Abstract
Vulnerability to emotional disorders like depression derives from interactions between early and late environments, including stressful conditions. The serotonin (5HT) system is strongly affected by stress and chronic unpredictable stress can alter the 5HT system. We evaluated the distribution of active serotonergic neurons in the dorsal raphe nucleus (DR) through immunohistochemistry in maternally separated and chronically stressed rats treated with an antidepressant, tianeptine, whose mechanism of action is still under review. Male Wistar rats were subjected to daily maternal separation (MS) for 4.5 h between postnatal days (PND) 1–21, or to animal facility rearing (AFR). Between (PND) days 50–74, rats were exposed to chronic unpredictable stress and were treated daily with tianeptine (10 mg/kg) or vehicle. We found an interaction between the effects of MS and chronic unpredictable stress on Fos-5HT immunoreactive cells at mid-caudal level of the DR. MS-chronically stressed rats showed an increase of Fos-5HT immunoreactive cells compared with AFR-chronically stressed rats. The ventrolateral (DRL/VLPAG) and dorsal (DRD) subdivisions of the DR were significantly more active than the ventral part (DRV). At the rostral level of the DR, tianeptine decreased the number of Fos-5HT cells in DR in the AFR groups, both unstressed and stressed. Overall, our results support the idea of a match in phenotype exhibited when the early and the adult environment correspond.
Introduction
Depression is a prevalent psychological disorder (Belzung, Willner, & Philippot, Citation2015; Roy & Campbell, Citation2013; Willner, Scheel-Kruger, & Belzung, Citation2013). Its etiopathogenesis is based upon multiple factors that may act at psychological, biological, genetic, and social levels (Chirita, Gheorman, Bondari, & Rogoveanu, Citation2015). There is great variability in individual susceptibility to develop depression, and one attempt to explain its etiology has been the diathesis/stress model, which considers separately the issues of vulnerability (the diathesis) and precipitation (the stress) (Monroe & Simons, Citation1991). The basic premise is that stress activates a diathesis, transforming the potential of predisposition into the presence of psychopathology.
The diathesis or predisposition to become depressed may arise in a variety of ways, and at different stages of the life cycle, including multiple risk genes and early life experiences (Andersen, Citation2015; Willner et al., Citation2013), particularly the disruption of the mother-offspring relationship (Hennessy, Kaiser, Tiedtke, & Sachser, Citation2015; Nishi, Horii-Hayashi, & Sasagawa, Citation2014; Vetulani, Citation2013). Rodent models of early maternal separation (MS) produce lasting modifications in the neurobiological substrate of the offspring, such as disturbances in the serotonergic system (Ohta et al., Citation2014; Own, Iqbal, & Patel, Citation2013), decrements in hippocampal neurogenesis (Lajud, Roque, Cajero, Gutierrez-Ospina, & Torner, Citation2012) and brain-derived neurotrophic factor protein expression (Xue, Shao, Wang, & Shao, Citation2013). Exposure to stressful events during early age will affect how offspring will cope with stressful events in adulthood (de Kloet, Joels, & Holsboer, Citation2005). Some of these experiences can lead to adverse outcomes, such as depression (McEwen, Eiland, Hunter, & Miller, Citation2012), but can also shape an individual’s physiological reactivity to future risk and provide protective factors and subsequent adaptation trajectories (Bock, Rether, Groger, Xie, & Braun, Citation2014; Santarelli et al., Citation2017).
In regard to precipitation, the onset of depression is promoted mostly by external events, either an acute event such as bereavement, or an accumulation of chronic minor stresses (Willner et al., Citation2013). The latter is emulated in the rodent model of chronic unpredictable stress, a widely used model of depression (Willner, Citation2005; Willner et al., Citation2013). The major physiological response to stress is the activation of the hypothalamic pituitary adrenal (HPA) axis. In response to stress, a chain of events involving corticotrophin-releasing hormone (CRF) and adrenocorticotropic hormone (ACTH) ultimately lead to the secretion of corticosteroids, including glucocorticoids. The HPA axis is normally regulated by a negative-feedback system, but chronic elevation of stress hormones impairs this regulatory system, and could lead to its continuous hyperactivity (Herman, Ostrander, Mueller, & Figueiredo, Citation2005; Pariante & Miller, Citation2001). Plasma salivary cortisol and cortisone levels have been found to be elevated in patients affected with depression, and the secretion of urinary free cortisol is increased (Roy & Campbell, Citation2013). Therefore, in both depression and chronic stress conditions, HPA axis dysregulation has been noted, associated with hypercortisolism and raised ACTH levels (Wong et al., Citation2000).
Together with hyperactivity of the HPA axis, elevated levels of pro-inflammatory cytokines and structural changes in certain brain regions, an imbalance of monoamines, particularly noradrenaline and serotonin (5HT), occurs in the brain during depression (Chirita et al., Citation2015; Haase & Brown, Citation2015; Roy & Campbell, Citation2013). Similarly, following chronic unpredictable stress, spectrophotometric, and neurochemical analyzes have found a depletion of 5HT in some brain regions, such as the hippocampus, cortex, and amygdala (Ahmad, Rasheed, Banu, & Palit, Citation2010; Gupta, Radhakrishnan, & Kurhe, Citation2014; Lin et al., Citation2016). Some antidepressants exert their therapeutic action by increasing the extracellular availability of monoamines, mostly at synaptic level, laying the foundations for the monoaminergic hypothesis of depression. Extensive research supports a role for the 5HT system in the development of both mood and anxiety disorders, and human and animal studies have also shown that the stress response is modulated in part by serotonergic neurotransmission (Waselus, Nazzaro, Valentino, & Van Bockstaele, Citation2009). The midbrain dorsal raphe nucleus (DR) is the origin of the central serotonergic system, containing approximately 50% of the 5HT neurons in the rat brain. These neurons are topographically organized, with anatomically distinct groups of 5HT neurons receiving synaptic input from specific forebrain and brainstem structures, giving rise to anatomically distinct efferents to forebrain and brainstem structures. Different groups of neurons are differently activated by stress exposure, and it has been suggested that it is mainly the mid-rostrocaudal and caudal parts of the DR that regulate anxiety-related responses, while 5HT neurons of the lateral wings of the DR inhibit panic-related responses (Hale & Lowry, Citation2011). Since serotonin is the most abundant neurotransmitter of the DR, this structure, due to its target areas and emerging fibers, is involved in the control of various physiological functions and has been implicated in brain dysfunction, especially mood disorders such as depression (Michelsen, Prickaerts, & Steinbusch, Citation2008). Multiple anxiety or stress-related behaviors alter the activation of serotonergic neurons in subregions of the DR (Hale, Shekhar, & Lowry, Citation2012), resulting in an increase in Fos expression (Bouwknecht et al., Citation2007), the protein product of the immediate-early gene marker of cellular activation, c-fos (Dragunow & Faull, Citation1989).
