Abstract
The protein digestion kinetics of goat milk infant formula (GMF) is previously shown to be more comparable to that of human milk (HM) than cow milk infant formula (CMF). To evaluate whether gastric behaviour contributes to differences in protein digestion kinetics, fresh HM, a GMF and a CMF were subjected to in vitro gastric digestion simulating infant conditions. Coagulation behaviour, particle size distribution and viscosity of the digesta were evaluated. After centrifugation of the digesta, total solids and protein distribution, and protein hydrolysis in the cream, serum and pellet fraction were investigated. The GMF and CMF were in general similar with respect to physicochemical and protein breakdown properties. However, a number of notable differences in physicochemical behaviour were observed, which may contribute to faster initial protein digestion of GMF. HM behaved differently from both formulas. These differences provide new insights into the possibilities for improvement of infant formulas.
Introduction
Nutrition in early life is crucial for both short-term and long-term health outcomes (Koletzko et al. Citation2017). Human milk (HM) is in general the ideal food for infants and the ‘gold’ standard for the design of infant formulae (IF). The chemical composition of IF and perhaps more realistically, the performance of IF, should be as similar as possible to those of HM in supporting infant growth and development (Lönnerdal Citation2014). This may be achieved through means including modifications of concentrations, chemical structure and sources of nutrients in IF, adding components with bioactivities similar to those in HM and optimisation of IF production process. Physicochemical differences between HM and the mammalian milk used for production of IF, as well as the processing of IF influences bioavailability and metabolic kinetics of their nutrients in infants. In an attempt to design an IF with its performance as close as possible to HM, understanding digestion and absorption of the nutrients in HM and IF in the infant gastrointestinal tract is essential. Particularly, protein digestion and absorption is critical for growth and health of infants. (Nelson and Kliegman Citation2011; Michaelsen and Greer Citation2014).
IF based on cow milk (CMF) is currently the most available and used alternative to HM when breastfeeding is not an option. Nevertheless, consumer interest for IF based on milk from other non-bovine animal species is increasing. Goat milk-based IF (GMF) has been shown to be a safe and efficacious alternative to CMF, providing adequate growth and being well tolerated in infants (Zhou et al. Citation2014).
Differences in protein composition are described among human, cow and goat milk. The casein to whey protein ratio is around 40:60 for HM and 80:20 for cow and goat milk. In HM, approximately 80% of total caseins is β- and κ-casein, with absence or a very low level of α-casein. Concentrations of αs1-casein in goat milk vary from zero to 25% of total protein, depending on the genetic polymorphisms of the goats. In contrast, αs1-casein in cow milk tends to be more consistent at an average 25% of total protein. α-Lactalbumin is the principal whey protein in HM. β-Lactoglobulin is absent in HM, while it is the principal whey protein in cow and goat milk, contributing to ∼15% of the total protein. Moreover, HM has higher levels of lactoferrin and some minor proteins (e.g. osteopontin and immunoglobulins) than cow and goat milk (Boland and Singh Citation2019; Prosser Citation2021).
Various in vitro models, either static or dynamic, have been developed to study digestion in infants (Nguyen et al. Citation2015). Compared to adults, Infant gastric condition is less acidic, with lower secretion level of pepsin (Bourlieu et al. Citation2014).
Protein from goat milk or GMF is reported to be more readily digested and has higher nitrogen utilisation than protein from cow milk as observed in in vitro (Pintado and Malcata Citation2000; Almaas et al. Citation2006; Hodgkinson et al. Citation2018; Ye et al. Citation2019) and animal studies (López-Aliaga et al. Citation2003; Ceballos et al. Citation2009). Digestion of goat and cow milk under simulated infant conditions generated peptides unique to goat or cow milk (Hodgkinson et al. Citation2019), possibly contributing to the reported differences in digestion characteristics between cow and goat milk .
Available studies indicate that differences in composition, physicochemical properties and structure of proteins between goat and cow milk or IF may contribute to the observed differences in protein digestion. A relatively lower casein content, in addition to a lower proportion of αs1-casein and higher proportion of β-casein in the casein fraction, as well as larger casein micelle size are believed to be responsible for the softer consistency of the curd of goat milk or GMF compared with the curd bovine milk or CMF when acidified (Wang et al. Citation2019). This has led to the hypothesis that goat milk may form softer and more loose aggregates in the human stomach compared to bovine milk, which is suggested to contribute to more efficient or rapid protein digestion compared to cow milk (Boland and Singh Citation2019).
