ABSTRACT
Chicken heterophils serve as the first-line defence in chicken innate immunity. The present study aimed to explore the effects of quercetin, a herbal phenolic substance known for its inflammatory modulation, on mRNA expression patterns in chicken heterophils. Isolated heterophils from two genetically distinct chicken lines, hybrid commercial and Thai indigenous chickens, Pradu Hang Dam, were in vitro co-cultured with live intestinal pathogenic Salmonella enteritidis under Phosphate-buffered saline (PBS) or quercetin supplementation. Quantitative real-time RT-PCR was performed to investigate the effects of quercetin on mRNA transcripts of 12 genes. The results showed that heterophils from hybrid chickens treated with quercetin had higher relative expression levels of IL6, IL8L1, IL8L2, IL18, CCL4, LITAF, and MIF. Unlike hybrid chickens, Pradu Hang Dam chickens had down-regulation of cytokine genes, IL1B, IL6, IL8L1, IL8L2, IL12B, and IL18, and chemokines, CCL4 and MIF. In addition, NOS2, IL17A, and TGFB1 were up-regulated in this lineage. In conclusion, our findings emphasize the modulatory effects of quercetin to down-regulate most pro-inflammatory genes, whereas it up-regulates anti-inflammatory gene (TGFB1) in Pradu Hang Dam chickens.
1. Introduction
Heterophils serve as the first line of innate immunity in chicken and avian species. In fact, chicken heterophils are among the terminally differentiated cells that can swiftly secrete many cytokines and chemokines during the activation phase when encountering external pathogens such as Salmonella spp., Escherichia coli, and Eimeria spp. (Mellata et al. Citation2003; Hong et al. Citation2006; Redmond et al. Citation2009; Crhanova et al. Citation2011). In response to bacterial infection, heterophils are being expressed and secreted as a major source of pro-inflammatory cytokines such as IL-1β, IL-6 and IL-8, as well as chemokines in order to communicate between avian innate, adaptive immune cells and other affected tissues (Sick et al. Citation2000; Kaiser et al. Citation2005; Redmond et al. Citation2009). Chicken heterophils may be primed and later activated at the site of infection. The elevation or reduction in gene expression of heterophils after being stimulated with bacterial microbial-associated molecular patterns and other pathogens are nicely described with in-depth explorations (Kaiser et al. Citation2005; Chiang et al. Citation2008). The collection of knowledge regarding what type of cytokines, chemokines, antimicrobial peptides, and macromolecules are produced in response to Salmonella spp. are clearly reported in the literature (Withanage et al. Citation2004; Kaiser et al. Citation2005).
To date, many cytokines and chemokines initially identified in mammals have also been identified, characterized, and re-evaluated in chickens for their presence and functional conservation to the mammalian system (Kaiser et al. Citation2005). The constitutive expressions of pro-inflammatory cytokines and other genes in chicken appeared to be relevant in promoting, mounting, or putting host innate cells on alert, which leads to tissue inflammation and inevitable damages later (Swaggerty et al. Citation2004).
To manipulate and to resolve the inflammatory process occurring during an initial phase of bacterial infection or bacterial-causing inflammation, many studies have applied the natural bioflavonoid from plants, such as quercetin, which are ubiquitously present in many plant products (Formica and Regelson Citation1995; Wadsworth and Koop Citation1999). From various in vitro and in vivo studies, it has been revealed that quercetin possesses the effects of anti-inflammation, anti-oxidation, antiviral as well as other activities (Alzoreky and Nakahara Citation2003; Cho et al. Citation2003; Chuammitri et al. Citation2015; Nair et al. Citation2006). Many studies in human and mammalian neutrophils from the aspects of anti-inflammation at a molecular level provide an insight into the effects of this compound that quercetin greatly reduced gene expression, especially targeted genes that were involved in early immune responses or pro-inflammatory cytokines, including IL-1β, IL-6, TNF-α, MIP-1α/β, MIP-2, IL-8, IL-6, and iNOS, which is vital to the host defence mechanism in mammals (Cho et al. Citation2003; Liu et al. Citation2005; Geraets et al. Citation2009; Boesch-Saadatmandi et al. Citation2011; Askari et al. Citation2012; Chan et al. Citation2012; Das et al. Citation2012; Freitas et al. Citation2014; Chuammitri et al. Citation2015; Huang et al. Citation2015). In this regards, the knowledge of quercetin pertaining to chicken heterophils is inadequately established. This prompted us to question how this substance would exert its immunomodulatory effects in chicken heterophils from two genetically different chickens. To improve our understanding of chicken heterophils in response to bacterial infection at molecular and gene expression levels, we have utilized isolated heterophils from commercial hybrid chickens and the Thai indigenous chicken (TIC) breed, Pradu Hang Dam, as innate cell models. Chicken heterophils were supplemented with quercetin, in order to explore the property of these phenolic compounds on gene expressions by real-time PCRs.
2. Materials and methods
The reagents ethylenediaminetetraacetic acid (EDTA) powder, Hank's balanced salt solution without calcium and magnesium and phenol red (HBSS-CMF), Histopaque, methylcellulose trypan blue, and quercetin hydrate (QH) were ordered from Sigma-Aldrich (St. Louis, MO, USA). Foetal bovine serum (FBS), Gibco RPMI 1640 medium with phenol red, and RNAlater were obtained from Life Technologies (Carlsbad, CA, USA).