Tianeptine is a modified tricyclic antidepressant with atypical pharmacological and neurochemical properties (Brink, Harvey, & Brand, Citation2006). The latest evidence on its mechanism of action indicates a dynamic interplay between numerous neurotransmitter systems and an ability to restore normal neuroplasticity in circumscribed limbic brain regions and to indirectly reverse stress-induced impairments in synaptic glutamate transmission (McEwen & Gianaros, Citation2011), via activation of opioid receptor signaling (as a μ-opioid receptor agonist) (Gassaway, Rives, Kruegel, Javitch, & Sames, Citation2014). This antidepressant selectively enhances the synaptic uptake of serotonin, but not of norepinephrine or dopamine, in rat cortex, hippocampus and hypothalamus (Brink et al., Citation2006). In both naive and chronic mildly stressed rats, tianeptine reduced the levels of CRH mRNA in the dorsal and ventral bed nucleus of the stria terminalis (BNST) and in the central amygdala (Kim et al., Citation2006). It also attenuated the increase of ACTH, corticosterone and Fos expression in the paraventricular nucleus induced by lipopolysaccharide or stress by immobilization (Castanon, Konsman, Medina, Chauvet, & Dantzer, Citation2003; Delbende, Contesse, Mocaer, Kamoun, & Vaudry, Citation1991). Clinical studies show that tianeptine has a better side effect profile than selective serotonin reuptake inhibitors and tricyclic antidepressants (Gassaway et al., Citation2014; Brink et al., Citation2006). In maternally deprived rats, tianeptine exerted antidepressant effects, altering neurochemical pathways related to depression (Della et al., Citation2013). Our previous results showed that tianeptine decreased the chronic stress-induced increase of epinephrine, norepinephrine, and anxiety behavior (Trujillo, Masseroni, Levin, & Suárez, Citation2009).
As the relationship between early environment events and subsequent stressors suffered in life can condition psychobiological health in adulthood, we asked, through an animal model, how the interaction between MS and chronic unpredictable stress during adult life alters the immunoreactivity of neurons that express Fos and 5HT in the DR nucleus. We also assessed whether tianeptine can revert some of these modifications.
Methods
Animals
All rat handling and experimental procedures were approved by the Institutional Committee for Care and Use of Experimental Animals of Faculty of Natural, Physical, and Exact Sciences CEFyN (Resol.571-HCD-2014; Acta 8/2015) in accordance with the NIH Guide on Care and Use of Laboratory Animals.
Wistar-derived rats were bred and reared in our colony. Rats were housed in a temperature-controlled room (21 ± 1 °C) under artificial illumination (12 h:12 h light/dark schedule; lights on at 07:00 h). Except when required by the stress paradigm the rats had ad libitum access to food (standard lab chow) and tap water.
Pregnancy and early MS
Two non-related females were mated with a male and they remained together until there was physical evidence of pregnancy. After that the male was removed, the females were housed together until around gestation day 15 when they were put in separate cages. Groups of dams were selected for either MS (n = 11) or standard animal facility rearing (AFR) (n = 11). The day of birth was designated as postnatal day (PND) 0. Litters were culled on day PND 1-10 pups/dam (4–5 females, 5–6 males).
The MS procedure was based on a previously standardized protocol. For MS litters, rats were separated from the mother for 4.5 h every day from PND1 to PND 21 (Ogawa et al., Citation1994). Separation consisted of removing the dam from the home cage and placing it alone into an adjacent cage while the litter was kept together in the nest. After the separation period, the dam was returned to the home cage.
Separations were carried out between 09:00 h and 13:30 h. In AFR litters, pups remained with the dams undisturbed until weaning age at PND 22, except for routine cage cleaning twice a week.
Post-weaning housing conditions
From PND 22 until PND 49, male rats were selected and housed in standard cages in groups of four. They were handled daily by the same researcher to minimize stress reactions to manipulation at the time of treatment application. At PND 49, male offspring from both rearing conditions (MS and AFR) were randomly subdivided into four treatments, unstressed with vehicle or with tianeptine, and unpredictable chronic stress with vehicle or with tianeptine, yielding eight experimental groups: 1 – AFR-unstressed/vehicle (control) (n = 4); 2 – AFR-unstressed/tianeptine (n = 5); 3 – AFR-chronic stress/vehicle (n = 4); 4 – AFR-chronic stress/tianeptine (n = 4), 5–MS-unstressed/vehicle (n = 4), 6– MS-unstressed/tianeptine (n = 5), 7 – MS-chronic stress/vehicle (n = 5), and 8 – MS-chronic stress/tianeptine (n = 5). To control for litter effects, each experimental group was made up of rats from different litters (one rat per litter per condition). Male offspring were housed in standard cages in groups of four until the end of the experimental period.
We thus used a factorial (2 × 2 × 2) experimental design, with AFR or MS, stress (unstressed or chronically stressed), and drug (vehicle or tianeptine) as between-subjects factors.
Unpredictable chronic stress
At PND 50, rats in the stressed groups were exposed to an unpredictable 24-day chronic stress paradigm (): 4 h of noise produced by an alarm bell (85 dB; 2.5 Hz) (randomly applied 6 times during stress protocol); loss of consciousness by ether anesthesia and subsequent ether exposure for 2 min (5 times); two intraperitoneal (i.p.) injections of 0.5 ml isotonic saline (ClNa 0.9%) solution at 4 h intervals (4 times); restraint for 1 h by placement inside a 6-cm-diameter metal grid cylinder (4 times); and food deprivation for 24 h (3 times). There were also days without stress (3 times) (Suarez et al., Citation1999; Trujillo, Durando, & Suarez, Citation2016). Only one stressor was applied per day. To maximize the unpredictability, the stressors were applied in random order and at varying times during the light phase, except on day 24 when noise was used as the last stressor.
Table 1. Chronic stress model.
All rats received the same sequence of stressors in each replication of the experiments. The unstressed control group was not exposed to any stressor and these rats remained in their home cages until euthanasia for brain processing.
Tianeptine and vehicle administration
Starting at PND 50 rats were treated daily during 24 days with either tianeptine (commercial name: Stablon; from Servier laboratories of France) or vehicle according to the designated group (). Tianeptine (10 mg/kg) was prepared diluted in 0.9% NaCl solution (vehicle). Both tianeptine and vehicle were administered i.p. in an end volume of 0.5 ml, between 12:00 h and 13:00 h.
Figure 1. Timeline of different protocols applied. AFR: Standard Animal Facility Reared; MS: maternal separated; PND: post natal day.
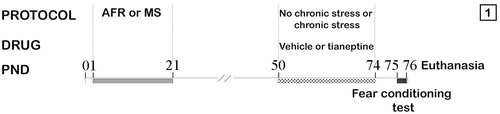
Twenty-four hours after the last stress session (PND 75), each rat was tested in a fear conditioning test from PND 75 to PND 76 (). The results of this test have been previously published (Pollano, Zalosnik, Durando, & Suarez, Citation2016).
Immunohistochemical procedure
At PND 77, rats (300–350 g body weight) were perfuse-fixed between 9:00 h and 12:00 h. The rats were deeply anesthetized using intraperitoneal injection of chloral hydrate (0.54 g/Kg) and transcardially perfused with heparinized 0.9% saline followed by 4% paraformaldehyde, 0.2% picric acid alcoholic saturated solution in 0.1 M phosphate buffer (PB); brains were removed, post-fixed in the perfusion solution for 24 h at 4 °C and then stored in 20% sucrose-PB solution at 4 °C. Brains were sectioned using a freezing microtome into coronal slice of 40 µm thickness containing the DR nucleus, according to a stereotaxic atlas of the rat brain (Paxinos & Watson, Citation2007). The slices were collected in a solution of 1.7% glycerol, 2% ethylene glycol and 3.4% PB 0.1 M at −20 °C until processing.
