Abstract
Haslea provincialis Gastineau, Hansen & Mouget, sp. nov., is a new, morphologically semicryptic blue diatom discovered on the French shores of the Mediterranean Sea. Like H. ostrearia and H. karadagensis, H. provincialis shares the capacity to synthesize a marennine-like blue pigment. Sexual reproduction between clones of H. provincialis has been repeatedly observed and resulted in viable initial cells. There were no sexual interactions with sexually competent clones of H. ostrearia or H. karadagensis, as would be expected for a separate biological species. There are strong similarities between the H. provincialis pigment and the marennine produced by H. ostrearia, evidenced by UV-visible spectrophotometry and Raman spectrometry. However, unlike the marennine from H. ostrearia, no differences were found between the extracellular and the intracellular forms of the pigment in H. provincialis. This indicates that the synthesis pathways and excretion mechanisms among the three ‘blue’ Haslea may be species-specific. Molecular taxonomy and phylogeny (based on rbcL, cox1 and SSU V4 DNA sequences) confirmed the distinct position of this species among the blue Haslea species. Haslea provincialis occurs in environments from which H. ostrearia has already been reported (mostly based on the presence of the blue cell vacuoles). Possible species misidentifications and the impact of the complex geological history of the Mediterranean Sea on blue diatom diversification are also discussed.
Introduction
The relatively small diatom genus Haslea Simonsen, with its generitype H. ostrearia (Gaillon) Simonsen was established by Simonsen (Citation1974). Haslea ostrearia was first identified as an animal, Vibrio ostrearius (Gaillon, Citation1820) and later reclassified in a third intermediary reign (‘psychodiaire’) by Bory (Citation1824) as Navicula ostrearia. It is famous for producing marennine, a blue pigment responsible for the greening of oysters (Sprat, Citation1669; Gaillon, Citation1820; Lankester, Citation1886), a phenomenon which occasionally occurs in ponds used for fattening oysters in the Western part of France. For 150 years, many authors recorded any blue diatoms worldwide as H. ostrearia (Gastineau et al., Citation2012, Citation2014), including on the coasts of the Mediterranean Sea. For example, Sauvageau (Citation1906) described N. ostrearia living as an epiphyte on the ectocarpalean Liebmannia leveillei J. Agardh in Banyuls (southern France). Similar diatoms were found on Padina sp. in the Gulf of Naples (Funk, Citation1919), and on the shores of different Italian islands (Solazzi & Tolomio, Citation1976; Tolomio, Citation1978; Barone, Citation1979), the Tyrrhenian Sea (Tolomio, Citation1984) and Adriatic (Molisch, Citation1903; Höfler et al., Citation1956; Solazzi et al., Citation1972; Tolomio, Citation1976, Citation1982, Citation1988; Tolomio & Bullo, Citation2001a, Citation2001b), and on the African side of the Mediterranean Sea in the Suez Canal (Madkour et al., Citation2007). In most of these cases, diatom identification was usually based on light microscopy (LM) only, and concerning N. ostrearia, the decisive feature was the presence of blue tips. After 1974, in accordance with Simonsen’s new classification, the name Haslea ostrearia gradually replaced N. ostrearia. In addition to the obvious pennate lanceolate shape, the main typical character of the genus Haslea is a two layer ‘sandwich-structured’ valve (Sterrenburg et al., Citation2015), with continuous longitudinal slits for the valve exterior and quadrangular areolae for the valve interior. These features are impossible to observe without scanning electron microscopy (SEM). Since Simonsen’s (Citation1974) pioneering work, the development of SEM observations has allowed the discovery of new species and reclassification of Navicula, or Gyrosigma species into the genus Haslea (Poulin et al., Citation2004; Talgatti et al., Citation2014; Sterrenburg et al., Citation2015) and the genus now contains more than 30 species. Recently, an approach combining SEM, molecular barcoding and reproductive biology resulted in the discovery of H. karadagensis Davidovich, Gastineau & Mouget, a new species of blueish diatom in the Black Sea (Gastineau et al., Citation2012). The discovery of this new species, in the basin where H. ostrearia had previously been reported (Proshkina-Lavrenko, Citation1963), suggests that Haslea diversity had been underestimated there. This raises questions about earlier, similar reports such as the published observations on the Mediterranean Sea.
In July 2009, a strain of diatom with blue apices was isolated from French Mediterranean shores at Boulouris, and kept in the Scandinavian Culture Collection of Algae and Protozoa (SCCAP). This diatom differed from both H. ostrearia and H. karadagensis, and therefore more clones were regularly isolated from other samples collected in the same area, indicating that the species is a regular component of the local phytoplankton community. We here present the results of an integrated approach to test if this morphotype differs from currently known species of blue diatoms belonging to the genus Haslea. Morphological characterization and biometry were conducted using SEM. Mating experiments were attempted between strains collected from Boulouris, and strains of H. ostrearia and H. karadagensis. The blue pigment produced by the Mediterranean clones was purified and preliminarily characterized using UV-visible spectrophotometry and Raman spectrometry. Finally, in order to assess how this species differs from and/or is similar to currently known blue diatom species, molecular barcoding was performed. Three different markers were used, representing parts of the three eukaryotic genomes found in phototrophs, the chloroplast rbcL gene (Mann et al., Citation2010; Gastineau et al., Citation2012), a fragment of the mitochondrial cox1 gene (Gastineau et al., Citation2013), and the V4 section of the nuclear 18S rDNA marker (Luddington et al., Citation2012).
Materials and methods
Diatom sampling, isolation and culture
All clones of H. provincialis originated from the beaches of Boulouris (43° 2′ 45″ N, 6° 47′ 42″ E), Côte d’Azur, France. The first was isolated in 2009, referenced SCCAP K-1343 by the Scandinavian Culture Collection of Algae and Protozoa (Copenhagen). This clone no longer exists, due to undergoing detrimental size reduction. Between 2010 and 2014, more than 20 clones were isolated and established in cultures. Three of them were preserved for several months in the Nantes Culture Collection and registered as NCC 341, NCC 342 and NCC 343. The H. ostrearia strains NCC 148.14c and NCC 158.4 were provided by the Nantes Culture Collection, originating from the Bay of Bourgneuf (46° 58′ 27″ N, 1° 59′ 55″ W). The H. karadagensis strain NCC 313 derived from an initial cell produced in January 2009 from a cross between clones originating from Karadag, on the Black Sea shores (44° 54′ 40″ N, 35° 12′ 05″ E). The Haslea pseudostrearia Massé, Rincé & E.J. Cox clone SCCAP K-1594 originated from Robben Island (33° 47′ 54″ S, 18° 22′ 32″ E, South Africa) and no longer exists, due to reaching detrimental size reduction. All strains were cultured in 250 ml Erlenmeyer flasks with 150 ml modified artificial seawater (Perkins et al., Citation2006), and kept in a culture cabinet at a controlled temperature of 15±1 °C and an irradiance of 60 µmol photons m–2 s–1, provided by fluorescent tubes (Philips TLD 36W/965), during a 14:10 h light:dark cycle.
Microscopic examination
For microscopic examination, diatom frustules were cleaned using warm sulphuric and nitric acids, then washed 3–4 times in distilled water and filtered on PTFE membranes, following Kaczmarska et al. (Citation2005). Cleaned frustules were mounted on glass slides using Naphrax and examined using an Olympus BX51 light microscope with a DP72 digital camera, equipped for Phase Contrast and Differential Interference Contrast (DIC). For SEM observations, cleaned diatom frustules were air-dried onto a circular coverslip, mounted on an aluminium stub and coated with platinum, palladium-platinum using a JEOL JUC-5000 sputter coater or JEOL JFC-2300 HR high-resolution fine coater, respectively. For the SEM images obtained in Mount Allison University, the specimens were coated with c. 15 nm gold using a Hummer 6.2 sputtering system (Anatech USA, Union City, California) with argon as the source gas. Diatom frustules were examined using a JEOL 7600F SEM at Université de Nantes, France (, , ), a JEOL 6335F at University of Copenhagen, Denmark, and a JEOL JSM-5600 at Mount Allison University, Canada (, , ), at accelerating voltages from 5 to 10 kV.
Induction of sexual reproduction and auxosporulation
The sexual reproduction of H. provincialis, H. ostrearia and H. karadagensis was induced following Davidovich et al. (Citation2009) and Mouget et al. (Citation2009). Pairs of clones in exponential growth were mixed in sterile Petri dishes to a final concentration of 2000 cells ml–1 and maintained in a culture room at a temperature of 15±1°C and an irradiance of c. 30 µmol photons m–2 s–1 with a 8:16 h light:dark cycle. Signs of auxosporulation were monitored daily. All clones of H. provincialis were mated with each other. In addition, four clones of H. provincialis (SCCAP K-1343, NCC 341, NCC 342 and 343) were mated with clones of H. ostrearia (NCC 158.4 and NCC 148.14c) and H. karadagensis (NCC 313). Experiments were conducted at least three times with each combination of clone pairs.
Extraction and purification of the blue pigments
Haslea provincialis was cultivated in a semi-continuous mode, and the biomass and supernatant were collected during the exponential growth phase. After centrifugation, culture supernatants were filtered on 0.45 µm for the purification of the extracellular form. The cell pellets were crushed with a mortar and pestle in 250 mM NH4HCO3 buffer solution (pH 8) in liquid nitrogen. The crude extract containing the intracellular form was then centrifuged and the supernatant collected for purification. Intracellular and extracellular pigments were purified separately following Pouvreau et al. (Citation2006b). The method consists of a two-step ultra-filtration (30 kDa then 3 kDa cut-off membrane), followed by anion-exchange chromatography and dialysis. A total culture volume of 20 l, corresponding to c. 20 g fresh weight of algal biomass, was produced, resulting in c. 20 mg of pigment of each of the two forms (intracellular and extracellular).