2.1. Birds
Commercial hybrid broiler chickens (Hybrid) aged range from 6 to 7 weeks (n = 36) were reared on a local farm in Chiang Mai, Thailand. Thai native chickens (Gallus domesticus), Pradu Hang Dam (Pradu) (Dorji et al. Citation2011; Akaboot et al. Citation2012), were reared on a Pradu Hang Dam Farm in Chiang Mai under promotions from the Research and Development Network Center for Animal Breeding (native chicken), Department of Livestock Development, Ministry of Agriculture and Cooperatives, Thailand. Eight-week-old chickens (n = 50) were used in this experiment. Pradu chickens had no vaccination history against Salmonella. Whole blood from hybrid or Pradu chickens were collected from wing veins (3 ml). Samples were mixed with EDTA solution (1% final concentration; Blood:EDTA v/v ratio was 10:1). Samples from groups of five to six individuals were pooled. The animal study protocol has been reviewed and approved by The Faculty of Veterinary Medicine, Chiang Mai University, Animal Care and Use Committee (FVM-ACUC) Ref. No. S11/2558.
2.2. Chicken heterophil isolation
Isolations of chicken heterophils from healthy hybrid or Pradu chickens were performed according to a previously described technique with a slight modification (Chuammitri et al. Citation2011). The purity of cells was more than 90% as determined under a microscope. The trypan blue was used to determine heterophil viability and cell density was adjusted to 1 × 106 cells/ml.
2.3. Quercetin
QH with purity of ≥95% by HPLC and containing ≥2.9% water as determined by Karl Fischer was used. Details regarding the preparation of stock quercetin solution (5 mM) and working solution (50 μM) were previously described (Chuammitri et al. Citation2015). This concentration was previously determined to be safe to use for stimulation of chicken heterophils (Nojit et al. Citation2014).
2.4. Salmonella enteritidis propagation
Salmonella enteritidis was kindly provided by Dr Prapas Patchanee, Faculty of Veterinary Medicine, Chiang Mai University. Salmonella enteritidis was freshly prepared by the colony picking method and propagation in Luria-Bertani Broth (LB broth, Caisson Laboratories, North Logan, UT, USA) at 37°C, 120 rpm in shaking incubator until the log phase was reached. The growth curve regression equation: y = (OD595) × (5.09 × 109) + (–8.3 × 108) was used to calculate bacterial concentration (CFU/ml). The bacterial number was adjusted to approximately 108 CFU/ml prior to use in the experiment (Chuammitri et al. Citation2011). The Salmonella isolates were identified by WHO National Salmonella and Shigella Center (NSSC) at the Department of Medical Sciences, Ministry of Public Health, Thailand (Boonkhot et al. Citation2015).
2.5. Heterophil stimulation
Heterophils (1 × 106 cells) were aliquoted into a 24-well cell culture plate (duplicate wells). Subsequently, heterophils were co-cultured with 10 multiplicity of infection of S. enteritidis (1 × 107 bacteria) in serum-free RPMI-1640 medium. A total of 50 μM quercetin or Phosphate-buffered saline (PBS) were supplemented and incubated at 41°C with 5% CO2 for 60 min. After stimulation, cells from two wells were combined, washed, and spun. Heterophil cell pellets were resuspended in RNAlater as per the manufacturer's instructions in order to preserve RNA for further analysis.
2.6. RNA extraction and complementary DNA synthesis
Heterophils in RNAlater were centrifuged at 11,000 rpm for 1 min and the liquid portion was discarded. Total RNA was extracted and purified by NucleoSpin RNA (Macherey-Nagel, Bethlehem, PA, USA) according to the manufacturer's recommendations. RNA concentration was quantified using a DU 730 nanoVette UV/VIS spectrophotometer (Beckman Coulter). Complementary DNA (cDNA) was reverse-transcribed from 2 μg of total RNA by Tetro cDNA Synthesis Kit (Bioline, Taunton, MA, USA). Briefly, 20 μl reaction mixture contained 4 μl 5× RT Buffer, 1 μl 10 mM dNTP mix (2.5 mM each), 1 μl Oligo(dT)18 (10 μM/μl), 1 μL Random Hexamer (10 μM/μl), 1 μl RNase Inhibitor (10 U/μl), 1 μl Tetro Reverse Transcriptase (200 U/μl), 2 μg Total RNA, and DEPC-treated H2O. The cDNA synthesis was carried out by LongGene A200 (LongGene Scientific Instruments, Hangzhou, China) by incubating the samples at 25°C for 10 min, 45°C for 30 min, followed by heating at 85°C for 5 min, chilled on ice, and stored reaction at −20°C until further use.
2.7. Primers
Primers for genes, IL6, IL8L1(K60; IL8), IL8L2 (CXCL8; EMF-1; CXCLi2), IL12B (IL-12p40), IL17A (ChIL-17), IL18, CCL4 (K203, MIP-1β), NOS2 (iNOS), LITAF (TNF-α), MIF, TGFB1 (formerly known as TGFB4; Halper et al. Citation2004) and reference gene (GAPDH) (Hong et al. Citation2006) were designed using Primer3 Plus (Untergasser et al. Citation2007). The primer sequence of chicken IL1B (IL-1β) was described previously (Higgs et al. Citation2006). The real-time PCR primers were synthesized by Macrogen (Seoul, Korea). The sequences of oligonucleotide primers used in this study were indicated in .
