Abstract
Sorghum grain is a staple food for about 500 million people in 30 countries in Africa and Asia. Despite this contribution to global food production, most of the world’s sorghum grain, and nearly all in Western countries, is used as animal feed. A combination of the increasingly important ability of sorghum crops to resist heat and drought, the limited history of the use of sorghum in Western foods, and the excellent functional properties of sorghum grain in healthy diets, suggests a greater focus on the development of new sorghum-based foods. An understanding of the structural and functional properties of sorghum grain to develop processes for production of new sorghum-based foods is required. In this review, we discuss the potential of sorghum in new food products, including sorghum grain composition, the functional properties of sorghum in foods, processing of sorghum-based products, the digestibility of sorghum protein and starch compared to other grains, and the health benefits of sorghum. In the potential for sorghum as a major ingredient in new foods, we suggest that the gluten-free status of sorghum is of relatively minor importance compared to the functionality of the slowly digested starch and the health benefits of the phenolic compounds present.
Introduction
Sorghum is a genus in the tribe Andropogoneae, the subfamily Panicoideae and the family Poaceae (the grasses) (Spangler et al. Citation1999). Cultivated sorghum (Sorghum bicolor (L.) Moench) is a C4 and self-pollinated plant with high photosynthetic efficiency (Gomez-Martinez and Culham Citation2000; Goyal et al. Citation2020). Sorghum is a drought-tolerant crop (Wagaw Citation2019) cultivated in arid and semi-arid regions of sub-Saharan Africa, Asia, the Middle East, as well as Central and North America and Australia. This cereal can also survive cool weather (12–15 °C), water-logged conditions (for short periods) and grow in high-rainfall areas (Lim Citation2012). Sorghum grain is a staple food in parts of Africa, Asia and other low-income regions, as well as a fodder and feed crop for livestock (Proietti, Frazzoli, and Mantovani Citation2015), with an annual world production (as of January 2021) of 61.62 million metric tonnes (MMT) (USDA-FAS Citation2021).
The major sorghum-producing countries are USA, Nigeria, Ethiopia, Sudan, Mexico, and India, followed by China, Argentina, Brazil and Niger (). In Africa and Asia, whole sorghum grain, decorticated grain and sorghum flour are used to make a large range of foods. These include thin or thick fermented or unfermented porridge, boiled products similar to those made with maize or rice grits, popped sorghum, flat bread and various types of deep-fried foods. Sorghum has been used in the production of beverages such as nonalcoholic fermented drinks and (alcoholic) beer in Africa and Mexico, as well as spirit and vinegar in China. Sorghum is also used as a wheat substitute to produce gluten-free foods (Chávez et al. Citation2018; Pineli et al. 2015) including pastas, breads, biscuits and porridges (Table 2).
Table 1. Sorghum grain production in the 10 leading sorghum-producing countries globally from 2018 to 2021 (USDA-FAS Citation2021).
Table 2. Sorghum foods from around the world.
Sorghum is a cereal crop of incredible genetic and phenotypic variability, both among S. bicolor and its wild relatives (Mace et al. Citation2021). Sorghum grain quality, appearance and color are affected by genes controlling the presence (B1 and B2) or absence (b1 and b2) of a testa layer, pericarp color (R and Y) and thickness (Z), as well as endosperm color and structure (wx) (Rooney and Miller Citation1982). A single recessive gene, wx, controls the production of waxy endosperm in sorghum. Sorghum with ∼25% amylose in the endosperm is termed normal (WxWxWx) while grain with almost 100% amylopectin is termed waxy (wxwxwx). Grain with an intermediate amount of amylose (∼15%) is known as heterowaxy (WxWxwx or Wxwxwx) (Sang et al. Citation2008).
Sorghum varieties have been classified in several different ways (Shin Citation1986):
Based on distinguishing plant characters (taxonomy): A: leaves, glumes, florets B: kernel size, shape, pericarp, endosperm and testa.
Based on plant responses to environmental factors such as drought, temperature, humidity and photoperiod.
Based on use: A: Sorgo or sweet sorghum (for biofuel and sorghum sirup) B: Broomcorn (for brooms and whiskbrooms) and C: Grain sorghum (for biofuel, human foods and animal feed), which is classified in five sub-groups based on growing region: 1) Durra: predominantly in the Mediterranean and Middle East; 2) Shallu: in India and tropical Africa; 3) Kafir: mainly in South Africa; 4) Kaoliang: predominant in Eastern Asia; 5) Milo: in Eastern Africa.
Based on grain color and presence/absence of a pigmented testa (USDA-FGIS Citation2009):
Sorghum – Low in tannin due to the absence of a pigmented testa. (Tannin consists of specific phenolic compounds with molecular weights between 500 and 3,000.) This class includes around 98% white sorghum grains and 2% tannin-containing sorghum grains among the grain traded. The pericarp colour of this class may be white, yellow, pink, orange, red or bronze.
Tannin Sorghum – Contains more than 90% tannin-containing grains as judged by the presence of a pigmented testa. The pericarp colour is usually brown but may also be white, yellow-pink, orange, red or bronze.
White Sorghum – Low tannin content due to the absence of a pigmented testa. The pericarp colour is white or translucent. This class may include sorghums containing dark spots that cover less than 25% of the kernel.
Mixed Sorghum – This class of sorghum does not meet the definitions for any of the other three classes.
Grain sorghums are also categorized into type I, II, and III based on their tannin content (Price and Butler Citation1977). Type I sorghum is tannin free, while type II and III sorghums contain low and high levels of tannins, respectively. Due to their resistance against birds and insects, extreme weather and their high yield potential, tannin-containing sorghums may be preferred by farmers (Lubadde et al. Citation2019). However, tannin is one of the major nutrient-limiting components of the grain for animal or human consumption. Tannin is reported to reduce starch and protein digestibility and to lower weight gain in monogastric animals such as poultry and rabbit (Liu et al. Citation2015; Muriu et al. Citation2002). Other studies have confirmed through in vitro tests the reduction in the digestibility of starch and protein as a result of interaction with tannin (Barros, Awika, and Rooney Citation2012, Citation2014; Dunn et al. Citation2015).
