ABSTRACT
Soybean meal (SBM) contains several anti-nutritional factors, which limit the use of soy protein. Exogenous enzyme supplementation and microbial fermentation can reduce anti-nutritional factors and increase the bioavailability of nutrients in SBM. In this study, we investigated the optimal parameters for the solid-state fermentation (SSF) of SBM by protease in combination with probiotics and evaluated its effect on the growth performance and immune response in broilers. Protease in combination with Bacillus subtilis significantly increased the degradation of soybean protein and soybean allergens in a dose-dependent manner during the SSF of soybean meal. Broilers fed 10% fermented SBM (FSBM), which was produced with protease and B. subtilis, consumed more daily feed on average during the entire feeding period than the SBM group did (P < 0.05). Dietary supplementation with 10% FSBM attenuated IL-4 mRNA expression in the spleen and bursa of Fabricius of broilers (P < 0.05). Furthermore, 10%-FSBM-fed broilers had significantly lower serum anti-soybean IgG levels than SBM-fed broilers (P < 0.05). These results suggest that the supplementation of B. subtilis and protease during the SSF of SBM reduces anti-nutritional factors. Moreover, dietary supplementation of 10% FSBM could inhibit the allergic immune response in broilers.
Introduction
Soy bean meal (SBM) is the main source of vegetable protein in animal feed because of its low cost and high nutritional value (Cromwell Citation2012). However, SBM contains several anti-nutritional factors, such as trypsin inhibitor, lectin, phytate, and saponin (Grant Citation1989). Moreover, two major soybean antigens, namely glycinin and β-conglycinin, can induce allergic immune response in animals (Wang et al. Citation2014). These anti-nutritional factors and soybean antigens reduce the utilization and digestibility of soybean protein, which leads to impaired growth performance in broilers (Erdaw et al. Citation2017).
Previous studies have demonstrated that anti-nutritional factors in SBM can degrade through microbial fermentation (Chi and Cho Citation2016; Zhang et al. Citation2017; Su et al. Citation2018). The fermentation of SBM by probiotics efficiently eliminates anti-nutritional factors and enhances nutritional value by producing several enzymes (Mukherjee et al. Citation2016). Dietary supplementation of probiotics can improve growth performance and prevent disease in broilers (Khan and Naz Citation2013; Abudabos et al. Citation2017). Aspergillus species are widely used to produce fermented SBM (FSBM) because of their capacity to produce multiple hydrolysis enzymes (Hong et al. Citation2004). Feeding of FSBM produced by Aspergillus species significantly increases the activity of intestinal digestive enzymes and improves growth performance during the growing period of broilers (Mathivanan et al. Citation2006; Feng et al. Citation2007a, Citation2007b). In addition to fungal fermentation, solid-state fermentation (SSF) of SBM by Lactobacillus species and Clostridium butyricum may reduce the soy carbohydrate and soy protein contents of FSBM (Su et al. Citation2018). Bacillus species can produce lactic acid and remove trypsin inhibitor during fermentation. Thus, they increase protein hydrolysis and liberate free amino acids (Mukherjee et al. Citation2016). Feeding of FSBM produced by B. subtilis during the early phase can improve growth performance in broilers (Kim et al. Citation2016). Exogenous enzyme supplementation is also widely used to enhance the degradation of soybean protein and inactive anti-nutritional factors during the SSF of SBM (Ma and Wang Citation2010; Amadou et al. Citation2011). Dietary enzyme supplementation improves the nutritional status and growth performance of broilers (Abudabos Citation2012; Abudabos and Yehia Citation2013; Abd El-Hack et al. Citation2018). However, to our knowledge, no study has reported the fermentation conditions of exogenous proteases and probiotics for the SSF of SBM. Furthermore, the effect of FSBM produced by protease and probiotics on the growth performance and immune response in broilers remains unverified.
Therefore, this study investigated the optimal parameters for the mixed SSF of SBM by protease and probiotics and evaluated the effect of FSBM on broilers. The results provide valuable information regarding the fermentation conditions of SBM by enzymes and probiotics. These conditions are suitable for use on an industrial scale to mass-produce functional fermented products.
Materials and methods
Microorganisms and culture conditions
All chemical reagents were purchased from Sigma-Aldrich (St. Louis, MO, USA) unless specified otherwise. B. subtilis (ATCC 6051) and A. oryzae (ATCC 11493) were purchased from the Food Industry Research and Development Institute (Hsinchu, Taiwan). After thawing, B. subtilis was inoculated into an Erlenmeyer flask containing brain heart infusion broth at 30°C for 18 h with shaking. A. oryzae was inoculated into an Erlenmeyer flask containing potato dextrose agar and incubated at 25°C for 5 d with shaking.
