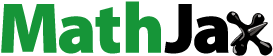
Abstract
Purpose
Accelerometry measurements are a promising method to provide quantitative information of upper limb function in daily life post stroke. Our purpose was to investigate i) the validity of accelerometer-based vector magnitude ratios (VMR) to distinguish upper limb function between individuals post-stroke and healthy controls during ambulatory and non-ambulatory activities and, ii) the association between the VMR and clinical assessment of upper limb function for individuals post-stroke.
Methods
Forty persons with stroke and 32 healthy controls wore wrist and hip accelerometers while performing three upper limb activities in a clinical setting and during three days in daily living. Clinical assessment of upper limb function was assessed with the Chedoke-McMaster Stroke Assessment measure and ABILHand.
Results
In the clinical setting and daily living, the VMR was significantly lower for individuals post-stroke compared to controls during non-ambulatory activities but not during ambulatory activities. There was a moderate to strong association between VMR and clinical assessment of upper limb function during all conditions, except for walking in the clinical setting.
Conclusion
The VMR could be used as a sensitive objective marker to measure upper limb function post-stroke during ambulatory and non-ambulatory daily activities.
Accelerometer-based assessment of upper limb function is a sensitive measure of upper limb function post stroke during different activity domains.
Accelerometers-based assessment of arm function can detect differences in arm function outside the clinical setting (daily living) over a prolonged period that are not always identified by clinical assessment or perceived ability.
Implications for rehabilitation
Introduction
Partial or complete upper limb hemiparesis is a common impairment after stroke, occurring in around two-thirds of this clinical population [Citation1–3]. Considering that activities of daily living largely depend on arm function, it is not surprising that stroke survivors with persistent arm impairments demonstrate greater dependency in basic activities of daily living and on the use of modern technologies (e.g., cellphones and computers) [Citation4]. One important goal of post-stroke rehabilitation is therefore to promote upper limb function to regain independence in daily life [Citation5,Citation6]. Although clinical assessment of upper limb function (e.g., clinical tests or questionnaires) gives useful information on the level of impairment or function, it does not provide quantitative information of actual motor ability, especially outside the clinical setting [Citation7].
Objective assessment through the use of accelerometers has therefore been proposed as a complimentary quantitative method for upper limb assessment [Citation1,Citation7–14]. Accelerometers measure mechanical movement (acceleration changes) due to body movement and yield primarily quantitative information such as frequency, duration and intensity of an activity [Citation15]. The benefit of this method is its ability to monitor arm use in daily life over a prolonged period which may provide a more robust marker of the level of upper limb activity than clinical assessment of function at a single time point. Most previous studies exploring the use of accelerometers for upper limb assessment post stroke have used the vector magnitude (VM) for analyses, which incorporates all three directional components (vertical, horizontal and perpendicular) of arm movements in daily life [Citation16]. The vector magnitude ratio (VMR, i.e., the relation of the VM of the affected side to the VM of the unaffected side with a value of 1 reflecting equal use of both limbs) is generally used when measuring upper limb activity in individuals post-stroke [Citation17,Citation18].
While previous studies [Citation5,Citation11,Citation17,Citation18] have successfully shown an association between upper limb impairment assessed using clinical tests and arm activity in daily life assessed using accelerometers, most studies have used accelerometers to measure arm activity as an overall estimate of all types of upper-limb activities occurring during a day. Moreover, the wrist worn sensors might be prone to detect sporadic upper-limb movements, e.g., movements that are not goal-directed and occur indirectly as a consequence of movement of another body segment. For example, people with hemiparetic stroke generally have an asymmetric gait pattern which is characterized by jerking movements of the trunk and reduced range of movement of the affected arm [Citation19]. The hemiparetic gait pattern could induce high amplitude acceleration signals and result in the VMR being falsely interpreted. Exploring different types of activities (e.g., arm movements during ambulation or goal-directed arm movements during non-ambulatory tasks) could therefore yield important information regarding the validity of accelerometers to assess upper limb activity post stroke.
This study aimed to explore the ability of accelerometers to distinguish upper limb function between individuals post-stroke and healthy controls during different activities in a clinical setting (e.g., setting a table, washing dishes and walking) and during non-ambulatory and ambulatory physical activity in daily living. We investigated two aspects of construct validity (known-group validity and convergent validity) of accelerometer-based VMR in individuals post-stroke. For known-group validity, we tested the hypotheses that VMR would be lower for individuals post-stroke compared to healthy controls during everyday upper limb activities performed in a standardized clinical setting and in daily life. For convergent validity, we tested the hypotheses that there is an association between clinical assessment of upper limb function (i.e., self-perceived impairment and clinical test) and VMR assessed in a clinical setting and in daily life for individuals post-stroke.