We recently studied protein digestion of HM, a GMF and a CMF (both whey-dominant) in a validated in vitro dynamic gastrointestinal model that simulates infant digestion conditions (Maathuis et al. Citation2017). While the protein quality was not different between the HM, GMF and CMF, the kinetics of protein digestion of the GMF was shown to be more comparable to that of HM than that of the CMF. HM and the GMF had faster initial protein digestion than the CMF. In the same study, during the simulated gastric digestion, coagulated particles of the CMF persisted for a longer time than those of the GMF. Gastric digestion is the first phase of digestion of dietary protein, followed by digestion in the small intestine. We postulated that the differences in gastric digestion behaviour of HM, GMF and CMF protein (in particular caseins) may explain differences in digestion kinetics. The aim of the present study therefore was to verify this hypothesis by comparing the physicochemical changes and protein digestion of HM, a GMF and a CMF under in vitro simulated infant gastric conditions.
Materials and methods
Milk samples
HM samples were provided by four healthy donors between 4 and 12 weeks postpartum twice on two dates (T1 and T2). Written permission was obtained from the donors for participation. Fresh milk samples from the four donors were pooled quantitatively in a ratio of 1:1:1:1 and subjected to the in vitro digestion on the day of milk expression. Experiments were carried out in duplicate on day T1 and T2.
The GMF and CMF were supplied by Ausnutria B.V. (Zwolle, The Netherlands). The GMF and CMF were manufactured under similar GMP processing and were whey protein enhanced to a whey:casein ratio of 60:40. Infant formula powder was reconstituted at 45 °C in MilliQ water at a target level of 1.7% (w/w) protein.
The protein levels (total nitrogen × 6.25) were 1.11, 1.05 g/100 ml in the pooled HM samples at T1 and T2, and 1.80, 1.67 g/100 ml in GMF and CMF, respectively. The non-protein nitrogen (NPN) were 0.048, 0.048 g/100 ml for the duplicate HM, and 0.033 and 0.020 g/100 ml in GMF and CMF, respectively. This resulted in a true protein level ((total nitrogen-NPN) × 6.25) of 0.81, 0.75, 1.60, and 1.55 g/100 ml for the duplicate HM, GMF and CMF, respectively. The true protein levels were used in calculating protein recovery for BCA analysis. The total protein levels are used in calculating protein recovery for Dumas analysis.
In vitro gastric digestion
The in vitro gastric digestion was carried out at NIZO (Ede, The Netherlands) using a semi-dynamic digestion model adapted to simulate infant gastric conditions (Wijayanti et al. Citation2019). For in vitro gastric digestion of HM, GMF and CMF, a 100 mL bottle containing 0.83 g 30 mM HCl was placed in an agitating water bath set at 37 °C. A 20 mL milk sample (equilibrated at 37 °C) was fed into the bottle at a rate of 1 mL/min. Simultaneously, gastric juice (kept on ice) was added at a rate of 0.13 mL/min. Gastric juice contained 30 mM HCl, 293 U/mL porcine pepsin (Sigma) and 13.125 U/mL lipase from Rhizopus oryzae (Sigma).
To allow sampling at target pH values, buffering curves of samples were first determined. For this purpose, samples were mixed with different volumes of 30 mM HCl and the pH was determined. Based on the amount of 30 mM HCl required to reach a certain pH and the gastric juice pumping speed, the time point at which the sample should be taken was calculated. To reach a pH of 3.5, 0.8 g, 1.5 g and 1.6 g of gastric juice per g sample was added to HM, CMF and GMF in 110 min, 225 min and 240 min, respectively. Sampling was carried out at four time points during the experiment corresponding to target pH values 6.0, 5.5, 4.5, and 3.5 as calculated from the buffer curve. To inhibit pepsin activity after sampling, a pepstatin A (0.02 g pepstatin A (Sigma) was added at a level of 50 μL/10 mL of digested sample.
The in vitro gastric digestion of human milk, GMF and CMF were performed in duplicate. Samples were taken at each of the four pH values during the digestion. An aliquot of each sample was observed visually and under a stereomicroscope for phase separation and aggregates formation, and images were made. Subsequently, the samples were centrifuged at 4500 × g for 10 minutes. After centrifugation, the fractions (cream, serum, pellet) were separated and freeze dried. The same fractions of the duplicate digestion of the IFs were pooled. The freeze dried samples were stored at −22 °C for further analyses.
