHI and the Radiation Emitting Device Act, they recognize the importance of comprehensive and consistent oversight of radiation safety. Furthermore, both HC and CNSC have federal nuclear emergency preparedness and response responsibilities. The MOU clearly outlines activities for consultation and cooperation to minimize duplication and use government resources effectively.
The CNSC and HC are building upon experience in research and radiation education and communication to ensure a more collaborative and coordinated approach to federal research and proper and effective dissemination of findings. To that end, HC and CNSC contribute to the Federal Nuclear Science & Technology (FNS&T) work plan, managed by the Atomic Energy Canada Limited (AECL) (Citation2020). The objective of this initiative is to perform nuclear-related S&T research to support core federal roles and responsibilities while maintaining the necessary capabilities and expertise at CNL. The CNSC and HC, along with other interested federal departments and agencies, actively participate in FNS&T activities that determine the nature and prioritization of projects. This helps enable collaborations between CNL and COHERE partners and channels research resources toward projects that meet some of the radiobiology needs of both the CNSC and HC (McNamee et al. Citation2009; Flegal et al. Citation2012; Gueguen et al. Citation2018; Chauhan, Said, et al. Citation2019). Current CNL radiobiology research, including but not limited to activities conducted under FNS&T, is described in a recent review by Wang et al. Citation2019.
Canadian organization on health effects from radiation exposure (COHERE)
COHERE and its vision
Inspired by the NEA initiative for global coordination and collaboration, the Radiation Protection Bureau and the Consumer and Clinical Radiation Protection Bureau of HC, and the Directorate of Environmental and Radiation Protection and Assessment of the CNSC, agreed to improve collaboration and coordination of LDRR by establishing a joint LDRR program: Canadian Organization on Health Effects from Radiation Exposure (COHERE). This organization acts as a tool that strengthens the activities under the HC-CNSC MOU, but with a focus on LDRR. Its vision is to lead, contribute, and coordinate Government of Canada low dose research in high priority areas of relevance to Canada and provide insights into priority questions using tools, such as the AOP approach to help guide questions, most notably:
What are the adverse outcome pathways of regulatory interest?
Which early molecular events translate to health outcomes?
Can biologically based models and approaches be valuable in assessing health risk to the population?
Can in silico-derived data produce valuable models to predict relevant radiogenic effects and inform decision-making?
Is an ‘all hazards’ approach to risk assessment feasible in radiation protection practices?
COHERE will build the knowledge base to strengthen the weight of evidence to help address potential health risks from radiation exposure while supporting the respective mandates of HC and the CNSC (). Currently, the structural framework is comprised only of representatives from CNSC and HC to better align the research priorities of these two agencies. Champions for the program provide oversight to a scientific committee, which is responsible for establishing a strategic research agenda and work plan for conducting, presenting, and publishing high-quality research. A communication committee contributes to a communication plan, designs and produces promotional products (e.g. newsletters and pamphlets), and a website presence. Program coordinators interface with the Champions and the scientific and communication committees to ensure all activities under COHERE are delivered ().
The research from COHERE will contribute to the body of knowledge reviewed by UNSCEAR and ICRP to support assessments of the hazards posed by ionizing radiation exposure and inform the international system of radiation protection. This includes directed questions related to population and biological effects at acute and protracted doses <100 mGy. These doses are similar to those emitted from medical diagnostic procedures, occupational and environmental sources, and naturally occurring radioactive materials. The COHERE Workplan will integrate systematic literature surveillance and AOP development with experiments in areas of knowledge gaps using in vitro and animal studies at all levels of biological organization. The data generated will be linked using modeling approaches relevant to humans (). Projects under the umbrella of this organization will focus on the following:
Figure 2. COHERE Research framework includes five main themes and contributes to Canadian guidance on radiation protection standards.
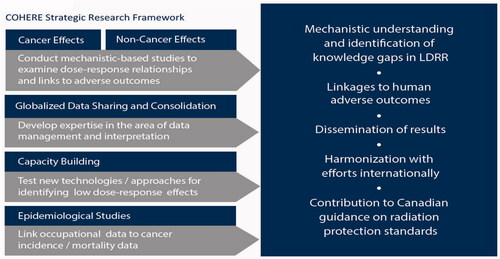
Understanding cancer risks from exposure to ionizing radiation at low doses and dose rates,
Understanding non-cancer risks from exposure to ionizing radiation at low doses and dose rates,
Leveraging globalized data-sharing initiatives to consolidate already-existing knowledge and identify knowledge gaps,
Validating emerging technologies and modernized approaches to support risk assessment,
Linking low dose radiation exposure data to cancer incidence and mortality effects.
Consistent with the aims of the international community, COHERE will also focus on risk communication. A communication strategy will guide the promotion of the program, the production of tools for the dissemination of information, and help with encapsulation of these tools in how the CNSC and HC regularly interact with the members of the public, Indigenous peoples, and other stakeholders.
Priority research areas
COHERE is aiming to align priority areas to that of the NEA’s initiative for the global coordination of low dose radiation research, the international [e.g. Multidisciplinary Effects of Low Dose Initiative (MELODI) and the ICRP] as well as the national priorities.
To facilitate this, COHERE has developed a strategic research agenda (SRA) to capture research priorities to address in the long term. This SRA will serve as a framework upon which COHERE can build collaborative relationships and develop a more coordinated approach both nationally and internationally. COHERE’s SRA has identified five key research themes that best align with COHERE’s priorities ():
Table 1. The five research themes under the COHERE SRA.