After endogenous peroxidase blocking (10% methanol and 10% H2O2 in PB; for 1 h) and nonspecific binding to serum constituents blocking (10% normal horse serum in PB; for 1 h), slices were incubated over 48 h at room temperature in primary rabbit polyclonal anti-Fos antiserum (Oncogen Science; 1:10,000) in 0.1 M PB with 0.1% Triton X-100 (PB-TX). After rinsing 3 times for 5 min in PB, the sections were further incubated in a biotinylated secondary antibody solution, anti-rabbit immunoglobulin (IgG) (anti-rabbit Jackson; 1:500) for 60 min at room temperature, and finally treated with the avidin–biotin–peroxidase complex (ABC Elite kit; Vector Laboratories) for 2 h at room temperature, using intensified Diaminobenzidine (DAB) as chromogen. This reaction was intensified by adding cobalt chloride (0.5%) and nickel sulfate (1%) to the developing medium. Immunoreactivity for Fos was localized to cell nuclei, appearing as a dark gray–black stain. For dual immunostaining for 5HT, the series of Fos-labeled sections were incubated at 4° C and for 48 h with the primary antibody: polyclonal rabbit anti-5HT antibody (ImmunoStar Inc; 1:10,000). After incubation, the sections were rinsed and incubated with biotin-labeled anti-rabbit IgG (anti-rabbit Jackson; 1:500) and avidin-biotin-peroxidase complex (ABC Elite kit; Vector Laboratories) for 2 h at room temperature, using DAB as a chromogen. 5HT immunoreactivity was localized to the cell cytoplasm and was visible as light-brown staining.
Free-floating sections were mounted on gelatinized glass slides using Albrecht gelatin, air-dried overnight, dehydrated in xylene, and cover-slipped with DPX mounting medium.
Cell counts and quantification
Slices were examined under a light microscope (BX 41 Olympus). Magnification was 400X (40 × objective; 10 × ocular). Images were captured using a high-resolution digital camera (Olympus Corporation, Tokyo, Japan). The numbers of Fos-5HT immunoreactive neurons were counted manually in the DR nucleus at three rostrocaudal levels: −6.96 (rostral); −7.68 (mid-rostral) and −8.04 (mid-caudal) mm form bregma (Paxinos & Watson, Citation2007). Each of these levels was counted in one slice per rat, using a total of three slices per rat. At the level of −8.04 mm form bregma, quantification of the cells was performed according to the subdivisions of the DR nucleus. Subdivisions are illustrated in , and are as follows: dorsal part (DRD), ventral part (DRV); ventrolateral part/ventrolateral periaqueductal gray (DRL/VLPAG). The number of cells sampled within the DRL/VLPAG was the sum of cells counted in the right and left sides of this subdivision. An observer blind to the assignment of treatment performed the counting of the immunoreactive cells. shows schematic photomicrographs of DRN analyzed sections at −8.04 mm from bregma.
Statistical analysis
Immunoreactivity data from each level of DR were evaluated using a three-way ANOVA, considering AFR or MS, chronic stress (unstressed or chronic stress), and drug (vehicle or tianeptine) as independent variables. Particularly, at the rostrocaudal level of −8.04 mm from bregma, data from the distinct subdivisions of DR were evaluated using a Repeated Measures model, considering subdivisions (DRL/VLPAG, DRD, and DRV) as intra subject factor and AFR or MS, chronic stress (unstressed or chronic stress), and drug (vehicle or tianeptine) as between factors. Data were presented as mean ± standard error of the mean (SEM). Fisher’s least significant difference (LSD) post hoc test was performed for further examination of group differences. Significance was set at p < .05. All analyzes were conducted by using Infostat software (www.infostat.com.ar).
Results
The results obtained in the three rostrocaudal levels analyzed (−6.96 mm, −7.68 mm and −8.04 mm from bregma) are shown below.
Number of fos-5-HT immunoreactive cells at −6.96 mm from bregma
There was not a significant MS × stress × drug interaction between factors (F1,28 < 0.01, p = 0.99) on the number of double-labeled cells for Fos and 5HT at −6.96 mm from bregma. However, a significant interaction was found between the effects produced by MS and drug on the number of double-labeled cells for Fos and 5-HT within the DR, quantified at −6.96 mm from bregma (F1,28= 5.06, p = 0.03, partial eta squared (ηp2) = 0.15). Post hoc analysis showed that, in AFR tianeptine-treated rats, the number of immunoreactive cells for Fos and 5HT was significantly lower compared with the rest of the experimental groups (p < .05) ().
Figure 3. Representative photomicrographs of Fos-5HT immunoreactivity within the DR at −8.04 mm from bregma of maternally separated, chronically stressed, and treated with tianeptine rats. Boxed areas indicate regions displayed at higher magnification below and in the left of each photo. White arrows indicate 5HT immunolabeled cells and green arrows indicate double immunolabeled cells for Fos and 5HT.
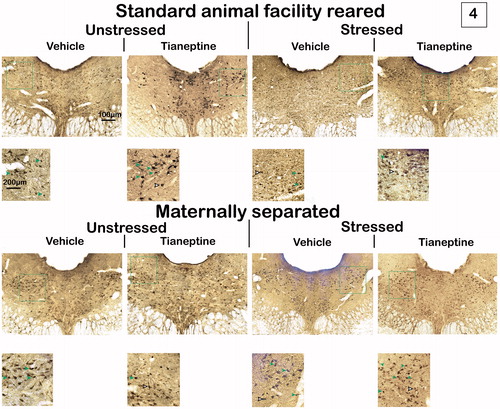
Figure 4. Effects of maternal separation (MS), chronic unpredictable stress (CUS) and tianeptine (tia) on Fos-5HT immunoreactivity within the DR at three rostrocaudal levels. Values are mean ± SEM of standard animal facility rearing (AFR) and maternally separated rats submitted to chronic stress or unstressed (Non-CUS) under tianeptine or vehicle (veh) treatment. The number of rats for each treatment is included inside each bar. (A) Immunoreactive cells for Fos-5HT at −6.96 mm from bregma. ANOVA revealed a significant maternal separation X drug interaction, #p < .05 versus the other groups, LSD post hoc test. (B) Immunoreactive cells for Fos-5HT at −7.68 mm from bregma. No significant effect or interaction was found. (C) Immunoreactive cells for Fos-5HT at −8.04 mm from bregma. Repeated measures revealed a significant maternal separation X chronic stress interaction. Different letters indicate significant differences between groups (p < .05), LSD post hoc test.
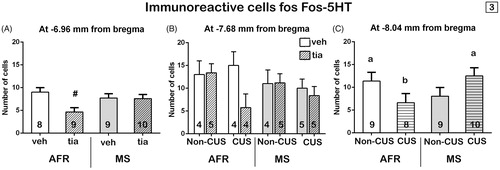
Figure 5. Effects of maternal separation (MS) and chronic unpredictable stress (CUS) on Fos-5HT immunoreactivity according to each subdivision within the dorsal raphe at −8.04 mm from bregma. Values are mean ± SEM of standard animal facility rearing (AFR) and maternally separated rats submitted to chronic stress or unstressed (Non-CUS). Repeated measures revealed a significant maternal separation X chronic stress interaction and a subdivision main effect.