SSF
SBM was ground to fine powder and mixed with water to the required relative moisture content in a space bag and autoclaved at 121°C for 30 min. The cooled substrates were mixed with different activities of protease (measured in casein digestion units, CDU). To study the effects of the initial moisture content and protease activity, fermentations were performed with different initial moisture contents (40%, 50%, and 100%, w/w) and activities of protease (104, 105, and 106 CDU/kg) in a chamber at 25°C. To study the effects of the initial moisture content, protease activity, and B. subtilis, fermentations were performed with different initial moisture contents (40%, 50%, and 100%, w/w), different activities of protease (104, 105, and 106 CDU/kg), and 10% inoculums (v/w) of B. subtilis in a chamber at 30°C. To study the effects of the initial moisture content, protease activity, and A. oryzae, fermentations were performed with different initial moisture contents (40%, 50%, and 100%, w/w), different activities of protease (104, 105, and 106 CDU/kg), and 10% inoculums (v/w) of A. oryzae in a chamber at 25°C. Different fermentation durations (6–24 h) were employed to study their effects on the SSF of SBM. All the experiments were performed three times. After fermentation, the FSBM samples were dried at 50°C for 24 h and homogenized through mechanical agitation. The fermented powder was then stored at 4°C prior to analysis.
Sodium dodecyl sulfate–polyacrylamide gel electrophoresis and western blot
The crude protein extracts from FSBM were separated through sodium dodecyl sulfate-polyacrylamide gel electrophoresis. After electrophoresis, the gel was stained with Coomassie Brilliant Blue R-250 to visualize the total protein content. Protein lysate was separated through sodium dodecyl sulfate-polyacrylamide gel electrophoresis and then transblotted onto a polyvinylidine fluoride membrane (Perkin Elmer, Norwalk, CT, USA). The β-conglycinin primary antibody was purchased from Abmart (Berkeley Heights, NJ, USA). The secondary antibody coupled to horseradish peroxidase was used in the diaminobenzidine staining procedure. The Western blotting procedure was performed according to the manufacturer's instruction.
Fast protein liquid chromatography (FPLC)
The FSBM was dissolved in distilled water and centrifuged for 10 min at 6000 × g. The supernatant was then tested for soy protein degradation. Chromatographic analyses were performed using the ÄKTAprime plus system (GE Healthcare, Marlborough, MA, USA). Samples were separated on a TOYOPEARL HW-50F column (Sigma-Aldrich, St. Louis, MO, USA) calibrated with molecular mass standards (GE Healthcare, Marlborough, MA, USA). The flow rate was 500 µL/min, and 50 mM phosphate buffer was used as a mobile-phase solution. The soy protein content was determined at a wavelength of 280 nm. The recorder was set to 60 min.
Animal study
Fifty-four female Ross 308 broilers (1 d old) from a local commercial hatchery were weighed and randomly allotted to one of three treatments, with three replicate cages of six birds per cage. Broilers were reared in stainless-steel and temperature-controlled cages (190 cm × 50 cm × 35 cm) for 3 weeks. Basal diets were formulated based on the National Research Council recommendations, as listed in . SBM used in the present study is high-protein SBM (47% CP). Thus, no additional lysine was added in the basal diet. The three groups were categorized according to their feeding regimens: (1) basal diet (CTRL), (2) 5% FSBM produced by protease and B. subtilis, or (3) 10% FSBM produced by protease and B. subtilis. Feed and water were offered ad libitum. The temperature was set at 32°C on the first day and gradually reduced to 24°C by the end of the experiment. The lighting schedule included 22 h of light and 2 h of dark throughout the experiment. The individual body weight, average daily feed intake, average daily gain, and feed conversion ratio (FCR) were recorded every week. Two chicks per replicate cage were sacrificed through cervical dislocation at 21 d of age, and the blood, spleen, and bursa of Fabricius were collected for analysis. Plasma samples (5 mL) were collected through cardiac puncture, centrifuged at 3000 × g for 10 min, and then stored at −20°C. The spleen and bursa of Fabricius of the birds were excised and subsequently frozen at −20°C for further analysis.
Table 1. Nutrient composition of the basal diet.
Serum immunoglobulin G and immunoglobulin A analysis
Blood samples were collected in nonheparinized tubes through cardiac puncture from two random chicks per replicate cage at 21 days old. Blood samples were centrifuged for 15 min at 3000 × g at 4°C. The serum was then stored at −4°C until the immunoglobulin G (IgG) and immunoglobulin A (IgA) concentrations were determined. The serum immunoglobulin levels were measured using an enzyme-linked immunosorbent assay kit (Bethyl Laboratories, Inc., Montgomery, TX, USA).