Theme area 1 cancer effects
Three radionuclides: radon, tritium, and uranium are of particular interest in Canada due to the nature of the nuclear industry. While external exposures are also of interest, internal exposures to radon, tritium, and uranium are at the forefront of concern for Canadians including radiation workers and members of the public and Indigenous peoples.
Radon (Rn-222) is a naturally occurring radioactive gas and is the second leading cause of lung cancer after smoking (International Agency for Research on Cancer Citation2012). It is estimated that 16% of annual lung cancer deaths are attributable to residential radon exposure in Canada. Accordingly, the investigation of lung cancer risk from radon gas exposure in occupational and residential exposure scenarios is of interest. The work in this area will improve mechanistic knowledge on chronic low dose radon exposure, to reduce the uncertainty around the dose-modeling of radon progeny.
Tritium exposure can pose a health risk if ingested through drinking water or food, or if inhaled or absorbed through the skin in large quantities. Issues raised in Canada include handling, control, and releases of tritium, tritium drinking water limits, tritium’s fate in the environment, and the health effects of tritium exposure (Canadian Nuclear Safety Commission Citation2010; Bundy Citation2012). The CNSC conducted research to address these concerns and increased our scientific understanding of tritium. Tritium research questions of interest include the mechanistic and biokinetic nature of HTO (tritiated water) and OBT (organically bound tritium) ingested and incorporated in different forms.
Uranium is widespread in nature; Canadians primarily receive uranium exposure through drinking water and food. Natural events, such as forest fires can release uranium into the environment as with its release from rock and soil through natural processes. Artificial sources of uranium, largely stemming from the nuclear energy industry, also contribute to the release of uranium to the environment and contribute to the occupational exposures of workers. Although the health effects of acute (and very high) exposures to natural and depleted uranium exposures including nephrotoxicity are well-known, the health risks from long-term chronic consumption of uranium are less understood and deserve further investigation.
Theme area 2 non-cancer effects
In 2011, the International Commission of Radiation Protection (ICRP) recommended a reduction to the equivalent dose limit for the lens of an eye, based on new scientific evidence (International Commission on Radiological Protection Citation2012; International Atomic Energy Agency Citation2014). The evidence indicated that tissue reactions for the lens of an eye have dose thresholds that are or might be, lower than previously considered. In response to the scientific evidence published to date, several countries are considering or implementing a reduction to the worker dose limit for the lens of an eye. In Canada, effective 1 January 2021, the equivalent dose limit to the lens of an eye for a nuclear energy worker (NEW) will be decreased from the current limit of 150 mSv to 50 mSv in a one-year dosimetry period. Stakeholders have concerns about the scientific evidence behind the recommendations. Many questions remain about the mechanism of cataract development. For these reasons, research on the development of cataracts is a priority for COHERE. Other non-cancer endpoints are currently being explored under the subsequent research theme using the adverse outcome pathway approach.
Theme area 3 globalized data sharing and consolidation
Decades of research to understand the human health effects from exposure to sources of ionizing radiation have produced vast amounts of data. There is a need to determine the best approach to harness these large and diverse datasets, identify knowledge gaps, and develop directed research studies that can better support human health risk assessment and management (Schofield et al. Citation2019; Zander et al. Citation2019). Systematic methodologies can identify research gaps and feed data into the development of Adverse Outcome Pathways in areas relevant to radiation risk assessment. These reviews will also provide a framework to consolidate the data. COHERE will explore these methodologies as a means to vet and filter data and bridge the gap between radiobiological studies with epidemiology, a long-held goal of the radiation protection community (Ruhm et al. Citation2017). Adverse outcome pathways that are of interest include both cancer (lung cancer) and non-cancer (cardiovascular, cognitive, cataracts, and bone loss) outcomes.
Theme area 4 capacity building
COHERE will explore emerging technologies and approaches that have shown promise in other disciplines for their sensitivity to detect radiation effects at low doses and dose rates of exposure. These approaches may also provide new avenues for the investigation of non-targeted and targeted biological effects and individual susceptibility. Examples include:
chromosome conformation technologies, approaches that take into account the spatial organization of DNA when analyzing gene-gene interaction,
phenotypic assays, that can quantify disease phenotype changes in cells (to better understand the underlying biology with the aim to identify biomarkers), and
benchmark dose modeling to quantitatively analyze and interpret dose-response data.
Theme area 5 epidemiological studies
New studies on various populations, such as medical, occupational, and environmental exposures support the atomic bomb findings that as radiation dose increases, so too does radiation-induced cancer risk. Additional linkage of epidemiological cohorts of people with chronic low dose occupational exposures to cancer incidence and mortality outcomes, pooling of cohorts, and their analyses, will better refine health risk estimates at low doses and low dose rates. COHERE will also investigate emerging mathematical approaches related to confounders in the context of epidemiological studies
Discussion and conclusions
Radiobiological studies can strengthen epidemiological data by providing insight into biological mechanisms that can help reduce uncertainties. Much research is needed to help understand how early biological events in the body may translate to observed health effects and disease. COHERE will help integrate mechanistic and population-based data to support radiation protection. It will primarily work toward the development of sensitive technologies (i.e. genomics and optical spectroscopy techniques) to detect subtle changes in the cellular environment following radiation exposure. It will also exploit tools and methodologies that inform chemical toxicology to help refine risk estimates and link them to phenotypic changes (i.e. AOPs and benchmark dose modeling; ). In addition, modeling approaches will help interpret and extrapolate the in vitro measures to epidemiological studies. Better understanding in this area will provide a more biologically meaningful basis for reliable health risk estimation that is essential for a robust system of radiation protection. Collaborative undertakings between the two federal agencies will facilitate better use of resources, expertise and enable effective responses to inquiries and concerns from Canadians. In the long term, this will strengthen the weight of evidence needed for policy development and improvement of regulation and decision-making to help modernize radiation protection practices.