Sorghum grain structure
The general composition of a sorghum grain has been reported to be 3–6% pericarp, 84–90% endosperm, and 5–10% germ () (Rooney, Rooney, and Serna-Saldivar Citation2016).
Figure 1. Sorghum kernel structure. Reprinted from Earp, McDonough, and Rooney (Citation2004) with permission from Elsevier.
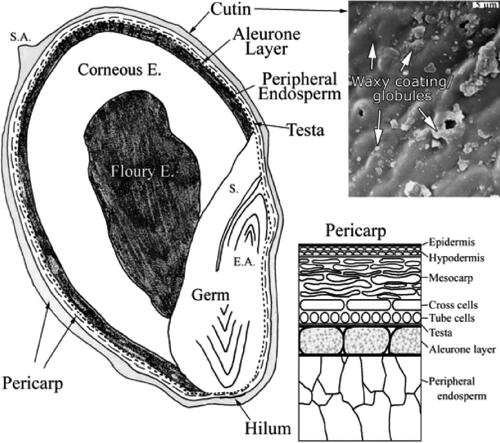
Pericarp
The pericarp is the outermost structure of the sorghum grain and is divided into three sub-tissues: epicarp, mesocarp and endocarp. The epicarp is made of elongated, rectangular cells covered by a thin waxy layer, and is composed of two sub-layers: the epidermis and hypodermis. Pigments are often present in the epidermis. Compared to the epidermis, the hypodermis has slightly thinner cells and is composed of one to three cell layers (Rooney, Rooney, and Serna-Saldivar Citation2016).
The mesocarp (the middle layer) is the thickest layer of the sorghum pericarp; however, its thickness differs greatly among genotypes. Unlike the mesocarp of most cereals, sorghum mesocarp contains starch granules (Waniska and Rooney Citation2000). The endocarp, the innermost layer, is composed of cross cells and tube cells.
The thickness of the pericarp is an important factor affecting grain processing. A thick pericarp along with a corneous endosperm is required to generate a high yield of sorghum flour (Shin Citation1986). A hard and thick pericarp is much easier to remove by pounding, which is a common practice in Africa, whereas a thin pericarp requires more time to remove, mainly achieved via a dehulling method (Guindo et al. Citation2016). However, sorghum varieties with a thick pericarp have some disadvantages, such as sensitivity to mold, grain weathering in the field during maturation, and rapid deterioration of grains during storage (Earp, McDonough, and Rooney Citation2004; Guindo et al. Citation2016).
The color of sorghum grain varies greatly due to pericarp pigmentation and thickness, as well as the presence of a testa. Phenolic compounds, particularly tannin, contribute to the pigmentation of the pericarp and testa (Sedghi et al. Citation2012).
Ferulic acid is the dominant phenolic acid in sorghum grain, as in many small-grained cereals (Boz Citation2016). Sorghum grain also contains flavonoids; e.g., flavonols (quercetin and kaempferol), flavanone (naringenin), and flavones (apigenin and luteolin) (Przybylska-Balcerek, Frankowski, and Stuper-Szablewska Citation2019).
Sorghum grain color is determined by the genes that control pericarp color (R and Y gene), pericarp thickness (Z gene), presence of pigmented testa (B1 and B2 genes), spreader (S gene), testa color (Tp genes), and the color intensifier (I gene) (Rooney and Waniska Citation2000; Xiong et al. Citation2019). According to the phenolic profile and genotype, sorghum can have different colors such as white (RRyy and rryy), yellow (rrYY), red (RRYY), brown (B1B2_), and black (RY_ and/or B1B2_) (Dykes et al. Citation2011; Dykes and Rooney Citation2006; Dykes, Rooney, and Rooney Citation2013; Waniska and Rooney Citation2000).
Dykes et al. (Citation2009) reported that the composition of flavonoids in red sorghum is related to its R_Y_ genes for red pericarp and that the composition varies among different sorghum genotypes. The authors reported that red sorghums, especially those with a black pericarp, have higher 3-deoxyanthocyanin levels and also stated that the pericarp thickness is controlled by the Z gene.
Testa
Beneath the endocarp is the testa layer or seed coat. The testa among sorghum genotypes can be pigmented or non-pigmented. The B1B2 gene combination generates a pigmented testa layer, in which tannin is present, while B1b2, b1B2, or b1b2 lead to an absence of pigmented testa. Tp genes control the specific color of the testa (Wu et al. Citation2012). A purple testa is found when Tp genes are homozygous recessive (tptp), while TpTp or Tptp will give rise to a brown testa. The highest level of condensed tannin is in sorghums containing dominant B1BB2S genes; the S gene controls the presence of brown pigments, as well as tannins in the epicarp and endocarp when a pigmented testa is present (Blakeley, Rooney, Sullins, & Miller, 1979). The B1B2 genes appear to exert control over the polymerization of flavans (anthocyanidins) to flavan-3-ols (building blocks for tannins).
Endosperm
As in other cereals, the endosperm is the storage tissue and major part of the sorghum grain. It consists of the aleurone layer, as well as the peripheral, corneous and floury zones. The aleurone lies just under the testa, and consists of cells with thick cell walls. The aleurone contains minerals, hydrolytic enzymes (mainly synthesized upon imbibition of the grain) and lipid (Waniska and Rooney Citation2000). The periphery of the endosperm (not including the aleurone layer) is composed of several layers of dense cells containing starch granules and protein.
The endosperm corneous and floury zones are composed of starch granules, a protein matrix, protein bodies and cell walls rich in glucuronoarabinoxylans (Hoseney Citation1994). The starch granules and protein bodies are trapped in a protein matrix in the peripheral and corneous regions of the endosperm. The floury endosperm is located in the core of the grain and has a very low abundance of protein bodies compared to the corneous and peripheral layers. The proportion of floury to corneous endosperm largely determines the endosperm texture, which is a property linked to milling yield of the grain and digestibility. The higher the ratio of corneous to floury endosperm, the easier it is to decorticate the sorghum grain, resulting in a higher yield of flour (Reichert, Youngs, and Oomah Citation1982).