Quantitative reverse-transcription polymerase chain reaction
Six birds from each group were randomly selected, sacrificed, and examined for gene expression. Total RNA was isolated from the spleen and bursa of Fabricius and homogenized in TRIzol Reagent (Invitrogen, Carlsbad, CA, USA) using a homogenizer (SpeedMill PLUS, Analytik Jena, Jena, Germany). Total RNA was then purified and reverse transcribed by a Transcriptor Reverse Transcriptase kit (Roche Applied Science, Indianapolis, IN, USA). Quantitative reverse-transcription polymerase chain reaction (PCR) was performed using a MiniOpticon real-time PCR detection system (Bio-Rad, Hercules, CA, USA) and KAPA SYBR FAST qPCR Kit (Kapa Biosystems, Boston, MA, USA). PCR was performed using 40 cycles at 95°C for 30 s, 58–60°C for 60 s, and 72°C for 30 s, and β-actin mRNA was determined as the internal control gene. The sequence of primers for quantitative reverse-transcription PCR is listed in . The mRNA expression of each gene was normalized to the β-actin mRNA expression in the same sample. The threshold cycle (C t) values were obtained, and the relative gene expression was calculated using the formula (1/2) C t target genes − Ct β-actin.
Table 2. Primer sequences for quantitative reverse-transcription PCR.
Ethics statement
All experiments were performed in accordance with the approved guidelines. The animal protocol was approved by the National Ilan University Institutional Animal Care and Use Committee.
Statistical analysis
Replicate means are the experimental units in the statistical analysis. The data were analyzed in a completely randomized design using the general linear model (GLM) procedure of the SAS software (SAS Institute, Cary, NC, USA). Means were compared using Tukey test when the probability values were significant (p < 0.05).
Results
Protease in combination with B. subtilis promotes soy protein degradation
The protease activity plays a critical role in soy protein degradation. We first investigated the effects of the initial moisture content, incubation duration, and protease activity on soy protein degradation. The degradation efficiency of 75-kDa soy protein was linearly correlated with increased protease activity (A). The content of 20-kDa soy protein was associated with increasing protease activity (A). Furthermore, 104 CDU/kg protease in combination with A. oryzae treatment promoted the degradation of 75-kDa soy protein at 100% initial moisture during 12- and 24-h fermentation periods (B). As expected, increased protease activity (105 and 106 CDU/kg) enhanced the degradation of 75-kDa soy protein during the SSF of SBM by A. oryzae (B). Moreover, 106 CDU/kg protease in combination with A. oryzae treatment further degraded 50-kDa soy protein at 100% initial moisture during a 24-h fermentation period (B). Similar to A. oryzae, B. subtilis treatment combined with 104 CDU/kg protease promoted the degradation of 75-kDa soy protein at 100% initial moisture during a 24-h fermentation period (C). In contrast to the A. oryzae treatment, B. subtilis treatment with 105 CDU/kg protease completely degraded the 75-kDa soy protein (B and 1C). Similarly, 106 CDU/kg protease in combination with B. subtilis treatment further degraded the 50-kDa soy protein at 100% initial moisture during a 24-h fermentation period (C).
Figure 1. Effect of protease and probiotics on the soy protein content of FSBM. (A) Effects of different protease activity (104, 105, and 106 CDU/kg), initial moisture (40%, 50%, and 100%), and fermentation durations (6, 12, and 24 h) on the soy protein content of FSBM. (B) Effects of different protease activity (104, 105, and 106 CDU/kg), initial moisture (40%, 50%, and 100%), and fermentation durations (6, 12, and 24 h) on the soy protein content of FSBM produced by A. oryzae. (C) Effects of different protease activity (104, 105, and 106 CDU/kg), initial moisture (40%, 50%, and 100%), and fermentation durations (6, 12, and 24 h) on the soy protein content of FSBM produced by B. subtilis. M represents the standard protein marker. Three experiments were conducted, and one representative result is displayed.
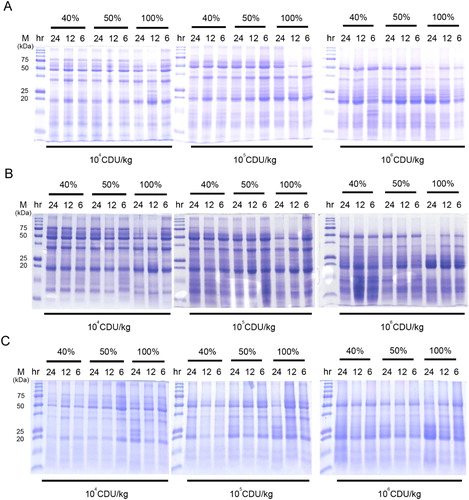
We then tested whether protease and probiotics could further reduce the β-conglycinin content of SBM at different initial moisture contents and incubation durations. The content of the α subunit of β-conglycinin (75 kDa) was significantly reduced in the presence of increased protease activity (106 CDU/kg; A). In combination with A. oryzae treatment, 104 and 105 CDU/kg protease promoted the degradation of the α subunit of β-conglycinin at 100% initial moisture during 12- and 24-h fermentation periods (B), and 106 CDU/kg protease completely degraded the α subunit of β-conglycinin under all fermentation conditions (B). Similar results were also observed for protease in combination with B. subtilis treatment (C).