Stakeholder engagement and coordination
COHERE will engage stakeholders and research partners within and outside Canada. The work will be of interest to, and support ongoing efforts of UNSCEAR, ICRP, NEA, and the European MELODI/ALLIANCE consortium.
Before a national radiation protection community can become involved with global coordination and collaboration, we need domestic coordination. MELODI, which developed an SRA to help guide LDRR in Europe, is an international community that has demonstrated effective coordination of LDRR (Salomaa et al. Citation2017) and has provided guidance for the formation of COHERE. Their SRA facilitates the development of research projects, but more importantly collaboration. Similarly, COHERE’s SRA is not limited to the federal government and the FNS&T workplan; it can serve as a basis for collaborative work with other government departments, academic researchers, and industry partners, creating a strong foundation for future national coordination. A step toward global coordination is the harmonization of federal government LDRR in Canada followed by potential national coordination, with stakeholder partners conducting independent and/or collaborative research. Currently, national coordination of LDRR in Canada does not exist, but some initiatives have strengthened this vision (e.g. FNS&T) (Atomic Energy Canada Limited 2020). Coordinating on a domestic level will strengthen the Canadian low dose research community and, indirectly, the broader radiation research community. A well-coordinated research network will demonstrate Canadian leadership and will allow for more effective collaboration and partnerships internationally. Knowledge of Canadian research and expertise is essential to the Government departments who contract research and represent Canada on an international stage (e.g. NEA CRPPH, UNSCEAR, etc.). It will also facilitate the inclusion of all Canadian research/researchers/infrastructure in international coordination efforts, such as NEA HLG LDR initiatives.
Next steps
Dialogue has begun between COHERE and external organizations involved in LDRR. The goal is to put forward an approach to involve the broader Canadian LDRR community and to assess its interest in national coordination and a potential framework. One benefit of national coordination will be the effective compilation of the broader Canadian LDRR as input into the global coordination efforts. Optimizing resources will increase research output and reduce unnecessary duplication of effort with development in the area of technology and mechanistic translational research. Further, COHERE is committed to improving the understanding of low-dose radiation health effects.
Conclusions
The Canadian Nuclear Safety Commission and Health Canada have a long history of conducting scientific research to support their mandates. COHERE is a first step toward coordinating LDRR activities between these organizations and will facilitate the integration of Government of Canada research into global collaborations. It also has the potential to serve as a foundational input into national LDRR coordination in Canada.
The COHERE work plan will be reviewed annually and focuses on joint activities to advance COHERE goals and the SRA. The current work plan includes:
Identifying and conducting joint projects to address research deficiencies in the area of LDRR without duplicating efforts nationally and internationally
Collaboration and activities with stakeholders to promote COHERE goals
Engaging with national and international community initiatives through working groups, conferences, meetings, etc.
Professional development and training in building complementary skillsets in different disciplines
Identifying, applying, and promoting emerging tools and techniques to inform and enhance radiation risk estimation, such as the Adverse Outcome Pathway ()
The progress of COHERE can be followed through ResearchGate (Canadian Nuclear Safety Commission 2020a) and the COHERE webpage on the CNSC website (Canadian Nuclear Safety Commission 2020b). Promotion and outreach will also be an ongoing activity, including communication and presentation at national and international forums (i.e. conferences, workshops, meetings, etc.).
Acknowledgments
The authors would like to acknowledge Katya Feder and Sami Qutob for editorial comments; and Annick Laporte for figure design.
Disclosure statement
The authors declare no conflict of interest.
Additional information
Notes on contributors
Vinita Chauhan
Dr. Vinita Chauhan is a Research Scientist at the Consumer and Clinical Radiation Protection Bureau of Health Canada. She is a Canadian Delegate on the High-Level Group on Low Dose Research (HLG-LDR) and Extended Advisory Group on Molecular Screening and Toxicogenomics of the Organisation for Economic Cooperation and Development and has recently been appointed as the co-chair of the newly formed RAD/CHEM AOP joint topical group through the HLG-LDR committee.
Julie Leblanc
Dr. Julie Leblanc is a Radiation Biologist with the Canadian Nuclear Safety Commission. Her research focuses on the potential health effects of exposure to low doses of ionizing radiation. She is a Canadian Delegate on the High-Level Group on Low Dose Research and International Commission on Radiological Protection mentee and member of task group 111.
Baki Sadi
Dr. Baki Sadi is a Research Scientist at the Radiation Protection Bureau.
Julie Burtt
Julie Burtt is a Radiation Biologist with the Canadian Nuclear Safety Commission. Her research focuses on the potential health effects of exposure to low doses of ionizing radiation. She has experience as an advisor for Canada to the United Nations Scientific Committee on the Effects of Atomic Radiation (UNSCEAR) as well as with the UNSCEAR Secretariat. She also serves as a Canadian representative on the National Energy Agency’s Expert Group on Non-radiological Public Health Aspects of Radiation Emergency Planning and Response.
