Abstract
Being chased by a predator or a dominant conspecific can induce significant stress. However, only a limited number of laboratory studies have employed chasing by itself as a stressor. In this study, we developed a novel stress paradigm in which rats were chased by a fast-moving object in an inescapable maze. In Experiment 1, defensive behaviors and stress hormone changes induced by chasing stress were measured. During the chasing stress, the chasing-stress group (n = 9) froze and emitted 22-kHz ultrasonic vocalizations (USVs), but the no-chasing control group (n = 10) did not. Plasma corticosterone levels significantly increased following the chasing and were comparable to those of the restraint-stress group (n = 6). In Experiment 2, the long-lasting memory of the chasing event was tested after three weeks. The chasing-stress group (n = 15) showed higher levels of freezing and USV than the no-chasing group (n = 14) when they were presented with the tone associated with the object’s chasing action. Subsequently, the rats were subjected to Pavlovian threat conditioning with a tone as a conditioned stimulus and footshock as an unconditioned stimulus. The chasing-stress group showed higher levels of freezing and USV during the conditioning session than the no-chasing group, indicating sensitized defensive reactions in a different threat situation. Taken together, the current results suggest that chasing stress can induce long-lasting memory and sensitization of defensive responses to a new aversive event as well as immediate, significant stress responses.
1. Introduction
Being chased by a predator or a dominant conspecific is a major psychogenic stressor for most animals and even for modern-day humans (Hansen et al., Citation2006). However, repeated chasing as a psychogenic stressor has not been systematically studied, perhaps due to the associated methodological difficulties. Most studies conducted on the effects of chasing have relied on uncontrolled field observations occurring during the capture of wild animals. For example, small animals such as white-crowned sparrows, lizards, and snakes displayed strong signs of stress including increased plasma corticosterone levels and decreased plasma testosterone levels following capture by a human experimenter (Moore, Lemaster, & Mason, Citation2000; Wilson & Wingfield, Citation1992; Wingfield, Smith, & Farner, Citation1982). Another field study observed that deer herds living inside a wide cattle ranch area also displayed intense fight-or-flight responses when chased by coyote packs (Lingle & Pellis, Citation2002). However, most field studies of chasing stress have provided limited insight regarding the effects of chasing stress, as the capture procedure involved multiple stressors including forced restraint and radical changes in housing conditions.
Alternatively, chasing can be staged in a laboratory setting using a more controlled protocol in which an artificial threat object is employed. For example, a fast-surging robot with the size and appearance of a natural predator produced an immediate escape response in rats together with defensive reactions such as freezing and ultrasonic vocalization (USV), which are typically observed when rats confront natural predators (Choi & Kim, Citation2010). In addition, a car-like moving robot was used to produce learned avoidance by applying an electric shock when the rat was within a particular distance from the robot (Telensky et al., Citation2011) or to challenge the rat to find an escape route based on previous exploratory experience (Renner, Citation1988). However, these studies mainly focused on immediate defensive responses to the robot or the moving object with little emphasis on the systematic investigation of stress responses.
Here, we developed a chasing-stress paradigm in which rats were repeatedly chased by a sizable object moving at a high speed in an inescapable, donut-shaped maze. To validate the effectiveness of chasing stress, behavioral and endocrine changes following the stress were characterized. In Experiment 1 (Exp. 1), defensive responses during chasing stress and plasma corticosterone (CORT) levels immediately after the stress were measured. In Experiment 2 (Exp. 2), the long-term effects of chasing stress on the persistence of fear memory, anxiety-related behavior, and sensitized responses to a different aversive situation were investigated after three weeks. This would be the first step toward investigating the effects of chasing as a form of psychogenic stress under precisely-controlled laboratory conditions.
2. Materials and methods
2.1. Subjects
Fifty-four adult (8 weeks old) male Sprague Dawley rats were used in this study. Thirty-seven of these animals were obtained from Orient Bio, Korea, and 17 were bred in our laboratory. The rats used for breeding in our laboratory were originally from the Orient Bio. In the Exp. 1, all rats were bought from the Orient Bio. In the Exp. 2, 6 out of 14 in the no-chasing group and 6 out of 15 in the chasing-stress group were bought from the Orient Bio. The rest were from our laboratory. As there were no significant statistical differences in the data from both breeders, the data were combined. Rats were group-housed (three/cage) and maintained in 12-h/12-h light/dark cycle at a constant temperature (21–23 °C) and humidity (50–60%). Rats were allowed ad libitum access to food and water. All experimental procedures were approved by the Korea University Institutional Animal Care and Use Committee.
2.2. Apparatus
2.2.1. Donut maze
The apparatus was an acrylic donut-shaped maze (60-cm outer diameter) with a circular track (18 cm in width) and a high wall (45 cm), and the wall was covered by black polyvinyl chloride foam sheets (). The maze was surrounded by a black curtain and illuminated with indirect halogen lighting. Three video cameras were placed inside the transparent cylinder located in the center of the maze. Additionally, a video camera and webcam were mounted on the ceiling. All five cameras were used for monitoring and recording the activity of the rats.
2.2.2. Chasing robot
The chasing robot was a box-shaped car (15 × 26 × 35 cm) with four high-traction wheels controlled by a Bluetooth®-based microcontroller (SPL-Duino BT, Helloapps, Korea). Two 8-ohm speakers were attached to the front and back of the robot through which a tone (2 kHz) was played. The robot was programmed to move at a specific speed and in different directions using custom-written software (Arduino, USA). In addition, the protocol of the chasing was included in the program. When an initiation command was given by the experimenter via Bluetooth® communication, the robot automatically started to move. The direction of the movement (clockwise/counterclockwise) was randomly decided with a 50/50 chance.