Table 1. Sequence of the primers used in real-time quantitative RT-PCR.
2.8. Quantitative real-time RT-PCR
Quantitative real-time RT-PCR (qRT-PCR) was performed to investigate the effect of quercetin on pro-inflammatory cytokines, chemokines and other related gene expressions in chicken heterophils. The quantification of cytokine or chemokine mRNAs was performed in triplicate on ABI 7300 Real-Time PCR System equipped with SDS Software v1.4 (Life Technologies). qRT-PCR was performed using SensiFAST SYBR Hi-ROX Kit (Bioline) as per the manufacturer's instruction. The reactions were run for 40 cycles in every tested gene. Next, dissociation curve analysis (Tm) (Figure S2) and 2% agarose gel electrophoresis were used to check real-time PCR product specificity (Chuammitri et al. Citation2017). Analysis of relative gene expression (RQ) was calculated from the Ct of the target gene and GAPDH. The expression levels (fold difference) were reported using 2−ΔΔC t method (Livak and Schmittgen Citation2001).
To generate standard curves for cytokine, chemokine, and GAPDH-specific reactions, qRT-PCR products were purified by NucleoSpin Gel and PCR clean-up kit (Machery-Nagel). One hundred nanograms of purified qRT-PCR DNA were serially diluted (10−1 to 10−6) in sterile RNase-free water. Regression analysis of the mean values of qRT-PCRs for the log10 dilution was used to create standard curves (Liu et al. Citation2010) (Figure S1).
2.9. Data analysis
The collected data were subjected to screening for potential outliers by robust regression and outlier removal (ROUT) methods (Motulsky and Brown Citation2006). The normality test, D’Agostino–Pearson omnibus test, was applied to raw data preceding various statistical methods: unpaired two-tailed Student t test or Mann–Whitney test. Statistical significance was achieved when p < .05. Data presentations are displayed as mean with standard error (mean ± SE). Graphical representations from analysing data are built using GraphPad Prism (GraphPad software, San Diego, CA, USA). Heat maps of the average gene expression levels were generated by Genesis 1.7.6 using an average linkage method with Euclidean distance to present gene expression patterns (Sturn et al. Citation2002). Selected genes from differential expression data were further created for a network of genes involved in inflammatory processes by CytoScape 3.0 with GeneMANIA plugin (Montojo et al. Citation2010).
3. Results
3.1. Standard curves of pro-inflammatory cytokines, chemokines, and other mRNAs in real-time PCR reactions
In order to access the efficiency in real-time PCR experiments, we have generated a standard curve of log10 serially diluted DNA for the different reactions. The linear regression between the Ct values and the amount of input DNA fitted with a straight line (Figure S1). The R2 values of IL1B, IL6, IL8L1, IL8L2, IL12B, IL17A, IL18, CCL4, LITAF, MIF, NOS2, TGFB1, and GAPDH were determined. All R2 values exceeded 0.99, and the equations of respective regression line of each gene were also given.
3.2. Effects of quercetin on the expressions of pro-inflammatory cytokines, chemokines, and other mRNAs in hybrid broiler chickens
Heterophils from hybrid chickens treated with quercetin had higher relative expression levels of IL6, IL8L1, IL8L2, IL18, CCL4, LITAF, and MIF (), but only two tested genes, IL8L1 and CCL4, had significantly higher relative expression levels when compared to non-stimulated heterophils. Of the 12 chosen genes for assessment in quercetin treatment cells, IL1B, NOS2, IL12B, and TGFB1 had lower relative expression levels compared to non-stimulated cells. IL1B, IL12B, and TGFB1 genes had statistical differences of expressions ().
Figure 1. Real-time RT-PCR analyses of pro-inflammatory, inflammatory, and anti-inflammatory genes of hybrid commercial chickens under quercetin supplementation or PBS. Bar graphs show relative mRNA expressions of study genes. The data are represented as mean ± SE (n = 4–7 pools), *p < .05, **p < .01, ***p < .001 by unpaired Student t test.
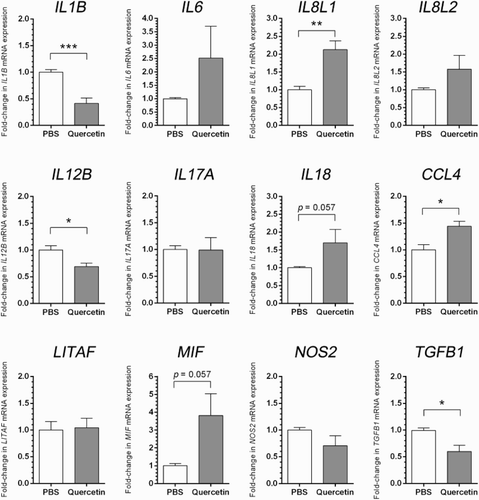
3.3. Gene expression patterns in indigenous Thai breed (Pradu Hang Dam) chickens under the effects of quercetin
In heterophils from Pradu chickens, 7 of 12 pro-inflammatory genes were down-regulated when supplemented with quercetin (). On the other hand, only 3 of 12 tested genes were up-regulated and 2 genes were slightly changed. The expressions of cytokine genes, IL1B, IL6, IL8L1, IL8L2, IL12B, IL18, and chemokines, CCL4 and MIF, were down-regulated. Specifically, only IL1B, IL8L1, IL8L2, IL12B, CCL4, and MIF genes in quercetin supplementation were significantly different from non-stimulated control. NOS2, IL17A, and TGFB1 were up-regulated. No change in expression level has been observed in IL6 and LITAF genes from heterophils of Pradu chickens supplemented with quercetin or cells without stimulation.