UV-visible spectrophotometry
Purified pigments were dissolved in distilled water to reach a final concentration of 10 mg ml–1, then mixed directly in a Helmma quartz cell with 0.25 M phosphate buffer solutions previously filtered on a 0.22 µm membrane, with pH ranging from 2 to 12 (volumes of 50 µl of buffer and 50 µl of pigment solution). Spectra from 250 to 800 nm were recorded using a Thermo electron Helios gamma UV-visible spectrophotometer, with the blank being the buffer.
Raman spectrometry
The Raman spectra were recorded at room temperature in the backscattering configuration on a T64000 Jobin-Yvon (Horiba) spectrometer under a microscope with a 100× objective focusing the 514 nm line from an Argon–Krypton ion laser (coherent, Innova) onto the coloured apices of diatoms. The spot size of the laser was estimated to be 0.8 μm and the laser power was limited to 0.1 mW at the specimen to minimize possible sample deterioration. Raman spectra were recorded using a single stage monochromator configuration (600 lines mm–1 diffraction grating) in the wavenumber region 250–2250 cm–1 with a spectral resolution of 2 cm–1. For in vivo micro-Raman spectrometry, cells in the exponential phase of growth were placed in Petri dishes a few hours before measurements. In vivo Raman spectra were collected on a series of about 10 cells and averaged with an integration time ranging between 6–10 s (depending on the sample). For in vitro measurements, an amount of 200–500 µg of freeze-dried pigment of both intra- and extracellular forms was dissolved in 0.25 M Na2HPO4/NaH2PO4 buffer, pH 7 and then the mixture was placed on a glass slide and dried at room temperature prior to experiments. The laser beam, with the same configuration as above, was focused directly on dried pigments with an integration time varying between 200 s and 600 s. In vivo experiments were conducted on both H. provincialis and H. ostrearia and in vitro experiments were performed with purified intra- and extracellular pigments from the two species. Acquisition and basic treatments of spectra were made with LabSpec V5.25 (Jobin Yvon-Horiba) software. Raman spectra are presented in – without baseline correction, and systematically shifted for clarity.
Molecular characterization and phylogeny
DNA of all strains was extracted according to a modified version of Doyle & Doyle (Citation1990). All primers and their annealing temperatures are summarized in . PCR reactions were conducted with a GoTaq® Flexi DNA Polymerase kit from Promega. For a final volume of 25 µl, mixtures were done with Green GoTaq® Flexi buffer (1×), 2.5 mM MgCl2, 0.2 µM of primers, 200 µM of PCR nucleotide mix (Promega, France) and finally 2.5 U of GoTaq® Flexi DNA. The Eppendorf Mastercycler Gradient thermocycler conducted 35 cycles of polymerizations with 1 min denaturation at 94°C, 1 min annealing at variable temperatures, and times of elongation ranging from 1 min to 1 min 30 s at 72°C. Primer sequences, temperature of annealing and time of elongation are listed in . PCR products were separated by agarose gel electrophoresis and visualized using a CN-1000 Darkroom by Vilber Lourmat. Elution was with a Wizard® SV Gel and PCR Clean-up System by Promega. The sequencing reactions on the cleaned PCR products were carried out by Beckmann Coulter Genomics (UK, http://www.cogenicsonline.com). Chromatograms were verified using Chromas software (http://www.technelysium.com.au/chromas.html).
Table 1. Molecular markers and primers used to characterize Haslea provincialis.
Sequences were blasted to confirm identification (http://blast.ncbi.nlm.nih.gov/Blast.cgi). When required, protein translations were done using ExPASy (http://web.expasy.org/translate). Sequences were aligned using Clustal Omega (https://www.ebi.ac.uk/Tools/msa/clustalo) and monitored with GeneDoc (http://www.nrbsc.org/gfx/genedoc). Maximum Likelihood (ML) and Maximum Parsimony (MP) analyses were done using Mega 6 (Tamura et al., Citation2013) (http://www.megasoftware.net), with 1000 replicates for bootstrap analyses. Bayesian inference (BI) analyses were performed with MrBayes 3.2. with 107 generations (Ronquist et al., Citation2012). The best evolutionary model was chosen using jModelTest 2 (Guindon & Gascuel, Citation2003; Darriba et al., Citation2012), and was for both cases the GTR+I+G.
Results
Haslea provincialis Gastineau, Hansen et Mouget, sp. nov.
–, –
Description: Living cells with two narrow band-like plastids lying appressed on each side of the valve margin and not reaching the apex; cell tips characteristically blue colour. Frustules narrowly rectangular in girdle view (). Valves narrowly lanceolate with acute apices (, , ), only a faint striation visible in LM (), 51.5 to 102.4 µm long (average length 65.8 ± 0.1 µm long, mean ± SE, n = 26) and 5.8 to 9.0 µm wide (average width 7.4 ± 0.1 µm, mean ± SE, n = 26). The equation of the fitting line for the relationship between length and width is y = 0.017 x + 6.326, with an R2 of 0.078 (). In external view (SEM), raphe system consists of two straight branches running on the sternum (, –), with approximate (< 0.5 µm), co-axial or gently curved pore-like central endings (), and straight, elongated and expanded apical endings (). Striation consists of transapical and parallel rows of internal areolae crossed at a right angle by longitudinal rows. Externally, the valve face is ornamented by continuous longitudinal slits running parallel to the raphe from one valve apex to the other (, –), and merging along their course with a peripheral stria that meets at the far end of the valve (). In SEM internal view, the raphe consists of two straight branches which open laterally on an accessory rib, except at centre and near the apices of the valve (, –). The wider side has a thick ridge, so the raphe opening appears lateral. Central endings of the raphe closely disposed (). The raphe terminates at the apices in elongated and slightly expanded helictoglossae (). Striae composed of quadrangular areolae with hymenes (), forming a transapical pattern crossed at right angle by longitudinal slits, characteristic of Haslea species (). The density of transapical striae is 31 to 34 in 10 µm (average density of 32.7 ± 0.2 in 10 µm), the density of longitudinal striae is 55 to 64 in 10 µm (average density of 60.2 ± 0.2 in 10 µm).
Figs 1–2. Haslea provincialis clone SCCAP K-1343, LM. . Living cells, with parietal chloroplasts and some apices filled with blue pigment, others without. . Cleaned frustule, DIC (above) and phase-contrast (below), respectively. Faint striation is visible in DIC. Scale bars: 10 μm ().
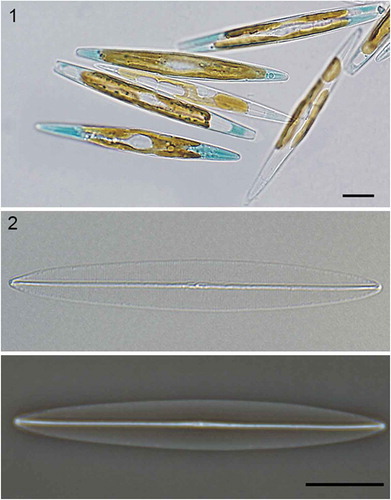
Figs 4–9. Haslea provincialis clone SCCAP K-1343, SEM. . Full view of the external valve face. . Full view of the internal valve face. . Apical raphe ending, external view. . Central raphe ending, external view. . Apical raphe ending, internal view. . Central raphe ending, internal view, areolae with cribrate-type hymenes (arrow). Scale bars: 1 µm (–, ), 0.1 µm (, –).
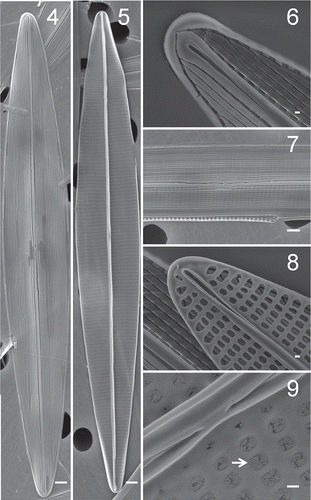
Holotype: Slide with acid cleaned embedded material of strain K-1343 has been deposited in the Danish Natural History Museum under accession number C-A-92076. was chosen to represent the type.
Isotypes: A SEM stub with acid cleaned valves of strain K-1343 has been deposited in the Danish Natural History Museum under the accession numbers C-A-92077 and C-A-92078, respectively.
Isotypes: –.
Type locality: Haslea provincialis was found in sandy beaches of rocky coves on the French Mediterranean coast in the area of Boulouris, France (43° 2’ 45” N, 6° 47’ 42” E), frequently associated with Padina sp., at depths less than 0.5 m.
Etymology: The species name refers to the Latin word ‘provincia’ that means ‘province’ and from which the word ‘Provence’ originates. Provence is the region along the south-east French Mediterranean coast where the species has been isolated.
Differential diagnosis: Haslea provincialis presents most of the morphological features already observed on the valve of two other species of blue diatoms (Gastineau et al., Citation2012). However, stria density in H. provincialis (transapical striae 32.7 ± 0.2 in 10 µm, longitudinal striae 60.2 ± 0.2 in 10 µm) is closer to that observed in H. karadagensis (31.4 ± 0.2 and 57.8 ± 0.3 in 10 µm, respectively) (Gastineau et al., Citation2012), than in H. ostrearia (34.8 ± 0.2 and 53.3 ± 0.2 in 10 µm, respectively). The ratio of longitudinal to transapical stria density is 1.6 in H. provincialis, as compared with 1.5 in H. karadagensis and 1.2 in H. ostrearia; summarizes the diagnostic characters of these and two other similar species (H. pseudostrearia Massé, Rincé & Cox and H. crucigera [W. Smith] Simonsen).