Methods
Study design and ethics
This cross-sectional study was approved by the Regional Board of Ethics in Stockholm (2017/1626-31 and 2018/2524-32) and all participants gave written informed consent prior to participation.
Study participants
People who had a stroke ≥3 months prior to study participation, with/without self-perceived upper limb impairment and with the ability to ambulate with/without a walking device or a wheelchair were recruited from rehabilitation centers and through advertisements. Exclusion criteria included cognitive deficit, severe neglect and aphasia, which affected the ability to give written consent and follow instructions. Healthy individuals with no upper limb impairments were recruited through advertisement and senior organizations as a reference group.
Data collection
All participants (stroke and healthy controls) attended one session at a rehabilitation facility, which included: collection of demographic data (sex, hand dominance, mobility, living situation and employment status), cognition, balance confidence, independence in daily living and self-perceived arm function. Cognition was tested using the Montreal Cognitive Assessment (MoCA), which is a quick and sensitive test for screening of cognitive function [Citation20]. The Katz ADL Index Extended (KATZ Index) was used to assess personal activities of daily living (ADL) including dressing, bathing, feeding, continence, toileting, transferring, and instrumental ADL including cleaning, shopping, transportation and cooking [Citation21]. Participants who reported dependency in one or more items of the two sub-domains of KATZ Index were classified as dependent in either personal or instrumental ADL. Further, the ABILHand, which consists of 23 questions, was used to assess the self-perceived level of difficulty experienced by the individuals whilst performing certain upper limb activities, such as drawing, closing a door, brushing one’s hair and teeth [Citation22,Citation23]. The sum score (ranging from 0 to 46) of the level of difficulty (0 = impossible, 1 = difficult and 2 = easy) of performing each upper limb activity was determined, with the higher score corresponding to better self-perceived upper limb function.
For participants with stroke, we also collected information about stroke chronicity, type of stroke and number of previous strokes. Severity of stroke was assessed with the National Institutes of Health Stroke Scale (NIHSS) [Citation24] and upper limb impairment with the Impairment Inventory of the Chedoke-McMaster Stroke Assessment measure (CMSA). The CMSA includes six subsections (recovery stage of the arm, hand, leg, foot, postural control, and shoulder pain). Each dimension is measured on a 7-point scale; a score of 1 reflect no recovery (i.e., paralysis) and a score of 7 reflects complete recovery (i.e., normal movement). In this study, we analyzed a sum-score of the arm and hand subsection (i.e., total score of 14) [Citation25,Citation26].
For assessment of standardized upper limb activities in the clinical setting, participants with stroke and healthy controls were fitted with two wrist-worn triaxial accelerometers (ActiGraph GT3X+). The accelerometers recorded time series acceleration data at a sampling rate of 30 Hz. The participant was asked to perform two standardized everyday activities involving arm movements (setting a table and washing dishes) and one activity involving ambulation (walking in a self-selected speed). These activities, detailed below, were chosen due to their occurrence in everyday living and their multi-dimensional nature of arm movements. The test leader recorded the start and stop time for each activity.
Setting a table
Participants sat on a chair while their maximum arm reach was marked on the table in front of them by the test leader. Three kitchen crockery sets (plate, glass, spoon) were positioned directly in front and to the left of the participant. The participants were then asked to align all items of the kitchen crockery sets (as if they were setting the table for dinner) at the marked position. After this was completed, participants returned all items back to the starting position (but this time on the right-hand side) and started the task again. This task continued for three minutes.
Washing dishes
Participants stood or sat in front of a standard kitchen sink with the same kitchen crockery sets as for the first task, positioned on the left-hand side of the sink. The participants were equipped with a dish cleaning brush and asked to wash the dishes, dry the dishes with a cloth and place them on the right-hand side of the sink. Thereafter, the participant repeated the movements, but this time moving the dishes from the right to the left-hand side. This task was performed for six minutes.
Walking at a self-selected speed
Participants walked at their self-selected speed with 180 degree turns every 60 meters. The walking was performed for 6 min and participants were allowed to use a walking aid if needed.