2.3. Behavioral procedure
2.3.1. Exp. 1: behavioral and endocrine responses to chasing stress within a session
In Exp. 1, the effectiveness of chasing stress was examined by measuring the immediate behavioral and endocrine responses: freezing, 22-kHz USV, and plasma CORT levels ().
Chasing stress. The chasing-stress (n = 9) and the no-chasing groups (n = 10) were habituated to the apparatus for two days. On the third day, the chasing-stress group underwent 60 trials of being chased by a fast-approaching (0.7 m/s) robot for approximately 25 min. Each trial consisted of 2.5 s of chasing (clockwise/counterclockwise randomly mixed) and 15–25 s of the inter-trial interval (ITI). The no-chasing group was exposed to the stationary robot for 25 min. Freezing and USV were measured during this stage (Supplemental movie 1). Immediately after chasing stress, blood samples were collected. The maze was cleaned with a 70% ethanol solution at the end of every session.
Restraint stress. The restraint-stress group (n = 6) was restrained in a transparent cylinder made of acryl (8 cm diameter, 20 cm high) for 30 min. Immediately after the restraint stress, blood samples were collected. At the termination of each behavioral session, the restrainer was cleaned with a 70% ethanol solution.
CORT immunoassay. Blood samples were collected from the lateral tail vein of the rats under isoflurane anesthesia during the early light phase. The early light phase, which was 7 pm to 9 pm in our laboratory, was approximately two hours after the light was turned on. The chasing procedure took 25 min and the blood sampling procedure including anesthesia took under 10 min. Thus, only two or three rats were subjected each day. All of the pre-treatment blood samples for baseline measurement were collected on the third day of handling, and the post-treatment blood samples were collected immediately after the treatments (no chasing; chasing; and restraint). The samples were initially centrifuged (3000 rpm, 10 min) to extract blood plasma, prior to centrifuging again (3000 rpm, 5 min) to remove any remaining blood clot. The final samples were stored at −20 °C until the assay was performed. Plasma CORT concentrations were measured using Corticosterone ELISA kits (DEV9922, Demeditec Diagnostics, Germany) and adhering to the procedures suggested by the manufacturer.
Behavioral analyses. To investigate the dynamics of passive and active reactions to chasing stress, two categories of defensive behaviors were monitored: freezing and USV (passive) and avoidance and escape (active) (). For every trial, the occurrence of each defensive behavior was counted and manually coded for later analyses (1 or 0) by monitoring video recordings. To identify the patterns of defensive response modulation, three trials were combined into a single block, generating a total of 20 blocks. We mainly focused our analyses on blocks 6–20 as the initial blocks were dominated by Pavlovian components including monotonic increases in conditioned freezing and USV (Young & Fanselow, Citation1992). Freezing was defined as the cessation of all movements except for those related to respiration. USVs were detected by a bat detector (Mini-3 Detector, Ultra Sound Advice, UK) tuned at 22 kHz. Avoidance is defined as the directional movement toward the opposite side of the robot in advance while the robot was stationary (during ITI). Escape is defined as the rapid movement away from the moving robot to prevent the contact with the robot (during the chasing).
2.3.2. Exp. 2: long-term effects of chasing stress
In Exp. 2, long-term effects of chasing stress were investigated. Three weeks following the chasing, the memory of the stress was tested by presenting a cue (a 2-kHz tone) that had been associated with the chasing action. In addition, anxiety-related behavior was measured using the elevated plus maze test. Finally, fear responses to a new aversive event were analyzed by conducting Pavlovian threat conditioning (PTC) and retention tests for PTC ().
Chasing stress. The chasing-stress group (n = 15) received similar chasing stress as described in Exp. 1, except for some procedural variations. Each trial consisted of the presentation of a chasing tone (2 kHz, 5 s) followed by chasing stress (2.5 s) co-terminating with the tone. Additionally, there were three stress sessions instead of one (20 trials per session, a total of 60 trials). The no-chasing group (n = 14) were subjected to the same conditions without chasing (tone only).
Chasing tone tests. Two different contexts were used to test the memory of chasing stress: the original (donut maze) and a different (a rectangular Plexiglas box with blue illumination and a flat floor) context. In the original context, following an acclimation period of 2 min, rats received three presentations of the chasing tone (2 kHz) that had been presented by the chasing robot, separated by 20-s ITIs. On the day following elevated plus maze test, they received five presentations of the chasing tone for 20 s separated by 60-s ITIs, following a 2-min acclimation in the different context. Freezing and USVs were measured during the tests. The maze or chamber was cleaned with a 70% ethanol solution at the end of every session.
Elevated plus maze test. The elevated plus maze consisted of two open and two closed arms (10 × 50 cm). The closed arms were lined with high walls (40 cm). The maze was elevated 50 cm above the floor. The open arms were illuminated with LED lighting (33 lux). At the beginning of the test session, rats were placed in the central area of the maze with their heads oriented toward an open arm. After 10 min of exploration, the rats were returned to their home cages. The number of entries to and the time spent in the open arms were analyzed by tracking the head of the animal using a video-tracking system (ANY-maze, Stoelting, Wood Dale, IL). The maze was cleaned with a 70% ethanol solution at the end of every session.