Particle size distribution
Particle size analysis was determined by light scattering (Malvern Mastersizer 2000, Malvern Instruments Ltd., Malvern, UK), with distilled water as the dispersion medium. Experimental parameters were as follows: temperature, 20 °C; particle refractive index 1.480; material absorption, 0.001.
Characterisation of the fractions after centrifugation
Chemical analyses
The total solids of each fraction were determined using a moisture analyser (Sartorius MA 150, Sartorius Weighing Technology GmbH, Goettingen, Germany). Total nitrogen (TN) and non-protein nitrogen (NPN) of each fraction and un-digested human milk and reconstituted IFs were determined using the bicinchoninic acid (BCA) protein assay (Pierce BCA Protein Assay Kit, Prod# 23225, Thermo Scientific, Rockford, IL, USA) by following the protocol of the producer.
SDS-PAGE under reducing conditions of each fraction
Samples were diluted with reverse osmosis water to a protein concentration of 2 mg/mL (as determined by BCA protein assay) and subsequently diluted 1:1 dilution with Laemmli buffer. For reducing conditions, the Laemmli buffer was supplemented with dithiothreitol to a level of 15 mg/mL. After heating at 90 °C for 10 min, sample (5 µL) was loaded on to the lanes of pre-cast 4-20% Tris-HCl gels in a Criterion cell system with 10xTris/Glycine/SDS Buffer (25 mM Tris, 192 mM glycine, 0.1% SDS, pH 8.3, Bio-Rad Laboratories) diluted 1:10 in reverse osmosis water. The gels were run with a constant voltage of 200 V for approximately 1 h and stained with Colloidal Coomassie Blue Stain and rinsed with water.
Rheological measurements
Measurements were performed using a cup-and-vane geometry (AR 2000, Waters TA Instruments, Leatherhead, UK). Seventy five grams of human milk or reconstituted IFs was added to the geometry and monitored for 3 h under steady shear conditions (75 s−1, 37 °C) at which the viscosity (plotted logarithmic) was recorded as a function of pH. After an initial 5 min of measuring, gastric juice (30 mM HCl, 293 U/mL porcine pepsin (Sigma) and 13.125 U/mL lipase) was added at a flow rate of 0.52 ml per minute. The end volume was 154 g.
An overview of the experiment flow is given in Figure 6 in the Supplementary materials.
Results
Fresh HM and reconstituted GMF, CMF were subjected to in vitro gastric digestion under simulated infant conditions. Samples were collected at pH 6.0, 5.5, 4.5, and 3.5. The time needed to reach different pH was given in Table 2 in the Supplementary materials.
Physicochemical changes during in vitro gastric digestion
Aggregates formation
During gastric digestion, milk protein will coagulate as a result of combined action of gastric enzymes and lowering pH by the gastric juice. Differences in physicochemical properties of milk proteins may result in different behaviour in aggregates formation, which may subsequently affect protein digestion. In the present study, during in vitro gastric digestion of HM, GMF and CMF, aggregates were observed visually starting from pH 6 and till pH 3.5 (stereomicroscopy images shown in ). Phase separation into a cream and a serum phase was visible by eye at pH 5.5 and pH 4.5 for GMF and CMF, respectively. This phase separation became more pronounced at lower pH in CMF; in GMF phase separation at pH 4.5 was more pronounced than that at pH 5.5, but was similar to that at pH 3.5. The serum phase of GMF appeared to be more turbid, and the cream phase thinner than that of CMF. HM showed slight phase separation starting from pH 4.5, with aggregates forming a sedimentation layer ().
Figure 1. Phase separation as observed in beakers (A) and aggregates as observed under stereomicroscope (B) during gastric digestion simulating infant conditions.
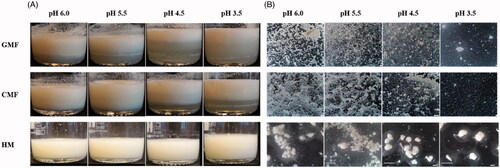
As observed under stereomicroscope, the aggregates of HM were larger than those of CMF and GMF, and surrounded by a translucent and viscous layer (). The aggregates of GMF were slightly larger, and had a coarser structure than those of CMF at pH 6.0 and pH 5.5. In GMF and CMF, the structure of the aggregates appeared close-knitted at pH 6.0 and became coarser when the pH was decreased from 6.0 to 4.5, the close-knit network disappeared at pH 4.5 in GMF and at pH 3.5 in CMF.