Germ
The germ is divided into two major parts: the embryonic axis and scutellum. The embryonic axis forms the new plant and is subdivided into a radicle and plumule. The radicle forms primary roots, whereas the plumule forms leaves and stems. The scutellum is the single cotyledon of the sorghum seed. It contains large amounts of oil (in spherosomes), proteins (including enzymes) and minerals, and serves as the connection between the endosperm and germ (Rooney, Rooney, and Serna-Saldivar Citation2016; Waniska and Rooney Citation2000).
Sorghum grain composition
Carbohydrates
The carbohydrates of sorghum grain are the polysaccharides starch, which is dominant, and fructosan, the trisaccharide raffinose, the disaccharides sucrose and maltose, and the monosaccharides glucose and fructose (Anglani Citation1998). Starch is the major component and is mainly located as granules in the endosperm; however, some starch granules can be found in the pericarp of the grain (Taylor & Emmambux, 2010). Khan et al. (Citation2013) reported that the range of starch content is 55.6–70.0% of dry grain weight depending on the cultivar.
Like starches from other sources, sorghum starch is made up of two biopolymers, amylose and amylopectin, with the ratio determining many physicochemical, thermal and rheological properties of the starch (Sang et al. Citation2008). Amylose and (to a lesser degree) amylopectin strongly affect starch gelatinization, retrogradation, paste viscosity, gelation and α-amylase digestibility (Dadnia, Mojaddam, and Ayran Citation2018; Sang et al. Citation2008; Yanagida et al. Citation2006). Typically, sorghum starches display higher gelatinization temperatures (68–78 °C), a higher degree of retrogradation, but slower enzymatic hydrolysis rates than starches from other cereals, such as maize (gelatinization at 62–72 °C), wheat (58–64 °C) and barley (51–60 °C) (Collar, 2017; Hoseney Citation1994). These properties could be due to the smaller number of short-branch chains in the amylopectin of sorghum compared to other cereals (Ai Citation2013). The high gelatinization temperature of sorghum starch may have an adverse impact on the quality of baked products (Taylor and Dewar Citation2001).
High peak paste viscosity, water uptake and set back, as well as low gelatinization temperature, have been shown to be advantageous in making flat breads such as Roti from sorghum, while low peak paste viscosity and high gelatinization temperature are desirable for making stiff porridge such as Indian Sankhati and African Tô (Ratnavathi and Patil Citation2013).
The free sugar content of sorghum grain is reported to be in the range 1–2% (Shin Citation1986). Non-starch polysaccharides (NSPs) are generally located in the pericarp and endosperm cell walls of sorghum grain. NSPs are the main cause of the insolubility and resistant nature of sorghum endosperm carbohydrates (Hoseney Citation1994). Wheat endosperm contains arabinoxylans, which are water-soluble; in contrast, sorghum endosperm cell walls contain high levels of glucuronoarabinoxylans, which are water-insoluble and may be disadvantageous in bread making and brewing (Taylor, Schober, and Bean Citation2006a; Taylor & Emmambux, 2010).
The in vitro digestibility of starch isolated from sorghum is reported to be 33–48%, which is considerably lower than that of corn starch at 53–58% (Sikabbubba Citation1989). Floury sorghum grains with their amorphous structure are more prone to enzymatic digestion as compared to the more ordered structure of corneous grains. Thus, starch from relatively floury sorghum grain after milling has a higher digestibility than starch from relatively corneous grains. This is because the floury grain particle size is lower, which facilitates enzymatic reactions required for digestion. Normal (non-waxy) sorghum is reported to have lower starch digestibility compared to waxy sorghum (Hibberd et al. Citation1982).
Proteins
Proteins make up a key component (on average 7–15%) of the sorghum grain, and are located mostly in the endosperm. Sorghum proteins are divided into albumins, globulins, glutelins and kafirins. The kafirins, which are hydrophobic, protease-resistant prolamins, represent 50–80% of total protein, with the glutelins being the second most abundant (Duodu et al. Citation2003; FAO Citation1995; Hamaker et al. Citation1995; Oria, Hamaker, and Shull Citation1995). The kafirins are divided into four subgroups based on differences in structure, solubility and molecular weight: α-kafirins (23 and 25 kDa, 80%), β-kafirins (20 kDa, 5%), γ-kafirins (28 kDa, 15%) and trace amounts of δ-kafirins (Belton et al. Citation2006; Izquierdo and Godwin Citation2005; Jambunathan, Mertz, and Axtell Citation1975; Taylor et al. Citation2007).
Amino acid analysis indicates that all three types of kafirins contain low amounts of lysine, an essential amino acid in the human diet. β-Kafirins and γ-kafirins are high in cysteine, and δ-kafirins are fairly high in methionine, another essential amino acid (Shull, Watterson, and Kirleis Citation1992). Protein bodies in both peripheral and central endosperm contain mainly α-kafirins, whereas the central endosperm contains greater amounts of β- and γ-kafirins than the periphery (Lim Citation2013).
Research on the structure of sorghum protein bodies shows that both γ- and β-kafirins cover the more digestible α-kafirins in a disulfide bond polymer network that shields the α-kafirins from exposure to proteases (Shull, Watterson, and Kirleis Citation1992).
In vivo and in vitro tests on sorghum protein digestibility have produced values ranging from 30 to 72%, which are lower than for wheat (81%) and maize (73%) (Axtell et al. Citation1981; Nawar et al. Citation1970). However, whole-grain sorghum with 46% protein digestibility showed a 35% increase in protein digestibility when the grains were decorticated and an extruded sorghum food was produced (MacLean et al. Citation1983).
Sorghum proteins are unable to form a gas-entrapping, viscoelastic dough, which is a basic demand for leavened bread making and for the production of many other baked products (Taylor and Dewar Citation2001). The other major issue with sorghum proteins is that their digestibility can be reduced if the grains are cooked during processing (Arbab and El Tinay Citation1997).