Kiza Sauvé
Kiza Sauvé is the Director of the Health Sciences and Environmental Compliance Division at the Canadian Nuclear Safety Commission (CNSC). With a wide range of expertise including in wastes and decommissioning, and environmental and radiation protection and assessment, she provides leadership and oversight to her team. She is a member of the Leading Group of Women in Nuclear (WiN) Canada, Eastern Ontario Chapter.
Rachel Lane
Dr. Rachel Lane is a Radiation and Health Sciences Specialist (epidemiology) with the Canadian Nuclear Safety Commission and an adjunct professor at Carleton University. Her research focuses on the health effects of occupational and environmental radiation exposure. Dr. Lane has been an advisor for Canada to the United Nations Scientific Committee on the Effects of Atomic Radiation (UNSCEAR) since 2006.
Kristi Randhawa
Kristi Randhawa is a Radiation and Health Sciences Officer (epidemiology) with the Canadian Nuclear Safety Commission. Her research involves studying the health effects of ionizing radiation exposure, particularly exposure to low doses of radiation or radiation delivered at low dose rates. She works as a coordinator and advisor for various research projects and working groups including for the International Society of Radiation Epidemiology and Dosimetry (ISoRED).
Ruth Wilkins
Dr. Ruth Wilkins is the Division Chief of the Ionizing Health Sciences Division at Health Canada. She is an alternate representative of the United Nations Scientific Committee on the Effects of Atomic Radiation (UNSCEAR).
Debora Quayle
Debora Quayle is section head of the Radiation Health Assessment Division at Health Canada. She is a member of the Canadian Delegation on the OECD Nuclear Energy Agency Committee of Radiological Protection and Public Health.
References
- Allen CH, Kumar A, Qutob S, Nyiri B, Chauhan V, Murugkar S. 2018. Raman micro-spectroscopy analysis of human lens epithelial cells exposed to a low-dose-range of ionizing radiation. Phys Med Biol. 63(2):025002.
- Andrievski A, Wilkins RC. 2009. The response of gamma-H2AX in human lymphocytes and lymphocytes subsets measured in whole blood cultures. Int J Radiat Biol. 85(4):369–376.
- Ankley GT, Bennett RS, Erickson RJ, Hoff DJ, Hornung MW, Johnson RD, Mount DR, Nichols JW, Russom CL, Schmieder PK, et al. 2010. Adverse outcome pathways: a conceptual framework to support ecotoxicology research and risk assessment. Environ Toxicol Chem. 29(3):730–741.
- Ashmore JP, Krewski D, Zielinski JM, Jiang H, Semenciw R, Band PR. 1998. First analysis of mortality and occupational radiation exposure based on the National Dose Registry of Canada. Am J Epidemiol. 148(6):564–574.
- Ashmore PJ, Gentner NE, Osborne RV. 2010. Incomplete data on the Canadian cohort may have affected the results of the study by the International Agency for Research on Cancer on the radiogenic cancer risk among nuclear industry workers in 15 countries. J Radiol Prot. 30:121–29.
- Atkins CG, Buckley K, Chen D, Schulze HG, Devine DV, Blades MW, Turner RF. 2016. Raman spectroscopy as a novel tool for monitoring biochemical changes and inter-donor variability in stored red blood cell units. Analyst. 141(11):3319–3327.
- Atomic Energy Canada Limited (AECL). 2020. Federal nuclear science and technology work plan. [accessed]. https://www.aecl.ca/science-technology/federal-science-and-technology-work-plan/
- Awadalla P, Boileau C, Payette Y, Idaghdour Y, Goulet JP, Knoppers B, Hamet P, Laberge C, Project CA, CARTaGENE Project. 2013. Cohort profile of the CARTaGENE study: Quebec's population-based biobank for public health and personalized genomics. Int J Epidemiol. 42(5):1285–1299.
- Ayotte P, Levesque B, Gauvin D, McGregor RG, Martel R, Gingras S, Walker WB, Letourneau EG. 1998. Indoor exposure to 222Rn: a public health perspective. Health Phys. 75(3):297–302.
- Bahia S, Blais E, Murugkar S, Chauhan V, Kumarathasan P. 2018. Oxidative and nitrative stress-related changes in human lens epithelial cells following exposure to X-rays. Int J Radiat Biol. 94(4):366–373.
- Baker MJ, Hussain SR, Lovergne L, Untereiner V, Hughes C, Lukaszewski RA, Thiefin G, Sockalingum GD. 2016. Developing and understanding biofluid vibrational spectroscopy: a critical review. Chem Soc Rev. 45(7):1803–1818.
- Beaton LA, Ferrarotto C, Marro L, Samiee S, Malone S, Grimes S, Malone K, Wilkins RC. 2013. Chromosome damage and cell proliferation rates in in vitro irradiated whole blood as markers of late radiation toxicity after radiation therapy to the prostate. Int J Radiat Oncol Biol Phys. 85(5):1346–1352.
- Beaton LA, Marro L, Malone S, Samiee S, Grimes S, Malone K, Wilkins RC. 2013. Investigating γ H2AX as a biomarker of radiosensitivity using flow cytometry methods. ISRN Radiol. 2013:704659.