The duplicate samples of HM, GMF and CMF were comparable in aggregate formation behaviour. The results of one duplicate sample are shown in .
Particle size distribution
Determination of particle size distribution may provide information on aggregate formation and breakdown during the simulated gastric formation. The three undigested milk samples all displayed a monomodal particle size distribution, with particle size radius ranging between 1 and 10 µm and 0.1 and 1 µm for HM and the two IF, respectively. Upon in vitro gastric digestion, HM shifted to a bimodal distribution, with two size populations between 1 and 10 µm and 10 and 1000 µm. The two IF maintained a monomodal distribution, but shifted to a larger size distribution with particle size radius ranging between 2 and 100 µm. GMF exhibited a decrease in particle size starting from pH 4.5. For CMF, particle size distribution stayed largely unchanged over the pH range of 6.0, 5.5, and 4.5, but shifted to a smaller particle size distribution at pH 3.5 (). The duplicate samples of HM, GMF, and CMF were comparable in particle size distribution. The results of one duplicate sample are shown in .
Fractionation after centrifugation
Digesta collected during gastric digestion at pH 6.0, 5.5, 4.5, and 3.5 was centrifuged. Differences in physicochemical properties of the undigested milk samples may result in differences in fractionation of the digesta, including distribution of protein and fat in the different phases. Analysis of the fractions may give insights into gastric behaviour of HM, GMF and CMF during gastric digestion. After centrifugation, HM was separated into a cream, serum and pellet phase, whereas both IF formed a cream and serum phase, with hardly any pellet (<1% of the total solids).
The duplicate samples of HM, GMF and CMF were comparable in fractionation patterns. The same fractions of the duplicate samples of GMF or CMF were combined. . The fractions were freeze dried for further determination of total solids and protein, and analysis with SDS-PAGE.
Protein breakdown during in vitro gastric digestion
Distribution of total solids and protein in centrifugation fractions
During gastric digestion, fat destabilisation may occur and thereby influence the coagulation behaviour and digestion of protein. Distribution of total solids and protein in the cream, serum and pellet fractions can give insight on protein digestion and interaction of protein and fat during digestion.
The distribution of total solids in different fractions after centrifugation is summarised in . The HM had a lower proportion of solids in the cream phase and higher proportion of solids in the pellet phase in comparison with both IF. The proportion of solids in the serum phase was greater in HM than in both IF at pH 6.0, 5.5, and 4.5, but comparable among all the samples at pH 3.5. The total solids recovery was relatively low in GMF and CMF at pH 6.0 and 5.5, probably due to difficulties in separating the serum and cream phases of both IF. GMF showed a trend of having more solids in the serum phase and less solids in the cream phase than CMF at all pH values. The distribution of solids over the cream (∼18% of the total solids), serum (∼74%) and pellet (∼8%) phase of HM remained fairly constant during the digestion. Both IF displayed a shift in distribution of the solids from the cream phase to the serum phase. As digestion progressed, the proportion of solids in the cream phase decreased from 35 to 27% in GMF and 45 to 32% in CMF, whereas that in the serum phase increased from 64 to 73% in GMF and 54 to 68% in CMF, respectively. The proportion of solids in the pellet phase of both IF were <1% and remained constant during digestion.
Table 1. Total solids distribution in the cream, serum and pellet fraction after centrifugation of the digesta from gastric digestion simulating infant conditions*.
The distribution of protein in different fractions after centrifugation is shown in . The protein content was first determined using the BCA assay. Compared to both IF, HM had a higher proportion of protein in the serum and pellet phase, and lower proportion of protein in the cream phase. In HM, 82, 86, 64, and 87% of the protein was present in the serum, at pH 6.0, 5.5, 4.5, and 3.5, respectively. Proportion of protein in pellet and cream phase in HM remained fairly constant during digestion. Compared to CMF, GMF had a higher protein content in the serum phase and lower protein content in the cream phase at pH 6.0 and pH 4.5; however, this difference tended to vanish at pH 3.5. During digestion of both IF, the proportion of protein in cream phase decreased, concomitantly the proportion of protein in the serum increased. The protein proportion in the pellet in IF was below detection limits, but estimated to be <1% of total protein since the pellet accounted for ≤1% of total solids, and therefore further analysis were not performed. For both IFs not all the protein was accounted for with the BCA assay. Therefore, the Dumas assay was used to confirm the trends observed. The protein content in the IF fractions was analysed by determining total N using the Dumas assay, which resulted in an improved protein recovery. The total N determined confirmed the findings regarding protein distribution in GMF and CMF analysed with the BCA assay.