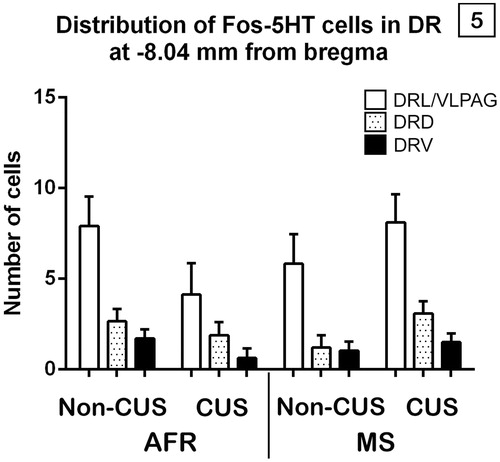
Number of fos-5-HT immunoreactive cells at −7.68 mm from bregma
At this level, MS (F1,28= 0.58, p = 0.45), stress (F1,28= 1.21, p = 0.28), drug (F1,28= 1.43, p = 0.24) and their interactions (MS × stress: F1,28= 0.05, p = 0.83; MS × drug: F1,28= 0.75, p = 0.39; stress × drug: F1,28= 1.78, p = 0.19; MS × stress × drug: F1,28= 0.84, p = 0.37) did not have significant effects on the number of Fos-5HT neurons ().
Number of fos-5HT immunoreactive cells at −8.04 mm from bregma
There was not a significant subdivisions × MS × stress × drug interaction between factors (F1,28= 0.18, p = 0.84) on the number of double-labeled cells for Fos and 5HT of each distinct subdivision of DR at −8.04 mm from bregma. However, a significant double interaction was found between the effects produced by MS and stress in adulthood on the total number of double-labeled cells for Fos and 5HT within the DR, quantified at −8.04 mm from bregma (F1,28= 7.49, p = 0.01, ηp2 = 0.21). Post hoc analysis showed that, in AFR non-chronically stressed and in MS chronically stressed rats, the number of total immunoreactive cells for Fos and 5-HT was significantly higher compared with AFR chronically stressed rats (p < .05) ().
Also, a significant main effect of subdivision (F1,28 =27.85, p = 0.001, ηp2 = 0.49) was found on the number of double-labeled cells for Fos and 5HT of DR at −8.04 mm from bregma. Post hoc analysis revealed that the number of Fos-5HT immunoreactive cells was significantly higher within the DRL/VLPAG than both within the DRD and the DRV; and within the DRD compared with the DRV (p < .01) ().
Discussion
Effects of MS, chronic unpredictable stress and tianeptine administration on fos-5HT immunoreactive neurons at −8.04 mm from bregma
This study assessed how combined MS and chronic unpredictable stress plus the use of tianeptine induced alterations in the activation patterns of serotonergic neurons within the DR. At −8.04 mm from bregma, an interaction was found between the two stress-protocols. The sum of the two stressors, MS and chronic unpredictable stress, significantly augmented the number of Fos-5HT immunoreactive neurons in the DR compared to rats that were subjected only to the chronic stress protocol, and are similar to the values obtained in the control group (AFR non-chronically stressed). However, a decrease of the number of Fos-5HT immunoreactive neurons in the DR tended to occur when only one stressor is present (MS or chronic variable stress). Looking at the whole interaction picture, we found a match between Fos-5HT immunoreactivity in the DR of rats subjected to the combination of stress protocols and that of control rats. Corroborating these findings, an unpredictable chronic variable mild stress protocol appeared to normalize the effects of prenatal stress on the 5HT system within the DR of male but not female rats (Van den Hove et al., Citation2014). These evidences imply that an adaptation phenomenon occurs in chronically stressed rats when early stress is present. The outcome is thus similar and adaptive when the early environment resembles that of adulthood - no stress in the case of controls or both stressors at different stages of life - while a greater risk for a pathological outcome is predicted for a mismatch between the conditions experienced during early development and those experienced later in life – only MS or chronic unpredictable stress (Bock et al., Citation2014; Schmidt, Citation2011). These observations raised a match/mismatch hypothesis, supported by studies that shows how early programing can change, for example, behavioral markers of psychosis susceptibility (Daskalakis, Oitzl, Schachinger, Champagne, & de Kloet, Citation2012), levels of 5HT receptor type 1 A (5HT1A) (Raftogianni, Diamantopoulou, Alikaridis, Stamatakis, & Stylianopoulou, Citation2012) and electrophysiological recordings of DR (Oosterhof, El Mansari, Merali, & Blier, Citation2016) when later environmental conditions matches.
Although the mechanisms underlying the programed changes following MS are not the subject of the present study, it remains possible that increases in Fos-5HT immunoreactivity seen in rats exposed to both protocols, compared to those exposed only to chronic unpredictable stress may be associated with CRF regulation. The DR is a complex nucleus, not only in terms of afferent and efferent organization, but also in the multitude of neurotransmitter/neuropeptide systems distributed throughout this region and the presence of multiple members of the CRF family (Waselus, Valentino, & Van Bockstaele, Citation2011). Stress effects on the serotonergic system in the DR are mediated by CRF, which alters the firing rate of these neurons and consequently their release to target regions (Waselus et al., Citation2011). CRF is regulated, in turn, by two different types of receptors: CRF1 and CRF2, both expressed in the DR. The CRF1 receptor generally predominates in most central nervous system; however, the DR is unique in also expressing relatively high levels of CRF2 receptor (Meloni, Reedy, Cohen, & Carlezon, Citation2008; Tanahashi, Yamamura, Nakagawa, Motomura, & Okada, Citation2012). Interestingly, the vast majority (85%–100%) of CRF2 receptor mRNA-expressing neurons are serotonergic with the exception of the caudal DR (approximately −8.40 to −8.60 mm bregma) (Staub, Spiga, & Lowry, Citation2005). While CRF1 activation seems to inhibit serotonergic neurons of the DR, CRF2 activation increases both discharge rates of 5-HT neurons and 5-HT release in certain forebrain targets (Donner et al., Citation2016; Korosi et al., Citation2006; Waselus et al., Citation2011). Thus, CRF has opposing effects in the DRN that depend on concentration-dependent CRF1 and CRF2 receptor activation (Tanahashi et al., Citation2012). MS could be associated with a redistribution of CRF1 and CRF2 in DR-5-HT neurons that primes the serotonergic system to be activated by subsequent stress (Bravo, Dinan, & Cryan, Citation2014). Probably the chronic stress protocol used activated CRF1, which may account for the decrease in the number of Fos-5HT neurons at this level. Supporting this explanation, chronic 30-day administration of CRF1 receptor antagonists ameliorated the effects of several months’ exposure to chronic mild stress, including body weight loss and deterioration in physical appearance, and altered the anxiety response (Griebel et al., Citation2002) and pre-perfusion with CRF1 antagonist into the DR inhibited CRF-induced serotonin reduction (Tanahashi et al., Citation2012).
Another possible explanation for the Fos-5HT immunoreactivity observed within the DR of the MS-chronically stressed group may be associated with alterations in the 5HT1A receptor, since somatodendritic 5HT1A receptors normally diminish the excitability of 5HT raphe neurons (Altieri, Garcia-Garcia, Leonardo, & Andrews, Citation2013). 5HT1A receptor antagonist administration potentiated the number of Fos-5HT positive neurons in the DR (Goel, Innala, & Viau, Citation2014). In line with this view, the MS protocol programs persistent changes in the sensitivity of DR serotonergic neurons to 5HT1A of 5HT neurotransmission in adult rats (Gartside, Johnson, Leitch, Troakes, & Ingram, Citation2003), which may result in changes in 5HT activation and neurotransmission under specific conditions or changes in the adaptive response to later chronic stress.