After completing the activities in the clinical setting, each participant was equipped with two wrist-worn and one hip worn Actigraph accelerometers and an activity diary for assessment of arm movements in daily life. The hip worn sensor was positioned on the unaffected and dominant side for the stroke and healthy participants, respectively. Participants were asked to wear the accelerometers for all waking hours for three consecutive days and to record wear time of the devices in a diary. Three days have been showed to be valid to measure arm movements in daily life in people with stroke [Citation7,Citation27]. They were asked to only take the device off whilst bathing or showering.
Data management
The acceleration data obtained from the clinical setting were converted to five seconds epochs and segmented into the individual activities (i.e., the total duration of each activity) using the start and stop times recorded by the test leader. For the daily living, all sensor data were synchronized, converted to five second epochs and divided into daily segments. Subsequently, a non-wear time algorithm, in accordance with Choi et al. 2011, were applied on the daily hip worn acceleration data. The algorithm takes into consideration 90 min of consecutive zero counts and 2 min of non-zero counts with an upstream/downstream of 30 min of consecutive zeros [Citation28]. A day was considered valid and included in the analysis if the wear time was ≥ 10 h. The periods of non-wear time were also confirmed with the participants daily diary entries.
In order to distinguish between different modes of activity in daily life, the daily living data were first divided into sedentary (< 100 vertical counts per minute) and physical activity (≥ 100 vertical counts per minute) using data from the hip worn accelerometer [Citation29]. Secondly, the physical activity domain was further divided into ambulation and non-ambulation physical activity. For ambulation, we used a cut-point threshold of 1041 vertical counts per minute corresponding to a walking speed of 0.88 m/s [Citation30]. In order to detect short walks occurring in daily life, we defined physically active ambulation if three or more consecutive five second epochs (i.e., ≥ 15 s in total) exceeded this cut-point. The criteria for physically active non-ambulation were defined as the epoch data that did not exceed this cut-point threshold for more than three consecutive five second epochs. The wrist worn data, for the different modes of activity, was delineated using the synchronized times with the hip worn data. All the counts per minute cut-points were rectified to counts per 5-s cut-points (i.e., divided by 12).
Subsequently, the VM was calculated for the right and left wrist worn sensors for each five second epoch as:
where X, Y and Z correspond to directions perpendicular to the skin surface, parallel to the forearm, and perpendicular to the forearm and parallel to the skin surface, respectively. The VMR was calculated as:
For the healthy participants, the VMR was calculated as the ratio of VM of the non-dominant to dominant side. Generally, the wrist has more complex movements and thus the VM is used to simplify the orientation of the activities [Citation11]. The VMR indicates which arm is used more, relative to the other arm. The VMR was averaged for the standardized activities in the clinical setting and for the different activity domains (sedentary, non-ambulation and ambulation) over three days in daily living.
Statistical analysis
Statistical analyses were carried out using IBM SPSS v.26 software. Descriptive variables and accelerometer outcomes (i.e., VMR) were tested for data normality using the Shapiro-Wilk Test and visual inspection of histogram. Descriptive statistics were used to present demographics, stroke-related and functioning variables, and compared between participants with stroke and healthy controls using the independent t-test, Chi square and the Fisher's exact test.
For known group validity, the Mann-Whitney U-test was used to investigate the difference in VMR between individuals post-stroke and the control group in the clinical setting and daily living. The percentage difference between the VMR of the individuals post stroke and the controls were calculated as
For convergent validity, the Spearman correlation coefficients were used to determine the associations between VMR in the clinical setting and daily life, and self-perceived upper-limb impairment (ABILHand) and clinical assessed upper-limb impairment (CMSA). The strength of the correlation coefficients was classified as follows: <0.10 = negligible; 0.10–0.39 = weak; 0.40–0.69 = moderate; 0.70–0.89 = strong and 0.90–1.00 = very strong [Citation31]. The level of significance was set to 5%. The control group average VMR and corresponding 95% confidence intervals during the clinical setting and daily living were presented as a reference.
Results
Participant characteristics
Forty individuals post-stroke and 32 healthy controls participated in this study. Three individuals post-stroke were excluded from the daily living analysis since they did not record sufficient daily accelerometer data. Four individuals post-stroke used a wheelchair and did not complete the walking task in the clinical setting. presents the demographics and clinical scores for both groups. For participants with stroke, mean time since stroke was 3.0 years (SD: 4.2) and the median arm and hand CMSA subsection were 4.0 and 5.0, respectively, with a large variation in both sub scores (min-max: 1–7). Eleven participants with stroke used a walking device (walking cane: n = 8 or stroller: n = 3) while performing the walking test in the clinical setting. Compared to the healthy control group, participants with stroke were on average 6 years older (p = 0.025), had a larger proportion of males (55% vs 28%, p = 0.022) and being on sick leave (23% vs 0%, p = 0.015).