SDS-PAGE analysis of the centrifugation fractions
Protein hydrolysis in the cream, serum and pellet fractions of the digesta separated by centrifugation from samples taken at different pH values was analysed using SDS-PAGE. The protein composition of undigested samples and fractions of the digesta on the SDS-PAGE gels are shown in . The duplicate samples of HM showed comparable results, the gel of one duplicate is shown in . SDS-PAGE analysis of the pellets of IF was not feasible due to the limited amount of pellets available (<10 mg). Bovine α-lactalbumin (α-la), β-lactoglobulin (β-la) and κ-casein (κ-csn) were selected as marker proteins since these proteins could be distinguished in GMF and CMF. Progression in protein digestion during gastric digestion was observed in all three samples, as evidenced by disappearance of and reduction in density of the bands associated with intact proteins, upon decreasing of pH. For instance, κ-casein was present in cream samples of HM, CMF and GMF at pH 6.0 and 5.5, but disappeared due to digestion at pH 4.5 and pH 3.5. κ-casein was also present in the pellet samples of HM, but absent in all serum samples. Another example to illustrate protein digestion is β-casein as it was present in the cream phase of all samples until pH 4.5 in HM, GMF and CMF. It disappeared at pH 3.5. Similarly, the pellet of HM was rich in β-casein until pH 3.5. Furthermore, in the cream and pellet samples of HM, the casein fragments started to overlap with the whey proteins upon decreasing of pH. Undigested proteins, as indicated by persistence of the same protein band at all pH, were also observed throughout the digestion. For instance, α-lactalbumin was present in all samples from pH 6.0, 5.5 and 4.5, fading only at pH 3.5. β-lactoglobulin persisted in the cream and serum phase of the IF at all pH values.
Viscosity under simulated gastric pH conditions
During acidification of the gastric content, protein may remain soluble or may coagulate which results in formation of aggregates or curd and changes in viscosity. The presence or absence of protein coagulation during acidification may affect accessibility to gastric enzymes and thus protein digestibility. In the present study, the viscosity of HM, GMF and CMF was monitored under simulated pH changes (pH decreased from ∼7.0 to ∼4 by adding gastric juice) during gastric digestion. At the end of the 3-h rheological measurement, the final pH reached was 4.0 for HM, 4.5 for GMF and 4.3 for CMF. As shown in , viscosity of HM remained low and constant during the simulated gastric digestion. GMF and CMF showed a slight increase in viscosity from pH 6.3 and pH 5.8, respectively, with the increase in CMF slightly higher than that of GMF.
Discussion
In the present study, the physicochemical changes and protein breakdown of fresh HM, a GMF and a CMF when subjected to in vitro simulated infant gastric conditions, were evaluated and compared. HM behaved differently from both IF for all properties investigated. Upon gastric digestion, HM formed notably larger aggregates than both IF. The viscosity of HM remained low and constant, whereas the two IF showed a slight increase in viscosity. HM showed a shift from a monomodal to a bimodal particle size distribution, coinciding with the presence of a cream, serum and pellet phase after centrifugation. The two IF maintained a monomodal distribution with a shift towards larger particle size, while only yielding a cream and serum phase.
The observed differences in particle size distribution between HM and the IF can be attributed to the difference between the native emulsions of HM fat globules and the processed (homogenization and heat treatment) submicronic emulsions in IF. The native HM fat globules have an average diameter of 4 μm (distribution 0.1–10 μm) and are stabilised by a 3-layered membrane mainly consisting of phospholipids, membrane-specific proteins, and cholesterol. Fat droplets in the conventional IF are 0.5 μm (distribution 0.1–1 μm) in size, contain vegetable oil, and are stabilised by a membrane based on caseins and whey protein aggregates (Michalski et al. Citation2005; Lopez and Menard Citation2011). The size and structure of the lipid droplets likely influence gastrointestinal lipolysis as well as proteolysis (Bourlieu et al. Citation2017). We speculate that in the present study, the initial fat globules of 1−10 μm in HM remained intact to a large extent during digestion and were present in the protein-depleted cream phase after centrifugation, whereas protein aggregates of 10−1000 μm sedimented to the pellet phase. In IF, on the other hand, initially protein-stabilised fat droplets of 0.1−1 μm were present; during digestion this size peak disappeared and a new size peak of 2−100 μm corresponding to the formation of protein-fat aggregates appeared. These aggregates were present in the protein-rich and fat-rich cream phase after centrifugation. The association between fat and protein in the IF cream phase may affect accessibility of digestive enzymes to these aggregates and passage of protein from stomach to the small intestine. During digestion, the protein content in the HM cream phase remained constant, whereas that in the IF shifted to the serum phase. The observed differences in particle size distribution between HM and the IF suggests that structure and processing of lipids in IF may influence digestion of protein in IF. Modification of lipids in IF towards being more similar to HM fat may improve protein digestion of IF.