The action of 5HT in the synapse is terminated by reuptake, so another cause of 5HT system deregulation could be the alteration of the serotonin transporter produced by MS, as has been reported by Vicentic et al. (Citation2006). Lastly, alterations in 5HT transporter function can cause adaptive changes in 5HT1A receptors (Vicentic et al., Citation2006). Consequently, further studies are necessary to clarify the exact mechanism underlying the effects of the combination of MS and chronic unpredictable stress on 5HT activation within the DR.
Our results indicate that the chronic stress protocol decreased the number of active serotonin cells in the DR. Similarly, chronic unpredictable stress for 6 weeks has been shown to induce a significant decline of about 35% in the mean spontaneous neural firing activity of 5-HT neurons recorded from the DR, and a significant decrease in the number of spontaneously active neurons (Bambico, Nguyen, & Gobbi, Citation2009).
Tianeptine did not produce any change in Fos expression within serotonergic neurons in the DR at −8.04 mm from bregma. Using the patch-clamp method, Kim et al. (Citation2002) reported that tianeptine inhibits currents induced by inhibitory GABA and glycine neurotransmitters, resulting in increased excitability of serotonergic neurons in this nucleus. This contrasts with our results. However, sustained tianeptine administration (20 mg/kg/day for 14 days) did not modify the firing activity of 5-HT neurons in the DR (Pineyro, Deveault, de Montigny, & Blier, Citation1995). One possible explanation for the lack of drug effect could be that tianeptine (10 mg/kg twice daily for 14 days) significantly reduced both the expression of serotonin transporter mRNA and serotonin transporter binding sites in the DR, but not in the median raphe nucleus (Kuroda, Watanabe, & McEwen, Citation1994).
Effects of MS, chronic unpredictable stress and tianeptine administration on fos-5HT immunoreactive neurons in the DR subdivisions
The DR is the main source of 5HT to limbic areas involved in depression, but what is the functional implication of its activation? We aimed to ask that question through the study of its subdivisions. 5HT neurons of distinct subdivisions of the DR regulate anxiety-related responses and are differently activated by stress exposure (Crawford, Craige, & Beck, Citation2010; Hale et al., Citation2012; Waselus et al., Citation2011), so it is important to understand the mechanisms through which subdivisions of the DR could be independently regulated. Regardless of the treatment, at −8.04 mm from bregma, the population of 5HT neurons was mostly activated in the DRL/VLPAG and DRD, although more Fos-5HT-labeled neurons were located in the DRL/VLPAG than in the DRD.
Deakin and Graeff et al. proposed a model of the dual role of 5HT in the control of anxiety and panic (Graeff, Guimaraes, De Andrade, & Deakin, Citation1996) in which 5HT neurons terminating in the DRL/VLPAG region serve to inhibit panic-like responses, while 5HT released in the amygdala serves to increase anxiety-like behavior and increase highly integrated defensive behaviors. Specifically, the DRL/VLPAG subdivision - referred to as the lateral wings of the DR - appears to be part of a sympathomotor control system that regulates the fight-or-flight response or other emotional behaviors involving changes in muscle tone, such as freezing (Hale & Lowry, Citation2011). Its activation seems to be related to the inhibition of panic-like physiological and behavioral responses, under conditions associated with antidepressant-like effects on behavior (Hale et al., Citation2012). Although we have not included behavioral analysis in this work, in a previous study conducted using rats from the same litters (Pollano et al., Citation2016), MS-chronically stressed rats displayed a higher percentage of freezing compared with non-MS-chronically stressed rats, as well as greater activation of 5HT neurons in the DRL/VLPAG suggesting a possible association between these two variables. DRL serotonergic neurons also provide a tonic inhibitory input to DRV serotonergic neurons (Hale et al., Citation2012; Hollis, Evans, Bruce, Lightman, & Lowry, Citation2006; Johnson, Lowry, Truitt, & Shekhar, Citation2008), which could explain the results obtained regarding the DRV subdivision. Electrophysiology experiments demonstrated that DRL/VLPAG 5HT neurons possess distinctive membrane properties that make these neurons more excitable than DRV 5HT neurons (Crawford et al., Citation2010).
On the other hand, DRD serotonergic neurons have reciprocal projections to anxiety-related structures, such as the medial prefrontal cortex and related limbic structures, including the basolateral and central amygdaloid nuclei, BNST, nucleus accumbens, dorsal hypothalamic area, and dorsolateral periaqueductal gray (Hale et al., Citation2012). Like the anxiogenic stimulus of the conditioned fear test in all rats in our work, other anxiogenic stimuli have been found to increase c-Fos expression, including anxiogenic drugs such as caffeine (Abrams, Johnson, Hollis, & Lowry, Citation2004), and a member of the CRF family of neuropeptides with high affinity for the CRF2 receptor, urocortin II (Hale & Lowry, Citation2011; Staub et al., Citation2005), and exposure to social defeat (Gardner, Thrivikraman, Lightman, Plotsky, & Lowry, Citation2005). Interestingly, DRD activation was similar in MS-chronically stressed and in controls, suggesting an adaptive phenomenon.
At −6.96 mm from bregma, the population of 5HT neurons is situated only in the DRD subdivision () of the DR, which extends from approximately −6.84 to −8.30 mm from bregma (Paxinos & Watson, Citation2007). Analyzing the Fos-5HT immunoreactivity, there was an interaction between the effects of MS and the drug. In non-maternally separated rats, the antidepressant decreased the number of positive neurons for Fos and 5HT, whereas in maternally separated rats, its effect was similar to that in vehicle-treated rats. As discussed above, the DRD is activated under anxiogenic stimulus. Employing the open field anxiety test, Bouwknecht et al. (Citation2007) detected a greater activation of rostral neurons of the DRD region. Inhibition of 5HT DRD neurons by drug microinjection decreases anxious behavior previously augmented by inescapable stress (Maier & Watkins, Citation2005). According to the results of our study at this level, tianeptine decreases the activation of these neurons in non-maternally separated rats (both AFR and chronically stressed), and could promote anxiolytic behaviors in these groups, as another study from our laboratory has reported using the open field anxiety test (Trujillo et al., Citation2016).
IMPLICATIONS Some theories about depression postulate that the effects of stress are cumulative and, therefore, aversive experiences in early life predispose the individual to be more vulnerable to adverse challenges in adulthood. Our findings provide experimental evidence that MS prepared subjects submitted to chronic unpredictable stress to have a pattern of activated 5HT neurons in the DR, similar to those of control rats. This suggests that the outcome of developmental stress exposure largely depends on environmental conditions later in life and vice versa. Thus, this work contributes to the literature regarding adaptive programing as a consequence of an early stress.
Acknowledgments
We are grateful to Servier Laboratories S.A. for kindly providing tianeptine sodium salt.
Disclosure statement
This research was supported by the National University of Córdoba (SECyT Grants 313/16). Doctoral Fellowship SECyT PhD, Faculty of Exact, Physical and Natural Sciences, National University of Córdoba, Argentina. The authors report no conflicts of interest and alone are responsible for the content and writing of this article and the funding sources had no influence in the content of this article.