Figure 2. Gene expressions in heterophils of Thai indigenous chicken breed (Pradu Hang Dam) under the effects of quercetin or PBS by real-time RT-PCR analyses. Bar graphs show relative mRNA expressions of study genes. The data are represented as mean ± SE (n = 8 pools), *p < .05, **p < .01, ***p < .001, ****p < .0001 by unpaired Student t test.
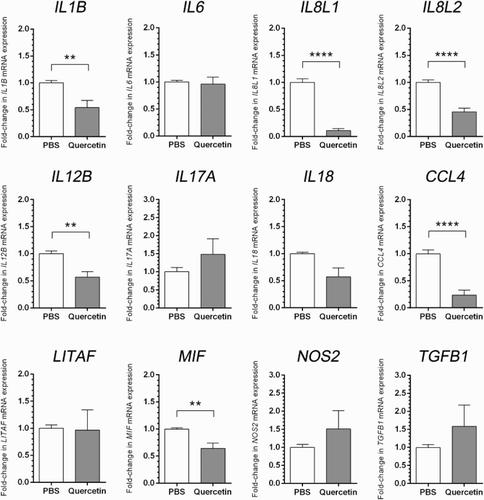
3.4. Comparison of gene expression patterns in commercial breeds and indigenous Thai breed chickens under the effects of quercetin
From gene expression data explored from different chicken origins, we have noticed that some genes shared the authenticated features in induction of pro-inflammation or involved in some parts of inflammatory processes conducted by heterophils under quercetin supplementation. Venn diagrams extracted from the results in gene expression patterns depicted the overlapping and underlying genes that could be affected by quercetin. In , some genes, IL6, IL17A, and LITAF, participated in the pro-inflammatory process induced by the presence of Lipopolysaccharides (LPS). On the other hand, pro-inflammatory cytokines, IL1B and IL12B, were profoundly decreased in both chicken origins. This finding may be due to the action of quercetin. The rest of studied genes appeared to be changed in the opposite directions ().
Figure 3. Venn diagram summarizes similarities and differences among up-regulated or down-regulated of gene expressions in heterophils supplemented with quercetin. As depicted, hybrid chickens are very different from Pradu chickens.
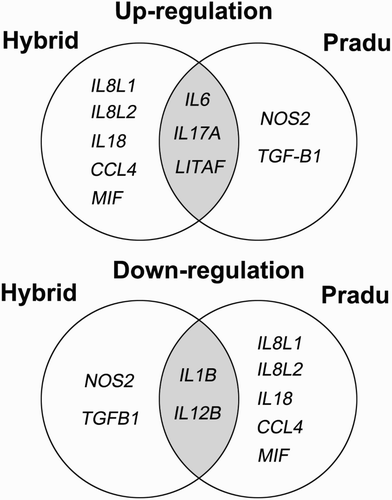
Gene expression patterns of quercetin-stimulated heterophils from two distinct broiler chicken origins are shown in . The heat map generated from individual data points of heterophils in hybrid chickens and Pradu chickens was connected by the similar patterns of up- or down-regulated genes. In this study, quercetin could facilitate in up-regulation of putative anti-inflammatory gene, TGFB1, in Pradu chicken; however, this phenomenon was conversely found in commercial hybrid chickens ().
Figure 4. The heat map depicts a mean expression level of each studied gene in chicken heterophils from hybrid broilers (A) and from Pradu chickens (B) with either PBS or quercetin treatment. The expression values ranged from −3 to +3. Red indicates up-regulation, green indicates down-regulation, and black indicates no change in gene expression. The expression levels are according to the colour scale of the log2 values.
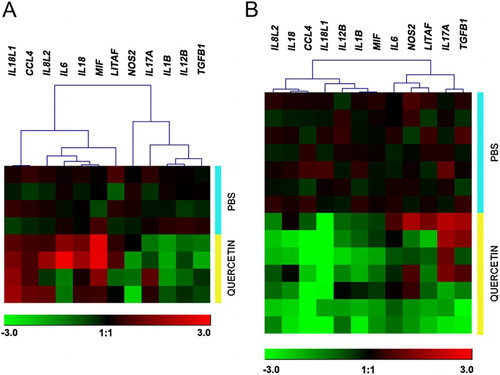
We further analysed the gene network that may be engaged in controlling pro-inflammatory cytokines and chemokines using CytoScape. Regardless of up- or down-regulations of the studied genes, we have input the gene annotation terms using publicly available human genes to find co-expression, co-localization, pathway, or physical interactions with the genes of interest in our studies. The gene network generated from CytoScape also discovered the relationships of the studied genes with other genes that can be correlated, for example response to molecule of bacterial origin, response to LPS, response to bacterium, positive regulation of cytokine production, leukocyte-mediated immunity, and inflammatory response ().