Lipids, vitamins and minerals
Sorghum grain contains nearly 3% lipid, which is lower than in millet and maize but higher than in rice and wheat (Dykes and Rooney Citation2006; Serna-Saldivar and Rooney Citation1995). Sorghum grain lipids are mainly found in the scutellum, and have a typical fatty acid profile of 49% linoleic (18:2), 31% oleic (18:1), 14% palmitic (16:0), 2.7% linolenic (18:3) and 2.1% stearic (18:0) acids (Dykes and Rooney Citation2006; Glew et al. Citation1997). Sorghum lipid would have an impact on the flavor and shelf life of sorghum-based foods (Shin Citation1986).
Sorghum grain also contains a considerable abundance of β-carotene (the provitamin of vitamin A) and tocopherols, and is a source of lipid-soluble vitamins such as A, D and K, as well as water-soluble vitamins such as thiamin, riboflavin, and pyridoxine (Martino et al. Citation2012).
Sorghum grain pericarp, aleurone layer and germ are rich in minerals such as magnesium, iron, zinc, copper, calcium, phosphorus and potassium (Anglani Citation1998; Glew et al. Citation1997). Vitamins and minerals in sorghum grain are mainly located in the aleurone and germ, while lipids are concentrated in the germ. For this reason, decortication of sorghum grain will result in the loss of important nutrients.
Phenolic compounds
Phenolic compounds represent the largest group of phytochemicals and are found throughout the Plant Kingdom. The basic structural feature of phenolic compounds is an aromatic ring bearing one or more hydroxyl groups (Chirinos et al. Citation2009). Plant phenolic compounds are classified as simple phenols or polyphenols based on the number of phenol units in the molecule. Thus, plant phenolics comprise simple phenols, coumarins, lignins, lignans, condensed and hydrolyzable tannins, phenolic acids and flavonoids (Soto-Vaca et al. Citation2012).
Phenolic compounds are synthesized in plants partly as a response to ecological and physiological pressures such as pathogen and insect attack, UV radiation and wounding (Diaz Napal et al. Citation2010; Kennedy and Wightman Citation2011; Zulak et al. Citation2006). These phytochemicals can act as antioxidants and are thought to contribute to the prevention of heart disease (Dai and Mumper Citation2010; Wijngaard, Rößle, and Brunton Citation2009), reduce inflammation (Zhang et al. Citation2011), lower the incidence of cancers (Sawadogo et al. Citation2012) and diabetes (Scalbert et al. Citation2005; Thompson et al. Citation1984), as well as reduce rates of mutagenesis in human cells (Gomez-Cordoves et al. Citation2001; Sawadogo et al. Citation2012). The disease protection afforded by the consumption of plant products such as fruits, vegetables and legumes is largely associated with the presence of phenolic compounds.
The chemo-protective nature of phytochemicals and the limitations on the addition of synthetic antioxidants to food has attracted worldwide attention on phytochemicals as good sources of antioxidants and food/beverage colorants (Fu et al. Citation2011; Zhang et al. Citation2015). Sorghum grain is an excellent example of a food ingredient that has been the subject of this interest. Sorghum phenolics comprise of three major categories: flavonoids, phenolic acids and tannins (Khoddami, Mohammadrezaei, and Roberts Citation2017; Khoddami et al. Citation2015). The phenolic compounds of sorghum are well known to act as antioxidants in in vitro assays (Awika Citation2003) and some are known to be more potent antioxidants than vitamins found in other plant species (Rhodes and Price Citation1997).
Sorghum phytochemicals, particularly anthocyanins and other flavonoids, are claimed in some studies to exhibit anti-inflammatory (Bralley et al. Citation2008; Burdette et al. Citation2010; Shim et al. Citation2013), anti-cardiovascular disease (Mellen, Walsh, and Herrington Citation2008), anti-diabetic (Farrar et al. Citation2008; Hargrove et al. Citation2011), anti-cancer (Chen et al. Citation2011; Massey, Reddivari, and Vanamala Citation2014; Wu et al. Citation2011), anti-lipidemic (Chung, Yeo, et al. Citation2011), immunomodulatory (Chung, Yeo, et al. Citation2011), anti-anemic (Oladiji, Jacob, and Yakubu Citation2007), neuroprotective (Oboh, Akomolafe, and Adetuyi Citation2010), anti-diarrheal (Nwinyi and Kwanashie Citation2009), anti-microbial (Mohamed et al. Citation2009; Svensson et al. Citation2010) and anthelminthic (Iqbal et al. Citation2001) activities. While this list of activities is compelling, it is not possible in this review to provide a detailed evaluation of the evidence for each of these claims. Consumption of whole-grain sorghum is reported to lead to lower plasma glucose in diabetic individuals compared to the consumption of whole wheat and decorticated sorghum grain (Lakshmi and Vimala Citation1996; Thompson et al. Citation1984).
The potential health effects of sorghum grain are not just due to the content of phytochemicals. Non-tannin sorghum lipid extract was found to lower the level of cholesterol absorption (in a hamster model), which might be due to the presence of policosanols (waxy, high-molecular-weight aliphatic alcohols with chain-lengths typically 24- to 34-carbons) and sterols in the grain lipid fraction (Carr et al. Citation2005).
Properties of sorghum grain and food quality
Protein and starch digestibility
Sorghum is often regarded as a low-value crop for food uses due to low digestibility of its protein and starch relative to other cereals (Duressa et al. Citation2018). Duodu et al. (Citation2003) reported that the low digestibility of sorghum protein is most pronounced in wet cooked foods where protein digestibility decreases by up to 50%.
The different digestibility of proteins among sorghum cultivars is likely to be related to many factors such as differences in genotype, grain structure, protein body structure, protein cross-linking, and the complement of phenolic compounds (Dunn et al. Citation2015; Kaufman et al. Citation2013; Lemilioglu-Austin Citation2014; Mertz et al. Citation1984; Moraes, Queiroz, et al. Citation2012; Wong et al. Citation2009).
Regardless of protein digestibility, starch digestibility in raw and processed sorghum grain is also low. This might in turn be related to starch and protein cross-linking or binding of starch to tannin, which could limit accessibility of starch granules to amylolytic enzymes (Barros, Awika, and Rooney Citation2012; Dunn et al. Citation2015; Ezeogu, Duodu, and Taylor Citation2005; Lemlioglu-Austin et al. Citation2012; Taylor & Emmambux, 2010; Wong et al. Citation2009a; Zhang and Hamaker Citation1998).