Additional information
Funding
References
- Abrams, J.K., Johnson, P.L., Hollis, J.H., & Lowry, C.A. (2004). Anatomic and functional topography of the dorsal raphe nucleus. Annals of the New York Academy of Sciences, 1018, 46–57. doi:10.1196/annals.1296.005
- Ahmad, A., Rasheed, N., Banu, N., & Palit, G. (2010). Alterations in monoamine levels and oxidative systems in frontal cortex, striatum, and hippocampus of the rat brain during chronic unpredictable stress. Stress, 13, 355–364. doi:10.3109/10253891003667862
- Altieri, S.C., Garcia-Garcia, A.L., Leonardo, E.D., & Andrews, A.M. (2013). Rethinking 5-HT1A receptors: Emerging modes of inhibitory feedback of relevance to emotion-related behavior. ACS Chemical Neuroscience, 4, 72–83. doi:10.1021/cn3002174
- Andersen, S.L. (2015). Exposure to early adversity: Points of cross-species translation that can lead to improved understanding of depression. Development and Psychopathology, 27, 477–491. doi:10.1017/S0954579415000103
- Bambico, F.R., Nguyen, N.T., & Gobbi, G. (2009). Decline in serotonergic firing activity and desensitization of 5-HT1A autoreceptors after chronic unpredictable stress. European Neuropsychopharmacology, 19, 215–228. doi:10.1016/j.euroneuro.2008.11.005
- Belzung, C., Willner, P., & Philippot, P. (2015). Depression: From psychopathology to pathophysiology. Current Opinion in Neurobiology, 30, 24–30. doi:10.1016/j.conb.2014.08.013
- Bock, J., Rether, K., Groger, N., Xie, L., & Braun, K. (2014). Perinatal programming of emotional brain circuits: An integrative view from systems to molecules. Frontiers in Neuroscience, 8, 11. doi:10.3389/fnins.2014.00011
- Bouwknecht, J.A., Spiga, F., Staub, D.R., Hale, M.W., Shekhar, A., & Lowry, C.A. (2007). Differential effects of exposure to low-light or high-light open-field on anxiety-related behaviors: Relationship to c-Fos expression in serotonergic and non-serotonergic neurons in the dorsal raphe nucleus. Brain Research Bulletin, 72, 32–43. doi:10.1016/j.brainresbull.2006.12.009
- Bravo, J.A., Dinan, T.G., & Cryan, J.F. (2014). Early-life stress induces persistent alterations in 5-HT1A receptor and serotonin transporter mRNA expression in the adult rat brain. Frontiers in Molecular Neuroscience, 7, 24. doi:10.3389/fnmol.2014.00024
- Brink, C.B., Harvey, B.H., & Brand, L. (2006). Tianeptine: A novel atypical antidepressant that may provide new insights into the biomolecular basis of depression. Recent Patents on Cns Drug Discovery, 1, 29–41. doi:10.2174/157488906775245327
- Castanon, N., Konsman, J.P., Medina, C., Chauvet, N., & Dantzer, R. (2003). Chronic treatment with the antidepressant tianeptine attenuates lipopolysaccharide-induced Fos expression in the rat paraventricular nucleus and HPA axis activation. Psychoneuroendocrinology, 28, 19–34. doi:10.1016/S0306-4530(02)00005-7
- Crawford, L.K., Craige, C.P., & Beck, S.G. (2010). Increased intrinsic excitability of lateral wing serotonin neurons of the dorsal raphe: A mechanism for selective activation in stress circuits. Journal of Neurophysiology, 103, 2652–2663. doi:10.1152/jn.01132.2009
- Chirita, A.L., Gheorman, V., Bondari, D., & Rogoveanu, I. (2015). Current understanding of the neurobiology of major depressive disorder. Romanian Journal of Morphology and Embryology, 56, 651–658.
- Daskalakis, N.P., Oitzl, M.S., Schachinger, H., Champagne, D.L., & de Kloet, E.R. (2012). Testing the cumulative stress and mismatch hypotheses of psychopathology in a rat model of early-life adversity. Physiology & Behavior, 106, 707–721. doi:10.1016/j.physbeh.2012.01.015
- de Kloet, E.R., Joels, M., & Holsboer, F. (2005). Stress and the brain: From adaptation to disease. Nature Reviews. Neuroscience, 6, 463–475. doi:10.1038/nrn1683
- Delbende, C., Contesse, V., Mocaer, E., Kamoun, A., & Vaudry, H. (1991). The novel antidepressant, tianeptine, reduces stress-evoked stimulation of the hypothalamo-pituitary-adrenal axis. European Journal of Pharmacology, 202, 391–396. doi:10.1016/0014-2999(91)90284-W
- Della, F.P., Abelaira, H.M., Reus, G.Z., Santos, M.A., Tomaz, D.B., Antunes, A.R., … Quevedo, J. (2013). Treatment with tianeptine induces antidepressive-like effects and alters the neurotrophin levels, mitochondrial respiratory chain and cycle Krebs enzymes in the brain of maternally deprived adult rats. Metabolic Brain Disease, 28, 93–105. doi:10.1007/s11011-012-9375-x
- Donner, N.C., Siebler, P.H., Johnson, D.T., Villarreal, M.D., Mani, S., Matti, A.J., & Lowry, C.A. (2016). Serotonergic systems in the balance: CRHR1 and CRHR2 differentially control stress-induced serotonin synthesis. Psychoneuroendocrinology, 63, 178–190. doi:10.1016/j.psyneuen.2015.09.024
- Dragunow, M., & Faull, R. (1989). The use of c-fos as a metabolic marker in neuronal pathway tracing. Journal of Neuroscience Methods, 29, 261–265. doi:10.1016/0165-0270(89)90150-7
- Gardner, K.L., Thrivikraman, K.V., Lightman, S.L., Plotsky, P.M., & Lowry, C.A. (2005). Early life experience alters behavior during social defeat: Focus on serotonergic systems. Neuroscience, 136, 181–191. doi:10.1016/j.neuroscience.2005.07.042
- Gartside, S.E., Johnson, D.A., Leitch, M.M., Troakes, C., & Ingram, C.D. (2003). Early life adversity programs changes in central 5-HT neuronal function in adulthood. The European Journal of Neuroscience, 17, 2401–2408. doi:10.1046/j.1460-9568.2003.02668.x
- Gassaway, M.M., Rives, M.L., Kruegel, A.C., Javitch, J.A., & Sames, D. (2014). The atypical antidepressant and neurorestorative agent tianeptine is a mu-opioid receptor agonist. Translational Psychiatry, 4, e411. doi:10.1038/tp.2014.30
- Goel, N., Innala, L., & Viau, V. (2014). Sex differences in serotonin (5-HT) 1A receptor regulation of HPA axis and dorsal raphe responses to acute restraint. Psychoneuroendocrinology, 40, 232–241. doi:10.1016/j.psyneuen.2013.11.020
- Graeff, F.G., Guimaraes, F.S., De Andrade, T.G., & Deakin, J.F. (1996). Role of 5-HT in stress, anxiety, and depression. Pharmacology, Biochemistry, and Behavior, 54, 129–141. doi:10.1016/0091-3057(95)02135-3
- Griebel, G., Simiand, J., Steinberg, R., Jung, M., Gully, D., Roger, P., … Soubrie, P. (2002). 4-(2-Chloro-4-methoxy-5-methylphenyl)-N-[(1S)-2-cyclopropyl-1-(3-fluoro-4-methylp henyl)ethyl]5-methyl-N-(2-propynyl)-1, 3-thiazol-2-amine hydrochloride (SSR125543A), a potent and selective corticotrophin-releasing factor(1) receptor antagonist. II. Characterization in rodent models of stress-related disorders. Journal of Pharmacology and Experimental Therapeutics, 301, 333–345. doi:10.1124/jpet.301.1.333
- Gupta, D., Radhakrishnan, M., & Kurhe, Y. (2014). 5HT3 receptor antagonist (ondansetron) reverses depressive behavior evoked by chronic unpredictable stress in mice: Modulation of hypothalamic-pituitary-adrenocortical and brain serotonergic system. Pharmacology Biochemistry and Behavior, 124, 129–136. doi:10.1016/j.pbb.2014.05.024