4. Discussion
To date, there are no intensive studies of the effects of quercetin on immune cells in avian species. The searchable literature emphasized the efficacy of these plant compounds only in promoting meat production and growth performance of chickens (Goliomytis et al. Citation2014). The present study provides novel information regarding the anti-inflammatory effects of quercetin in chicken heterophil gene expressions from two genetically different chickens: commercial broiler and TIC. We previously reported the effects of quercetin hydrate on heterophil functions from broilers on ROS production, phagocytosis, and heterophil extracellular traps of the gram-negative bacteria S. enteritidis (Nojit et al. Citation2014). To the best of our knowledge, this is the first report to evaluate the anti-inflammatory effects of quercetin on chicken innate immune cells with respect to its influence on gene expression. According to Dorji et al. (Citation2011), the similarity in genetics and genetic distance of two meat-type chickens (broiler and Pradu) has been addressed. Pradu chickens were already genetically determined in their meat production traits, and the authors suggested that these two chicken lineages were closely related than other Thai native chickens.
Bacterial invasion often triggers the innate immunity by releasing pro-inflammatory cytokines, antimicrobial peptides, or enzymes from innate immune cells. Pro-inflammatory cytokines and chemokines have a crucial role in initiating an innate immune response and assist in generating a local inflammatory response (Hughes et al. Citation2007). Pro-inflammatory cytokines, such as IL-1β, IL-6, and IL-8, were involved in S. enteritidis infection (Cheeseman et al. Citation2007; Abasht et al. Citation2008). The effects of quercetin on S. enteritidis-stimulated heterophils in this study revealed clear instances that many inflammatory cytokine/chemokine genes could be successfully suppressed only in native chickens. In comparison, the commercial chicken breeds showed no decline in inflammation, as depicted by the up-regulation of genes after quercetin supplementation.
As reported by Malek et al. (Citation2004) regarding Fayoumi, native chicken of Egypt, many candidate genes were possibly controlling response to S. enteritidis, e.g. TLR4, CD28, and MD-2. Moreover, heterophils from this native chicken respond more robustly to in vitro S. enteritidis infection by expressing IL-6, GM-CSF, and anti-inflammatory cytokines (IL-10 and TGF-β4) (Cheeseman et al. Citation2008). In this study, quercetin also exerted anti-inflammatory action by up-regulation of TGF-β1 in Pradu, which was consistent with the results from the aforementioned work. By analogy with the broilers with a long history of genetic selection to increase growth or reproduction, native chickens may have been favourable for the reduction or suppression of inflammatory responses. The importance of genetic background in chicken aids in a strong anti-inflammatory nature, but the supplementation of known immunomodulatory substances such as quercetin or other derivatives could be of great interest in mounting an immune response in poultry.
The increase in pro-inflammatory gene expression (i.e. IL-6 and IL-8) has also been suggested to be associated with the resistance to extraintestinal S. enteritidis (Kogut et al. Citation2003). From our observations, the expressions of IL6, IL17A, and LITAF in Pradu's heterophils stayed above the basal levels even after exposing to quercetin. This finding may imply the process in which cells readily prepare for S. enteritidis response by generating a handful of inflammatory mediators. Indeed, quercetin was actively involved in LPS-stimulated inflammation at the early phase of infection. Even though the suppressive properties of quercetin on inflammation are actually exerted, higher level of presenting inflammatory mRNAs could eventually overcome the in vitro actions of quercetin. Another study on two Malaysian indigenous chicken breeds extrapolated that iNOS genes may contribute to the resistance to S. enteritidis (Tohidi et al. Citation2012). That finding is also in accordance with our data in term of the increase in NOS2 gene expression in Pradu chickens receiving quercetin, but not in hybrid commercial chickens. Quercetin up-regulated NOS2 gene in Pradu heterophils because the product of this gene, nitric oxide, helped generate an environment that is extremely hostile for the bacterium (S. enteritidis).
Many reports clearly demonstrated and have agreed that quercetin interferes with signalling proteins, such as p38, Akt, IκBα, IκBβ, NF-κB, and AP-1, which are mainly involved in the process of signal transduction and gene transcriptions after LPS stimulation in mammals (Chang et al. Citation2013; Endale et al. Citation2013; Yang et al. Citation2014). The bacterial component, LPS, in the current study was a major player in initiating inflammatory signals in this particular assay setting. Nevertheless, quercetin could possibly lessen pro-inflammatory gene expressions via inhibition of key transcription factors (Boesch-Saadatmandi et al. Citation2011), but the actual signalling pathway in chicken heterophils remained unanswered.
As we were anticipating, quercetin attenuates more than half of studying pro-inflammatory, inflammatory cytokines, and chemokine genes in our assay settings. We asked whether those down-regulated or up-regulated genes from our results are connected to genes within the pathways of inflammation. One way for an in-depth exploration of information is to in silico predict a group of genes already intensively studied by others (Kaiser et al. Citation2005; Szmolka et al. Citation2015). The results of gene network analysis reveal many annotated genes that envisage the immune response manipulated by quercetin. In conclusion, our data emphasize the modulatory effects of quercetin in controlling pro-inflammatory cytokines, anti-inflammatory cytokine, chemokines, and other genes involved in inflammatory processes. The importance of these findings needs further investigation in terms of exploiting in in vivo study.