Figure 2. Effects of protease and probiotics on the β-conglycinin content of FSBM. (A) Effects of different protease activity (104, 105, and 106 CDU/kg), initial moisture (40%, 50%, and 100%), and fermentation durations (6, 12, and 24 h) on the β-conglycinin contents of FSBM. (B) Effect of different protease activity (104, 105, and 106 CDU/kg), initial moisture (40%, 50%, and 100%), and fermentation durations (6, 12, and 24 h) on the β-conglycinin content of FSBM produced by A. oryzae. (C) Effects of different protease activity (104, 105, and 106 CDU/kg), initial moisture (40%, 50%, and 100%), and fermentation durations (6, 12, and 24 h) on the β-conglycinin content of FSBM produced by B. subtilis. M represents the standard protein marker. Three experiments were conducted, and one representative result is displayed.
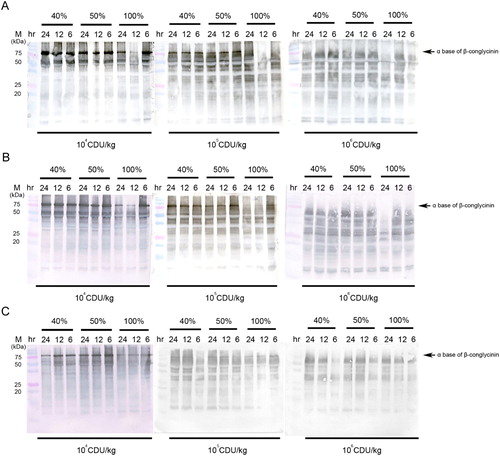
We further examined the differences in the degradation efficiency of the α and β subunits of β-conglycinin of A. oryzae and B. subtilis in the presence of 104 CDU/kg protease at 100% initial moisture during a 24-h fermentation period. Both protease alone and protease in combination with A. oryzae treatment sufficiently degraded the α subunit of β-conglycinin compared with untreated SBM (A). In addition to the degradation of the α subunit of β-conglycinin, protease in combination with B. subtilis treatment further degraded the β base of the β-conglycinin subunits (A). Moreover, FPLC demonstrated that large-molecular-weight soy protein was reduced in the presence of protease and probiotics compared with untreated SBM (A and 4B). The levels of low-molecular-weight (<6.5 kDa) soy protein were significantly higher when protease was used in combination with B. subtilis rather than A. oryzae (B, black arrow). These findings indicate that optimal SSF for soy protein degradation utilizes protease in combination with B. subtilis treatment at 100% initial moisture for 24 h.
Figure 3. Effects of protease and probiotics on the subunits of β-conglycinin content of FSBM. The SBM was fermented with or without 105 CDU/kg protease and probiotics (A. oryzae or B. subtilis) at an initial moisture content of 40% for 24 h. M represents the standard protein marker. Three experiments were conducted, and one representative result is displayed. The intensity of the β-conglycinin (α and β subunits) band was analyzed relative to the intensity of the untreated (SBM) group by using densitometer software. The numbers are the means of three independent experiments.
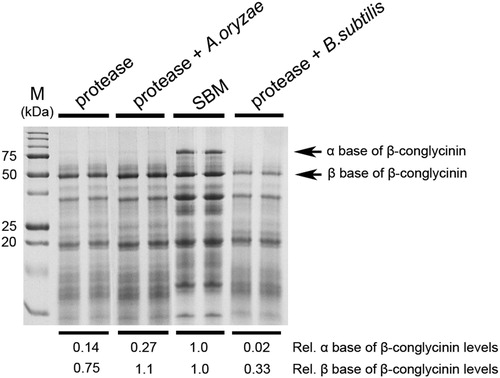
Figure 4. FPLC patterns of soy protein degradation by protease and probiotics. The SBM was fermented with 105 CDU/kg protease and probiotics (A. oryzae or B. subtilis) at an initial moisture content of 40% for 24 h. (A) FPLC measurement of standard patterns of soy protein. (B) FPLC measurement of soy protein degradation in FSBM produced by protease and probiotics. The two black lines is used as an indicator of standard proteins (66 and 6.5 kDa) based on its retention time. Three experiments were conducted, and one representative result is displayed.