Table 1. Demographics and clinical scores of participants with stroke and controls.
Stroke vs healthy controls (known-group validity)
As shown in , the average VMR for the control group in the clinical setting was close to 1 for setting the table (VMR: 0.95) and straight walking (VMR: 1.01); reflecting that dominant and non-dominant arms were used relatively equally during these activities. In contrast, the average VMR for the control group for washing the dishes was greater than 1 (VMR: 1.12, i.e., non-dominant arm was on average being used more). Individuals post-stroke had an average 77% and 93% lower VMR than healthy controls for setting the table and washing the dishes, respectively (p < 0.001). There was no significant difference in VMR between individuals post-stroke and controls during walking (p = 0.12). The results also showed no significant difference in VMR for participants with stroke who used a walking device during the straight walking task compared to those who walked without a device (1.33 vs 0.89, p = 0.47).
Table 2. Comparison between the VMR of the stroke participants and the healthy controls in the clinical and daily living (known-group validity).
While the average (SD) total wear time of the assessment in daily life was similar between individuals post-stroke and healthy controls (937 min/day SD: 234 vs. 922 min/day SD: 303, p = 0.81), individuals post-stroke spent significantly more time than controls being sedentary (845 min/day SD: 206 vs. 687 min/day SD: 227, p < 0.001), and less time in non-ambulation (72 min/day SD: 4 vs. 157 min/day SD: 54, p < 0.001) and in ambulation (20 min/day SD: 25 vs. 78 min/day SD: 42, p < 0.001). Individuals post-stroke also had a 65% (0.45 vs 0.88, p < 0.001) and 52% (0.54 vs 0.92, p < 0.001) lower VMR than controls during sedentary and active non-ambulation, respectively (). There was also a significant difference between the individuals post stroke and controls (24%, 0.72 vs 1.00, p < 0.001) during active ambulation.
Association between VMR and clinical assessment of upper limb impairment (convergent validity)
As illustrated in and , the VMR during setting the table and doing the dishes in the clinical setting was strong to very strong positively associated with clinical assessed upper limb impairment as measured by CMSA (r = 0.83–0.89, p < 0.001) and the self-perceived upper limb impairment as measured by the ABILHand (r = 0.76–0.81, p < 0.001) in individuals post-stroke. In contrast, there was a negligible association (r = 0.01) between the VMR during walking and clinical assessed upper limb and self-perceived upper limb impairment (, ). shows little to no overlap in the VMR between individuals with stroke and the lower and upper 95% confidence interval of healthy controls for setting the table and washing the dishes whereas there is an overlap for self-selective walking. It is worth noting that there were 17 participants with stroke (43%) whose affected side corresponded to their dominant side. There was no significant difference in VMR between individuals post-stroke whose dominant side was their affected side and those whose non-dominant was their affected side during standardized activities; setting the table (0.52 vs. 0.35, p = 0.11), washing the dishes (0.48 vs. 0.35, p = 0.23) and walking (0.95 vs. 0.88, p = 0.96).
Figure 1. The association between clinical assessment of upper limb function; Chedoke-McMaster Stroke Assessment and ABILHand, and Vector Magnitude Ratio while setting the table (A-B) and washing dishes (C,D). The solid and dashed line represent the mean and 95% confidence intervals of the VMR for the control group, respectively. (A) Scatterplot showing the positive relationship between the Chedoke-McMaster Stroke Assessment and Vector Magnitude Ratio for setting the table for individuals post-stroke. (B) Scatterplot showing the positive relationship between the ABILHand and Vector Magnitude Ratio for setting the table for individuals post-stroke. (C) Scatterplot showing the positive relationship between the Chedoke-McMaster Stroke Assessment and Vector Magnitude Ratio for washing the dishes for individuals post-stroke. (D) Scatterplot showing the positive relationship between the ABILHand and Vector Magnitude Ratio for washing the dishes for individuals post-stroke. (E) Scatterplot showing no relationship between the Chedoke-McMaster Stroke Assessment and Vector Magnitude Ratio for self-selective walking for individuals post-stroke. (F) Scatterplot showing no relationship between the ABILHand and Vector Magnitude Ratio for self-selective walking for individuals post-stroke.