In the present study, GMF and CMF were in general similar with regard to physicochemical behaviour and protein breakdown properties during in vitro gastric digestion. However, there were a number of notable differences which may help to understand our previous observations that initial protein digestion is faster in the GMF than in the CMF and the overall protein digestion kinetics of GMF is more comparable to HM than that of CMF (Maathuis et al. Citation2017). Firstly, while HM formed larger aggregates than both IF, GMF coagulated to slightly larger aggregates than CMF. The aggregates of GMF appeared less compact than those of CMF as suggested by the coarser structure shown under the stereomicroscope and the higher turbidity of the serum phase of the GMF. Under infant gastric digestion conditions, the less compact structure and larger size of GMF aggregates may allow easier diffusion of pepsin and other enzymes than those of CMF, which may facilitate a more efficient digestion. This is supported by a previous study showing that the diffusion coefficients of pepsin decrease in rennet gels with increasing firmness as a result of increasing casein concentration (Thévenot et al. Citation2017). Secondly, the proportions of protein in serum phase in HM were higher than those in both IF. Although the protein recovery for GMF and CMF was lower than that for HM, the protein measurements using both the BCA assay and the Dumas method showed a similar trend: GMF had a higher protein content in the serum phase and lower protein content in the cream phase in comparison with CMF at pH 6.0 and 4.5, but this difference tended to vanish at pH 3.5. A similar trend was observed for distribution of solids in the serum phase at all pH, suggesting a correlation between protein distribution and total solids distribution. In adults, gastric emptying of the aqueous phase is rapid, whereas the creamy phase is emptied more slowly (Golding and Wooster Citation2010). As a result of higher protein content in the serum phase and lower protein content in the cream phase, protein in HM may be more readily emptied from the stomach for further digestion in duodenum. Gastric emptying of HM is faster than IF, for which the reason remains to be elucidated (Bourlieu et al. Citation2014). The results of the present study, demonstrating a higher protein content in the serum phase of HM compared to conventional IF, provide an possible explanation to this observation. Gastric emptying is a major factor controlling the kinetics of milk nitrogen absorption, as milk proteins are shown to be rapidly absorbed after they reach the small intestine (Boland and Singh Citation2019). The protein content in the serum phase of the GMF was lower than that of HM but tended to be higher than that of the CMF, which may explain, at least partly, the faster initial digestion of HM and GMF as observed previously (Maathuis et al. Citation2017). In this study, physicochemical changes upon gastric digestion of protein, including separation into a cream and serum phase, disappearance of close-knit network under stereomicroscope, decreases in particle size and a slight increase in viscosity (lower than CMF), were observed at an earlier stage of digestion, i.e. at higher pH, in GMF than in CMF. This might be correlated to a faster initial digestion as reported by Maathuis et al. (Citation2017).
Similar observations on aggregation formation during in vitro gastric digestion behaviour was described by Ye et al. (Citation2019). Gastric digestion behaviour of two GMF and two CMF with a casein to whey protein ratio of 80:20 and 40:60, respectively, were studied using a dynamic digestion model. Differences in aggregation behaviour were observed between CMF and GMF, which might lead to faster digestion in GMF. Casein-dominant IFs displayed greater extent of aggregation leading to slower casein digestion in comparison with whey-dominant IFs. Fondaco et al. (Citation2015) studied physicochemical behaviour of HM and IF during in vitro digestion. The initial droplet size of HM was larger than that of four types CMF tested. HM developed less viscosity than the CMF over the range of pH encountered during neonatal gastric phase (pH 6–4 mainly). Whether this limited viscosity could impact on gastric emptying was questioned by the authors. Increasing the viscosity of liquid meals has been suggested to delay gastric emptying (Benini et al. Citation1995), however, considerable increase is required to have a significant impact on gastric emptying (Camps et al. Citation2016). In the present study, the viscosity of HM remained low, the viscosity of the GMF was lower than that of the CMF during gastric digestion. However, it is not clear whether the observed differences in viscosity among HM, GMF and CMF may affect gastric emptying.