Pavlovian threat conditioning. Two identical Plexiglas boxes (25 × 30 × 40 cm), illuminated by red lights, were used for PTC. Each box was floored with a grid composed of 16 stainless steel rods (0.5 cm in diameter, 1.5 cm apart) connected to a scrambled shock generator (Precision Animal Shocker, Coulbourn Instruments, Whitehall, PA). To present a tone (4 kHz, 75–80 dB, 20 s) as a conditioned stimulus (CS), a tone generator (Programmable Tone/Noise Generator, Coulbourn Instruments, Whitehall, PA) was connected to a small speaker attached to the side wall of each box through an amplifier (CPA-200, Vascom, Korea). A footshock (0.6 mA, 1 s) as an unconditioned stimulus (US) was delivered through the grid floor. During tone habituation, rats received two presentations of CS with 110–130 s of ITIs (120 s on average) after 2 min of acclimation. On the following day, rats underwent PTC in which three pairings of CS and US were given after 2 min of acclimation. In each trial, the final 1 s of CS was concurrent with the US. ITI was 110–130 s (120 s on average). Freezing and USVs during tone habituation and PTC were measured. The chamber and grid were cleaned with a 70% ethanol solution at the end of every session.
Retention tests for PTC. Following PTC, retention tests for the context and tone used during PTC were conducted the next day. During the PTC context retention test, rats were placed in the same Plexiglas box used during PTC for 5 min. Three hours later, rats underwent the PTC tone retention test in a different context (green light and a flat floor covered with bedding materials). After a 2-min acclimation period, rats were presented with two CSs (20 s) separated by 110–130 s of ITI. Freezing and USVs during the PTC retention tests were measured. The chamber and grid (or the flat floor) were cleaned with a 70% ethanol solution at the end of every session.
Analyses of freezing and USV. Video recordings were analyzed using a video-tracking system (ANY-maze, Stoelting, Wood Dale, IL) to measure freezing duration. USVs from a bat detector (Mini-3 Detector, Ultra Sound Advice, UK), which was tuned to 22 kHz, were recorded on the audio track of the video recording. The background noise and mechanical noise created by the motor in the robot were reduced with a digital filter using a sound analysis program (Adobe Audition, Adobe Systems, San Jose, CA). Finally, the duration of USV and number of USV calls were analyzed by an in-house program written in MATLAB (MathWorks, Natick, MA, ).
Figure 2. Behavioral and endocrinal responses to chasing in Exp. 1. (A) Freezing and ultrasonic vocalization (USV) responses during the chasing-stress treatment. (B) Changes in plasma corticosterone levels following no chasing, chasing stress, and restraint stress. (C) USVs recorded during the entire training session (approximately 25 min) from all animals used in Exp. 1. Each tick mark indicates one USV call, with a typical duration of 0.5–1.5 s. Note that only one animal in the no-chasing group showed brief bouts of USV compared with all animals in the chasing-stress group. *: p < .05; **: p < .01.
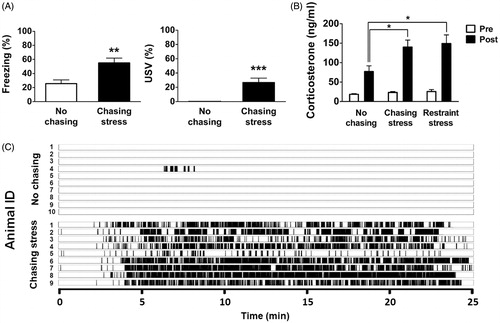
2.4. Statistical analysis
Statistical analyses were performed using a statistics software package (SPSS version 20.0, IBM SPSS, Armonk, NY). To analyze freezing and USV during chasing stress and chasing tone tests, Student’s t tests or Mann–Whitney U tests were conducted. To analyze CORT levels, a two-way repeated measures analysis of variance (ANOVA) with Bonferroni corrections was conducted. The factors were Group (no chasing, chasing stress, and restraint stress) and Time (pre- and post-treatment). In addition, to identify switching between passive and active defensive responses over the course of chasing stress, linear and second-order polynomial analyses were performed on freezing and avoidance responses across blocks 6–20. To analyze the results of the elevated plus maze test, Student’s t-tests were conducted. To analyze freezing and USV during PTC, two-way repeated measures ANOVA with Bonferroni corrections were conducted. The factors were group (no chasing and chasing stress) and trial. To analyze the ratio of the number of animals showing USVs during the chasing tone retention tests and PTC, Fisher’s exact tests were conducted. To analyze changes in freezing and USV before and after the first shock, two-way ANOVA with Bonferroni corrections were conducted. The factors were Group (no chasing and chasing-stress) and Shock (before- and after-shock). To analyze the difference in freezing differentials (Freezing1st ITI – Freezing1st CS) and USV differentials (USV1st ITI – USV1st CS), Student’s t-tests were conducted. To analyze freezing and USV during PTC retention tests, Student’s t-tests or Mann–Whitney U tests were conducted. A p-value of <.05 was considered statistically significant.
3. Results
3.1. Exp. 1: behavioral and endocrine responses to chasing stress within a session
Most animals in the chasing-stress group displayed an immediate increase in passive defensive reactions after the first encounter with chasing stress. As a result, the chasing-stress group showed significantly higher levels of freezing (t (18) = −3.41, p < .01) and USV (U = 90, p < .001) than the no-chasing group within the entire session (). In particular, USV was almost exclusively found in the chasing-stress group as depicted in . The call pattern and duration of the individual bout were similar to those found in previous studies of acute stress in aversive situations (for example, Lee, Choi, Brown, & Kim, Citation2001). These results indicate that chasing can serve as an effective aversive stimulus.