Table 2. Biometric data and morphological features of Haslea provincialis (this study), compared with those of H. ostrearia, H. karadagensis, H. pseudostrearia and H. crucigera obtained from the literature (*: taken from Gastineau et al., Citation2012; **: taken from Massé et al., Citation2001). Values are given with Standard Error. NA: no data.
DNA sequence information: Sequences were deposited at GenBank under the following accession numbers: KT257732 (18S rDNA SSU-V4), KT257733 (cox1), KT257734 (rbcL).
Auxosporulation and reproductive isolation
No signs of sexual interactions were observed between sexually competent clones of H. provincialis and either H. ostrearia or H. karadagensis, but H. provincialis clones, NCC 341 and NCC 342 were sexually compatible. Pairing of the compatible parental cells (not shown) was followed by the formation of two gametes per cell (–). Syngamy occurred, resulting in zygotes (), which started growing and expanding as tubular auxospores (–). Eventually, initial cells were formed () and liberated from the perizonium. Gametes, zygotes, auxospores and initial cells all contained blue pigment (–). Both NCC 341 and NCC 342 were c. 50.0 µm long when mating experiments were conducted. During these experiments, several auxospores and a few initial cells were observed. The length of expanded auxospores ranged from 84.4 to 114.1 µm (average length 98.9 ± 1.3 µm, mean ± SE, n = 23), and width from 8.3 to 12.3 µm (average 10.4 ± 0.3 µm). The size of the initial cells ranged from 98.1 to 108.8 µm (average length 103.4 ± 2.2 µm, mean ± SE, n = 5). Once, a possibly triploid auxospore with three branches was found (). Its dimensions, measured from five different images, were (from arm extremities to barycentre): upper arm, 31.8 ± 0.2 µm long and 7.8 ± 0.1 µm wide; left arm, 37.9 ± 0.1 µm long and 7.5 ± 0.2 µm wide; right arm, 42.9 ± 0.1 µm long, and 9.5 ± 0.2 µm wide (all measurements are means ± SE, n = 5). The approximate values of the angles formed by the arms, measured on five different pictures of the same specimen, were: bottom angle 89.9 ± 0.7°; upper left angle 141.1 ± 0.5°; upper right angle 136.3 ± 1.2° (means ± SE, n = 5).
Figs 10–18. Sexual reproduction in Haslea provincialis. . Gametogenesis in the mixture of sexually compatible clones NCC 341 and NCC 342. Gametes released to the medium have low chance to fuse. . Gametes in the pair of gametangia shortly before fusion (arrow), note that the other pair of gametes probably started development earlier and has swelled (below). . Normally two pairs of gametes produced fuse to give rise to two zygotes (left); but sometimes, when more gametes fuse, a huge zygote results (right). . Two young auxospores. . Middle stage of auxospore growth with the development of the perizonium. . Unique triradiate and normal tubular (bi-radiate) auxospores. . Sometimes, in a pair of gametangia only one zygote passes to the stage of auxospore while the other one fails to develop. . Normally developing pair of auxospores. . Initial cell formed inside the auxospore. Scale bars: 20 μm ().
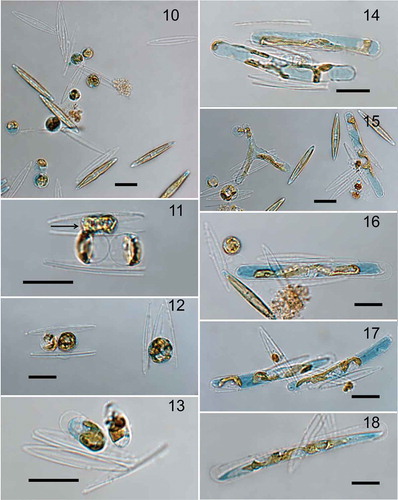
Pigment characterization
UV-visible spectrophotometry showed that the intra- and extracellular forms of H. provincialis blue pigment exhibited spectra with a single isobestic point at 640 nm. Both molecules absorb in the UV and visible regions, with absorbance peaks ranging from 570 nm (pH = 2) to 670 nm (pH = 12) (–).
Fig. 19. Absorbance spectrum of H. provincialis intracellular pigment, from 250 to 800 nm. Below and above the isobestic point (640 nm), pH 2–12 top to bottom and bottom to top, respectively.
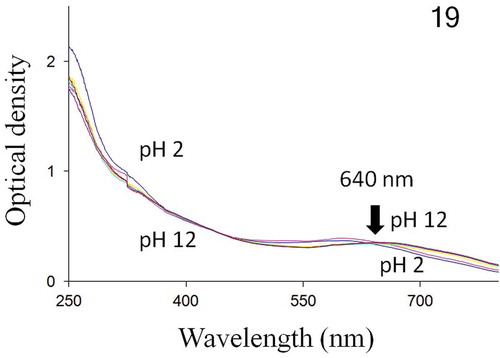
Fig. 20. Absorbance spectrum of H. provincialis extracellular pigment, from 250 to 800 nm. Below and above the isobestic point (640 nm), pH 2–12 top to bottom and bottom to top, respectively.
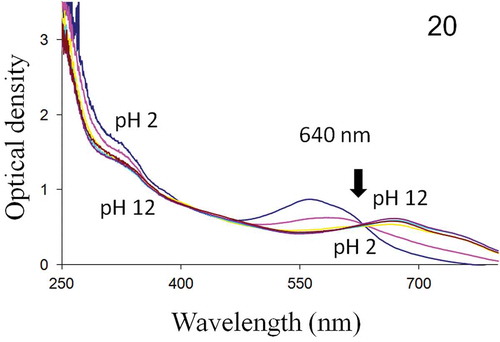
In vivo Raman measurements, performed on the apices of both H. ostrearia and H. provincialis, displayed similar spectra (), with strong peaks at 1163, 1211, 1294, 1464, 1577, 1607 and 1652 cm–1. The Raman spectra of the purified intracellular forms of the same diatom’s pigments are shown in and exhibit a series of broad bands in the wavenumber range 1200–1700 cm–1. Five small intensity peaks can also be distinguished at 1335, 1400, 1470, 1540 and 1610 cm–1, suggesting that the pigments share a number of functional groups and structural features. The Raman spectra of the purified extracellular forms of H. provincialis and H. ostrearia pigments are shown in . The spectrum of the extracellular marennine produced by H. ostrearia differs in both intensity and topography from the extracellular pigment of H. provincialis. There is a high intensity peak around 1470 cm–1 for H. ostrearia whereas H. provincialis has broader and flatter peaks between about 1300–1650 cm–1, very similar to the intracellular form.
Fig. 21. In vivo Raman spectra of H. provincialis and H. ostrearia, recorded at 514.5 nm on the coloured apices of cells.
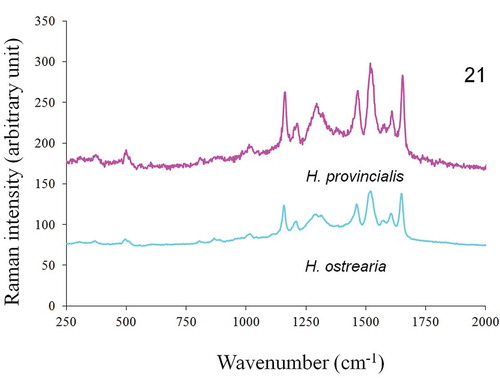
Molecular characterization
The Haslea provincialis rbcL sequence differs from H. ostrearia strains by 21 base pairs, whereas it differs from H. karadagensis by 19 bases. However, H. provincialis and H. karadagensis share common polymorphisms, compared with H. ostrearia. Hence, both species have C instead of T (357), TGT instead of GTA (715–717), G instead of A (777), T instead of C (840), another T instead of C (877), C instead of T (1047), C instead of A (1066), C instead of T (1068), A instead of G (1137), C instead of T (1188) and G instead of A (1204). There were 68 nucleotide differences in the cox1 fragments between H. provincialis and H. ostrearia, and 64 between H. provincialis and H. karadagensis. Twenty-six polymorphisms were common to H. provincialis and H. karadagensis and 33 to H. ostrearia and H. provincialis. With respect to the V4 part of the 18S rDNA gene (Zimmerman et al., Citation2011), there were 10 differences between H. provincialis and H. ostrearia, and 12 differences between H. provincialis and H. karadagensis. There were five polymorphisms common to H. provincialis and H. ostrearia, three common to H. provincialis and H. karadagensis, and six common between H. ostrearia and H. karadagensis. Concatenating these molecular markers, phylogenetic trees were constructed, which were rooted with Phaeodactylum tricornutum Bohlin and Synedra acus Kützing, for which an identical set of markers was available. Sequences used are listed in . All trees (bootstrap consensus trees for ML/MP, BI tree) show H. provincialis grouped weakly with H. karadagensis, with lower bootstrap values or posterior probabilities than the other nodes ( and ).
Table 3. List of the accession numbers of the sequences used in the phylogenetic analyses.
Discussion
For decades, Haslea ostrearia was considered the only diatom species that produces a highly specific blue pigment commonly called marennine (Gastineau et al., Citation2014). The discovery of several genetically distinct sub-populations of H. ostrearia worldwide (Gastineau et al., Citation2013, Citation2014), and of the new species H. karadagensis (Gastineau et al., Citation2012), suggests that species richness in this group is probably underestimated, and that still more new blue diatoms remain undescribed.
Morphology
Haslea provincialis cells are free living, but tend to form aggregates and films on the bottom of the flasks in culture. Apices are usually filled with a blue pigment, and monoclonal cultures appear green (). However, depending on the light conditions and age of the cultures, some cells may almost lack blue pigment (), which has probably contributed to the taxonomic confusion surrounding members of the genus. Bluishness of the apices is similar to those of H. ostrearia, and differs from the blue-grey colour of H. karadagensis (Gastineau et al., Citation2012).