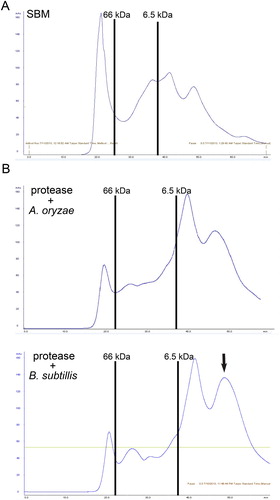
Effect of FSBM produced by B. subtilis on the growth performance, immune-response-associated gene expression, and serum immunoglobulin levels in broilers
To examine the effect of FSBM produced by B. subtilis on the growth performance, broilers were fed with 5% and 10% FSBM for three weeks. However, no differences were statistically confirmed in the body weight between the different diets during the entire feeding period (). Broilers fed 5% FSBM had a lower feed intake than broilers fed other treatments from Day 1 to 7 (, P < 0.05). The group with high-concentration FSBM supplementation exhibited higher feed intake throughout the feeding period than the groups with other treatments (, P < 0.05). No statistical differences were confirmed in the FCR between different FSBM supplementations during the entire feeding period (). To examine the effect of different concentrations of FSBM on the inflammatory gene expression in the spleen and bursa of Fabricius, broilers were fed with 5% and 10% FSBM. Supplementation of 5% FSBM in the diet for 21 d had no significant effect on IL-4 mRNA expression in the spleen compared with the control group (A), whereas IL-4 mRNA expression in the spleen was significantly reduced after 10% FSBM supplementation in broilers (A, P < 0.05). Similarly, 5% FSBM did not inhibit IL-10 mRNA expression in the spleen of broilers at 21 d of age (B). However, IL-10 mRNA expression was significantly reduced in the spleens of broilers after treatment with 10% FSBM (B, P < 0.05). In the bursa of Fabricius of broilers, IL-4 mRNA expression was not affected by 5% FSBM treatments over 21 d (C), whereas 10% FSBM treatments efficiently inhibited IL-4 mRNA expression in broilers (C, P < 0.05). However, no differences in IL-10 mRNA were statistically confirmed for different FSBM supplementations in the bursa of Fabricius of broilers (D). Moreover, supplementation of 5% FSBM in the diet for 21 d had no significant effect on anti-soybean allergen IgG levels compared with the control group (A), whereas anti-soybean allergen IgG levels were significantly reduced after treatment with 10% FSBM (A, P < 0.05). By contrast, no significant differences were observed in anti-soybean allergen IgA levels for different FSBM supplementation at 21 d of age (B). These results indicate that supplementation of FSBM in the diet has no effect on the growth performance of broilers. However, FSBM produced by protease and B. subtilis could alleviate the immune response caused by soybean allergens in broilers.
Figure 5. Effect of FSBM produced by B. subtilis on the immune-response-associated gene expression in the spleens and bursa of Fabricius of broilers. Effects of control (CTRL), 5% FSBM produced by B. subtilis (5% FSBM), and 10% FSBM produced by B. subtilis (10% FSBM) on IL-4 (A) and IL-10 (B) mRNA expression in the spleens of broilers at 21 d. Effects of control (CTRL), 5% FSBM produced by B. subtilis (5% FSBM), and 10% FSBM produced by B. subtilis (10% FSBM) on IL-4 (C) and IL-10 (D) mRNA expression in the bursa of Fabricius of broilers at 21 d. The values are expressed as mean ± standard deviation (n = 3). The means with different letter superscripts are significantly different (P < 0.05).
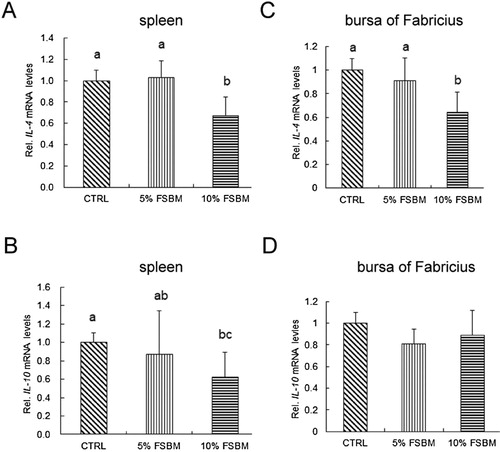
Figure 6. Effect of FSBM produced by B. subtilis on soybean-protein-specific immunoglobulin levels in broilers. (A) Effect of control (CTRL), 5% FSBM produced by B. subtilis (5% FSBM), and 10% FSBM produced by B. subtilis (10% FSBM) on the anti-soybean allergen IgG levels in broilers. (B) Effect of control (CTRL), 5% FSBM produced by B. subtilis (5% FSBM), and 10% FSBM produced by B. subtilis (10% FSBM) on the anti-soybean allergen IgA levels. The values are expressed as mean ± standard deviation (n = 3). The means with different letter superscripts are significantly different (P < 0.05).