The results of the plasma CORT immunoassay are illustrated in . There were significant main effects of Group (F (2, 22) = 5.53, p < .05) and Time (F (1, 22) = 83.52, p < .001). In addition, there was a significant interaction between Group × Time (F (2, 22) = 4.23, p < .05). Post hoc analyses with Bonferroni correction demonstrated that there were no statistically significant differences in CORT levels before stress. However, after stress, CORT levels of the chasing-stress group and the restraint-stress group were significantly higher than those of the no-chasing group. These analyses indicate that chasing stress can induce a significant neuroendocrine stress response, equivalent to that seen following restraint stress, a stress paradigm well established by previous studies (for example, Radley, Arias, & Sawchenko, Citation2006).
During chasing stress, the rats showed a varying amount of two types of defensive responses, namely, passive (freezing, USV) and active (avoidance, escape) (). The most frequently observed behaviors were freezing and USV (each representing 42% of the entire number of observed defensive responses). The proportion of avoidance was 12% and that of escape was 4% (). After the initial phase of chasing stress (blocks 1–6) during which Pavlovian conditioned responses rapidly and invariably developed, the interesting linear and non-linear trends were observed with respect to the within-session modulation of the passive and active defensive responses. Regression analysis demonstrated that the pattern of freezing modulation between blocks 6–20 was marginally significant for a third-order polynomial curve ( red line, R2 = 0.48, p < .06) but not significant for a linear curve ( black line, R2 = 0.01, p > .05) and for a second-order polynomial curve ( blue line, R2 = 0.28, p > .05). In addition, regression analysis showed that the pattern of avoidance modulation between blocks 6–20 was significant for a linear curve ( black line, R2 = 0.36, p < .05) and for a second-order polynomial curve ( blue line, R2 = 0.46, p < .05) but not significant for a third-order polynomial curve ( red line, R2 = 0.47, p > .05). These analyses suggest that the passive and active defensive responses were developed with opposite patterns.
Figure 3. Modulation of defensive response across trials during the chasing stress. (A) Two categories of defensive responses: passive responses such as freezing and USV and active responses such as avoidance and escape. (B) Proportions of trials showing different types of defensive responses. (C) Modulation of freezing across blocks of three trials. A marginally significant non-linear trend (third-order polynomial curve; red line) was identified between blocks 6–20. (D) Modulation of avoidance across blocks of three trials. Significant linear (black line) and non-linear trends (second-order polynomial curve; blue line) were identified between blocks 6–20.
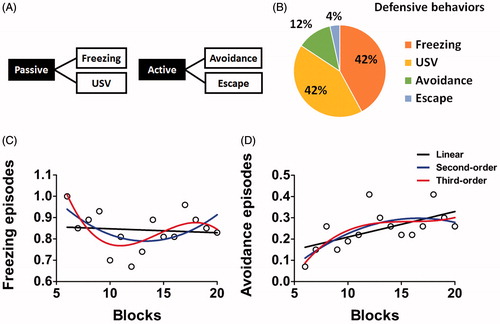
3.2. Exp. 2: long-term effects of chasing stress
The long-term effects of chasing stress were examined by several behavioral tests conducted three weeks after the event: chasing tone tests, elevated plus maze test, PTC, and retention tests for PTC.
3.2.1. Chasing tone tests
To test whether the memory of chasing stress was preserved after an extended period of time, chasing tone tests were conducted in the original and a different context.
During the chasing tone test in the original context, the chasing-stress group showed a significantly higher level of freezing, as revealed by the Mann–Whitney U test (, U = 209.00, p < .001). The amount of USVs produced by the chasing-stress group was not significantly different from that produced by the no-chasing group ( below, U = 147.00, p = .07). However, the number of animals that showed USVs in the chasing-stress group was significantly higher than that in the no-chasing group ( above, 6 out of 15 in the chasing-stress group vs. 0 out of 14 in the no-chasing group; Fisher’s exact test, p < .05). The number of fecal boli was also higher in the chasing-stress group (t (27) = −3.84, p < .01, ).
Figure 4. Defensive responses during the chasing tone tests. (A, D) Amount of freezing during the chasing tone in the donut maze and in a different context. (B, E) Amount of ultrasonic vocalization (USV) during the chasing tone in the donut maze and in a different context. (C, F) Number of fecal boli collected from the donut maze and from a different context. *: p < .05; **: p < .01; ***: p < .001.
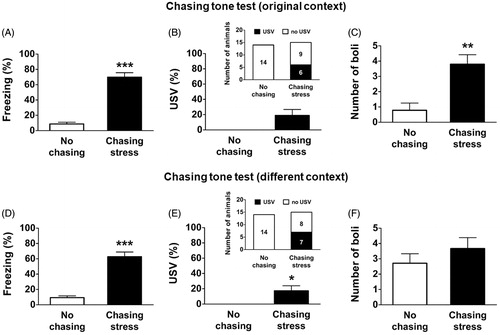
During the chasing tone test in a different context, the chasing-stress group showed a significantly higher level of freezing than the no-chasing group (, U = 203.00, p < .001). In addition, the chasing-stress group showed a significantly higher level of USV ( below, U = 154.00, p < .05). The number of animals that showed USVs in the chasing-stress group was significantly higher than that in the no-chasing group ( above, 7 out of 15 in the chasing-stress group vs. 0 out of 14 in the no-chasing group; Fisher’s exact test, p < .01). There was no significant difference in the number of fecal boli between the two groups (, U = 134.00, p > .05). Taken together, these data indicate that the experience of being chased by the robot was stored as a long-lasting memory, which could be triggered by the cue associated with the event. Furthermore, the animals were in a state of distress when they retrieved the chasing-related memory.
3.2.2. Elevated plus maze test
To assess whether chasing stress increased anxiety-related behavior, rats were placed on the elevated plus maze and allowed to explore the maze for 10 min.