- Beaton LA, Marro L, Samiee S, Malone S, Grimes S, Malone K, Wilkins RC. 2013. Investigating chromosome damage using fluorescent in situ hybridization to identify biomarkers of radiosensitivity in prostate cancer patients. Int J Radiat Biol. 89(12):1087–1093.
- Beaton-Green LA, Barr T, Ainsbury EA, Wilkins RC. 2016. Retrospective biodosimetry of an occupational overexposure-case study. Radiat Prot Dosimetry. 172(1–3):254–259.
- Beaton-Green LA, Rodrigues MA, Lachapelle S, Wilkins RC. 2017. Foundations of identifying individual chromosomes by imaging flow cytometry with applications in radiation biodosimetry. Methods. 112:18–24.
- Berger AJ, Koo TW, Itzkan I, Horowitz G, Feld MS. 1999. Multicomponent blood analysis by near-infrared Raman spectroscopy. Appl Opt. 38(13):2916–2926.
- Bergholt MS, Hassing S. 2009. Quantification of C-reactive protein in human blood plasma using near-infrared Raman spectroscopy. Analyst. 134(10):2123–2127.
- Bonifacio A, Cervo S, Sergo V. 2015. Label-free surface-enhanced Raman spectroscopy of biofluids: fundamental aspects and diagnostic applications. Anal Bioanal Chem. 407(27):8265–8277.
- Brunner H, Sussner H. 1973. Resonance Raman scattering on haemoglobin. Biochim Biophys Acta. 310(1):20–31.
- Brunner H. 1974. Identification of the iron-ligand vibration of oxyhemoglobin. Naturwissenschaften. 61(3):129–129.
- Bundy K. 2012. Radiation effects of tritium. In: Meyers R, editor. Encyclopedia of sustainability science and technology. New York (NY): Springer Science.
- Burtt J, Ilin M, Lane R, Thompson P, Phaneuf M, Reinhardt P. 2011. Use of a weight of evidence approach to determine the likelihood of adverse effects on human health from the presence of uranium facilities in Port Hope. JEP. 2(9):1149–1161.
- Burtt JJ, Rickard M, McAllister A, Bergman L, Zablotska LB. 2020. Projecting thyroid cancer risk to the general public from radiation exposure following hypothetical severe nuclear accidents in Canada. J Radiol Prot. 40:1091–1110.
- Burtt JJ, Thompson PA, Lafrenie RM. 2016. Non-targeted effects and radiation-induced carcinogenesis: a review. J Radiol Prot. 36(1):R23–R35.
- Canadian Nuclear Safety Commission. 2010. Health effects, dosimetry and radiological protection of tritium. Part of the tritium studies project. INFO-0799.
- Canadian Nuclear Safety Commission. 2020a. Canadian Organization on Health Effects from Radiation Exposure (COHERE) Researchgate project. [accessed]. https://www.researchgate.net/project/Federal-Organizations-for-Low-Dose-Radiation-Research-COHERE
- Canadian Nuclear Safety Commission. 2020b. Canadian Organization on Health Effects from Radiation Exposure (COHERE) webpage. [accessed]. http://www.nuclearsafety.gc.ca/eng/resources/research/cohere/index.cfm
- Canadian Nuclear Safety Commission. 2020c. Learning portal. [accessed]. http://www.nuclearsafety.gc.ca/eng/resources/learning-portal/index.cfm
- Canadian Nuclear Safety Commission. 2020d. Research and support program. [accessed]. https://nuclearsafety.gc.ca/eng/resources/research/research-and-support-program/index.cfm
- Cardis E, Gilbert ES, Carpenter L, Howe G, Kato I, Armstrong BK, Beral V, Cowper G, Douglas A, Fix J. 1995. Effects of low doses and low dose rates of external ionizing radiation: cancer mortality among nuclear industry workers in three countries. Radiat Res. 142(2):117–132.
- Cardis E, Vrijheid M, Blettner M, Gilbert E, Hakama M, Hill C, Howe G, Kaldor J, Muirhead CR, Schubauer-Berigan M, et al. 2007. The 15-country collaborative study of cancer risk among radiation workers in the nuclear industry: estimates of radiation-related cancer risks. Radiat Res. 167(4):396–416.
- Cardis E, Vrijheid M, Blettner M, Gilbert E, Hakama M, Hill C, Howe G, Kaldor J, Muirhead CR, Schubauer-Berigan M, et al. 2005. Risk of cancer after low doses of ionising radiation: retrospective cohort study in 15 countries. BMJ. 331(7508):77.
- Chauhan V, Adam N, Kuo B, Williams A, Yauk CL, Wilkins R, Stainforth R. 2021. Meta-analysis of transcriptomic datasets using benchmark dose modeling shows value in supporting radiation risk assessment. Int J Radiat Biol. 97(1):31–49.
- Chauhan V, Howland M, Wilkins R. 2014. Identification of gene-based responses in human blood cells exposed to alpha particle radiation. BMC Med Genomics. 7:43.
- Chauhan V, Kuo B, McNamee JP, Wilkins RC, Yauk CL. 2016. Transcriptional benchmark dose modeling: Exploring how advances in chemical risk assessment may be applied to the radiation field. Environ Mol Mutagen. 57(8):589–604.
- Chauhan V, Rowan-Carroll A, Gagne R, Kuo B, Williams A, Yauk CL. 2019. The use of in vitro transcriptional data to identify thresholds of effects in a human lens epithelial cell-line exposed to ionizing radiation. Int J Radiat Biol. 95(2):156–169.