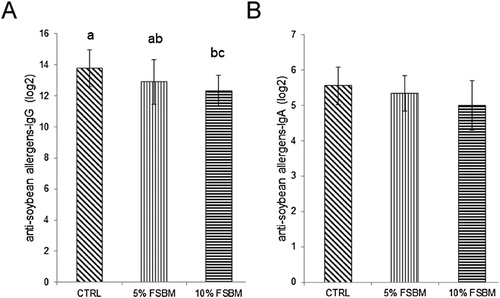
Table 3. Effect of FSBM on the growth performance of broilers.
Discussion
We demonstrated that in optimal SSF conditions for soy protein degradation, protease is used in combination with B. subtilis treatment at 100% initial moisture during a 24-h fermentation period. FSBM produced by protease and B. subtilis did not affect the growth performance of broilers. Dietary supplementation with FSBM significantly reduced the allergic immune-response-associated gene expression and serum anti-soybean allergen IgG levels in broilers.
Several reports have demonstrated that soybean oligosaccharides and crude proteins can be degraded during microbial fermentation by producing a variety of enzymes (Hong et al. Citation2004; Adeyemo and Onilude Citation2014; Su et al. Citation2018). Exogenous enzyme supplementation also increases the degradation of soybean protein and inactive anti-nutritional factors in the fermentation of SBM (Ma and Wang Citation2010; Amadou et al. Citation2011). Protease supplementation efficiently improved the nutritional value of SBM during SSF through the degradation of the large-molecular-weight soybean protein (Wang et al. Citation2014). We previously demonstrated that oligosaccharides in SBM were efficiently reduced during SSF by Lactobacillus species and C. butyricum (Su et al. Citation2018). Here, we discovered that protease supplementation increased soy protein degradation in a dose-dependent manner. Previous studies have indicated that A. oryzae and B. subtilis can degrade anti-nutritional factors and increase small-size peptides in the fermentation of SBM by producing multiple hydrolysis enzymes (Hong et al. Citation2004; Teng et al. Citation2012). In this study, a significantly larger improvement in soy protein and soybean allergen degradation during SSF was observed when probiotic supplementation was used in combination with protease than when protease treatment was used alone. Furthermore, a previous study demonstrated that trypsin inhibitor and large-size protein in FSBM were lower for treatment with B. subtilis than for treatment with A. oryzae (Teng et al. Citation2012). In this study, the anti-nutritional factors and small-size protein content in FSBM exhibited a larger improvement when protease was used in combination with B. subtilis treatment rather than A. oryzae treatment. Whether B. subtilis has a higher capacity to produce enzymes than does A. oryzae remains to be investigated.
Newborn chicks with immature digestive systems are inefficient in nutrient utilization from SBM because of insufficient endogenous enzymes (Batal and Parsons Citation2002; Choct et al. Citation2010). Feeding of SBM fermented with Aspergillus species was reported to elevate the activities of intestinal digestive enzymes and intestinal morphology in broilers (Feng et al. Citation2007b). Furthermore, feeding broilers with FSBM produced by Aspergillus species could improve growth performance (Hirabayashi et al. Citation1998; Mathivanan et al. Citation2006; Feng et al. Citation2007b). Similarly, feeding of FSBM produced by B. subtilis during the early phase benefits the growth performance of broilers (Kim et al. Citation2016). Although changes in growth performance did not reach statistical significance in the present study, FSBM produced by protease and B. subtilis still caused a similar trend in improving the FCR in broilers between Day 1 and Day 21. Microbial fermentation has been demonstrated to degrade anti-nutritional factors and increase small-size peptides (Hong et al. Citation2004; Teng et al. Citation2012) and therefore increase the availability of nutrients in broilers. Thus, the improved growth performance in FSBM-fed broilers may be caused by increased levels of amino acids and reduced anti-nutritional factors resulting from fermentation. However, the nutritional value of FSBM produced by protease and B. subtilis should be reanalyzed in a future study.
SBM containing soybean allergens (glycinin and β-conglycinin) has been reported to induce local mucosal T-cell and B-cell infiltration (Dreau et al. Citation1995), which leads to impaired growth performance in broilers (Palacios et al. Citation2004). These soybean allergens can be broken down by fermentation processes using bacteria or exogenous enzymes (Yamanishi et al. Citation1995; Feng et al. Citation2007b; Wang et al. Citation2011). In this study, protease in combination with B. subtilis treatment degraded the α (75 kDa) and β (50 kDa) subunits of β-conglycinin during the SSF of SBM. This result is consistent with a previous observation that the α and β subunits of β-conglycinin could be degraded by B. subtilis during the SSF of SBM (Zhang et al. Citation2018). Broilers fed FSBM produced by Aspergillus species had a higher level of total serum IgA and IgM than those fed the basal diet (Feng et al. Citation2007b). In this study, the serum levels of anti-soybean allergen IgG in broilers were significantly reduced by FSBM produced by protease and B. subtilis in a dose-dependent manner. Although it did not reach statistical significance, FSBM produced by protease and B. subtilis with low levels of soybean allergen also caused a similar decreasing trend in the serum levels of anti-soybean allergen IgA. A previous study reported that intestinal mucosal immune responses to soybean allergen were elevated by high levels of inflammatory cytokines, such as IL-4 and IL-10 (Sun et al. Citation2008). Glycinin and β-conglycinin in SBM could promote intestinal epithelial cells to express inflammatory cytokines (Xu et al. Citation2010). Reduced IL-4 and IL-6 mRNA expression was observed in the small intestine of pigs fed FSBM (Zhang et al. Citation2018). In this study, IL-4 mRNA expression in the spleen and bursa of Fabricius of broilers consistently decreased with increasing FSBM supplementation. The results of serum immunoglobulin levels and inflammatory gene expression implied that reduced soybean allergen contents in FSBM could improve allergic immune response in broilers.