The results of the test are shown in . There were no significant differences in the number of entries to the open arms (, t (27) = −0.83, p > .05) or in the time spent in the open arms (, t (27) = −1.04, p > .05). These data indicate that chasing stress did not alter anxiety-related behavior.
3.2.3. PTC
To test whether chasing stress induced sensitized defensive responses in a different aversive situation, PTC was conducted using a tone CS and footshock US following the habituation session.
During the habituation session, the chasing-stress group showed a significantly higher level of freezing (, t (27) = −2.58, p < .05. In addition, only one rat in the chasing-stress group but none in the no-chasing group emitted USVs (). These results indicate that prior experience of chasing stress can induce generalized defensive responses to CS, perhaps due to its similarity to the chasing tone.
Figure 6. Freezing and ultrasonic vocalization (USV) before and during Pavlovian threat conditioning (PTC). (A, B) Freezing and USV during the habituation session. (C, D) Freezing and USV in response to the tone CS during the conditioning session. (E, F) Freezing and USV during inter-trial interval (ITI). (G, H) Freezing and USV changes before and after the 1st US presentation. *: p < .05; **: p < .01.
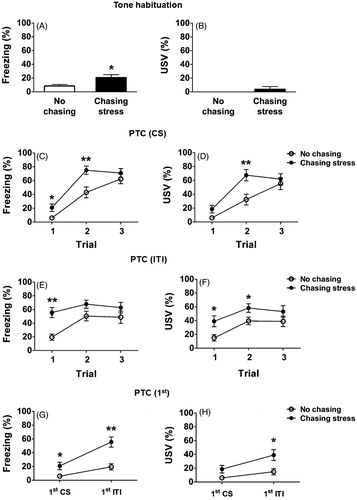
During PTC, rats received three pairings of CS (a 4-kHz tone) and US (footshock). Freezing and USV during the presentations of CS are depicted in . There was a significant main effect of Group (chasing stress vs. no chasing) on the levels of freezing (F (1, 27) = 7.54, p < .05). In addition, there was a significant main effect of Trial (F (2, 26) = 51.05, p < .001). There was a significant interaction between Group × Trial (F (2, 26) = 4.16, p < .05). Post hoc analyses with Bonferroni adjustments showed that the levels of freezing of the chasing-stress group were significantly higher than those of the no-chasing group on trial 1 (p < .05) and trial 2 (p < .01) ().
There were significant main effects of Group (F (1, 27) = 4.80, p < .05) and Trial (F (2, 26) = 32.63, p < .001) on the levels of USV. There was a significant interaction between Group × Trial (F (2, 26) = 5.28, p < .05). Post hoc analyses with Bonferroni adjustments showed that there was a marginally significant difference on trial 1 (p = .057) and a significant difference on trial 2 (p < .01) between the two groups ().
illustrate freezing and USV during ITI. There were significant main effects of Group (F (1, 27) = 7.82, p < .01) and Trial (F (2, 26) = 6.99, p < .001), but no significant Group × Trial interaction (F (2, 26) = 1.46, p > .05), with respect to the levels of freezing. Post hoc analyses with Bonferroni adjustments demonstrated a significantly higher level of freezing in the chasing-stress group than in the no-chasing group on trial 1 (p < .01) ().
There were significant main effects of Group (F (1, 27) = 5.95, p < .05) and Trial (F (2, 26) = 7.32, p < .01), but no significant interaction between Group × Trial (F (2, 26) = 0.28, p > .05) with respect to the levels of USV. Post hoc analyses with Bonferroni adjustments showed significantly higher levels of USV in the chasing-stress group than in the no-chasing group on trial 1 (p < .05) and trial 2 (p < .05) (). In addition, the number of animals that showed USVs in the chasing-stress group was significantly higher than that in the no-chasing group (Figure not shown, 15 out of 15 in the chasing-stress group vs. 8 out of 14 in the no-chasing group; Fisher’s exact test, p < .01).
To examine the sensitized response to the shock more closely, freezing and USV before (i.e. during the 1st CS) and after (i.e. during the 1st ITI) the first shock were compared. Regarding freezing, there were significant main effects of Group (F (1, 27) = 14.21, p < .01) and Shock (F (1, 27) = 34.76, p < .001). There was also a significant Group × Shock interaction (F (1, 27) = 6.66, p < .05) with respect to the levels of freezing. Post hoc analyses with Bonferroni adjustments showed significantly higher levels of freezing in the chasing-stress group than in the no-chasing group during the 1st CS (p < .05) and during the 1st ITI (p < .01) (). To further examine the interaction, differential freezing before and after the shock was compared between the two groups. A t-test showed that the level of differential freezing was significantly higher in the chasing-stress group than in the no-chasing group (Figure not shown, t (27) = −2.58, p < .05).
Regarding USV, there were significant main effects of Group (F (1, 27) = 5.98, p < .05) and Shock (F (1, 27) = 20.79, p < .001). There was no significant Group × Shock interaction (F (1, 27) = 3.20, p = .085). Post hoc analyses with Bonferroni adjustments showed that the difference between the two groups was significant during the 1st ITI (p < .05) but was only marginally significant during the 1st CS (p < .06) (). The differential USV before and after the shock was not significantly different between the two groups (Figure not shown, t (27) = −1.79, p > .05).
Taken together, these results indicate that chasing stress induced sensitized defensive responses to a completely different aversive stimulus such as footshock.
3.2.4. PTC retention tests
To test the effects of chasing stress on the formation of new memories, retention tests for the context and tone used during PTC were conducted.
During the PTC context retention test, rats were placed in the conditioning chamber for 5 min. There were no significant differences between the two groups in the levels of freezing (t (27) = −0.41, p > .05) or USV (U = 103.00, p > .05).