- Chauhan V, Said Z, Daka J, Sadi B, Bijlani D, Marchetti F, Beaton D, Gaw A, Li C, Burtt J, et al. 2019. Is there a role for the adverse outcome pathway framework to support radiation protection? Int J Radiat Biol. 95(2):225–232.
- Chauhan V, Sherman S, Said Z, Yauk CL, Stainforth R. 2021. A case example of a radiation-relevant adverse outcome pathway to lung cancer. Int J Radiat Biol. 97(1):68–84.
- Chauhan V, Stricklin D, Cool D. 2021. The integration of the adverse outcome pathway framework to radiation risk assessment. Int J Radiat Biol. 97(1):60–67.
- Chauhan V, Villeneuve D, Cool D. 2021. Collaborative efforts are needed among the scientific community to advance the adverse outcome pathway concept in areas of radiation risk assessment. Int J Radiat Biol. 97:6:815–823.
- Chen J, Moir D, Lane R, Thompson P. 2013. An ecological study of cancer incidence in Port Hope, Ontario from 1992 to 2007. J Radiol Prot. 33(1):227–242.
- Chen J, Moir D. 2010. An updated assessment of radon exposure in Canada. Radiat Prot Dosimetry. 140(2):166–170.
- Chen J, Timmins R, Verdecchia K, Sato T. 2009. An estimation of Canadian population exposure to cosmic rays. Radiat Environ Biophys. 48(3):317–322.
- Chen J. 2005a. Canadian individual risks of radon-induced lung cancer for different exposure profiles. Can J Public Health. 96(5):360–363.
- Chen J. 2005b. Estimated risks of radon-induced lung cancer for different exposure profiles based on the new EPA model. Health Phys. 88(4):323–333.
- Chen J. 2019. Risk assessment for radon exposure in various indoor environments. Radiat Prot Dosimetry. 185(2):143–150.
- Dummer TJB, Awadalla P, Boileau C, Craig C, Fortier I, Goel V, Hicks JMT, Jacquemont S, Knoppers BM, Le N, with the CPTP Regional Cohort Consortium, et al. 2018. The Canadian Partnership for Tomorrow Project: a pan-Canadian platform for research on chronic disease prevention. CMAJ. 190(23):E710–E717.
- Flegal FN, Devantier Y, Marro L, Wilkins RC. 2012. Validation of QuickScan dicentric chromosome analysis for high throughput radiation biological dosimetry. Health Phys. 102(2):143–153.
- Gribbin MA, Weeks JL, Howe GR. 1993. Cancer mortality (1956–1985) among male employees of Atomic Energy of Canada Limited with respect to occupational exposure to external low-linear-energy-transfer ionizing radiation. Radiat Res. 133(3):375–380.
- Gueguen Y, Priest ND, Dublineau I, Bannister L, Benderitter M, Durand C, Ebrahimian TG, Gregoire E, Grison S, Ibanez C, et al. 2018. In vivo animal studies help achieve international consensus on standards and guidelines for health risk estimates for chronic exposure to low levels of tritium in drinking water. Environ Mol Mutagen. 59(7):586–594.
- Hansson B, Allen CH, Qutob S, Behr B, Nyiri B, Chauhan V, Murugkar S. 2019. Development of a flow cell based Raman spectroscopy technique to overcome photodegradation in human blood. Biomed Opt Express. 10(5):2275–2288.
- Health Physics Special Issue: Canadian Radiation Protection and Research. 2019. Health physics. [accessed 2021]. https://journals.lww.com/health-physics/toc/2019/09000
- Huang YX, Wu ZJ, Mehrishi J, Huang BT, Chen XY, Zheng XJ, Liu WJ, Luo M. 2011. Human red blood cell aging: correlative changes in surface charge and cell properties. J Cell Mol Med. 15(12):2634–2642.
- IAEA. 2014. Radiation protection and safety of radiation sources: international basic safety standards. Vienna: International Atomic Energy Agency. https://www.iaea.org/publications/8930/radiation-protection-and-safety-of-radiation-sources-international-basic-safety-standards
- ICRP. 2007. The 2007 Recommendations of the International Commission on Radiological Protection. ICRP Publication 103. Ann. ICRP. 37(2–4).
- ICRU 2019. Methods for initial-phase assessment of individual doses following acute exposure to ionizing radiation, ICRU report No. 94. Bethesda (MD): International Commission on Radiation Units and Measurements.
- International Agency for Research on Cancer. 2012. Radiation: A review of human carcinogens. Lyon: World Health Organization.
- ISO 17099. 2014. Radiological protection – performance criteria for laboratories using the cytokinesis block micronucleus (CBMN) assay in peripheral blood lymphocytes for biological dosimetry.
- ISO 19238 2014. Radiological protection – performance criteria for service laboratories performing biological dosimetry by cytogenetics.
- ISO 20046 2019. Radiological protection – performance criteria for laboratories using fluorescence in situ hybridization (FISH) translocation assay for assessment of exposure to ionizing radiation.
- Jahn IJ, Zukovskaja O, Zheng XS, Weber K, Bocklitz TW, Cialla-May D, Popp J. 2017. Surface-enhanced Raman spectroscopy and microfluidic platforms: challenges, solutions and potential applications. Analyst. 142(7):1022–1047.