It is common to consider low starch digestibility as a negative attribute; however, since many companies employ energy- and resource-intensive processes to create resistant starches, sorghum-based foods with this property may be valuable in countries with a high incidence of obesity (Awika and Rooney Citation2004).
Health benefits
The chemical composition of sorghum grain is comparable with that of maize, and its nutrient profile is similar to that of wheat (Dicko et al. Citation2006). The nutrients in sorghum grain have a positive role in human health and nutrition, especially in people suffering from disorders such as celiac disease, diabetes and obesity (Pontieri et al. Citation2013).
Consumption of sorghum-based food products might help control the body’s glycemic response, leading to a lower risk of metabolic diseases such as diabetes (Zhang and Hamaker Citation2009). A proportion of the starch in sorghum could also act as resistant starch (dietary fiber), lowering the incidence of some diseases such colon cancer and diabetes (Birt et al. Citation2013). Sorghum-based food products, including bread, biscuits, porridge, pasta and pastry, are excellent for sufferers of Celiac disease due to the absence of gluten in the grain protein profile (Lemilioglu-Austin Citation2014).
Sorghum offers exciting opportunities as a food ingredient and source of compounds that can be used to enhance the health properties of foods. The array of phenolic compounds in sorghum are not commonly found in other cereal grains (Awika Citation2011; Awika and Rooney Citation2004). Sorghum polyphenols have higher potential to suppress cancer cell proliferation than their analogs found in other food sources (Awika Citation2017). Yang et al. (Citation2015) reported that phenolics and other compounds in sorghum have important and unique bioactive properties relevant to cancer prevention, cardiovascular health, and reduced chronic inflammation and oxidative stress, among others.
Recent evidence also suggests polymeric sorghum polyphenols (tannins) may be useful as natural ingredients to reduce the caloric impact of starch (Amoako and Awika Citation2016). The starch and protein in sorghum take a longer time to digest than other similar products. This slow digestion results in low glycemic index and is thus particularly helpful for individuals with diabetes (Hargrove et al. Citation2011; Zhang and Hamaker Citation2009).
Monomeric flavonoids in sorghum have been reported to have varying levels of anti-inflammatory activity (Moraes, Natal, et al. Citation2012). It also reduces the risk of or delay the development or recurrence of cancer (Park et al. Citation2012). Sorghum extracts have anticancer bioactivities higher than other grains like wheat, millet, and panicum, indicating that sorghum can serve as an effective and inexpensive edible supplement in cancer management (Xie et al. Citation2019).
Sorghum is rich in potassium and phosphorus. It has good levels of calcium with small amounts of iron and sodium. It is also high in fiber and protein, making it one of the healthier options for those who cannot consume gluten and therefore cannot eat traditional forms of flour. Malted sorghum flour can be used to make anti-constipation drinks and formulations of other foods. Lawan et al. (Citation2020) tested malted and milled sorghum cultivars (pelpeli white and chakalari white) to make different formulations with cowpea. They reported that a ratio of 70:30 sorghum:cowpea (Pelembe, Erasmus, and Taylor Citation2002) was associated with a slight increase of vitamins in the malted formulations than in formulations without malt. The increase or decrease in vitamins varied with the nature of the raw material, processing methods and supplementations.
Sorghum is a also a good source of vitamins. Egbujie and Okoye (Citation2019) reported that complementary foods formulated with sorghum, yam bean and crayfish had higher levels of ascorbic acid, niacin, thiamin, vitamin A and folic acids than the non-substituted controls. The increase in the vitamin content of the formulations confirms the beneficial effect of complementation.
Eze et al. (Citation2020) reported that when extruded healthy snacks were made from sorghum and charamenya flour blends, extrusion affected vitamin and antinutrient content. The content of vitamins (A, B1, B2, B6, B12, C, and D) and antinutrients (phytate, oxalate and tannin) was reduced post extrusion when compared with the flour blends prior to extrusion. They were significantly (p < 0.05) affected by the feed composition (10–30%), feed moisture content (25–30%) and exit barrel temperature (110–130 °C).
Sorghum-based products
Research on the pasting quality of sorghum indicates that a high setback viscosity is advantageous for porridge making while a low setback is advantageous for bread making (Shin Citation1986). Microscopy showed that waxy grain (high in amylopectin) had larger starch granules with less protein than non-waxy grains (Shin Citation1986).
The main reason that sorghum based-food products are not in favor (globally) compared to other grain products is due to their inferior sensory qualities. For instance, foods based on tannin sorghum have a bitter and astringent taste (Khan et al. Citation2014; Kobue-Lekalake, Taylor, and De-Kock Citation2007; Rose et al. Citation2014). Sorghum containing tannin is reported to have a relatively soft endosperm texture, while tannin-free sorghum is reported to have a hard endosperm with sweet and maize-like flavor (Kobue-Lekalake, Taylor, and De-Kock Citation2007). Decortication of sorghum grain to make sorghum flour is reported to be preferred by consumers because of the lower astringency, lighter color of the food products, and higher digestibility (Taylor and Dewar Citation2001). However, decortication reduces the level of healthy secondary compounds such as phenolics (Buitimea-Cantúa et al. Citation2013; Chiremba, Taylor, and Duodu Citation2009; Dlamini, Taylor, and Rooney Citation2007).
Sorghum is the basis for relatively few food products in the Western world. For example, in contrast to oat, rice, maize and wheat, sorghum grain has not been widely used in the production of ready-to-eat breakfast cereals (Serna-Saldivar and Rooney Citation1995; Welch Citation2011). There are few research publications on producing ready-to-eat sorghum-based foods that have indicated positive feedback from consumers. In a study comparing whole-grain sorghum flakes to wheat bran flakes, consumers reported a better texture and almost the same flavor scores for the sorghum-based product (Shin Citation1986). In other research, a product made from flaked sorghum was compared with rolled oats. The panelists favored the sorghum-based product over the oat-based product (Celis, Rooney, and McDonough Citation1996). Dlamini, Taylor, and Rooney (Citation2007) described the production of extruded sorghum cereals from both tannin-based sorghum (reported to have high antioxidant activity) and non-tannin sorghum. The results indicated that processing in general could decrease the antioxidant activity of sorghum. However, this antioxidant level was lower in extruded sorghum than in conventionally cooked porridge. A combination of sorghum/corn/defatted soy flour combined with whey protein has been shown to improve the sensory qualities of cereal products (Devi et al. Citation2013).