Morphologically, H. provincialis is similar to two other blue Haslea species, H. ostrearia and H. karadagensis (Gastineau et al., Citation2012), with lanceolate valves, acute apices and rectangular frustules in girdle view. All cells have two band-like plastids in parietal positions. Cleaned valves embedded in Naphrax are hyaline (), with only the raphe and its central nodule distinguishable. The striation is barely visible in LM, and therefore is of limited use for routine species determination. All raphe endings are straight and the raphe is parallel to the longitudinal slits displayed by the valve exterior. As in other blue Haslea species, there is no pseudo-stauros. Areolae in H. provincialis are rectangular, occluded by hymenes (Ross et al., Citation1979; Round et al., Citation1990). Transapical stria density in H. provincialis is between those of H. ostrearia and H. karadagensis, whereas its longitudinal stria density is higher ().
Reproductive isolation and behaviour
Sexually competent clones of Haslea provincialis never showed any sign of sexual interactions with other blue Haslea species. They readily sexualized and auxosporulated with other clones of H. provincialis, using the same sex-induction protocol of light intensity and photoperiod as for H. ostrearia (Davidovich et al., Citation2009; Mouget et al., Citation2009) and H. karadagensis (Davidovich et al., Citation2012a). The maximal initial cell length in H. provincialis (109 µm) is closer to the maximum observed in H. karadagensis (97 µm, Davidovich et al., Citation2012a), than in H. ostrearia (143 µm, Davidovich et al., Citation2009). It must be noted, however, that most mating experiments were conducted when the mean cell length of the clones was c. 50 µm. As a consequence, it is impossible to precisely define the threshold of size for the induction of auxosporulation, which impacts the size of initial cells (Davidovich, Citation2001), thus indicating the need for more crossing experiments. One striking event which happened during the crossing experiments was the occurrence of a possible triploid auxospore, a rare phenomenon in diatoms but already noted in a few pennate species (Mann, Citation1994; Chepurnov et al., Citation2002, Citation2005; Poulíčková et al., Citation2007). The triploid auxospore presented an unusual triradiate shape, a feature rarely observed (Chepurnov & Roschin, Citation1995). Auxospore caps were only present on the two lower branches (). This auxospore was unlikely to develop to maturity, and we did not observe triradiate initial or vegetative cells. Haslea provincialis is only the fifth species of Haslea whose sexual reproduction has been shown and described. The four other Haslea species are: H. crucigera (Von Karsten, Citation1897), H. subagnita (Proshkina-Lavrenko) Makarova & Karayeva (Chepurnov, Citation1993), H. ostrearia (Neuville & Daste, Citation1975, Citation1979; Davidovich et al., Citation2009) and H. karadagensis (Davidovich et al., Citation2012a). They all seem to follow Geitler’s IB2a pattern (Geitler, Citation1973).
The blue pigment of Haslea provincialis
No difference has been detected between intra- and extracellular forms of the pigment produced by H. provincialis, in contrast to marennine in H. ostrearia (Pouvreau et al., Citation2006a, Citation2006b). Furthermore, UV-visible spectra of both forms of the H. provincialis pigment are very similar to those of intracellular marennine, with only one isobestic point at 640 nm (this study) and 650 nm (Pouvreau et al., Citation2006a), whereas extracellular marennine exhibits two isobestic points at 490 nm and 635 nm (Pouvreau et al., Citation2006a). The UV-visible spectrophotometry result was confirmed by Raman spectra recorded using pigments extracted from both species, the intracellular forms and the extracellular form of H. provincialis being similar and significantly different from extracellular marennine in H. ostrearia. The distinction between intra- and extracellular marennine in H. ostrearia has been documented by Pouvreau et al. (Citation2006a). A possible mechanism of pigment excretion has been suggested by the activity of specific vesicles inside H. ostrearia cells (Nassiri et al., Citation1998) and also from the cells to the environment by the presence of transitory external vesicles containing the pigment close to the apices (personal observations). It is possible that the two diatom species differ in the mechanism of pigment externalization and the physiological, biochemical and molecular bases of this phenomenon have still to be clarified. The distribution of a mechanism of synthesis and excretion among species of blue Haslea and its development during speciation constitute a promising field of research, adding interest to this peculiar microalgal model.
Molecular discrimination and phylogeny
In the present study, the choice was made to amplify the complete rbcL gene, as described in Evans et al. (Citation2007), in order to obtain long sequences and more information, but length was not the only factor taken into account. The 540 bp fragment used by MacGillivary & Kaczmarska (Citation2011), although less informative than the complete gene sequence, was more informative than the 748 bp suggested by Hamsher et al. (Citation2011). Indeed the 540 bp fragment revealed a potentially significant polymorphism, TGT rather than GTA at the 715–717 position, a difference leading to the replacement of a non-polar, aliphatic valine (in H. ostrearia) by a polar cysteine (in H. provincialis and H. karadagensis). This TGT modification can also be found in H. pseudostrearia. Another codon difference is found in H. karadagensis, with an ATG (for methionine) instead of a CAA (for leucine) as in the other Haslea species.
Concatenated trees, made by combining the V4-18S rDNA, cox1 and rbcL sequences and using various types of analyses (ML, MP and BI), display a similar topology, and all show H. karadagensis grouped with H. provincialis. However this node always appears the least robust, suggesting that the relationship between the three species is not fully resolved. Most of the morphological features observed in this study support this association. However, if we compare the spectrometry data, the H. provincialis blue pigment is definitely closer to marennine (as in H. ostrearia) than to the blue-grey pigment produced by H. karadagensis. Since no other blue Haslea collected between the Strait of Gibraltar and the Black Sea has been examined using methods described here, it would be premature to speculate whether the marennine-like blue pigment synthesized by H. ostrearia and H. provincialis is the most common in the genus, or if H. provincialis could be a ‘missing link’ between the Atlantic Ocean, H. ostrearia type, and the Black Sea, H. karadagensis type of blue Haslea, or which of these species is closer to an ‘ancestral type‘. Should a wider collection of strains and species of blue Haslea become available, it would be possible to better resolve their phylogeny, and to look for a relationship between their biogeography and biodiversity or functional traits.
Cryptic diversity and geographic separation
Cryptic species cannot be distinguished morphologically, but they present different physiological or life cycle traits and are incapable of interbreeding. The complex of blue Haslea species could thus represent an example of cryptic diversity in phytoplankton. Indeed, our results demonstrate that H. provincialis is very similar to H. ostrearia and to a lesser degree to H. karagadensis (), but none of them can interbreed with each other. Valve outline, proportions and ornamentation differ subtly between the three species, which can thus be considered ‘pseudocryptic’ (Vanormelingen et al., Citation2013). Pigmented cells of H. provincialis are difficult to distinguish from H. ostrearia, but the pigment colour distinguishes these two blue species from H. karagadensis. All three species are reproductively and genetically separated, even though their close phylogenetic relationship is clearly reflected by comparatively weak support for their clade. Moreover, the cleaned frustule of H. provincialis may be readily distinguished from the genetically close non-blue Haslea, H. pseudostrearia, by its finer longitudinal striation and other characters of the valve structure (, ). Together this suggests relatively recent separation of H. karagadensis and H. provincialis and H. ostrearia.
This work therefore strongly suggests that H. provincialis may have been observed in the Mediterranean Sea, reported by Sauvageau (Citation1906) and various Italian authors, but mistaken for H. ostrearia. Because of the lack of preserved specimens from these studies, no comparison is possible. Furthermore, this may not be the only misidentification of other blue diatoms as H. ostrearia. Indeed, H. karadagensis was discovered in a region where Mereschkowsky (Citation1903) and Proshkina-Lavrenko (Citation1963) reported H. ostrearia (as Navicula ostrearia) in their respective publications. Currently, H. karadagensis and H. provincialis distributions appear allopatric with each other but sympatric with H. ostrearia (if the earlier reports of this species are correct) in the region spanning from the Mediterranean coast of France to the Black Sea.
The geological history of the Mediterranean and Black Sea is complex and encompasses periods of closing/opening and water exchanges with the Atlantic Ocean and each other (Cavazza & Wezel, Citation2003), consequently followed by changes in the flora and fauna and introduction of foreign species (Hsü et al., Citation1977). Palaeogeographic, palaeogeological and climatic changes, as well as variation in seawater salinities because of streams, are all factors reported to play a role in biological speciation (Stefanni et al., 2002; Faria et al., Citation2006; Mäkinen & Merilä, 2007; Audzijonyte et al., Citation2008; Jesse et al., Citation2011; Mejri et al., Citation2011). In particular, salinity is known to be a potential factor promoting speciation (e.g. Wiens, Citation2004) or of ‘ecological’ speciation (e.g. Fuller et al., Citation2007) in some aquatic organisms, and studying its impact may evidence cryptic diversity (Koch & Ekelund, Citation2005). In other studies, however, no links have been found between variations in salinity and genetic divergence (De Vargas et al., Citation1999). Salinity obviously differs between the Atlantic Ocean waters where clones of H. ostrearia are commonly found (28–35‰ for the Bay of Bourgneuf and Marennes-Oléron), the Mediterranean Sea (> 38‰) and the Black Sea (c. 17‰). However, for blue Haslea, the impact of salinity on the speciation process may not be decisive, considering that the species so far investigated seem mostly euryhaline. Both H. ostrearia (Neuville & Daste, Citation1978; Wraige et al., Citation1998) and H. karadagensis (Davidovich et al., Citation2012a) are tolerant of abrupt changes in salinity. Moreover, euryhalinity is a peculiarity that could allow a wider dispersal and prevent the selection of ecotypes among populations. On several occasions, blue diatoms, identified as N. ostrearia, were found in different lagoons, some composed of brackish water, others with wide variations of salinity, ranging from c. 13‰ to 35‰, depending on the season and water depth (Tolomio, Citation1976). Salinity tolerance and/or preference for H. provincialis are not yet known.