- Haase, J., & Brown, E. (2015). Integrating the monoamine, neurotrophin and cytokine hypotheses of depression-a central role for the serotonin transporter? Pharmacology: Therapeutics, 147, 1–11. doi:10.1016/j.pharmthera.2014.10.002
- Hale, M.W., & Lowry, C.A. (2011). Functional topography of midbrain and pontine serotonergic systems: Implications for synaptic regulation of serotonergic circuits. Psychopharmacology (Berl), 213, 243–264. doi:10.1007/s00213-010-2089-z
- Hale, M.W., Shekhar, A., & Lowry, C.A. (2012). Stress-related serotonergic systems: Implications for symptomatology of anxiety and affective disorders. Cellular and Molecular Neurobiology, 32, 695–708. doi:10.1007/s10571-012-9827-1
- Hennessy, M.B., Kaiser, S., Tiedtke, T., & Sachser, N. (2015). Stability and change: Stress responses and the shaping of behavioral phenotypes over the life span. Frontiers in Zoology, 12, S18. doi:10.1186/1742-9994-12-S1-S18
- Herman, J.P., Ostrander, M.M., Mueller, N.K., & Figueiredo, H. (2005). Limbic system mechanisms of stress regulation: Hypothalamo-pituitary-adrenocortical axis. Progress in Neuro-Psychopharmacology & Biological Psychiatry, 29, 1201–1213. doi:10.1016/j.pnpbp.2005.08.006
- Hollis, J.H., Evans, A.K., Bruce, K.P., Lightman, S.L., & Lowry, C.A. (2006). Lipopolysaccharide has indomethacin-sensitive actions on Fos expression in topographically organized subpopulations of serotonergic neurons. Brain, Behavior, and Immunity, 20, 569–577. doi:10.1016/j.bbi.2006.01.006
- Johnson, P., Lowry, C., Truitt, W., & Shekhar, A. (2008). Disruption of GABAergic tone in the dorsomedial hypothalamus attenuates responses in a subset of serotonergic neurons in the dorsal raphe nucleus following lactate-induced panic. Journal of Psychopharmacology, 22, 642–652. doi:10.1177/0269881107082900
- Kim, S.J., Park, S.H., Choi, S.H., Moon, B.H., Lee, K.J., Kang, S.W., … Shin, K.H. (2006). Effects of repeated tianeptine treatment on CRF mRNA expression in non-stressed and chronic mild stress-exposed rats. Neuropharmacology, 50, 824–833. doi:10.1016/j.neuropharm.2005.12.003
- Kim, Y.J., Shin, M.C., Kim, S.A., Chung, J.H., Kim, E.H., & Kim, C.J. (2002). Modulation of tianeptine on ion currents induced by inhibitory neurotransmitters in acutely dissociated dorsal raphe neurons of Sprague-Dawley rats. European Neuropsychopharmacology, 12, 417–425. doi:10.1016/S0924-977X(02)00054-8
- Korosi, A., Veening, J.G., Kozicz, T., Henckens, M., Dederen, J., Groenink, L., … Roubos, E.W. (2006). Distribution and expression of CRF receptor 1 and 2 mRNAs in the CRF over-expressing mouse brain. Brain Research, 1072, 46–54. doi:10.1016/j.brainres.2005.12.034
- Kuroda, Y., Watanabe, Y., & McEwen, B.S. (1994). Tianeptine decreases both serotonin transporter mRNA and binding sites in rat brain. European Journal of Pharmacology, 268, R3–R5. doi:10.1016/0922-4106(94)90127-9
- Lajud, N., Roque, A., Cajero, M., Gutierrez-Ospina, G., & Torner, L. (2012). Periodic maternal separation decreases hippocampal neurogenesis without affecting basal corticosterone during the stress hyporesponsive period, but alters HPA axis and coping behavior in adulthood. Psychoneuroendocrinology, 37, 410–420. doi:10.1016/j.psyneuen.2011.07.011
- Lin, Y.E., Lin, S.H., Chen, W.C., Ho, C.T., Lai, Y.S., Panyod, S., & Sheen, L.Y. (2016). Antidepressant-like effects of water extract of Gastrodia elata Blume in rats exposed to unpredictable chronic mild stress via modulation of monoamine regulatory pathways. Journal of Ethnopharmacology, 187, 57–65. doi:10.1016/j.jep.2016.04.032
- Maier, S.F., & Watkins, L.R. (2005). Stressor controllability and learned helplessness: The roles of the dorsal raphe nucleus, serotonin, and corticotropin-releasing factor. Neuroscience & Biobehavioral Reviews, 29, 829–841. doi:10.1016/j.neubiorev.2005.03.021
- McEwen, B.S., Eiland, L., Hunter, R.G., & Miller, M.M. (2012). Stress and anxiety: structural plasticity and epigenetic regulation as a consequence of stress. Neuropharmacology, 62, 3–12. doi:10.1016/j.neuropharm.2011.07.014
- McEwen, B.S., & Gianaros, P.J. (2011). Stress- and allostasis-induced brain plasticity. Annual Review of Medicine, 62, 431–445. doi:10.1146/annurev-med-052209-100430
- Meloni, E.G., Reedy, C.L., Cohen, B.M., & Carlezon, W.A. Jr. (2008). Activation of raphe efferents to the medial prefrontal cortex by corticotropin-releasing factor: Correlation with anxiety-like behavior. Biological Psychiatry, 63, 832–839. doi:10.1016/j.biopsych.2007.10.016
- Michelsen, K.A., Prickaerts, J., & Steinbusch, H.W. (2008). The dorsal raphe nucleus and serotonin: Implications for neuroplasticity linked to major depression and Alzheimer's disease. Progress in Brain Research, 172, 233–264. doi:10.1016/S0079-6123(08)00912-6
- Monroe, S.M., & Simons, A.D. (1991). Diathesis-stress theories in the context of life stress research: Implications for the depressive disorders. Psychological Bulletin, 110, 406–425. doi:10.1037/0033-2909.110.3.406
- Nishi, M., Horii-Hayashi, N., & Sasagawa, T. (2014). Effects of early life adverse experiences on the brain: Implications from maternal separation models in rodents. Frontiers in Neuroscience, 8, 166. doi:10.3389/fnins.2014.00166
- Ogawa, T., Mikuni, M., Kuroda, Y., Muneoka, K., Mori, K.J., & Takahashi, K. (1994). Periodic maternal deprivation alters stress response in adult offspring: potentiates the negative feedback regulation of restraint stress-induced adrenocortical response and reduces the frequencies of open field-induced behaviors. Pharmacology, Biochemistry, and Behavior, 49, 961–967. doi:10.1016/0091-3057(94)90250-X
- Ohta, K., Miki, T., Warita, K., Suzuki, S., Kusaka, T., Yakura, T., … Takeuchi, Y. (2014). Prolonged maternal separation disturbs the serotonergic system during early brain development. International Journal of Developmental Neuroscience, 33, 15–21. doi:10.1016/j.ijdevneu.2013.10.007
- Oosterhof, C.A., El Mansari, M., Merali, Z., & Blier, P. (2016). Altered monoamine system activities after prenatal and adult stress: A role for stress resilience? Brain Research, 1642, 409–418. doi:10.1016/j.brainres.2016.04.032
- Own, L.S., Iqbal, R., & Patel, P.D. (2013). Maternal separation alters serotonergic and HPA axis gene expression independent of separation duration in mice. Brain Research, 1515, 29–38. doi:10.1016/j.brainres.2013.03.032
- Pariante, C.M., & Miller, A.H. (2001). Glucocorticoid receptors in major depression: Relevance to pathophysiology and treatment. Biological Psychiatry, 49, 391–404. doi:10.1016/S0006-3223(00)01088-X
- Paxinos, G., & Watson, C. (2007). The Rat Brain in Stereotaxic Coordinates (6th ed). San Diego: Elsevier Academic Pess.