In conclusion, supplementation of B. subtilis and protease during the SSF of SBM increases the protein degradation and reduces the soybean allergen content. FSBM produced by B. subtilis and protease with high levels of small-size protein and low levels of soybean allergen could improve immune response in broilers.
Disclosure statement
No potential conflict of interest was reported by the authors.
ORCID
Yu-Hsiang Yu http://orcid.org/0000-0001-9629-8478
References
- Abd El-Hack M , Alagawany M , Arif M , Emam M , Saeed M , Arain MA , Siyal FA , Patra A , Elnesr SS , Khan RU. 2018. The uses of microbial phytase as a feed additive in poultry nutrition – a review. Ann Anim Sci. 18:639–658. doi: 10.2478/aoas-2018-0009
- Abudabos AM. 2012. Effect of enzyme supplementation to normal and low density broiler diets based on corn-soybean meal. Asian J Anim Vet Adv. 7:139–148. doi: 10.3923/ajava.2012.139.148
- Abudabos AM , Alyemni AH , Dafalla YM , Khan RU. 2017. Effect of organic acid blend and Bacillus subtilis alone or in combination on growth traits, blood biochemical and antioxidant status in broiler exposed to Salmonella typhimurium challenge during the starter phase. J Appl Anim Res. 45:538–542. doi: 10.1080/09712119.2016.1219665
- Abudabos AM , Yehia HM. 2013. Performance, intestinal morphology and microbiology of broilers fed corn-soybean meal diets supplemented with enzyme and antibiotic. J Food Agric Environ. 11:642–646.
- Adeyemo SM , Onilude AA. 2014. Reduction of oligosaccharide content of soybeans by the action of Lactobacillus plantarum isolated from fermented cereals. Afr J Biotechnol. 13:3790–3796. doi: 10.5897/AJB2013.13398
- Amadou I , Le GW , Shi YH , Gbadamosi OS , Kamara MT , Jin S. 2011. Optimized Lactobacillus Plantarum Lp6 solid-state fermentation and proteolytic hydrolysis improve some nutritional attributes of soybean protein meal. J Food Biochem. 35:1686–1694. doi: 10.1111/j.1745-4514.2010.00493.x
- Batal AB , Parsons CM. 2002. Effect of fasting versus feeding oasis after hatching on nutrient utilization in chicks. Poult Sci. 81:853–859. doi: 10.1093/ps/81.6.853
- Chi CH , Cho SJ. 2016. Improvement of bioactivity of soybean meal by solid state fermentation with Bacillus amyloliquefaciens versus Lactobacillus spp. and Saccharomyces cerevisiae . LWT-Food Sci Technol. 68:619–625. doi: 10.1016/j.lwt.2015.12.002
- Choct M , Dersjant-Li Y , McLeish J , Peisker M. 2010. Soy oligosaccharides and soluble non-starch polysaccharides: a review of digestion, nutritive and anti-nutritive effects in pigs and poultry. Asian-Australas J Anim Sci. 23:1386–1398. doi: 10.5713/ajas.2010.90222
- Cromwell GL. 2012. Soybean meal: an exceptional protein source. Soybean Meal InfoCenter, Iowa (USA).
- Dreau D , Lalles J , Toullec R , Salmon H. 1995. B and T lymphocytes are enhanced in the gut of piglets fed heat-treated soyabean proteins. Vet Immunol Immunopathol. 47:69–79. doi: 10.1016/0165-2427(94)05376-4
- Erdaw MM , Wu S , Iji PA. 2017. Growth and physiological responses of broiler chickens to diets containing raw, full-fat soybean and supplemented with a high-impact microbial protease. Asian-Australas J Anim Sci. 30:1303–1313. doi: 10.5713/ajas.16.0714
- Feng J , Liu X , Xu ZR , Liu YY , Lu YP. 2007a. Effects of Aspergillus oryzae 3.042 fermented soybean meal on growth performance and plasma biochemical parameters in broilers. Anim Feed Sci Technol. 134:235–242. doi: 10.1016/j.anifeedsci.2006.08.018
- Feng J , Liu X , Xu ZR , Wang YZ , Liu JX. 2007b. Effects of fermented soybean meal on digestive enzyme activities and intestinal morphology in broilers. Poult Sci. 86:1149–1154. doi: 10.1093/ps/86.6.1149
- Grant G. 1989. Anti-nutritional effects of soyabean: a review. Prog Food Nutr Sci. 13:317–348.