- Lane R, Dagher E, Burtt J, Thompson P. 2013. Radiation exposure and cancer incidence (1990 to 2008) around nuclear power plants in Ontario. JEP. 4(09):888–913.
- Lane RS, Frost SE, Howe GR, Zablotska LB. 2010. Mortality (1950–1999) and cancer incidence (1969–1999) in the cohort of Eldorado uranium workers. Radiat Res. 174(6A):773–785.
- Leblanc JE, Burtt JJ. 2019. Radiation biology and its role in the Canadian Radiation Protection Framework. Health Phys. 117(3):319–329.
- Letourneau EG. 1985. Limitation of exposure to natural radioactivity in Canada. Sci Total Environ. 45:647–656.
- Levesque B, Gauvin D, McGregor RG, Martel R, Gingras S, Dontigny A, Walker WB, Lajoie P, Letourneau E. 1997. Radon in residences: influences of geological and housing characteristics. Health Phys. 72(6):907–914.
- Macdonald CR, Elkin BT, Tracy BL. 2007. Radiocesium in caribou and reindeer in northern Canada, Alaska and Greenland from 1958 to 2000. J Environ Radioact. 93(1):1–25.
- McCullough RS, Letourneau EG, Waight PJ. 1981. A four factor model for estimating human radiation exposure to radon daughters in the home. Health Phys. 40(3):299–305.
- McNamee JP, Flegal FN, Greene HB, Marro L, Wilkins RC. 2009. Validation of the cytokinesis-block micronucleus (CBMN) assay for use as a triage biological dosimetry tool. Radiat Prot Dosimetry. 135(4):232–242.
- National Academies of Sciences, Engineering, and Medicine, Division on Earth and Life Studies, Nuclear and Radiation Studies Board. 2019. The future of low dose radiation research in the United States: proceedings of a symposium. Washington (DC): National Academies Press.
- National Research Council. 1999. Health effects of exposure to radon: BEIR VI. Washington, DC: The National Academies Press.
- Navaranjan G, Berriault C, Do M, Villeneuve PJ, Demers PA. 2016. Cancer incidence and mortality from exposure to radon progeny among Ontario uranium miners. Occup Environ Med. 73(12):838–845.
- Perko T, Van Oudheusden M, Turcanu C, Pölzl-Viol C, Oughton D, Schieber C, Schneider T, Zölzer F, Mays C, Martell M, et al. 2019. Towards a strategic research agenda for social sciences and humanities in radiological protection. J Radiol Prot. 39(3):766–784.
- Premasiri WR, Lee JC, Ziegler LD. 2012. Surface-enhanced Raman scattering of whole human blood, blood plasma, and red blood cells: cellular processes and bioanalytical sensing. J Phys Chem B. 116(31):9376–9386.
- Qu JY, Wilson BC, Suria D. 1999. Concentration measurements of multiple analytes in human sera by near-infrared laser Raman spectroscopy. Appl Opt. 38(25):5491–5498.
- Qutob SS, Chauhan V, Kuo B, Williams A, Yauk CL, McNamee JP, Gollapudi B. 2018. The application of transcriptional benchmark dose modeling for deriving thresholds of effects associated with solar-simulated ultraviolet radiation exposure. Environ Mol Mutagen. 59(6):502–515.
- Radiation Research Special Issue: Snolab. 2017. Radiation research. [accessed]. https://www.radres.org/news/374563/Radiation-Research-Table-of-Contents-October-2017–Part-2.htm
- Rage E, Richardson DB, Demers PA, Do M, Fenske N, Kreuzer M, Samet J, Wiggins C, Schubauer-Berigan MK, Kelly-Reif K, et al. 2020. PUMA – pooled uranium miners analysis: cohort profile. Occup Environ Med. 77(3):194–200.
- Repussard J. 2019. Understanding low dose radiation exposure effects: MELODI's views on developing international cooperation. Int J Radiat Biol. 95(10):1354–1357.
- Rodrigues MA, Probst CE, Beaton-Green LA, Wilkins RC. 2016. Optimized automated data analysis for the cytokinesis-block micronucleus assay using imaging flow cytometry for high throughput radiation biodosimetry. Cytometry A. 89(7):653–662.
- Ruhm W, Eidemuller M, Kaiser JC. 2017. Biologically-based mechanistic models of radiation-related carcinogenesis applied to epidemiological data. Int J Radiat Biol. 93(10):1093–1117.
- Rusciano G, De Luca AC, Pesce G, Sasso A. 2008. Raman tweezers as a diagnostic tool of hemoglobin-related blood disorders. Sensors. 8(12):7818–7832.
- Salomaa S, Jourdain JR, Kreuzer M, Jung T, Repussard J. 2017. Multidisciplinary European low dose initiative: an update of the MELODI program. Int J Radiat Biol. 93(10):1035–1039.
- Schofield PN, Kulka U, Tapio S, Grosche B. 2019. Big data in radiation biology and epidemiology; an overview of the historical and contemporary landscape of data and biomaterial archives. Int J Radiat Biol. 95(7):861–878.
- Sont WN, Zielinski JM, Ashmore JP, Jiang H, Krewski D, Fair ME, Band PR, Letourneau EG. 2001. First analysis of cancer incidence and occupational radiation exposure based on the National Dose Registry of Canada. Am J Epidemiol. 153(4):309–318.
- Spiro TG, Strekas TC. 1974. Resonance Raman spectra of heme proteins. Effects of oxidation and spin state. J Am Chem Soc. 96(2):338–345.