- Pineyro, G., Deveault, L., de Montigny, C., & Blier, P. (1995). Effect of prolonged administration of tianeptine on 5-HT neurotransmission: An electrophysiological study in the rat hippocampus and dorsal raphe. Naunyn-Schmiedeberg's Archives of Pharmacology, 351, 119–125. doi:10.1007/BF00169325
- Pollano, A., Zalosnik, M.I., Durando, P.E., & Suarez, M.M. (2016). Differential effects of tianeptine on the dorsal hippocampal volume of rats submitted to maternal separation followed by chronic unpredictable stress in adulthood. Stress, 19, 599–608. doi:10.1080/10253890.2016.1224842
- Raftogianni, A., Diamantopoulou, A., Alikaridis, F., Stamatakis, A., & Stylianopoulou, F. (2012). Effects of interaction of an early experience of reward through maternal contact or its denial with social stress during adolescence on the serotonergic system and the stress responsiveness of adult female rats. Neuroscience, 209, 84–96. doi:10.1016/j.neuroscience.2012.01.032
- Roy, A., & Campbell, M.K. (2013). A unifying framework for depression: Bridging the major biological and psychosocial theories through stress. Clinical & Investigative Medicine, 36, E170–E190.
- Santarelli, S., Zimmermann, C., Kalideris, G., Lesuis, S.L., Arloth, J., Uribe, A., … Schmidt, M.V. (2017). An adverse early life environment can enhance stress resilience in adulthood. Psychoneuroendocrinology, 78, 213–221. doi:10.1016/j.psyneuen.2017.01.021
- Schmidt, M.V. (2011). Animal models for depression and the mismatch hypothesis of disease. Psychoneuroendocrinology, 36, 330–338. doi:10.1016/j.psyneuen.2010.07.001
- Staub, D.R., Spiga, F., & Lowry, C.A. (2005). Urocortin 2 increases c-Fos expression in topographically organized subpopulations of serotonergic neurons in the rat dorsal raphe nucleus. Brain Research, 1044, 176–189. doi:10.1016/j.brainres.2005.02.080
- Suarez, M., Paglini, P., Fernandez, R., Enders, J., Maglianesi, M., Perassi, N., & Palma, J. (1999). Influence of anterodorsal thalamic nuclei on the hypophyseal-adrenal axis and cardiac beta receptors in rats submitted to variable chronic stress. Acta Physiologica, Pharmacologica Et Therapeutica Latinoamericana: Organo De La Asociacion Latinoamericana De Ciencias Fisiologicas y [De] La Asociacion Latinoamericana De Farmacologia, 49, 71–78.
- Tanahashi, S., Yamamura, S., Nakagawa, M., Motomura, E., & Okada, M. (2012). Effect of lamotrigine and carbamazepine on corticotropin-releasing factor-associated serotonergic transmission in rat dorsal raphe nucleus. Psychopharmacology (Berl), 220, 599–610. doi:10.1007/s00213-011-2506-y
- Trujillo, V., Durando, P.E., & Suarez, M.M. (2016). Maternal separation in early life modifies anxious behavior and Fos and glucocorticoid receptor expression in limbic neurons after chronic stress in rats: Effects of tianeptine. Stress, 19, 91–103. doi:10.3109/10253890.2015.1105958
- Trujillo, V., Masseroni, M.L., Levin, G., & Suárez, M.M. (2009). Tianeptine influence on plasmatic catecholamine levels and anxiety index in rats under variable chronic stress after early maternal separation. The International Journal of Neuroscience, 119, 1210–1227. doi:10.1080/00207450802324713
- Van den Hove, D.L., Leibold, N.K., Strackx, E., Martinez-Claros, M., Lesch, K.P., Steinbusch, H.W., … Prickaerts, J. (2014). Prenatal stress and subsequent exposure to chronic mild stress in rats; interdependent effects on emotional behavior and the serotonergic system. European Neuropsychopharmacology, 24, 595–607. doi:10.1016/j.euroneuro.2013.09.006
- Vetulani, J. (2013). Early maternal separation: a rodent model of depression and a prevailing human condition. Pharmacological Reports, 65, 1451–1461. doi:10.1016/S1734-1140(13)71505-6
- Vicentic, A., Francis, D., Moffett, M., Lakatos, A., Rogge, G., Hubert, G.W., … Kuhar, M.J. (2006). Maternal separation alters serotonergic transporter densities and serotonergic 1A receptors in rat brain. Neuroscience, 140, 355–365. doi:10.1016/j.neuroscience.2006.02.008
- Waselus, M., Nazzaro, C., Valentino, R.J., & Van Bockstaele, E.J. (2009). Stress-induced redistribution of corticotropin-releasing factor receptor subtypes in the dorsal raphe nucleus. Biological Psychiatry, 66, 76–83. doi:10.1016/j.biopsych.2009.02.014
- Waselus, M., Valentino, R.J., & Van Bockstaele, E.J. (2011). Collateralized dorsal raphe nucleus projections: A mechanism for the integration of diverse functions during stress. Journal of Chemical Neuroanatomy, 41, 266–280. doi:10.1016/j.jchemneu.2011.05.011
- Willner, P. (2005). Chronic mild stress (CMS) revisited: Consistency and behavioural-neurobiological concordance in the effects of CMS. Neuropsychobiology, 52, 90–110. doi:10.1159/000087097
- Willner, P., Scheel-Kruger, J., & Belzung, C. (2013). The neurobiology of depression and antidepressant action. Neuroscience and Biobehavioral Reviews, 37, 2331–2371. doi:10.1016/j.neubiorev.2012.12.007
- Wong, M.L., Kling, M.A., Munson, P.J., Listwak, S., Licinio, J., Prolo, P., … Gold, P.W. (2000). Pronounced and sustained central hypernoradrenergic function in major depression with melancholic features: Relation to hypercortisolism and corticotropin-releasing hormone. Proceedings of the National Academy of Sciences of the United States of America, 97, 325–330. doi:10.3389/fnbeh.2015.00094
- Xue, X., Shao, S., Wang, W., & Shao, F. (2013). Maternal separation induces alterations in reversal learning and brain-derived neurotrophic factor expression in adult rats. Neuropsychobiology, 68, 243–249. doi:10.1016/j.brainresbull.2013.03.003