5. Conclusions
The results of this study emphasized that quercetin could potentially down-regulate pro-inflammatory genes such as IL1B along with anti-inflammatory TGFB1. Quercetin had also exerted its effects on promoting the expression of pro-inflammatory cytokines such as IL6 and other chemokines such as IL8L1, IL8L2, CCL4, IL18, MIF, but only exerted a slight increase in IL17A and LITAF in chicken heterophils.
TAAR_1405814_Supplementary_Figures.zip
Download Zip (538.9 KB)Disclosure statement
No potential conflict of interest was reported by the authors.
Additional information
Funding
References
- Abasht B, Kaiser M, Lamont S. 2008. Toll-like receptor gene expression in cecum and spleen of advanced intercross line chicks infected with Salmonella enterica serovar Enteritidis. Vet Immunol Immunopathol. 123:314–323. doi: 10.1016/j.vetimm.2008.02.010
- Akaboot P, Duangjinda M, Phasuk Y, Kaenechan C, Chinchiyanond W. 2012. Genetic characterization of Red Junglefowl (Gallus gallus), Thai indigenous chicken (Gallus domesticus), and two commercial lines using selective functional genes compared to microsatellite markers. Genet Mol Res. 11:1881–1890. doi: 10.4238/2012.July.19.7
- Alzoreky N, Nakahara K. 2003. Antibacterial activity of extracts from some edible plants commonly consumed in Asia. Int J Food Microbiol. 80:223–230. doi: 10.1016/S0168-1605(02)00169-1
- Askari G, Ghiasvand R, Feizi A, Ghanadian SM, Karimian J. 2012. The effect of quercetin supplementation on selected markers of inflammation and oxidative stress. J Res Med Sci. 17:637–641.
- Boesch-Saadatmandi C, Loboda A, Wagner AE, Stachurska A, Jozkowicz A, Dulak J, Döring F, Wolffram S, Rimbach G. 2011. Effect of quercetin and its metabolites isorhamnetin and quercetin-3-glucuronide on inflammatory gene expression: role of miR-155. J Nutr Biochem. 22:293–299. doi: 10.1016/j.jnutbio.2010.02.008
- Boonkhot P, Tadee P, Patchanee P. 2015. Serodiversity and antimicrobial resistance profiles of detected salmonella on swine production chain in Chiang Mai and Lamphun, Thailand. Acta Sci Vet. 43:1263.
- Chan S-T, Chuang C-H, Yeh C-L, Liao J-W, Liu K-L, Tseng M-J, Yeh S-L. 2012. Quercetin supplementation suppresses the secretion of pro-inflammatory cytokines in the lungs of Mongolian gerbils and in A549 cells exposed to benzo [a] pyrene alone or in combination with β-carotene: in vivo and ex vivo studies. J Nutr Biochem. 23:179–185. doi: 10.1016/j.jnutbio.2010.11.014
- Chang Y-C, Tsai M-H, Sheu WH-H, Hsieh S-C, Chiang A-N. 2013. The therapeutic potential and mechanisms of action of quercetin in relation to lipopolysaccharide-induced sepsis in vitro and in vivo. PLoS One. 8:e80744. doi: 10.1371/journal.pone.0080744
- Cheeseman JH, Kaiser MG, Ciraci C, Kaiser P, Lamont SJ. 2007. Breed effect on early cytokine mRNA expression in spleen and cecum of chickens with and without Salmonella enteritidis infection. Dev Comp Immunol. 31:52–60. doi: 10.1016/j.dci.2006.04.001
- Cheeseman JH, Lillehoj HS, Lamont SJ. 2008. Reduced nitric oxide production and iNOS mRNA expression in IFN-γ-stimulated chicken macrophages transfected with iNOS siRNAs. Vet Immunol Immunopathol. 125:375–380. doi: 10.1016/j.vetimm.2008.05.015
- Chiang H-I, Swaggerty CL, Kogut MH, Dowd SE, Li X, Pevzner IY, Zhou H. 2008. Gene expression profiling in chicken heterophils with Salmonella enteritidis stimulation using a chicken 44K Agilent microarray. BMC Genomics. 9:526. doi: 10.1186/1471-2164-9-526
- Cho S-Y, Park S-J, Kwon M-J, Jeong T-S, Bok S-H, Choi W-Y, Jeong W-I, Ryu S-Y, Do S-H, Lee C-S. 2003. Quercetin suppresses proinflammatory cytokines production through MAP kinases and NF-κB pathway in lipopolysaccharide-stimulated macrophage. Mol Cell Biochem. 243:153–160. doi: 10.1023/A:1021624520740
- Chuammitri P, Amphaiphan C, Nojit P. 2015. In vitro modulatory effects of quercetin on bovine neutrophil effector functions. Thai J Vet Med. 45:63–72.