A sorghum-cowpea composite has been developed to make an instant porridge (Pelembe, Erasmus, and Taylor Citation2002). The authors found that a mixture of 50% sorghum and 50% cowpea extruded at 130 °C had functional properties closest to those of a commercial instant maize–soya composite porridge. In another study, cookies made from a combination of cocoyam flour and fermented sorghum flour (50:50) had a high ‘biological value’ (the efficiency of utilization of absorbed nitrogen) and net protein utilization (a measurement of biological value and digestibility of amino acid mixture absorbed from food) (Okpala and Okoli Citation2014).
Production of a low-digestibility pasta that could maintain adequate cooking quality and consumer acceptability was the aim of a study by Khan et al. (Citation2014). Results showed that adding 20% red sorghum flour or 30% white sorghum flour to pasta could reduce starch digestibility without major impacts on preset criteria (the pasta was considered to be acceptable if the mean sensory score for overall acceptability was equal to or higher than 6.0, corresponding to “like slightly” on the 9-point hedonic scale). In another study, incorporation of 40% sorghum flour into flat wheat bread significantly decreased in vitro starch digestibility while maintaining sensory acceptability (Yousif, Nhepera, and Johnson Citation2012).
Processing of sorghum grain
Milling
Milling is the process of grinding, crushing, or pulverizing grains into fine particles with the aim of maximizing the yield of endosperm flour (Taylor and Dewar Citation2001). The kernel size of sorghum is similar to wheat and the pericarp of sorghum is more friable than other cereals (Zhao and Ambrose Citation2018). The friable nature of the sorghum pericarp is probably related to its unique starch (Earp, McDonough, and Rooney Citation2004). The thickness of the pericarp, which is determined by the mesocarp, affects milling properties. Since the pericarp breaks at the mesocarp layer, a thick pericarp holds together better and is removed in larger pieces during dehulling, thus requiring less decorticating time (Zhao Citation2016).
Sorghum kernels are primarily decorticated and milled into flour, or flaked for further processing. Milling sorghum to flour is not as well developed as wheat and traditional milling methods are still used in many countries (Dahir et al. Citation2015). Milling is performed mainly by using a roller mill (similar procedure used for wheat and corn), producing a gritty and speckled flour (Zhao Citation2016).
Dry milling
Methods of sorghum dry milling include various combinations of milling processes. They can involve removing the bran and germ and then reducing the endosperm to fine particles, breaking the kernel and then scraping the endosperm from the bran, or using abrasive decortication to remove the bran, followed by hammer milling. Removal of the germ from sorghum grain to give a clean endosperm is difficult in all milling methods (Shin Citation1986), and failure to remove the germ can substantially reduce the shelf life of sorghum flour as compared to wheat flour.
In grain processing generally, a large germ in proportion to the whole seed is potentially problematic because of the high fat content of the germ, leading to rancidity and thus a relatively low shelf life of the milled product if the endosperm flour is contaminated with germ.
In addition, the sorghum pericarp is highly fragile and, since most of the sorghum polyphenolic compounds (some of which are colored) are located in the pericarp, use of the milled product in foods can lead to an appearance undesirable to the consumer (Taylor and Dewar Citation2001).
Application of the breaking kernel method in sorghum flour production has adverse consequences on the final flour. As mentioned earlier, sorghum bran is highly fragile, and breaking the kernel can result in discoloration of the flour. Using decortication + milling is the most common method in sorghum milling. It involves abrading off the testa and pericarp, thereby reducing unfavorable discoloration due to polyphenolic compounds as well as the antinutrient impact of tannin on the flour (Serna-Saldivar and Rooney Citation1995). In an attempt to overcome these problems, sorghum grain was conditioned to reach 26% moisture at 60 °C for more than 6 hours. The results showed a good level of bran and germ separation but still a low flour yield (Cecil Citation1992). In other research, conditioning to about 16% moisture followed by milling using double rollers gave a higher yield and lower germ contamination in sorghum flour (Gomez Citation1993).
A study on dry grit milling on the milling performance of various sorghum grains from floury to corneous endosperm was performed by Maxson et al. (Citation1971). The floury endosperm grain shattered in the milling process, resulting in a low yield of grit and a high level of lipid, whereas the corneous sorghum grain gave the highest yield of grit with lower levels of ash and lipid.
Wahyuningsih et al. (Citation2019) reported that when dry and wet milling methods were compared, dry milled sorghum flour had a higher protein concentration than wet milled sorghum flour, except for the cross-linked kafirin fraction. Dry milled sorghum flour had more cross-linked kafirin fraction, whereas wet milled sorghum flour only had a small number of protein fraction ribbons from the cross-linked kafirin.
Wet milling
Clean sorghum grain can be soaked in water for about 2 days and then crushed to loosen the embryo. The starch/protein suspension is then centrifuged to separate the protein from the starch. The separated starch is then washed, and either dried for food production or hydrolyzed to make glucose sirup (Shin Citation1986).
The aim of wet milling is mainly to separate and isolate various grain components such as protein, starch and fiber from grains. Wet milling is mostly practised for wheat and maize, and tends not to be applied to sorghum due to difficulties in further processing to separate clean and pure starch, protein and oil (Taylor and Dewar Citation2001).
Amoura, Mokrane, and Nadjemi (Citation2020) reported that when whole sorghum grain flour was wet milled, the protein content and water binding capacity decreased. The authors also reported that gelling concentration, oil binding capacity and the foam capacity increased but in the meantime foam stability and emulsifying stability decreased in a dough made from sorghum flour.