The current study and the available studies comparing digestive behaviours of milk protein from goat, cow and HM are mostly focussed on gastric digestion. Gastric digestion is the first phase of protein digestion. Products of gastric digestion may influence a number of gastric functions, such as secretion of acid and pepsinogen, rate of gastric emptying, and control of the pyloric sphincter (Erickson and Kim Citation1990). However, protein digestion in the stomach in young infants is likely limited, due to a low level of pepsin and high gastric pH which is not optimal for pepsin activities. A large portion of protein degradation occurs in the small intestine (Dallas et al. Citation2012; Bourlieu et al. Citation2014). Therefore, studying the digestive behaviours of milk protein in the small intestine of infants can be of interest for future studies. Furthermore, it needs further investigation to clarify whether differences in digestion behaviour of protein between HM and IF based on milk from different animal species may have physiological consequences in infants, for instance, on protein metabolism, intestinal tolerance or growth of the infants.
Altogether, the present study shows that when subjected to in vitro gastric digestion simulating infant conditions, the GMF and CMF are in general similar with regard to physicochemical and protein breakdown properties. However, a number of notable differences in physicochemical behaviour exist, which may contribute to the previously-reported faster initial digestion of GMF and digestion kinetics more comparable to that of HM. While the level of protein in the serum phase was highest for HM, the level was higher for GMF compared to CMF (at pH 6.0 and pH 4.5). In addition, GMF appears to form larger and less compact aggregates than the CMF. Moreover, physicochemical changes upon gastric digestion of protein were observed at an earlier stage of digestion in the GMF than in the CMF. HM behaves differently from the IF. The differences between HM, the GMF and CMF provide new insights into the possibilities for improvement of infant formulas, for instance, modification of lipids in IF.
Supplemental Material
Download Zip (127.9 KB)Acknowledgments
The authors thank Alfred Haandrikman, Randolph Happe, Lucie van der Zee and Alwine Kardinaal for helpful discussion and carefully reading the manuscript.
Disclosure statement
Tao He and Wolf Rombouts are employees of Ausnutria B.V.
Additional information
Funding
References
- Almaas H, Cases AL, Devold TG, Holm H, Langsrud T, Aabakken L, Aadnoey T, Vegarud GE. 2006. In vitro digestion of bovine and caprine milk by human gastric and duodenal enzymes. Int Dairy J. 16(9):961–968.
- Benini L, Castellani G, Brighenti F, Heaton KW, Brentegani MT, Casiraghi MC, Sembenini C, Pellegrini N, Fioretta A, Minniti G. 1995. Gastric emptying of a solidmeal is accelerated by the removal of dietary fibre naturally present in food. Gut. 36(6):825–830.
- Boland M, Singh H. 2019. Milk proteins. 3rd ed. London (UK): Academic Press.
- Bourlieu C, Ménard O, Bouzerzour K, Mandalari G, Macierzanka A, Mackie AR, Dupont D. 2014. Specificity of infant digestive conditions: some clues for developing relevant in vitro models. Crit Rev Food Sci Nutr. 54 (11):1427–1457.
- Bourlieu C, Deglaire A, de Oliveira SC, Ménard O, Le Gouar Y, Carrière F, Dupont D. 2017. Towards infant formula biomimetic of human milk structure and digestive behaviour. OCL. 24(2):D206.
- Camps G, Mars M, de Graaf C, Smeets PA. 2016. Empty calories and phantom fullness: a randomized trial studying the relative effects of energy density and viscosity on gastric emptying determined by MRI and satiety. Am J Clin Nutr. 104(1):73–80.
- Ceballos LS, Morales ER, Martínez LP, Extremera FG, Sampelayo MRS. 2009. Utilization of nitrogen and energy from diets containing protein and fat derived from either goat milk or cow milk. J Dairy Res. 76(4):497–504.
- Dallas DC, Underwood MA, Zivkovic AM, German JB. 2012. Digestion of protein in premature and term infants. J Nutr Disord Ther. 2(3):112.
- Erickson RH, Kim YS. 1990. Digestion and absorption of dietary protein. Annu Rev Med. 41:133–139.