- Chuammitri P, Redmond SB, Kimura K, Andreasen CB, Lamont SJ, Palić D. 2011. Heterophil functional responses to dietary immunomodulators vary in genetically distinct chicken lines. Vet Immunol Immunopathol. 142:219–227. doi: 10.1016/j.vetimm.2011.05.019
- Chuammitri P, Srikok S, Saipinta D, Boonyayatra S. 2017. The effects of quercetin on microRNA and inflammatory gene expression in lipopolysaccharide-stimulated bovine neutrophils. Vet World. 10:403–410. doi: 10.14202/vetworld.2017.403-410
- Crhanova M, Hradecka H, Faldynova M, Matulova M, Havlickova H, Sisak F, Rychlik I. 2011. Immune response of chicken gut to natural colonization by gut microflora and to Salmonella enterica serovar enteritidis infection. Infect Immun. 79:2755–2763. doi: 10.1128/IAI.01375-10
- Das T, Mukherjee S, Chaudhuri K. 2012. Effect of quercetin on Vibrio cholerae induced nuclear factor-κB activation and interleukin-8 expression in intestinal epithelial cells. Microbes Infect. 14:690–695. doi: 10.1016/j.micinf.2012.02.007
- Dorji N, Daungjinda M, Phasuk Y. 2011. Genetic characterization of Thai indigenous chickens compared with commercial lines. Trop Anim Health Prod. 43:779–785. doi: 10.1007/s11250-010-9763-3
- Endale M, Park S-C, Kim S, Kim S-H, Yang Y, Cho JY, Rhee MH. 2013. Quercetin disrupts tyrosine-phosphorylated phosphatidylinositol 3-kinase and myeloid differentiation factor-88 association, and inhibits MAPK/AP-1 and IKK/NF-κB-induced inflammatory mediators production in RAW 264.7 cells. Immunobiology. 218:1452–1467. doi: 10.1016/j.imbio.2013.04.019
- Formica JV, Regelson W. 1995. Review of the biology of quercetin and related bioflavonoids. Food Chem Toxicol. 33:1061–1080. doi: 10.1016/0278-6915(95)00077-1
- Freitas M, Ribeiro D, Tomé SM, Silva AM, Fernandes E. 2014. Synthesis of chlorinated flavonoids with anti-inflammatory and pro-apoptotic activities in human neutrophils. Eur J Med Chem. 86:153–164. doi: 10.1016/j.ejmech.2014.08.035
- Geraets L, Haegens A, Brauers K, Haydock JA, Vernooy JH, Wouters EF, Bast A, Hageman GJ. 2009. Inhibition of LPS-induced pulmonary inflammation by specific flavonoids. Biochem Biophys Res Commun. 382:598–603. doi: 10.1016/j.bbrc.2009.03.071
- Goliomytis M, Tsoureki D, Simitzis P, Charismiadou M, Hager-Theodorides A, Deligeorgis S. 2014. The effects of quercetin dietary supplementation on broiler growth performance, meat quality, and oxidative stability. Poult Sci. 93:1957–1962. doi: 10.3382/ps.2013-03585
- Halper J, Burt DW, Romanov MN. On reassessment of the chicken TGFB4 gene as TGFB1. Growth Factors. 22:121–122. doi: 10.1080/08977190410001712878
- Higgs R, Cormican P, Cahalane S, Allan B, Lloyd AT, Meade K, James T, Lynn DJ, Babiuk LA, O’Farrelly C. 2006. Induction of a novel chicken Toll-like receptor following Salmonella enterica serovar Typhimurium infection. Infect Immun. 74:1692–1698. doi: 10.1128/IAI.74.3.1692-1698.2006
- Hong YH, Lillehoj HS, Lee SH, Dalloul RA, Lillehoj EP. 2006. Analysis of chicken cytokine and chemokine gene expression following Eimeria acervulina and Eimeria tenella infections. Vet Immunol Immunopathol. 114:209–223. doi: 10.1016/j.vetimm.2006.07.007
- Huang R, Zhong T, Wu H. 2015. Quercetin protects against lipopolysaccharide-induced acute lung injury in rats through suppression of inflammation and oxidative stress. Arch Med Sci. 11:427–432. doi: 10.5114/aoms.2015.50975
- Hughes S, Poh T-Y, Bumstead N, Kaiser P. 2007. Re-evaluation of the chicken MIP family of chemokines and their receptors suggests that CCL5 is the prototypic MIP family chemokine, and that different species have developed different repertoires of both the CC chemokines and their receptors. Dev Comp Immunol. 31:72–86. doi: 10.1016/j.dci.2006.04.003
- Kaiser P, Poh TY, Rothwell L, Avery S, Balu S, Pathania US, Hughes S, Goodchild M, Morrell S, Watson M. 2005. A genomic analysis of chicken cytokines and chemokines. J Interferon Cytokine Res. 25:467–484. doi: 10.1089/jir.2005.25.467
- Kogut MH, Rothwell L, Kaiser P. 2003. Differential regulation of cytokine gene expression by avian heterophils during receptor-mediated phagocytosis of opsonized and nonopsonized Salmonella enteritidis. J Interferon Cytokine Res. 23:319–327. doi: 10.1089/107999003766628160
- Liu J, Li X, Yue Y, Li J, He T, He Y. 2005. The inhibitory effect of quercetin on IL-6 production by LPS stimulated neutrophils. Cell Mol Immunol. 2:455–460.