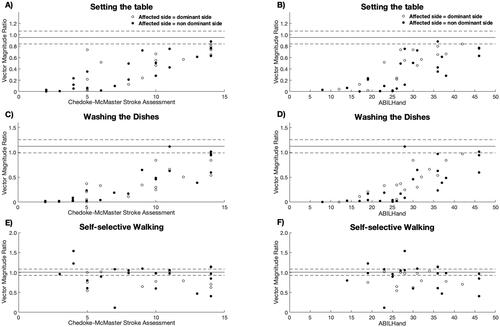
Table 3. Correlation coefficients (p-values) between accelerometer-based assessment of upper limb function (VMR) and clinical assessment of upper-limb function (CMSA and ABILHand) in individuals post-stroke.
For daily living, the VMR was moderate positively associated with the clinical assessed upper limb impairment (CMSA) and self-perceived upper limb impairment (ABILHand) during sedentary (r = 0.57–0.58), active non-ambulation (r = 0.57–0.63) and ambulation (r = 0.43–0.49), respectively ( and ). shows an overlap in the VMR between individuals with stroke and the lower and upper 95% confidence interval of healthy controls during ambulatory activities in daily living, whereas there was little to no overlap between the groups during non-ambulatory (sedentary and active ambulation) activities. The overlap during ambulation ( and ) indicates the sensitivity of the wrist-worn accelerometers for individuals with stroke.
Figure 2. The association between clinical assessment of upper limb function; Chedoke-McMaster Stroke Assessment and ABILHand, and Vector Magnitude Ratio (VMR) averaged over three days in daily living. The solid and dashed line represents the mean and 95% confidence intervals of the VMR for the control group, respectively. (A) Scatterplot showing the positive relationship between the Chedoke-McMaster Stroke Assessment and Vector Magnitude Ratio during the sedentary state in daily living for individuals post-stroke. (B) Scatterplot showing the positive relationship between the ABILHand and Vector Magnitude Ratio during the sedentary state in daily living for individuals post-stroke. (C) Scatterplot showing the positive relationship between the Chedoke-McMaster Stroke Assessment and Vector Magnitude Ratio during the active non-ambulation state in daily living for individuals post-stroke. (D) Scatterplot showing the positive relationship between the ABILHand and Vector Magnitude Ratio during the active non-ambulation state in daily living for individuals post-stroke. (E) Scatterplot showing no relationship shown between the Chedoke-McMaster Stroke Assessment and Vector Magnitude Ratio during the active ambulation state in daily living for individuals post-stroke. (F) Scatterplot showing no relationship between the ABILHand and Vector Magnitude Ratio during the active ambulation state in daily living for people with stroke.
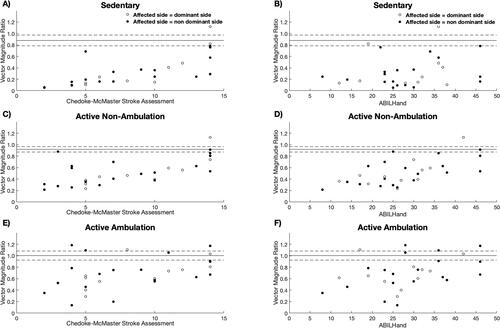
Discussion
The purpose of this study was to test the ability of accelerometer-based VMR to distinguish between individuals post-stroke and healthy participants (known-group validity) in different settings (clinical and daily life) and activity modes (ambulatory and non-ambulatory activities), and to assess the association between accelerometer-based VMR and clinical assessment of upper limb function (convergent validity) for individuals post-stroke. The results showed a significant difference in the accelerometer-based measurements of arm activity in individuals post-stroke and healthy controls for both standardized clinical setting and daily living, with no significant difference observed during the walking activity in the clinical setting. Our results suggest that accelerometer-based VMR is a promising approach for evaluating arm use post stroke and indicates that accelerometer-based VMR are more sensitive to detect post stroke impairments during stationary activities than during walking.