- Fondaco D, AlHasawi F, Lan Y, Ben-Elazar S, Connolly K, Rogers M. 2015. Biophysical aspects of lipid digestion in human breast milk and similac(TM) infant formulas. Food Biophys. 10(3):282–291.
- Golding M, Wooster TJ. 2010. The influence of emulsion structure and stability on lipid digestion. Curr Opin Colloid Interface Sci. 15 (1–2):90–101.
- Hodgkinson AJ, Wallace OAM, Boggs I, Broadhurst M, Prosser CG. 2018. Gastric digestion of cow and goat milk: impact of infant and young child in vitro digestion conditions. Food Chem. 245:275–281.
- Hodgkinson AJ, Wallace OAM, Smolenski G, Prosser CG. 2019. Gastric digestion of cow and goat milk: peptides derived from simulated conditions of infant digestion. Food Chem. 276:619–625.
- Koletzko B, Brands B, Grote V, Kirchberg FF, Prell C, Rzehak P, Uhl O, Weber M, Early Nutrition Programming Project 2017. Long-term health impact of early nutrition: the power of programming. Ann Nutr Metab. 70(3):161–169.
- Lopez C, Menard O. 2011. Human milk fat globules: polar lipid composition and in situ structural investigations revealing the heterogeneous distribution of proteins and the lateral segregation of sphingomyelin in the biological membrane. Colloids Surf B Biointerf. 83(1):29–41.
- López-Aliaga I, Alferez MJM, Barrionuevo M, Nestares T, Sanz Sampelayo MR, Campos MS. 2003. Study of nutritive utilization of protein and magnesium in rats with resection of the distal small intestine. Beneficial effect of goat milk. J Dairy Sci. 86(9):2958–2966.
- Lönnerdal B. 2014. Infant formula and infant nutrition: bioactive proteins of human milk and implications for composition of infant formulas. Am J Clin Nutr. 99(3):712s–717s.
- Maathuis A, Havenaar R, He T, Bellmann S. 2017. Protein digestion and quality of goat and cow milk infant formula and human milk under simulated infant conditions. J Pediatr Gastroenterol Nutr. 65(6):661–666.
- Michalski MC, Briard V, Michel F, Tasson F, Poulain P. 2005. Size distribution of fat globules in human colostrum, breast milk, and infant formula. J Dairy Sci. 88(6):1927–1940.
- Michaelsen KF, Greer FR. 2014. Protein needs early in life and long-term health. Am J Clin Nutr. 99(3):718S–722S.
- Nguyen TTP, Bhandari B, Cichero J, Prakash S. 2015. A comprehensive review on in vitro digestion of infant formula. Food Res Int. 76 (3):373–386.
- Nelson WE, Kliegman R. 2011. Nelson textbook of pediatrics. Philadelphia (PA): Saunders.
- Pintado ME, Malcata FX. 2000. Hydrolysis of ovine, caprine and bovine whey proteins by trypsin and pepsin. Bioproc Eng. 23:275–282.
- Prosser CG. 2021. Compositional and functional characteristics of goat milk and relevance as a base for infant formula. J Food Sci. 86:257–265.
- Thévenot J, Cauty C, Legland D, Dupont D, Floury J. 2017. Pepsin diffusion in dairy gels depends on casein concentration and microstructure. Food Chem. 223:54–61.
- Ye A, Cui J, Carpenter E, Prosser C, Singh H. 2019. Dynamic in vitro gastric digestion of infant formulae made with goat milk and cow milk: Influence of protein composition. Int Dairy J. 97:76–85.
- Wang Y, Eastwood B, Yang Z, de Campo L, Knott R, Prosser C, Carpenter E, Hemar Y. 2019. Rheological and structural characterization of acidified skim milks and infant formulae made from cow and goat milk. Food Hydrocoll. 96:161–170.
- Wijayanti H, Essers J, de Groot J, de Jong S, Hotrum N, Kardinaal A. 2019. Comparative use of two in vitro digestion models to understand milk protein digestion. Poster presented at 6th International Conference on Food Digestion, Granada, April 2019.
- Zhou SJ, Sullivan T, Gibson RA, Lönnerdal B, Prosser CG, Lowry DJ, Makrides M. 2014. Nutritional adequacy of goat milk infant formulas for term infants: a double-blind randomised controlled trial. Br J Nutr. 111(9):1641–1651.