- Liu H, Zhang M, Han H, Yuan J, Li Z. 2010. Comparison of the expression of cytokine genes in the bursal tissues of the chickens following challenge with infectious bursal disease viruses of varying virulence. Virol J. 7:364. doi: 10.1186/1743-422X-7-364
- Livak KJ, Schmittgen TD. 2001. Analysis of relative gene expression data using real-time quantitative PCR and the 2− ΔΔCT method. Methods. 25:402–408. doi: 10.1006/meth.2001.1262
- Malek M, Hasenstein J, Lamont S. 2004. Analysis of chicken TLR4, CD28, MIF, MD-2, and LITAF genes in a Salmonella enteritidis resource population. Poult Sci. 83:544–549. doi: 10.1093/ps/83.4.544
- Mellata M, Dho-Moulin M, Dozois CM, Curtiss III R, Lehoux B, Fairbrother JM. 2003. Role of avian pathogenic Escherichia coli virulence factors in bacterial interaction with chicken heterophils and macrophages. Infect Immun. 71:494–503. doi: 10.1128/IAI.71.1.494-503.2003
- Montojo J, Zuberi K, Rodriguez H, Kazi F, Wright G, Donaldson SL, Morris Q, Bader GD. 2010. Genemania Cytoscape plugin: fast gene function predictions on the desktop. Bioinformatics. 26:2927–2928. doi: 10.1093/bioinformatics/btq562
- Motulsky HJ, Brown RE. 2006. Detecting outliers when fitting data with nonlinear regression–a new method based on robust nonlinear regression and the false discovery rate. BMC Bioinformatics. 7:123. doi: 10.1186/1471-2105-7-123
- Nair MP, Mahajan S, Reynolds JL, Aalinkeel R, Nair H, Schwartz SA, Kandaswami C. 2006. The flavonoid quercetin inhibits proinflammatory cytokine (tumor necrosis factor alpha) gene expression in normal peripheral blood mononuclear cells via modulation of the NF-κβ system. Clin Vaccine Immunol. 13:319–328. doi: 10.1128/CVI.13.3.319-328.2006
- Nojit P, Ampaipun J, Chuammitri P. 2014. Effects of quercetin on chicken heterophil cellular functions. Chaing Mai Vet J. 12:167–178.
- Redmond SB, Chuammitri P, Andreasen CB, Palić D, Lamont SJ. 2009. Chicken heterophils from commercially selected and non-selected genetic lines express cytokines differently after in vitro exposure to Salmonella enteritidis. Vet Immunol Immunopathol. 132:129–134. doi: 10.1016/j.vetimm.2009.05.010
- Sick C, Schneider K, Staeheli P, Weining KC. 2000. Novel chicken CXC and CC chemokines. Cytokine. 12:181–186. doi: 10.1006/cyto.1999.0543
- Sturn A, Quackenbush J, Trajanoski Z. 2002. Genesis: cluster analysis of microarray data. Bioinformatics. 18:207–208. doi: 10.1093/bioinformatics/18.1.207
- Swaggerty CL, Kogut MH, Ferro PJ, Rothwell L, Pevzner IY, Kaiser P. 2004. Differential cytokine mRNA expression in heterophils isolated from Salmonella-resistant and-susceptible chickens. Immunology. 113:139–148. doi: 10.1111/j.1365-2567.2004.01939.x
- Szmolka A, Wiener Z, Matulova ME, Varmuzova K, Rychlik I. 2015. Gene expression profiles of chicken embryo fibroblasts in response to Salmonella enteritidis infection. PLoS One. 10:e0127708. doi: 10.1371/journal.pone.0127708
- Tohidi R, Idris IB, Panandam JM, Bejo MH. 2012. The effects of polymorphisms in IL-2, IFN-γ, TGF-β2, IgL, TLR-4, MD-2, and iNOS genes on resistance to Salmonella enteritidis in indigenous chickens. Avian Pathol. 41:605–612. doi: 10.1080/03079457.2012.739680
- Untergasser A, Nijveen H, Rao X, Bisseling T, Geurts R, Leunissen JA. 2007. Primer3Plus, an enhanced web interface to Primer3. Nucleic Acids Res. 35:W71–W74. doi: 10.1093/nar/gkm306
- Wadsworth TL, Koop DR. 1999. Effects of the wine polyphenolics quercetin and resveratrol on pro-inflammatory cytokine expression in RAW 264.7 macrophages. Biochem Pharmacol. 57:941–949. doi: 10.1016/S0006-2952(99)00002-7
- Withanage G, Kaiser P, Wigley P, Powers C, Mastroeni P, Brooks H, Barrow P, Smith A, Maskell D, McConnell I. 2004. Rapid expression of chemokines and proinflammatory cytokines in newly hatched chickens infected with Salmonella enterica serovar typhimurium. Infect Immun. 72:2152–2159. doi: 10.1128/IAI.72.4.2152-2159.2004
- Yang WS, Jeong D, Yi Y-S, Lee B-H, Kim TW, Htwe KM, Kim Y-D, Yoon KD, Hong S, Lee W-S. 2014. Myrsine seguinii ethanolic extract and its active component quercetin inhibit macrophage activation and peritonitis induced by LPS by targeting to Syk/Src/IRAK-1. J Ethnopharmacol. 151:1165–1174. doi: 10.1016/j.jep.2013.12.033