Therefore, the influence of geographic isolation on speciation in blue diatoms must be considered, although there is no consensus about this impact on speciation in diatoms, and most reports of endemic diatom species have been made on freshwater species (Vanormelingen et al., Citation2008b). For example, Cermeño & Falkowski (Citation2009), whose studies were conducted on fossil diatoms, argue that geographic isolation has only a weak impact. Obviously, only the morphology of the frustules was used for species identification in such work. There are therefore no data on important features such as molecular divergence or reproductive isolation. On the other hand, these factors are taken into account in a study of the planktonic Pseudo-nitzschia pungens (Grunow ex Cleve) Hasle (Casteleyn et al., Citation2010), which suggested that a speciation process may be ongoing among geographically separated populations. More recently, Mann & Vanormelingen (Citation2013) proposed an ‘intermediate dispersal hypothesis’, which balances the roles of allopatric and parapatric speciation. It has recently been shown that a biogeographic speciation process may arise without selective pressure when the rate of neutral mutations exceeds the rate of oceanic dispersal, as demonstrated studying a prokaryotic model, the Pelagibacter marine bacteria (Giovannoni & Nemergut, Citation2014; Hellweger et al., Citation2014).
To date, H. karadagensis and H. provincialis have only been found in their respective areas of discovery, whereas H. ostrearia, previously hypothesized as tychopelagic (Robert, Citation1973, Citation1974) and thus not restricted to a benthic life, is more widely distributed with observations from the eastern Atlantic coast (France), the southwest coast of Sweden, the western Atlantic coast (USA) and the South Pacific Ocean (South Australia), evidenced using molecular markers. Interestingly, the molecular analyses reveal that each area is inhabited by a genetically distinct sub-population of H. ostrearia. One spans the Atlantic Ocean, the second is present in the Kattegat Strait between Sweden and Denmark, and the third inhabits Coffin Bay in Australia (Gastineau et al., Citation2013, Citation2014). Similar distribution patterns have also been documented for Thalassiosira rotula, another cosmopolitan diatom (Whittaker et al. Citation2012), supporting the notion that geographically specific distribution may occur among diatoms.
The blue members of the genus Haslea do not exhibit the strong morphological variations found among non-blue species, gigantic cells like H. gigantea (Hustedt) Simonsen, planktonic with very long, acute apices like H. wawrikae (Hustedt) Simonsen (Simonsen, Citation1974), with a pseudo-stauros like H. crucigera (W. Smith) Simonsen or H. salstonica Massé, Rincé & Cox (Massé et al., Citation2001), and they are always lanceolate and not sigmoid like H. nipkowii Poulin & Massé (Poulin et al., Citation2004) or H. sigma Talgatti, Sar & Carvalho Torgan (Talgatti et al., Citation2014). The differences are restricted to the striation patterns and are subtle, although statistically significant. On the other hand the genetic variation is unambiguous, especially in a strongly conserved marker such as the rbcL. But the most convincing evidence, congruent with the biological species concept, is the reproductive isolation between all these species. It has already been demonstrated that sexual reproduction can occur between specimens of clones derived from geographically distant (Kaczmarska et al., Citation2009) or sympatric (Vanormelingen et al., Citation2008a) sub-populations of a species, despite morphological and molecular differences. However, it should be kept in mind that mating systems in diatoms are complex and variable (Chepurnov et al., Citation2004). For example, Tabularia fasciculata (Kaczmarska et al., Citation2009) produces and releases motile male gametes distantly from their female counterpart (Davidovich et al., Citation2012b; Edgar et al., Citation2014). This does not require physical contact between the parental gametangia. In contrast, the mating system in all these blue Haslea requires the pairing of parental cells and physical cell-to-cell contact. All attempts to induce sexualization without physical contact between gametangia, such as using filtrate obtained from previous successful mating experiments possibly containing pheromones (cf. Sato et al., Citation2011), have been unsuccessful (unpublished). Thus, reproductive isolation among blue Haslea must be pre-zygotic, and no pairing has been observed between cells from combinations of different species in a same Petri dish. This suggests that in this group of species, mechanisms of cell–cell recognition and pairing evolved faster than valve morphology.
Acknowledgements
We would like to thank P. Gaudin for his help with SEM, and C. Tolomio for granting us complete access to his works.
Disclosure statement
No potential conflict of interest was reported by the author(s).
Additional information
Funding
Notes on contributors
Romain Gastineau
R. Gastineau: algal isolation and culture, planing of experiments, molecular analyses, interpretation of data, drafting and editing manuscript; G. Hansen: collection of the first strains of H. provincialis, pictures, and type material; N.A. Davidovich and O. Davidovich: breeding experiments; J.-F. Bardeau: RAMAN experiments and interpretation of data; I. Kaczmarska: interpretation of the phylogenetic trees; J.M. Ehrman: scanning electron microscopy; V. Leignel and Y. Hardivillier: help for molecular biology analyses; B. Jacquette: algal cultures and preparation of material for analyses; M. Poulin: taxonomy and valve morphological analyses; M. Morançais and J. Fleurence: extraction and purification of pigments; J.-L. Mouget: BIOVADIA PI, sampling and isolation of algal strains, conception of experiments, revising and editing manuscript. All authors read and approved the final manuscript.
Gert Hansen
R. Gastineau: algal isolation and culture, planing of experiments, molecular analyses, interpretation of data, drafting and editing manuscript; G. Hansen: collection of the first strains of H. provincialis, pictures, and type material; N.A. Davidovich and O. Davidovich: breeding experiments; J.-F. Bardeau: RAMAN experiments and interpretation of data; I. Kaczmarska: interpretation of the phylogenetic trees; J.M. Ehrman: scanning electron microscopy; V. Leignel and Y. Hardivillier: help for molecular biology analyses; B. Jacquette: algal cultures and preparation of material for analyses; M. Poulin: taxonomy and valve morphological analyses; M. Morançais and J. Fleurence: extraction and purification of pigments; J.-L. Mouget: BIOVADIA PI, sampling and isolation of algal strains, conception of experiments, revising and editing manuscript. All authors read and approved the final manuscript.
Nikolaï A. Davidovich
R. Gastineau: algal isolation and culture, planing of experiments, molecular analyses, interpretation of data, drafting and editing manuscript; G. Hansen: collection of the first strains of H. provincialis, pictures, and type material; N.A. Davidovich and O. Davidovich: breeding experiments; J.-F. Bardeau: RAMAN experiments and interpretation of data; I. Kaczmarska: interpretation of the phylogenetic trees; J.M. Ehrman: scanning electron microscopy; V. Leignel and Y. Hardivillier: help for molecular biology analyses; B. Jacquette: algal cultures and preparation of material for analyses; M. Poulin: taxonomy and valve morphological analyses; M. Morançais and J. Fleurence: extraction and purification of pigments; J.-L. Mouget: BIOVADIA PI, sampling and isolation of algal strains, conception of experiments, revising and editing manuscript. All authors read and approved the final manuscript.
Olga Davidovich
R. Gastineau: algal isolation and culture, planing of experiments, molecular analyses, interpretation of data, drafting and editing manuscript; G. Hansen: collection of the first strains of H. provincialis, pictures, and type material; N.A. Davidovich and O. Davidovich: breeding experiments; J.-F. Bardeau: RAMAN experiments and interpretation of data; I. Kaczmarska: interpretation of the phylogenetic trees; J.M. Ehrman: scanning electron microscopy; V. Leignel and Y. Hardivillier: help for molecular biology analyses; B. Jacquette: algal cultures and preparation of material for analyses; M. Poulin: taxonomy and valve morphological analyses; M. Morançais and J. Fleurence: extraction and purification of pigments; J.-L. Mouget: BIOVADIA PI, sampling and isolation of algal strains, conception of experiments, revising and editing manuscript. All authors read and approved the final manuscript.
Jean-François Bardeau
R. Gastineau: algal isolation and culture, planing of experiments, molecular analyses, interpretation of data, drafting and editing manuscript; G. Hansen: collection of the first strains of H. provincialis, pictures, and type material; N.A. Davidovich and O. Davidovich: breeding experiments; J.-F. Bardeau: RAMAN experiments and interpretation of data; I. Kaczmarska: interpretation of the phylogenetic trees; J.M. Ehrman: scanning electron microscopy; V. Leignel and Y. Hardivillier: help for molecular biology analyses; B. Jacquette: algal cultures and preparation of material for analyses; M. Poulin: taxonomy and valve morphological analyses; M. Morançais and J. Fleurence: extraction and purification of pigments; J.-L. Mouget: BIOVADIA PI, sampling and isolation of algal strains, conception of experiments, revising and editing manuscript. All authors read and approved the final manuscript.
Irena Kaczmarska
R. Gastineau: algal isolation and culture, planing of experiments, molecular analyses, interpretation of data, drafting and editing manuscript; G. Hansen: collection of the first strains of H. provincialis, pictures, and type material; N.A. Davidovich and O. Davidovich: breeding experiments; J.-F. Bardeau: RAMAN experiments and interpretation of data; I. Kaczmarska: interpretation of the phylogenetic trees; J.M. Ehrman: scanning electron microscopy; V. Leignel and Y. Hardivillier: help for molecular biology analyses; B. Jacquette: algal cultures and preparation of material for analyses; M. Poulin: taxonomy and valve morphological analyses; M. Morançais and J. Fleurence: extraction and purification of pigments; J.-L. Mouget: BIOVADIA PI, sampling and isolation of algal strains, conception of experiments, revising and editing manuscript. All authors read and approved the final manuscript.