Three hours later, rats were subjected to the PTC tone retention test. They were presented with two trials of the tone CS in a different context. There were no significant differences between the two groups in the levels of freezing (U = 130.00, p > .05) or USV (U = 120.00, p > .05). Results from the PTC retention tests indicate that the sensitizing effect of chasing stress does not facilitate or extend to the formation of new fear memories.
4. Discussion
In the current study, we developed a novel stress paradigm in which the rats were repeatedly chased by a fast-approaching robot in an unpredictable, inescapable manner. The validity of chasing stress as a model of psychogenic stress was examined. First, we observed increased CORT levels in response to chasing stress, comparable to those induced by restraint stress, a commonly used stress treatment. It has been revealed that stress induces activation of the hypothalamic–pituitary–adrenal (HPA) axis resulting in an increase in CORT secretion, whereas there could be other factors causing elevation of CORT (Kim & Diamond, Citation2002). Physical activity would be the most reasonable factor in the current study. However, the increased CORT level may not be due to the animal’s movement as the chasing-stress group froze more than the no-chasing group during chasing. Therefore, it could be concluded that chasing stress, indeed, induced a physiological stress response.
Second, the rats undergoing chasing stress displayed various defensive responses during chasing. The most frequently observed defensive responses were freezing, USV, and defecation, which have been used as representative indices of an internal state of fear and distress in rodent research (Antoniadis & McDonald, Citation1999; Blanchard & Blanchard, Citation1969; Brudzynski & Ociepa, Citation1992).
Interestingly, during the course of the chasing-stress procedure (In Exp. 1, 60 trials), there were significant non-linear modulations of passive and active responses. Following the initial phase, during which a steady increase in passive responses due to PTC was invariably observed, there was a temporary reduction in freezing () coinciding with increased frequency of an avoidance response (), perhaps representing spontaneous switching from a passive to an active response strategy. This mirroring of the two response outcomes may indicate a mutually exclusive interaction between the two response systems, which could be explained at the level of neural circuits (LeDoux & Gorman, Citation2001).
Although animals in threat situations can demonstrate an overwhelming number of passive coping responses such as immobility due to PTC, a body of evidence suggests that appropriate training can redirect a passive response to a more active, avoidance-oriented response strategy (Amorapanth, LeDoux, & Nader, Citation2000). However, other evidence supports the argument that distinctive pathways within the amygdala, namely from the lateral nucleus (LA) to the central nucleus (CE) and from the LA to the basal nucleus (BA), are responsible for activating different coping responses (Choi, Cain, & LeDoux, Citation2010). Therefore, inhibition of CE, which is necessary for the expression of passive responses, could switch a passive response to an active response (Gozzi et al., Citation2010; Moscarello & LeDoux, Citation2013; Yu, da Silva, Albeanu, & Li, Citation2016).
In the majority of previous studies, switching between active and passive defensive reactions was induced by explicit instrumental training (Amorapanth et al., Citation2000). The current chasing-stress paradigm, however, offers a natural window to examine spontaneous transition between the two response strategies, thereby closely mimicking maladaptive responses following repeated failures in coping responses under stressful conditions such as in the learned helplessness model (Maier & Seligman, Citation1976).
Chasing stress induced not only immediate defensive responses but also a long-lasting fear memory and alters the way aversive information was processed during different events. First, the memory of being chased remained strong even 3 weeks after the stress. Rats displayed a substantial number of defensive responses to the tone that had been emitted by the robot, as well as the chasing context (donut maze + stationary robot). This persistence of the fear memory is consistent with previous studies of contextual and cued PTC with a predator (Zoladz, Fleshner, & Diamond, Citation2012) or tactile pain (Maren, Aharonov, & Fanselow, Citation1997) as the US. Therefore, the current paradigm with a chasing robot was not only effective in inducing short-term stress responses but also in forming a long-term fear memory with conditioned emotional responses to a specific cue.
Second, chasing stress induced sensitization to a new aversive situation that involved a completely different set of stimuli (that is, a fast-moving robot in a circular maze vs. an electric shock in a small enclosure). During subsequent PTC with a 4-kHz tone CS and footshock US, the rats in the chasing-stress group showed significantly higher levels of freezing and USV immediately after the first footshock had been presented. Although the chasing-stress group also showed a generalized fear response to CS even before the presentation of the footshock US, the magnitude of the sensitized response after the first footshock was even greater than what would have been expected with generalization alone (). Indeed, the sensitized responses have been observed in contextual fear conditioning following the chasing stress, which supports that the sensitization effect may not be due to the generalization across similar stimuli but due to the chasing stress (data not published). This is consistent with the sensitized reactions of PTSD patients in aversive situations following an initial traumatic experience. The fact that the chasing-stress group did not show increased open arm exploration in the elevated plus maze test indicates that the sensitized reaction in PTC is a specific alarm response to a noxious stimulus rather than generalized anxiety in a new environment.