Irrespective of setting, the results showed a strong association between the accelerometer-based assessment of arm activity and clinical assessment (self-perceived and clinical test) of upper limb function during non-ambulatory activities in the clinical setting (i.e., washing the dishes and setting the table). The present results showed that individuals post-stroke used their paretic arm less than the non-paretic limb, even amongst those individuals post-stroke who did not perceive problems with upper-limb function in daily life. This indicates that accelerometers-based assessment of arm function was able to detect differences in arm function that were not identified by clinical assessment or perceived ability. The present result is consistent with previous studies reporting accelerometer measurements of upper limb activity to be associated with clinical test of upper limb function [Citation10,Citation32,Citation33]. Our results are in line with a previous study by van der Pas et al. 2011 who reported moderate associations (r = 0.58–0.66) between accelerometer-based VMR in daily living and clinical assessment of upper limb in individuals post-stroke at different stages after stroke onset [Citation34]. In addition, our results are in line with findings by Thrane et al. (2011), who found a strong (r = 0.85) association between VMR assessed in daily living and clinical assessment of upper extremity in individuals post-stroke [Citation35].
Our results also showed no association between VMR during walking and clinical assessment of upper-limb function in the individuals post-stroke. This could be attributed to the asymmetric gait pattern of hemiparetic stroke characterized by jerking movements by the trunk and reduced coordination and range of motion of the affected arm [Citation19]. Hemiparetic gait could in turn cause the movement of the affected arm to induce high amplitudes of the accelerometer signal despite impaired arm swing. Alternatively, the lack of association between VMR and upper-limb function during walking could be attributed to the use of walking devices (cane or walking frame) among some of the participants with stroke in our study, which would suggest that walking devices influence the sensitivity of the wrist-worn accelerometers during ambulation. This was also evident in the 40% higher VMR (although not significantly different) observed for the stroke participants who utilized a walking device compared to those who did not use a walking device (1.33 vs 0.89). The higher VMR for those using a walking device could be attributed to the non-paretic arm holding the walking device (e.g., cane) fixed to the floor while the body and the paretic upper limb is being advanced forward.
For the participants with stroke, the ambulatory VMR was also higher during walking in the clinical setting (1.13) compared to ambulation in daily living (0.72). In contrast to walking in the clinical setting, there was also a moderate association between VMR and clinical assessment of upper-limb function during active ambulation in daily living. The cut-points used for defining active ambulation in our study corresponds to a walking speed of 0.88 m/s, which is approximately 0.07 m/s (8.3%) faster than the mean comfortable walking speed (0.81 m/s) of the stroke group. It is possible that the stroke participants walked with a greater walking speed during the clinical testing than their normal walking speed in daily living. If so, the impact of jerking trunk and arm movements on VMR during walking might have affected the assessment in the clinical setting but not in daily living.
Individuals post-stroke generally perform better in a standardized clinical upper limb assessment when asked to perform a specific activity compared to daily living [Citation7]. It is worth noting that the average VMR in individuals post-stroke was slightly greater in daily living (sedentary: 0.45, active non-ambulation: 0.54) than in the clinical environment (setting the table: 0.42, washing the dishes: 0.41). This could be attributed to the limitation of only measuring a few upper limb activities in a controlled setting, reflecting a limited movement pattern compared to the range of different movement patterns naturally occurring in different activities in daily life. Additionally, this could also reflect a behavior developed by individuals post-stroke to meet the new demands of performing daily activities despite impairment. Studies by Roby-Brami et al. [Citation36] and Liu et al. [Citation37] have documented chronic stroke patients developing compensatory movement strategies of the paretic arm [Citation36,Citation37]. Their studies showed that the overall dynamics of reaching was characterized by manipulating the trunk, elbow and shoulder resulting in excessive upper limb movements which in turn could result in higher VMR. This sort of compensation is common with multiple repetition of movement and could be a plausible explanation for the somewhat higher VMR in the daily living in our study, especially as the average time since stroke onset was almost 3 years. Although the VMR gives an indication of asymmetric upper limb activity, it is worth noting that other factors such as pain and motivation can also contribute to reduced activity of the affected limb. These factors should be taken into account in future studies.
Study limitations include the accelerometer’s inability to measure specific activities in daily living. Since we recorded accelerometer data for three consecutive days in daily living, we are unsure if recording over a longer period would contribute to more reliable estimate of upper limb activity. Additionally, we only conducted three standardized activities (washing the dishes, setting the table and straight walking) in the clinical setting; performing a greater number of upper limb tasks in the clinical setting would represent a better reflection of daily living. Furthermore, we had a relatively small sample size for the stroke participants, which consisted of people with chronic stroke. The stroke group was also older, with a larger proportion of males and being on sick leave compared to the control group. Therefore, our results may not be generalizable to the overall stroke population and the reference group in our study might not be a true representation of arm activity in the healthy population. Still, for the reference group, the accelerometer-based VMR measured in the clinical setting and daily life was overall centered around 1 (i.e., symmetric arm activity use), or slightly below 1 (i.e., the dominant hand is used more than the non-dominant) which is in line with previous studies [Citation38].