Malting
Malting can be defined as the process of steeping, germination and drying (kilning) of cereal grains to advance the development of hydrolytic enzymes (responsible for converting starch into simple sugars, and other hydrolytic activities), which are absent in un-germinated grains (Taylor and Dewar Citation2001). During steeping, grain moisture content reaches around 45% (Contreras-Jiménez et al. Citation2019; Vinje, Duke, and Henson Citation2015). In germination, metabolism is very active, and enzymes such as hemicellulases, amylases, proteases, oxidases, among others, are involved. The germination process is stopped by kilning. This stage is key to stop the metabolic activity and maintain the quality characteristic of the malt, including the flavor, aroma, and obtaining a malt with a long shelf life (Vinje, Duke, and Henson Citation2015).
Malt (particularly from barley) acts as a crucial ingredient with multiple functions in many beverages and food products, including as a dough leavening agent; in browning of bakery crust; softening and moisture retention of bakery crumb; color, aroma and flavor enhancement; texture improvement and nutrition enrichment, as well as in commercial beverages products like Milo® and Ovaltine®, which are a dry blend of malt extract and milk powder, and Horlicks®, a blend of malt extract, milk powder and wheat flour mashed together and dried (Joe White Malting System Citation2002; Taylor and Emmambux Citation2008).
The malting process has been shown to result in higher in vitro sorghum protein digestibility (Wang and Fields Citation1978). The addition of malted sorghum flour to thick porridge could help to break down the complex carbohydrates present and thereby liquefy the porridge. This in turn could enhance vitamin and mineral availability, as well as the sweetness and flavor of the porridge (Nout et al. Citation1988).
Malting has an impact on the phytochemicals in sorghum, which in turn has an influence on the possible health effects of the finished product. Mixed results have been obtained on the effects of malting on the abundance of phenolics in sorghum grain, although most studies have found a decrease (Ahmed, Mahgoub, and Babiker Citation1996; Bvochora et al. Citation2005; Bvochora et al. Citation1999; Elmaki et al. Citation1999; Kayodé, Hounhouigan, and Nout Citation2007; Khoddami Citation2016; Nwanguma and Eze Citation1996; Taylor and Duodu Citation2015; Towo, Svanberg, and Ndossi Citation2003).
Oseguera-Toledo et al. (Citation2020) reported that during malting of sorghum grain, the amount of reducing sugars increased due to the enzymatic action in amylose and debranching of amylopectin, decreasing the viscosity. Djameh et al. (Citation2015) reported that germination time significantly influenced diastatic power, free amino nitrogen and yield of malt. It was found that optimal conditions for the malting process were 12 h steeping, 5 days of germination at 30 °C and drying at 40 °C.
Fermentation
Fermentation is a process in which a range of food properties are changed by the activities of lactic acid bacteria, a combination of both yeast and lactic acid bacteria, and in some cases specific species of molds. Fermented sorghum products comprise flatbread, doughs, dumplings, porridges, gruels, as well as alcoholic and nonalcoholic beverages (Anukam and Reid Citation2009; Elkhalifa, Ali, and El-Tinay Citation2007; Kalui, Mathara, and Kutima Citation2010; Nout Citation2009; Taylor and Duodu Citation2015).
Several authors have reported that fermentation processes have an impact on the levels of phenolic compounds and the functional properties of sorghum. Adebo, Njobeh, and Kayitesi (Citation2018) reported a decrease in the level of phenolic compounds after fermentation. The drop in the level of these compounds could be due to polyphenol oxidase and peroxidase activities, which degrade polyphenolics, cleavage of proanthocyanidins into flavan-3ols, which are then oxidized to quinones, or polymerization of or interaction with proteins (Starzyńska-Janiszewska et al. Citation2019; Beta et al. Citation2000; Dlamini, Taylor, and Rooney Citation2007; Porter, Hrstich, and Chan Citation1985; Scalbert et al. Citation2000; Towo, Matuschek, and Svanberg Citation2006).
A decrease in total phenolic content and total tannin content has been associated with fermenting sorghum, with this effect attributed to the ability of tannins to bind with proteins and other components, reducing extractability as well as tannin degradation (Adebo, Njobeh, and Kayitesi Citation2018; Dlamini, Taylor, and Rooney Citation2007). In another study, treatment of tannin sorghum gruels by adding germinated sorghum flour (to 5%) and then fermenting the gruels resulted in a significant decrease in the level of both polyphenolics and phytates, with an increase in the level of accessible iron, compared to the untreated and unfermented gruels (Taylor & Duodu, Citation2019). The reduction in polyphenolics and phytates and the increase in iron accessibility were greater when the mixture was incubated with phytase and polyphenol oxidase (Towo, Matuschek, and Svanberg Citation2006).
Fermented sorghum products are microbiologically safe due to low pH (<4) preventing the growth of gram-negative pathogens such as Escherichia coli, Campylobacter jejuni, Shigella flexneri and Salmonella typhimurium. Moreover, the fermentation could inhibit the growth of gram-positive Staphylococcus aureus (Kingamkono et al. Citation1995; Sekwati-Monang and Gänzle Citation2011; Svanberg et al. Citation1992) to prevent infection that could cause serious diseases such as pneumonia.
Sorghum fermentation can have both probiotic and prebiotic activity. For instance, fermented sorghum sourdough inoculated with the probiotic gram-positive bacterium, Weisella cibaria, produced substantial levels of prebiotics (carbohydrates that cannot be digested by human enzymes), which can stimulate ‘good’ bacteria in the colon (Schwab et al. Citation2008).
Ojha et al. (Citation2018) reported that fermentation of sorghum grain reduced bulk density, viscosity and antimicrobial factors in sorghum flour while its functional properties were improved compared to the unfermented control.
Thermal processing
One of the most common methods to process cereal grains is thermal processing, which can be performed by wet, steam and extrusion cooking, micronization (the process of reducing the average diameter of a solid material's particles), gun or oven puffing, baking and roasting (Niernberger and Taylor Citation2001; Taylor and Duodu Citation2015) and recently the use of microwave heat treatment (Sharanagat et al. Citation2019; Adebowale, Taylor, and de Kock Citation2020).
White non-tannin and red tannin sorghums have been compared to oats as main ingredients of breakfast cereals and processed using a twin screw extruder. The red sorghum had a higher level of protein while the white sorghum had more starch (p < 0.05). The red sorghum protein digestibility dropped compared to the oat reference and white sorghum grain, while the level of resistant starch was higher in the white sorghum than the red sorghum and oat cereal product (Mkandawire et al. Citation2015).