Our data contribute to the existing line of research showing the facilitative effect of strong initial stress on responses to another stressor (Armario, Gil, Marti, Pol, & Balasch, Citation1991; Weinberg et al., Citation2009) and the processing of subsequent aversive learning (Rau & Fanselow, Citation2009; Rau, DeCola, & Fanselow, Citation2005; Shors, Citation2001; Shors, Weiss, & Thompson, Citation1992). Such initial stress also sensitizes the responsivity of the HPA axis to subsequent stress (Armario et al., Citation1991; Armario, Vallès, Dal-Zotto, Márquez, & Belda, Citation2004; Martí, García, Vellès, Harbuz, & Armario, Citation2001; van Dijken et al., Citation1993). Thus, increased activation of the paraventricular nucleus of the hypothalamus, a brain region involved in the modulation of the HPA axis, has been proposed as a candidate mechanism underlying sensitization (Bruijnzeel et al., Citation1999; Bruijnzeel, Stam, Compaan, & Wiegant, Citation2001; Inoue et al., Citation2013; Bains, Citation2014; Weinberg et al., Citation2009). The fact that the chasing robot and footshock share very few sensory components convincingly demonstrates that the metaplastic effect of the stress is not a simple transfer of associative learning, but a non-associative sensitization. Psychogenic stressors such as predator odor have been shown to sensitize subsequent stress responses after a relatively short delay (Weinberg et al., Citation2009). The current study confirms the persistence of sensitization effects of psychogenic stress even after a long delay.
In summary, the current stress paradigm is characterized by distinct behavioral and endocrine responses, indicating high ecological validity. In addition, we have demonstrated that chasing stress can induce long-lasting fear memory and a sensitized response to footshock. Together with other animal models of PTSD employing psychosocial stress (Zoladz et al., Citation2012; Zoladz, Park, Fleshner, & Diamond, Citation2015), chasing stress could provide a convenient and effective way of inducing psychogenic stress based on the evolutionary history of the prey animal.
Supplemental_movie_1.mp4
Download MP4 Video (4.7 MB)Disclosure statement
No potential conflict of interest was reported by the authors.
Additional information
Funding
References
- Amorapanth, P., LeDoux, J.E., & Nader, K. (2000). Different lateral amygdala outputs mediate reactions and actions elicited by a fear-arousing stimulus. Nature Neuroscience, 3, 74–79.doi:10.1038/71145
- Antoniadis, E.A., & McDonald, R. (1999). Discriminative fear conditioning to context expressed by multiple measures of fear in the rat. Behavioural Brain Research, 101, 1–13.
- Armario, A., Gil, M., Marti, J., Pol, O., & Balasch, J. (1991). Influence of various acute stressors on the activity of adult male rats in a holeboard and in the forced swim test. Pharmacology, Biochemistry, and Behavior, 39, 373–377.
- Armario, A., Vallès, A., Dal-Zotto, S., Márquez, C., & Belda, X. (2004). A single exposure to severe stressors causes long-term desensitisation of the physiological response to the homotypic stressor. Stress, 7, 157–172. doi:10.1080/10253890400010721
- Blanchard, R.J., & Blanchard, D.C. (1969). Passive and active reactions to fear-eliciting stimuli. Journal of Comparative and Physiological Psychology, 68, 129–135.
- Bains, J. (2014). Stress-induced metaplasticity at GABA synapses. In M. Popoli, D. Diamond & G. Sanacora (Eds.), Synaptic stress and pathogenesis of neuropsychiatric disorders. (pp. 125–136). New York, NY: Springer.
- Brudzynski, S.M., & Ociepa, D. (1992). Ultrasonic vocalization of laboratory rats in response to handling and touch. Physiology & Behavior, 52, 655–660.
- Bruijnzeel, A.W., Stam, R., Compaan, J.C., Croiset, G., Akkermans, L.M., Olivier, B., & Wiegant, V.M. (1999). Long-term sensitization of Fos-responsivity in the rat central nervous system after a single stressful experience. Brain Research, 819, 15–22.
- Bruijnzeel, A.W., Stam, R., Compaan, J.C., & Wiegant, V.M. (2001). Stress-induced sensitization of CRH-ir but not P-CREB-ir responsivity in the rat central nervous system. Brain Research, 908, 187–196.
- Choi, J.S., Cain, C.K., & LeDoux, J.E. (2010). The role of amygdala nuclei in the expression of auditory signaled two-way active avoidance in rats. Learning &Amp; Memory (Cold Spring Harbor, N.Y.), 17, 139–147. doi:10.1101/lm.1676610
- Choi, J.S., & Kim, J.J. (2010). Amygdala regulates risk of predation in rats foraging in a dynamic fear environment. Proceedings of the National Academy of Sciences of the United States of America, 107, 21773–21777. doi:10.1073/pnas.1010079108
- Gozzi, A., Jain, A., Giovanelli, A., Bertollini, C., Crestan, V., Schwarz, A.J., … Bifone, A. (2010). A neural switch for active and passive fear. Neuron, 67, 656–666. doi:10.1016/j.neuron.2010.07.008
- Hansen, Å.M., Hogh, A., Persson, R., Karlson, B., Garde, A.H., & Ørbaek, P. (2006). Bullying at work, health outcomes, and physiological stress response. Journal of Psychosomatic Research, 60, 63–72. doi:10.1016/j.jpsychores.2005.06.078
- Inoue, W., Baimoukhametova, D.V., Füzesi, T., Cusulin, J.I., Koblinger, K., Whelan, P.J., … Bains, J.S. (2013). Noradrenaline is a stress-associated metaplastic signal at GABA synapses. Nature Neuroscience, 16, 605–612. doi:10.1038/nn.3373
- Kim, J.J., & Diamond, D.M. (2002). The stressed hippocampus, synaptic plasticity and lost memories. Nature Reviews Neuroscience, 3, 453–456. doi:10.1038/nrn849
- LeDoux, J.E., & Gorman, J.M. (2001). A call to action: Overcoming anxiety through active coping. American Journal of Psychiatry, 158, 1953–1955. doi:10.1176/appi.ajp.158.12.1953
- Lee, H.J., Choi, J.S., Brown, T.H., & Kim, J.J. (2001). Amygdalar NMDA receptors are critical for the expression of multiple conditioned fear responses. The Journal of Neuroscience: The Official Journal of the Society for Neuroscience, 21, 4116–4124.