Our results suggest that accelerometer-based assessment of upper limb activity is a valid method to distinguish upper limb function between individuals post-stroke and healthy controls. The VMR could be used as a sensitive objective measure for upper limb function post-stroke in different activity domains (i.e., sedentary, active ambulation and active non-ambulation). Measuring VMR could result in a more robust marker of the level of upper limb impairment during different daily activities and therefore identify content specific rehabilitation exercises (e.g., symmetry and intensity of use).
Acknowledgments
The authors thank all the participants for their valuable time and efforts and physiotherapist Frida Wallén who took part in data collection. The authors also thank the Norrbacka-Eugenia foundation, Promobilia foundation and NEURO Sweden for financial support.
Disclosure statement
The authors report no conflict of interest.
References
- de Niet M, Bussmann JB, Ribbers GM, et al. The stroke upper-limb activity monitor: its sensitivity to measure hemiplegic upper-limb activity during daily life. Arch Phys Med Rehabil. 2007;88(9):1121–1126.
- Gebruers N, Vanroy C, Truijen S, et al. Monitoring of physical activity after stroke: a systematic review of accelerometry-based measures. Arch Phys Med Rehabil. 2010;91(2):288–297.
- Kwakkel G, Kollen B. Predicting improvement in the upper paretic limb after stroke: a longitudinal prospective study. Restor Neurol Neurosci. 2007;25(5–6):453–460.
- Kwakkel G, Kollen BJ, van der Grond J, et al. Probability of regaining dexterity in the flaccid upper limb: impact of severity of paresis and time since onset in acute stroke. Stroke. 2003;34(9):2181–2186.
- Rand D, Eng JJ. Disparity between functional recovery and daily use of the upper and lower extremities during subacute stroke rehabilitation. Neurorehabil Neural Repair. 2012; 26(1):76–84.
- Reiterer V, Sauter C, Klosch G, et al. Actigraphy-a useful tool for motor activity monitoring in stroke patients. Eur Neurol. 2008;60(6):285–291.
- Uswatte G, Foo WL, Olmstead H, et al. Ambulatory monitoring of arm movement using accelerometry: an objective measure of upper-extremity rehabilitation in persons with chronic stroke. Arch Phys Med Rehabil. 2005;86(7):1498–1501.
- Bernmark E, Wiktorin C. A triaxial accelerometer for measuring arm movements. Appl Ergon. 2002;33(6):541–547.
- Chen KY, Acra SA, Majchrzak K, et al. Predicting energy expenditure of physical activity using hip- and wrist-worn accelerometers. Diabetes Technol Ther. 2003;5(6):1023–1033.
- Lang CE, Wagner JM, Edwards DF, et al. Upper extremity use in people with hemiparesis in the first few weeks after stroke. J Neurol Phys Ther. 2007;31(2):56–63.
- Leuenberger K, Gonzenbach R, Wachter S, et al. A method to qualitatively assess arm use in stroke survivors in the home environment. Med Biol Eng Comput. 2017;55(1):141–150.
- Lonini L, Dai A, Shawen N, et al. Wearable sensors for parkinson's disease: which data are worth collecting for training symptom detection models. NPJ Digit Med. 2018;1:64.
- Silfee VJ, Haughton CF, Jake-Schoffman DE, et al. Objective measurement of physical activity outcomes in lifestyle interventions among adults: a systematic review. Prev Med Rep. 2018;11:74–80.
- Suzuki M, Mitoma H, Yoneyama M. Quantitative analysis of motor status in parkinson's disease using wearable devices: from methodological considerations to problems in clinical applications. Parkinsons Dis. 2017;2017:6139716.
- Arvidsson D, Fridolfsson J, Borjesson M, et al. Re-examination of accelerometer data processing and calibration for the assessment of physical activity intensity. Scand J Med Sci Sports. 2019;29(10):1442–1452.
- Leeger-Aschmann CS, Schmutz EA, Zysset AE, et al. Accelerometer-derived physical activity estimation in preschoolers - comparison of cut-point sets incorporating the vector magnitude vs the vertical axis. BMC Public Health. 2019;19(1):513.