James M. Ehrman
R. Gastineau: algal isolation and culture, planing of experiments, molecular analyses, interpretation of data, drafting and editing manuscript; G. Hansen: collection of the first strains of H. provincialis, pictures, and type material; N.A. Davidovich and O. Davidovich: breeding experiments; J.-F. Bardeau: RAMAN experiments and interpretation of data; I. Kaczmarska: interpretation of the phylogenetic trees; J.M. Ehrman: scanning electron microscopy; V. Leignel and Y. Hardivillier: help for molecular biology analyses; B. Jacquette: algal cultures and preparation of material for analyses; M. Poulin: taxonomy and valve morphological analyses; M. Morançais and J. Fleurence: extraction and purification of pigments; J.-L. Mouget: BIOVADIA PI, sampling and isolation of algal strains, conception of experiments, revising and editing manuscript. All authors read and approved the final manuscript.
Vincent Leignel
R. Gastineau: algal isolation and culture, planing of experiments, molecular analyses, interpretation of data, drafting and editing manuscript; G. Hansen: collection of the first strains of H. provincialis, pictures, and type material; N.A. Davidovich and O. Davidovich: breeding experiments; J.-F. Bardeau: RAMAN experiments and interpretation of data; I. Kaczmarska: interpretation of the phylogenetic trees; J.M. Ehrman: scanning electron microscopy; V. Leignel and Y. Hardivillier: help for molecular biology analyses; B. Jacquette: algal cultures and preparation of material for analyses; M. Poulin: taxonomy and valve morphological analyses; M. Morançais and J. Fleurence: extraction and purification of pigments; J.-L. Mouget: BIOVADIA PI, sampling and isolation of algal strains, conception of experiments, revising and editing manuscript. All authors read and approved the final manuscript.
Yann Hardivillier
R. Gastineau: algal isolation and culture, planing of experiments, molecular analyses, interpretation of data, drafting and editing manuscript; G. Hansen: collection of the first strains of H. provincialis, pictures, and type material; N.A. Davidovich and O. Davidovich: breeding experiments; J.-F. Bardeau: RAMAN experiments and interpretation of data; I. Kaczmarska: interpretation of the phylogenetic trees; J.M. Ehrman: scanning electron microscopy; V. Leignel and Y. Hardivillier: help for molecular biology analyses; B. Jacquette: algal cultures and preparation of material for analyses; M. Poulin: taxonomy and valve morphological analyses; M. Morançais and J. Fleurence: extraction and purification of pigments; J.-L. Mouget: BIOVADIA PI, sampling and isolation of algal strains, conception of experiments, revising and editing manuscript. All authors read and approved the final manuscript.
Boris Jacquette
R. Gastineau: algal isolation and culture, planing of experiments, molecular analyses, interpretation of data, drafting and editing manuscript; G. Hansen: collection of the first strains of H. provincialis, pictures, and type material; N.A. Davidovich and O. Davidovich: breeding experiments; J.-F. Bardeau: RAMAN experiments and interpretation of data; I. Kaczmarska: interpretation of the phylogenetic trees; J.M. Ehrman: scanning electron microscopy; V. Leignel and Y. Hardivillier: help for molecular biology analyses; B. Jacquette: algal cultures and preparation of material for analyses; M. Poulin: taxonomy and valve morphological analyses; M. Morançais and J. Fleurence: extraction and purification of pigments; J.-L. Mouget: BIOVADIA PI, sampling and isolation of algal strains, conception of experiments, revising and editing manuscript. All authors read and approved the final manuscript.
Michel Poulin
R. Gastineau: algal isolation and culture, planing of experiments, molecular analyses, interpretation of data, drafting and editing manuscript; G. Hansen: collection of the first strains of H. provincialis, pictures, and type material; N.A. Davidovich and O. Davidovich: breeding experiments; J.-F. Bardeau: RAMAN experiments and interpretation of data; I. Kaczmarska: interpretation of the phylogenetic trees; J.M. Ehrman: scanning electron microscopy; V. Leignel and Y. Hardivillier: help for molecular biology analyses; B. Jacquette: algal cultures and preparation of material for analyses; M. Poulin: taxonomy and valve morphological analyses; M. Morançais and J. Fleurence: extraction and purification of pigments; J.-L. Mouget: BIOVADIA PI, sampling and isolation of algal strains, conception of experiments, revising and editing manuscript. All authors read and approved the final manuscript.
Michèle Morançais
R. Gastineau: algal isolation and culture, planing of experiments, molecular analyses, interpretation of data, drafting and editing manuscript; G. Hansen: collection of the first strains of H. provincialis, pictures, and type material; N.A. Davidovich and O. Davidovich: breeding experiments; J.-F. Bardeau: RAMAN experiments and interpretation of data; I. Kaczmarska: interpretation of the phylogenetic trees; J.M. Ehrman: scanning electron microscopy; V. Leignel and Y. Hardivillier: help for molecular biology analyses; B. Jacquette: algal cultures and preparation of material for analyses; M. Poulin: taxonomy and valve morphological analyses; M. Morançais and J. Fleurence: extraction and purification of pigments; J.-L. Mouget: BIOVADIA PI, sampling and isolation of algal strains, conception of experiments, revising and editing manuscript. All authors read and approved the final manuscript.
Joël Fleurence
R. Gastineau: algal isolation and culture, planing of experiments, molecular analyses, interpretation of data, drafting and editing manuscript; G. Hansen: collection of the first strains of H. provincialis, pictures, and type material; N.A. Davidovich and O. Davidovich: breeding experiments; J.-F. Bardeau: RAMAN experiments and interpretation of data; I. Kaczmarska: interpretation of the phylogenetic trees; J.M. Ehrman: scanning electron microscopy; V. Leignel and Y. Hardivillier: help for molecular biology analyses; B. Jacquette: algal cultures and preparation of material for analyses; M. Poulin: taxonomy and valve morphological analyses; M. Morançais and J. Fleurence: extraction and purification of pigments; J.-L. Mouget: BIOVADIA PI, sampling and isolation of algal strains, conception of experiments, revising and editing manuscript. All authors read and approved the final manuscript.
Jean-Luc Mouget
R. Gastineau: algal isolation and culture, planing of experiments, molecular analyses, interpretation of data, drafting and editing manuscript; G. Hansen: collection of the first strains of H. provincialis, pictures, and type material; N.A. Davidovich and O. Davidovich: breeding experiments; J.-F. Bardeau: RAMAN experiments and interpretation of data; I. Kaczmarska: interpretation of the phylogenetic trees; J.M. Ehrman: scanning electron microscopy; V. Leignel and Y. Hardivillier: help for molecular biology analyses; B. Jacquette: algal cultures and preparation of material for analyses; M. Poulin: taxonomy and valve morphological analyses; M. Morançais and J. Fleurence: extraction and purification of pigments; J.-L. Mouget: BIOVADIA PI, sampling and isolation of algal strains, conception of experiments, revising and editing manuscript. All authors read and approved the final manuscript.
References
- Audzijonyte, A., Daneliya, M.E., Mugue, N. & Väonölä, R. (2008). Phylogeny of Paramysis (Crustacea: Mysida) and the origin of Ponto-Caspian endemic diversity: resolving power from nuclear protein-coding genes. Molecular Phylogenetics and Evolution, 46: 738–759.
- Barone, R. (1979). La diatomee bentoniche del porto di Palermo. Giornale Botanico Italiano, 3: 187–295.
- Bory de Saint-Vincent, J.B. (1824). Encyclopédie méthodique. Agasse, Paris.
- Casteleyn, G., Leliaert, F., Backeljau, T., Debeer, A.E., Kotaki, Y., Rhodes, L., Lundholm, N., Sabbe, K. & Vyverman, W. (2010). Limits to gene flow in a cosmopolitan marine planktonic diatom. Proceedings of the National Academy of Sciences USA, 107: 12952–12957.
- Cavazza, W. & Wezel, F.C. (2003). The Mediterranean region – a geological primer. Episodes, 26: 160–168.
- Cermeño, P. & Falkowski, P.G. (2009). Controls on diatom biogeography in the ocean. Science, 325: 1539–1541.
- Chepurnov, V.A. (1993). Polovoj protsess u dvudomnoj vodorosli Haslea subagnita (Pr.-Lavr.) Makar. et Kar. (Bacillariophyta). Algologiya, 3: 37–40.
- Chepurnov, V.A. & Roschin, A.M. (1995). Inbreeding influence on sexual reproduction of Achnantes longipes Ag. (Bacillariophyta). Diatom Research, 10: 21–29.
- Chepurnov, V.A., Mann, D.G., Vyverman, W., Sabbe, K. & Danielidis, D.B. (2002). Sexual reproduction, mating system, and protoplast dynamics of Seminavis (Bacillariophyceae). Journal of Phycology, 38: 1004–1019.
- Chepurnov, V.A., Mann, D.G., Sabbe, K. & Vyverman, W. (2004). Experimental studies on sexual reproduction in diatoms. International Review of Cytology, 237: 91–154.
- Chepurnov, V.A., Mann, D.G., Sabbe, K., Vannerum, K., Casteleyn, G., Verleyen, E., Peperzak, L. & Vyverman, W. (2005). Sexual reproduction, mating system, chloroplast dynamics and abrupt cell size reduction in Pseudo-nitzschia pungens from the North Sea (Bacillariophyta). European Journal of Phycology, 40: 379–395.