- Hirabayashi M , Matsui T , Yano H , Nakajima T. 1998. Fermentation of soybean meal with Aspergillus usamii reduces phosphorus excretion in chicks. Poult Sci. 77:552–556. doi: 10.1093/ps/77.4.552
- Hong KJ , Lee CH , Kim SW. 2004. Aspergillus oryzae GB-107 fermentation improves nutritional quality of food soybeans and soybean meal. J Med Food. 7:430–435. doi: 10.1089/jmf.2004.7.430
- Khan RU , Naz S. 2013. Applications of probiotics in poultry production. Worlds Poult Sci J. 69:621–632. doi: 10.1017/S0043933913000627
- Kim SK , Kim TH , Lee SK , Chang KH , Cho SJ , Lee KW , An BK. 2016. The use of fermented soybean meals during early phase affects subsequent growth and physiological response in broiler chicks. Asian-Australas J Anim Sci. 29:1287–1293. doi: 10.5713/ajas.15.0653
- Ma Y , Wang T. 2010. Deactivation of soybean agglutinin by enzymatic and other physical treatments. J Agric Food Chem. 58:11413–11419. doi: 10.1021/jf1017466
- Mathivanan R , Selvaraj P , Nanjappan K. 2006. Feeding of fermented soybean meal on broiler performance. Int J Poult Sci. 5:868–872. doi: 10.3923/ijps.2006.868.872
- Mukherjee R , Chakraborty R , Dutta A. 2016. Role of fermentation in improving nutritional quality of soybean meal – a review. Asian-Australas J Anim Sci. 29:1523–1529. doi: 10.5713/ajas.15.0627
- Palacios MF , Easter RA , Soltwedel KT , Parsons CM , Douglas MW , Hymowitz T , Pettigrew JE. 2004. Effect of soybean variety and processing on growth performance of young chicks and pigs. J Anim Sci. 82:1108–1114. doi: 10.1093/ansci/82.4.1108
- Su LW , Cheng YH , Hsiao FSH , Han JC , Yu YH. 2018. Optimization of mixed solid-state fermentation of soybean meal by Lactobacillus species and Clostridium butyricum . Pol J Microbiol. 67:297–305. doi: 10.21307/pjm-2018-035
- Sun P , Li D , Li Z , Dong B , Wang F. 2008. Effects of glycinin on IgE-mediated increase of mast cell numbers and histamine release in the small intestine. J Nutr Biochem. 19:627–633. doi: 10.1016/j.jnutbio.2007.08.007
- Teng D , Gao M , Yang Y , Liu B , Tian Z , Wang J. 2012. Bio-modification of soybean meal with Bacillus subtilis or Aspergillus oryzae . Biocatal Agric Biotechnol. 1:32–38. doi: 10.1016/j.bcab.2011.08.005
- Wang J , Liu N , Song M , Qin C , Ma C. 2011. Effect of enzymolytic soybean meal on growth performance, nutrient digestibility and immune function of growing broilers. Anim Feed Sci Technol. 169:224–229. doi: 10.1016/j.anifeedsci.2011.06.012
- Wang T , Qin GX , Sun ZW , Zhao Y. 2014. Advances of research on glycinin and β-conglycinin: a review of two major soybean allergenic proteins. Crit Rev Food Sci Nutr. 54:850–862. doi: 10.1080/10408398.2011.613534
- Xu J , Zhou A , Wang Z , Ai D. 2010. Effects of glycinin and β-conglycinin on integrity and immune responses of mouse intestinal epithelial cells. J Anim Plant Sci. 20:170–174.
- Yamanishi R , Huang T , Tsuji H , Bando N , Ogawa T. 1995. Reduction of the soybean allergenicity by the fermentation with Bacillus natto . Food Sci Technol Int. 1:14–17.
- Zhang Y , Shi C , Wang C , Lu Z , Wang F , Feng J , Wang Y. 2018. Effect of soybean meal fermented with Bacillus subtilis BS12 on growth performance and small intestinal immune status of piglets. Food Agric Immunol. 29:133–146. doi: 10.1080/09540105.2017.1360258
- Zhang YT , Yu B , Lu YH , Wang J , Liang JB , Tufarelli V , Laudadio V , Liao XD. 2017. Optimization of the fermentation conditions to reduce anti-nutritive factors in soybean meal. J Food Process Preserv. 41:e13114. doi: 10.1111/jfpp.13114