- Bailey RR, Klaesner JW, Lang CE. An accelerometry-based methodology for assessment of real-world bilateral upper extremity activity. PLoS One. 2014;9(7):e103135.
- Lang CE, Waddell KJ, Klaesner JW, et al. A method for quantifying upper limb performance in daily life using accelerometers. J Vis Exp. 2017;(122):55673. DOI:10.3791/55673.
- Little VL, Perry LA, Mercado MWV, et al. Gait asymmetry pattern following stroke determines acute response to locomotor task. Gait Posture. 2020;77:300–307.
- Nasreddine ZS, Phillips NA, Bedirian V, et al. The montreal cognitive assessment, MoCA: a brief screening tool for mild cognitive impairment. J Am Geriatr Soc. 2005;53(4):695–699.
- Hermodsson Y, Ekdahl C. Early planning of care and rehabilitation after amputation for vascular disease by means of katz index of activities of daily living. Scand J Caring Sci. 1999;13(4):234–239.
- Penta M, Tesio L, Arnould C, et al. The ABILHAND questionnaire as a measure of manual ability in chronic stroke patients: Rasch-based validation and relationship to upper limb impairment. Stroke. 2001;32(7):1627–1634.
- Penta M, Thonnard JL, Tesio L. ABILHAND: a rasch-built measure of manual ability. Arch Phys Med Rehabil. 1998;79(9):1038–1042.
- Lyden P. Using the national institutes of health stroke scale: a cautionary tale. Stroke. 2017;48(2):513–519.
- Dang M, Ramsaran KD, Street ME, et al. Estimating the accuracy of the Chedoke-McMaster stroke assessment predictive equations for stroke rehabilitation. Physiother Can. 2011;63(3):334–341.
- Gowland C, Stratford P, Ward M, et al. Measuring physical impairment and disability with the Chedoke-McMaster stroke assessment. Stroke. 1993;24(1):58–63.
- Uswatte G, Giuliani C, Winstein C, et al. Validity of accelerometry for monitoring real-world arm activity in patients with subacute stroke: evidence from the extremity constraint-induced therapy evaluation trial. Arch Phys Med Rehabil. 2006;87(10):1340–1345.
- Choi L, Liu Z, Matthews CE, et al. Validation of accelerometer wear and nonwear time classification algorithm. Med Sci Sports Exerc. 2011;43(2):357–364.
- Hernandez H, Myers SA, Schieber M, et al. Quantification of daily physical activity and sedentary behavior of claudicating patients. Ann Vasc Surg. 2019;55:112–121.
- Copeland JL, Esliger DW. Accelerometer assessment of physical activity in active, healthy older adults. J Aging Phys Act. 2009;17(1):17–30.
- Schober P, Boer C, Schwarte LA. Correlation coefficients: Appropriate use and interpretation. Anesth Analg. 2018;126(5):1763–1768.
- Lee JH, Park JH, Kim YJ. Sensitivity of the accelerometer as a measurement tool for upper extremity movement by stroke patients: a comparison with the action research arm test. J Phys Ther Sci. 2015;27(4):1053–1054.
- Murphy MAA, Danielsson A, Wipenmyr J, et al. Comparison of accelerometer-based arm, leg and trunk activity at weekdays and weekends during subacute inpatient rehabilitation after stroke. J Rehabil Med. 2019;51(6):426–433.
- van der Pas SC, Verbunt JA, Breukelaar DE, et al. Assessment of arm activity using triaxial accelerometry in patients with a stroke. Arch Phys Med Rehabil. 2011;92(9):1437–1442.
- Thrane G, Emaus N, Askim T, et al. Arm use in patients with subacute stroke monitored by accelerometry: association with motor impairment and influence on self-dependence. J Rehabil Med. 2011;43(4):299–304.
- Roby-Brami A, Feydy A, Combeaud M, et al. Motor compensation and recovery for reaching in stroke patients. Acta Neurol Scand. 2003;107(5):369–381.
- Liu W, McCombe Waller S, Kepple TM, et al. Compensatory arm reaching strategies after stroke: induced position analysis. J Rehabil Res Dev. 2013;50(1):71–84.
- Hayward KS, Eng JJ, Boyd LA, et al. Exploring the role of accelerometers in the measurement of real world Upper-Limb use after stroke. Brain Impair. 2016;17(1):16–33.