- Darriba, D., Taboada, G.L., Doallo, R. & Posada, D. (2012). jModelTest 2: more models, new heuristics and parallel computing. Nature Methods, 9: 772.
- Daugbjerg, N. & Andersen, R.A. (1997). A molecular phylogeny of the heterokont algae based on analyses of chloroplast encoded rbcL sequence data. Journal of Phycology, 33: 1031–1041.
- Davidovich, N.A. (2001). Species specific sizes and size range of sexual reproduction in diatoms. In Proceedings of the 16th International Diatom Symposium (Economou-Amilli, A., editor), 191–196. Amvrosiou Press, Athens.
- Davidovich, N.A., Mouget, J.L. & Gaudin, P. (2009). Heterothallism in the pennate diatom Haslea ostrearia (Bacillariophyta). European Journal of Phycology, 44: 251–261.
- Davidovich, N.A., Gastineau, R., Gaudin, P., Davidovich, O.I. & Mouget, J.L. (2012a). Sexual reproduction in the newly-described blue diatom, Haslea karadagensis. Fottea, 12: 219–229.
- Davidovich, N.A., Kaczmarska, I., Karpov, S.A., Davidovich, O.I., MacGillivary, M.L. & Mather, L. (2012b). Mechanism of male gamete motility in araphid pennate diatoms from the genus Tabularia (Bacillariophyta). Protist, 163: 480–494.
- De Vargas, C., Norris, R., Zaninetti, L., Gibb, S.W. & Pawlowski, J. (1999). Molecular evidence of cryptic speciation in planktonic foraminifers and their relation to oceanic provinces. Proceedings of the National Academy of Sciences USA, 96: 2864–2868.
- Doyle, J.J. & Doyle, J.L. (1990). Isolation of plant DNA from fresh tissue. Focus, 12: 13–15.
- Edgar, R., Drolet, D., Ehrman, J.M. & Kaczmarska, I. (2014). Motile male gametes of the araphid diatom Tabularia fasciculata search randomly for mates. PLoS ONE 9(7): e101767. doi: 10.1371/journal.pone.0101767.
- Evans, K.M., Wortley, A.H. & Mann, D.G. (2007). An assessment of potential diatom “barcode” genes (cox1, rbcL, 18S and ITS rDNA) and their effectiveness in determining relationships in Sellaphora (Bacillariophyta). Protist, 158: 349–364.
- Faria, R., Weiss, S. & Alexandrino, P. (2006). A molecular phylogenetic perspective on the evolutionary history of Alosa spp. (Clupeidae). Molecular Phylogenetics and Evolution, 40: 298–304.
- Fuller, R.C., McGhee, K.E. & Schrader, M. (2007). Speciation in killifish and the role of salt tolerance. Journal of Evolutionary Biology, 20: 1962–1975.
- Funk, G. (1919). Notizen über Meeresdiatomeen. Berichte der Deutschen Botanischen Gesellschaft, 37: 187–192.
- Gaillon, B. (1820). Des huîtres vertes, et des causes de cette coloration. Journal de Physique, de Chimie, d’Histoire Naturelle et des Arts, 91: 222–225.
- Gastineau, R., Davidovich, N.A., Bardeau, J.-F., Caruso, A., Leignel, V., Hardivillier, Y., Jacquette, B., Davidovich, O., Rincé, Y., Gaudin, P., Cox, E. & Mouget, J.-L. (2012). Haslea karadagensis (Bacillariophyta): a second blue diatom, recorded from the Black Sea and producing a novel blue pigment. European Journal of Phycology, 47: 469–479.
- Gastineau, R., Leignel, V., Jacquette, B., Bendahmane, D., Hardivillier, Y., Wulff, A., Gaudin P., Kaczmarska, I., Davidovich, N. A. & Mouget, J.-L. (2013). Inheritance of mitochondrial DNA in the pennate diatom Haslea ostrearia (Naviculaceae) during auxosporulation suggests a uniparental transmission. Protist, 164: 340–351.
- Gastineau, R., Davidovich, N.A., Hansen, G., Rines, J., Wulff, A., Kaczmarska, I., Ehrman, J., Hermann, D., Maumus, F., Hardivillier, Y., Leignel, V., Jacquette, B., Méléder, V., Hallegraeff, G., Yallop, M., Perkins, R., Cadoret, J.-P., Saint-Jean, B., Carrier, G. & Mouget, J.-L. (2014). Haslea ostrearia-like diatoms: biodiversity out of the blue. Advances in Botanical Research, 71: 441–446.
- Geitler, L. (1973). Auxosporenbildung und Systematik bei pennaten Diatomeen und die Cytologie von Cocconeis-Sippen. Österreichische Botanische Zeitschrift, 122: 299–321.
- Giovannoni, S. & Nemergut, D. (2014). Microbes ride the current. Science, 345: 1246–1247.
- Guindon, S. & Gascuel, O. (2003). A simple, fast and accurate method to estimate large phylogenies by maximum-likelihood. Systematic Biology, 52: 696–704.
- Hamsher, S.E., Evans, K.M., Mann, D.G., Poulíčková, A. & Saunders, G.W. (2011). Barcoding diatoms: exploring alternatives to COI-5P. Protist, 162: 405–422.
- Hellweger, F.L., van Sebille, E. & Fredrick, N.D. (2014). Biogeographic patterns in ocean microbes emerge in a neutral agent-based model. Science, 345: 1346–1349.
- Höfler, K., Url, W. & Diskus, A. (1956). Zellphysiologie Beobachtungen und Versuche an Algen der Lagune von Venedig. Bollettino del Museo civico di Storia Naturale di Venezia, 9: 63–94.
- Hsü, K.J., Montadert, L., Bernoulli, D., Cita, M.B., Erickson, A., Garrison, R.E., Kidd, R.B., Mèlierés, F., Müller, C. & Wright, R. (1977). History of the Mediterranean salinity crisis. Nature, 267: 399–403.
- Jesse, R., Grudinski, M., Klaus, S., Streit, B. & Pfenninger, M. (2011). Evolution of freshwater crab diversity in the Aegean region (Crustacea: Brachyura: Potamidae). Molecular Phylogenetics and Evolution, 59: 23–33.
- Jones, H.M., Simpson, G.E., Stickle, A.J. & Mann, D.G. (2005). Life history and systematics of Petroneis (Bacillariophyta) with special reference to British waters. European Journal of Phycology, 40: 61–87.
- Kaczmarska, I., LeGresley, M.M., Martin, J.L. & Ehrman, J.M. (2005). Diversity of the diatom genus Pseudo-nitzschia Peragallo in the Quoddy region of the Bay of Fundy, Canada. Harmful Algae, 4: 1–19.
- Kaczmarska, I., Ehrman, J.M., Moniz, M.B.J. & Davidovich, N.A. (2009). Phenotypic and genetic structure of interbreeding populations of the diatom Tabularia fasciculata (Bacillariophyta). Phycologia, 48: 391–403.
- Koch, T.A. & Ekelund, F. (2005). Strains of the heterotrophic flagellate Bodo designis from different environments vary considerably with respect to salinity preference and SSU rRNA gene composition. Protist, 156: 97–112.
- Lankester, E.R. (1886). On green oysters. Quarterly Journal of Microscopical Science, 26: 71–94.
- Luddington, I.A., Kaczmarska, I. & Lovejoy, C. (2012). Distance and character-based evaluation of the V4 region of the 18S rRNA gene for the identification of diatoms (Bacillariophyceae). PLoS ONE, 7(9): e45664. doi:10.1371/journal.pone.0045664.
- MacGillivary, M.L. & Kaczmarska, I. (2011). Survey of the efficacy of a short fragment of the rbcL gene as a supplemental DNA barcode for diatoms. Journal of Eukaryotic Microbiology, 58: 529–536.
- Madkour, F.A., Dorgham, M.M., Hanafi, M.H. & Holligan, P.M. (2007). Comprehensive hydro-biological observations on the Suez Canal. International Journal of Oceans and Oceanography, 2: 125–137.
- Mäkinen, H.S. & Merilä, J. (2008). Mitochondrial DNA phylogeography of the three-spined stickleback (Gasterosteus aculeatus) in Europe – evidence for multiple glacial refugia. Molecular Phylogenetics and Evolution, 46: 167–182.
- Mann, D.G. (1994). Auxospore formation, reproductive plasticity and cell structure in Navicula ulvacea and the resurrection of the genus Dickieia (Bacillariophyta). European Journal of Phycology, 29: 141–157.
- Mann, D.G. & Vanormelingen, P. (2013). An inordinate fondness? The number, distributions and origins of diatom species. Journal of Eukaryotic Microbiology, 60: 414–420.
- Mann, D.G., Sato, S., Trobajo, R., Vanormelingen, P. & Souffreau, C. (2010). DNA barcoding for species identification and discovery in diatoms. Cryptogamie Algologie, 31: 557–577.
- Massé, G., Rincé, Y., Cox, E.J., Allard, G., Belt, S.T. & Rowland, S.J. (2001). Haslea salstonica sp. nov. and Haslea pseudostrearia sp. nov. (Bacillariophyta), two new epibenthic diatoms from the Kingsbridge estuary, United Kingdom. Comptes Rendus de l’Académie des Sciences de Paris, Sciences de la vie, 324: 617–626.
- Mejri, R., Arculeo, M., Ben Hassine, O.K. & Lo Brutto, S. (2011). Genetic architecture of the marbled goby Pomatoschistus marmoratus (Perciformes, Gobiidae) in the Mediterranean Sea. Molecular Phylogenetics and Evolution, 58: 395–403.
- Mereschkowsky, K.S. (1903). K morfologii diatomovyh vodoroslej. Tipo-litografіya Imperatorskago Universiteta, Kazan.