- Strekas TC, Spiro TG. 1972. Hemoglobin: resonance Raman spectra. Biochim Biophys Acta. 263(3):830–833.
- Sweeney E, Cui Y, DeClercq V, Devichand P, Forbes C, Grandy S, Hicks JMT, Keats M, Parker L, Thompson D, et al. 2017. Cohort profile: the Atlantic Partnership for Tomorrow's Health (Atlantic PATH) Study. Int J Epidemiol. 46(6):1762–1763.
- Thomas PA, Fisenne I, Chorney D, Baweja AS, Tracy BL. 2001. Human absorption and retention of polonium-210 from Caribou meat. Radiat Prot Dosimetry. 97(3):241–250.
- Thomas RS, Philbert MA, Auerbach SS, Wetmore BA, Devito MJ, Cote I, Rowlands JC, Whelan MP, Hays SM, Andersen ME, et al. 2013. Incorporating new technologies into toxicity testing and risk assessment: moving from 21st century vision to a data-driven framework. Toxicol Sci. 136(1):4–18.
- Thompson PA, Hamlat MS, Lane R, Mihok S, Reinhardt P, Bundy K. 2011. Revue de l’état des connaissances des effets du tritium sur la santé et l’environnement au Canada–un outil pour orienter la surveillance réglementaire [Review of the knowledge available to date on the effects of tritium exposure on health and the environment in Canada–a tool to guide regulatory compliance monitoring]. Radioprotection. 46(4):511–531.
- Tracy BL, Kramer GH, Zielinski JM, Jiang H. 1997. Radiocesium body burdens in residents of northern Canada from 1963–1990. Health Phys. 72(3):431–442.
- Tracy BL, Krewski D, Chen J, Zielinski JM, Brand KP, Meyerhof D. 2006. Assessment and management of residential radon health risks: a report from the health Canada radon workshop. J Toxicol Environ Health A. 69(7):735–758.
- Tracy BL, Prantl FA, Quinn JM. 1983. Transfer of 226Ra, 210Pb and uranium from soil to garden produce: assessment of risk. Health Phys. 44(5):469–477.
- United Nations. 2008. Sources and effects of ionizing radiation. UNSCEAR 2006 Report to the General Assembly with Scientific Annexes. Volume I, Annex A: Epidemiological studies of radiation and cancer. New York (NY).
- United Nations. 2009. Effects of ionizing radiation. United Nations Scientific Committee on the Effects of Atomic Radiation. UNSCEAR 2006 report to the general assembly with scientific annexes. New York (NY).
- Villeneuve PJ, Lane RS, Morrison HI. 2007. Coronary heart disease mortality and radon exposure in the Newfoundland fluorspar miners' cohort, 1950–2001. Radiat Environ Biophys. 46(3):291–296.
- Wang Y, Bannister LA, Sebastian S, Le Y, Ismail Y, Didychuk C, Richardson RB, Flegal F, Paterson LC, Causey P, et al. 2019. Low-dose radiobiology program at Canadian nuclear laboratories: past, present, and future. Int J Radiat Biol. 95(10):1361–1371.
- Weiss W, Yonekura Y. 2019. International co-operation in biological research to address low dose radiation risk: Osaka call-for-action. Int J Radiat Biol. 95(10):1351–1353.
- Wilkins RC, Rodrigues MA, Beaton-Green LA. 2017. The application of imaging flow cytometry to high-throughput biodosimetry. Genome Integr. 8(1):7–7.
- Wood BR, Hammer L, Davis L, McNaughton D. 2005. Raman microspectroscopy and imaging provides insights into heme aggregation and denaturation within human erythrocytes. J Biomed Opt. 10(1):14005.
- Wu Y, Huang YX, Kang LL, Wu ZJ, Luo M. 2010. Effect of pH on molecular constitution and distribution of hemoglobin in living erythrocyte. Biopolymers. 93(4):348–354.
- Yan F, Vo-Dinh T. 2007. Surface-enhanced Raman scattering detection of chemical and biological agents using a portable Raman integrated tunable sensor. Sens Actuators B. 121(1):61–66.
- Yang L, Allen BC, Thomas RS. 2007. BMDExpress: a software tool for the benchmark dose analyses of genomic data. BMC Genomics. 8:387.
- Zablotska LB, Ashmore JP, Howe GR. 2004. Analysis of mortality among Canadian nuclear power industry workers after chronic low-dose exposure to ionizing radiation. Radiat Res. 161(6):633–641.
- Zablotska LB, Lane RS, Thompson PA. 2014. A reanalysis of cancer mortality in Canadian nuclear workers (1956–1994) based on revised exposure and cohort data. Br J Cancer. 110(1):214–223.
- Zamora ML, Tracy BL, Zielinski JM, Meyerhof DP, Moss MA. 1998. Chronic ingestion of uranium in drinking water: a study of kidney bioeffects in humans. Toxicol Sci. 43(1):68–77.
- Zamora ML, Zielinski JM, Moodie GB, Falcomer RA, Hunt WC, Capello K. 2009. Uranium in drinking water: renal effects of long-term ingestion by an aboriginal community. Arch Environ Occup Health. 64(4):228–241.
- Zander A, Paunesku T, Woloschak G. 2019. Radiation databases and archives – examples and comparisons. Int J Radiat Biol. 95(10):1378–1389.