- Lingle, S., & Pellis, S. (2002). Fight or flight? Antipredator behavior and the escalation of coyote encounters with deer. Oecologia, 131, 154–164. doi:10.1007/s00442-001-0858-4
- Maier, S.F., & Seligman, M.E. (1976). Learned helplessness: Theory and evidence. Journal of Experimental Psychology General, 105, 3–46.
- Maren, S., Aharonov, G., & Fanselow, M.S. (1997). Neurotoxic lesions of the dorsal hippocampus and Pavlovian fear conditioning in rats. Behavioural Brain Research, 88, 261–274.
- Martí, O., García, A., Vellès, A., Harbuz, M.S., & Armario, A. (2001). Evidence that a single exposure to aversive stimuli triggers long-lasting effects in the hypothalamus-pituitary-adrenal axis that consolidate with time. The European Journal of Neuroscience, 13, 129–136.
- Moore, I.T., Lemaster, M.P., & Mason, R.T. (2000). Behavioural and hormonal responses to capture stress in the male red-sided garter snake, thamnophis sirtalis parietalis. Animal Behaviour, 59, 529–534. doi:10.1006/anbe.1999.1344
- Moscarello, J.M., & LeDoux, J.E. (2013). Active avoidance learning requires prefrontal suppression of amygdala-mediated defensive reactions. The Journal of Neuroscience: The Official Journal of the Society for Neuroscience, 33, 3815–3823. doi:10.1523/JNEUROSCI.2596-12.2013
- Radley, J.J., Arias, C.M., & Sawchenko, P.E. (2006). Regional differentiation of the medial prefrontal cortex in regulating adaptive responses to acute emotional stress. The Journal of Neuroscience: The Official Journal of the Society for Neuroscience, 26, 12967–12976. doi:10.1523/JNEUROSCI.4297-06.2006
- Rau, V., DeCola, J.P., & Fanselow, M.S. (2005). Stress-induced enhancement of fear learning: An animal model of posttraumatic stress disorder. Neuroscience and Biobehavioral Reviews, 29, 1207–1223. doi:10.1016/j.neubiorev.2005.04.010
- Rau, V., & Fanselow, M.S. (2009). Exposure to a stressor produces a long lasting enhancement of fear learning in rats. Stress, 12, 125–133. doi:10.1080/10253890802137320
- Renner, M.J. (1988). Learning during exploration: The role of behavioral topography during exploration in determining subsequent adaptive behavior in the sprague-dawley rat. International Journal of Comparative Psychology, 2, 43–56.
- Shors, T.J. (2001). Acute stress rapidly and persistently enhances memory formation in the male rat. Neurobiology of Learning and Memory, 75, 10–29. doi:10.1006/nlme.1999.3956
- Shors, T.J., Weiss, C., & Thompson, R.F. (1992). Stress-induced facilitation of classical conditioning. Science (New York, N.Y.), 257, 537–539.
- Telensky, P., Svoboda, J., Blahna, K., Bureš, J., Kubik, S., & Stuchlik, A. (2011). Functional inactivation of the rat hippocampus disrupts avoidance of a moving object. Proceedings of the National Academy of Sciences of the United States of America, 108, 5414–5418. doi:10.1073/pnas.1102525108
- van Dijken, H.H., de Goeij, D.C., Sutanto, W., Mos, J., de Kloet, R., & Tilders, F.J. (1993). Short inescapable stress produces long-lasting changes in the brain-pituitary-adrenal axis of adult male rats. Neuroendocrinology, 58, 57–64. doi:10.1159/000126512
- Weinberg, M.S., Bhatt, A.P., Girotti, M., Masini, C.V., Day, H.E., Campeau, S., & Spencer, R.L. (2009). Repeated ferret odor exposure induces different temporal patterns of same-stressor habituation and novel-stressor sensitization in both hypothalamic-pituitary-adrenal axis activity and forebrain c-fos expression in the rat. Endocrinology, 150, 749–761. doi:10.1210/en.2008-0958
- Wilson, B.S., & Wingfield, J.C. (1992). Correlation between female reproductive condition and plasma corticosterone in the lizard Uta stansburiana. Copeia, 3, 691–697.
- Wingfield, J.C., Smith, J.P., & Farner, D.S. (1982). Endocrine responses of white-crowned sparrows to environmental stress. Condor, 84, 399–409.
- Young, S.L., & Fanselow, M.S. (1992). Associative regulation of Pavlovian fear conditioning: unconditional stimulus intensity, incentive shifts, and latent inhibition. Journal of Experimental Psychology. Animal Behavior Processes, 18, 400–413.
- Yu, K., da Silva, P.G., Albeanu, D.F., & Li, B. (2016). Central amygdala somatostatin neurons gate passive and active defensive behaviors. The Journal of Neuroscience, 36, 6488–6496. doi:10.1523/JNEUROSCI.4419-15.2016
- Zoladz, P.R., Fleshner, M., & Diamond, D.M. (2012). Psychosocial animal model of PTSD produces a long-lasting traumatic memory, an increase in general anxiety and PTSD-like glucocorticoid abnormalities. Psychoneuroendocrinology, 37, 1531–1545. doi:10.1016/j.psyneuen.2012.02.007
- Zoladz, P.R., Park, C.R., Fleshner, M., & Diamond, D.M. (2015). Psychosocial predator-based animal model of PTSD produces physiological and behavioral sequelae and a traumatic memory four months following stress onset. Physiology & Behavior, 147, 183–192. doi:10.1016/j.physbeh.2015.04.032