- Molisch, H. (1903). Notiz über eine blaue Diatomee. Bericht der Deutschen botanischen Gesellschaft, 21: 23–26.
- Mouget, J.L., Gastineau, R., Davidovich, O., Gaudin, P. & Davidovich, N.A. (2009). Light is a key factor in triggering sexual reproduction in the pennate diatom Haslea ostrearia. FEMS Microbiology Ecology, 69: 194–201.
- Nassiri, Y., Robert, J.-M., Rincé, Y. & Ginsburger-Vogel, T. (1998). The cytoplasmic fine structure of the diatom Haslea ostrearia (Bacillariophyceae) in relation to marennine production. Phycologia, 37: 84–91.
- Neuville, D. & Daste, P. (1975). Observations préliminaires concernant l’auxosporulation chez la diatomée Navicula ostrearia (Gaillon) Bory en culture in vitro. Comptes Rendus de l’Academie des Sciences., 281 (Série D): 1753–1756.
- Neuville, D. & Daste, P. (1978). Recherches sur le déterminisme de la production de marennine par la diatomée marine Navicula ostrearia (Gaillon) Bory en culture in vitro. Revue générale de botanique, 85: 255–303.
- Neuville, D. & Daste, P. (1979). Observations concernant les phases de l’auxosporulation chez la diatomée Navicula ostrearia (Gaillon) Bory en culture in vitro. Comptes Rendus de l’Academie des Sciences, 288 ( Série D): 1496–1498.
- Perkins, R.G., Mouget, J.-L., Lefebvre, S. & Lavaud, J. (2006). Light response curve methodology and possible implications in the application of chlorophyll fluorescence to benthic diatoms. Marine Biology, 149: 703–712.
- Poulíčková, A., Mayama, S., Chepurnov, V.A. & Mann, D.G. (2007). Heterothallic auxosporulation, incunabula and perizonium in Pinnularia (Bacillariophyceae). European Journal of Phycology, 42: 367–390.
- Poulin, M., Massé, G., Belt, S.T., Delavault, P., Rousseau, F., Robert, J.M. & Rowland, S.J. (2004). Morphological, biochemical and molecular evidence for the transfer of Gyrosigma nipkowii Meister to the genus Haslea (Bacillariophyta). European Journal of Phycology, 39: 181–195.
- Pouvreau J.B., Morançais, M., Fleury, F., Rosa, P., Thion, L., Cahingt, B., Zal, F., Fleurence, J. & Pondaven, P. (2006a). Preliminary characterisation of the blue-green pigment ‘‘marennine’’ from the tychopelagic diatom Haslea ostrearia (Gaillon/Bory) Simonsen. Journal of Applied Phycology, 18: 757–767.
- Pouvreau, J.B., Morançais, M., Massé, G., Rosa, P., Robert, J.-M., Fleurence, J. & Pondaven, P. (2006b). Purification of the blue-green pigment “marennine” from the marine tychopelagic diatom Haslea ostrearia (Gaillon/Bory) Simonsen. Journal of Applied Phycology, 18: 769–781.
- Proshkina-Lavrenko, A.I. (1963). Diatomovye vodorosli bentosa Chernogo moria. Akademii Nauk SSSR, Leningrad.
- Robert, J.-M. (1973). La diatomée Navicula ostrearia Bory en baie de Bourgneuf. Revue des Travaux de l’Institut des Pêches Maritimes, 37: 363–368.
- Robert, J.-M. (1974). Etude préliminaire des relations entre l’état de verdissement d’une claire ostréicole et l’examen biométrique de populations planctoniques et benthiques du Navicula ostrearia Bory, pigmenté; applications. Comptes Rendus de l’Académie des Sciences – Série D – Sciences Naturelles, 278: 1405–1408.
- Ronquist, F., Teslenko, M., van der Mark, P., Ayres, D.L., Darling, A., Höhna, S., Larget, B., Liu, L., Suchard, M.A. & Huelsenbeck, J.P. (2012). MrBayes 3.2: efficient Bayesian phylogenetic inference and model choice across a large model space. Systematic Biology, 61: 539–542.
- Ross, R., Cox, E.J., Karayeva, N.I., Mann, D.G., Paddock, T.B.B., Simonsen, R. & Sims, P.A. (1979). An amended terminology for the siliceous components of the diatom cell. Nova Hedwigia, 64: 513–533.
- Round, F.E., Crawford, R.M. & Mann, D.G. (1990). The Diatoms. Biology and Morphology of the Genera. Cambridge University Press, Cambridge.
- Sato, S., Beakes, G., Idei, M., Nagumo, T. & Mann, D.G. (2011). Novel sex cells and evidence for sex pheromones in diatoms. PLoS ONE, 6(10): e26923. doi:10.1371/journal.pone.0026923.
- Sauvageau, C. (1906). A propos de la présence de la diatomée bleue dans la Méditerranée. Travaux des laboratoires (Arcachon), 6: 46–59.
- Simonsen, R. (1974). The diatom plankton of the Indian Ocean expedition of RV “Meteor”. “Meteor” Forschungsergebnisse, Reihe D, 19: 46–49.
- Solazzi, A. & Tolomio, C. (1976). Il fitoplancton primaverile ed estivo lungo le coste settentrionali della Sardegna. Archivio di Oceanografia e Limnologia, 18: 389–409.
- Solazzi, A., Tolomio, C., Andreoli, C., Caniglia, G. & Colliva, V. (1972). La Laguna di Lesina (Foggia). Carico microfitico in un ciclo annuale. Memorie di Biogeografica Adriatica, 9: 1–42.
- Sprat, T. (1669). The history of the generation and ordering of green Oysters, commonly called Colchester Oysters. History of the Royal Society (London), 307–319.
- Sterrenburg, F.A.S., Tiffany, M.A., Hinz, F., Herwig, W.E. & Hargraves, P.E. (2015). Seven new species expand the morphological spectrum of Haslea. A comparison with Gyrosigma and Pleurosigma (Bacillariophyta). Phytotaxa, 207: 143–162.
- Talgatti, D., Sar, E.A. & Carvalho Torgan, L. (2014). Haslea sigma (Naviculaceae, Bacillariophyta) a new sigmoid benthic species from salt marshes of Southern Brazil. Phytotaxa, 177: 231–238.
- Tamura, K., Stecher, G., Peterson, D., Filipski, A. & Kumar, S. (2013). MEGA6: Molecular Evolutionary Genetics Analysis version 6.0. Molecular Biology and Evolution, 30: 2725–2729.
- Tolomio, C. (1976). Variazioni stagionali e stazionali del firoplancton nella Laguna di Marano (Udine). Pubblicazioni della Stazione Zoologica di Napoli, 40: 133–237.
- Tolomio, C. (1978). Catalogo delle diatomee e delle peridinee più significative segnalate nelle acque salmastre italiane. Memorie di Biologia Marina e di Oceanografia, 8: 129–150.
- Tolomio, C. (1982). Ricerche sul fitoplancton e su alcuni fattori ambientali nella laguna di grado (Gorizia). Rivista di Idrobiologia, 21: 75–96.
- Tolomio, C. (1984). Osservazioni a breve termine sulla microflora pelagica estiva in due stazioni costiere nel Tirreno Settentrionale. Quaderni del Museo di Storia Naturale di Livorno, 5: 61–68.
- Tolomio, C. (1988). Il fitoplancton della valle di brenta (laguna veneta). Indagini stagionali: giugno 1980-marzo 1982. Archivio di Oceanografia e Limnologia, 21: 117–150.
- Tolomio, C. & Bullo, L. (2001a). Influenza delle maree di sizigie e di quadratura sulle comunità fitoplanctoniche del bacino di chioggia. Lavori Società Veneziana di Scienze Naturali, 26: 53–69.
- Tolomio, C. & Bullo, L. (2001b). Prelievi giornalieri di fitoplancton in una stazione del bacino meridionale della Laguna di Venezia. Bollettino del Museo Civico di Storia Naturale di Venezia, 52: 1–23.
- Vanormelingen, P., Chepurnov, V.A., Mann, D.G., Sabbe, K. & Vyverman, W. (2008a). Genetic divergence and reproductive barriers among morphologically heterogeneous sympatric clones of Eunotia bilunaris sensu lato (Bacillariophyta). Protist, 159: 73–90.
- Vanormelingen, P., Verleyen, E., & Vyverman, W. (2008b). The diversity and distribution of diatoms: from cosmopolitanism to narrow endemism. Biodiversity and Conservation, 17: 393–405.
- Vanormelingen, P., Evans, K.M., Chepurnov, V.A., Vyverman, W. & Mann, D.G. (2013). Molecular species discovery in the diatom Sellaphora and its congruence with mating trials. Fottea, 13: 133–148.
- Von Karsten, G. (1897). Untersuchungen über Diatomeen. III. Flora, 83: 203–221.
- Whittaker, K.A., Rignanese, D.R., Olson, R.J. & Rynearson, T.A. (2012). Molecular subdivision of the marine diatom Thalassiosira rotula in relation to geographic distribution, genome size, and physiology. BMC Evolutionary Biology, 12: 209.
- Wiens, J.T. (2004). What is speciation and how should we study it? American Naturalist, 163: 914–923.
- Wraige, E.J., Belt, S.T., Massé, G., Robert, J.M. & Rowland, S.J. (1998). Variations in distributions of C25 highly branched isoprenoid (HBI) alkenes in the diatom, Haslea ostrearia: influence of salinity. Organic Geochemistry, 28: 855–859.
- Zimmermann, J., Jahn, R. & Gemeinholzer, B. (2011). Barcoding diatoms: evaluation of the V4 subregion on the 18S rRNA gene, including new primers and protocols. Organisms, Diversity and Evolution, 11: 173–192.