Al-Rabadi et al. (Citation2011) reported that particle size and extrusion temperature had a significant impact on processing parameters including specific mechanical energy. Licata et al. (Citation2014) reported for extruded sorghum-maize composite flour (red sorghum grain, of a tannin-free variety, ‘Alpha’) that slowly digestible starch increased with increasing sorghum level and decreased as the barrel temperature increased. The barrel consisted of four independent zones, electrically heated and cooled by water. The barrel temperature zone profiles used were 90/95/100/120 °C and 90/105/125/150 °C.
Duodu et al. (Citation2002) reported that when a cooking process was performed in sorghum and in maize, a lower digestibility of the protein was observed in sorghum due to the formation of enzyme-resistant, disulfide-bonded oligomers. In another study, application of high-temperature, high-pressure extrusion led to protein-polyphenolic interactions that resulted in lower extractability of phenolic compounds (Dlamini, Taylor, and Rooney Citation2007). Therefore, phenolic compounds (mainly tannins) may interact with proteins to form a complex, which may lead to lower digestibility (Duodu et al. Citation2002, Citation2003; Taylor et al. Citation2007).
Phytate may also be another factor reducing sorghum protein digestibility (Carnovale, Lugaro, and Lombardi-Boccia Citation1988). Sorghum starch and protein bodies are closely associated with each other as large spherical protein bodies surrounding starch granules. By cooking and gelatinizing the starch, the accessibility of enzymes to the protein bodies is reported to be reduced, resulting in lower protein digestibility (Shull et al. Citation1990). Other researchers also postulate that low protein digestibility might be due to a significant increase in the level of resistant starch in cooled cooked porridge compared to sorghum flour. The authors assumed that protein may form a complex with resistant starch, making the protein less available for enzyme attack (Knudsen et al. Citation1988).
Arbab and El Tinay (Citation1997) studied the impact of adding reducing agents (sodium bisulfite and ascorbic acid) on cooked and un-cooked low-tannin and high-tannin sorghum. The results showed an increase in in vitro protein digestibility with the addition of reducing agent; however, the high-tannin sorghum had lower digestibility than the low-tannin sorghum.
The effect of heat treatment on sorghum flour used to make sorghum-based gluten-free bread and cake was studied by Marston, Khouryieh, and Aramouni (Citation2016). For both products, dry heating the sorghum flour before application for processing resulted in a greater number and volume of cells per slice area than in the unheated control. In addition, the acceptability scores for both the bread and cake made with heat-treated sorghum flour were higher than the control samples.
Benhur et al. (Citation2015) reported that when different proportions of sorghum and wheat semolina were used to make pasta, applying a low temperature short-time (LTST) extruder process, optimal sensory tests and nutritional data were obtained when rates of 50/50, 60/40 and also 70/30 (sorghum/wheat semonila) was used.
The effects of extrusion temperature and grit moisture content on the physico-chemical and textural properties of whole-grain red sorghum have been investigated (Llopart et al. Citation2014). Water sorption and water solubility of the extrudate were shown to have a direct relationship to temperature and an inverse relationship to moisture. The results indicated that the highest degree of cooking was obtained at 200 °C with 14% moisture; however, the product that showed the greatest expansion properties was from extrusion at 182 °C with 14% moisture.
The impacts of sorghum milling extraction rate (60, 80 and 100%), pin milling (no pin milling, low and high speed) and particle size of flour on the quality of gluten-free bread have been investigated by Trappey et al. (Citation2015). Higher specific volume, lower crumb firmness and better crumb properties were found for the 60% extraction rate compared to the higher rates.
Thermal processing methods have been reported to either increase or decrease the level of phenolic compounds and antioxidant activity in sorghum grain products. The variation between studies in the changes in levels of phenolic compounds and antioxidant activity after thermal processing are mainly due to polymerization and oxidation, thermal degradation, depolymerization, release of bound phenolics or the formation of Maillard reaction by-products (Awika et al. Citation2003; Chiremba, Taylor, and Duodu Citation2009; Dlamini, Taylor, and Rooney Citation2007; Emmambux and Taylor Citation2003; Itagi et al. Citation2012; Towo, Svanberg, and Ndossi Citation2003; Wu et al. Citation2013).
Morais Cardoso et al. (Citation2014) studied the impact of dry and wet heating on sorghum antioxidant activity, vitamin E and phenolic compounds. Dry heat increased the level of vitamin E and reduced the abundance of carotenoids but had no impact on phenolic compounds, while wet heating resulted in a decrease in the levels of all antioxidants except the carotenoids.
The operating parameters for microwave puffing to produce a sorghum-soy ready-to-eat snack (90/10) have been optimized (Pawar et al. Citation2014). The optimal process was to apply convective heating at 210 °C for 240 s, then microwave heating at 80% power using a 1350-W oven for 60 s. In another study, the effects of moisture level, microwave power and time on puffed sorghum quality were that the grain with 21% moisture exposed to 100% microwave power for 3 min had the highest puffing yield, expansion ratio and flake size volume (Sharma, Champawat, and Mudgal Citation2014).
Possible future trends for sorghum
There are general challenges that influence the future production, demand, and use of sorghum as compared to other cereal crops such as corn, wheat and barley. One of the main challenges for farmers around the globe is adaptation to climate change and its impact on crop farming and production. Sorghum has high tolerance to drought and heat compared to many other kinds of cereal. Therefore, the selection of sorghum to grow in regions that are experiencing an increase in drought and heat will probably be a valuable option (Akinseye et al. Citation2020). To help farmers and food producers to have access and grow sorghum cultivars that give the best yield with high crop nutritional quality, several different approaches have been taken into account.
Breeding new varieties of cereal crops that are tolerant to climate change is another approach. Therefore, research to explore and exploit sorghum genetic variation by looking at sorghum’s wild relatives that potentially survived for centuries under severe climate conditions is of interest to many researchers (Mace et al. Citation2021). Future research into the genetic and physiological mechanisms underlying drought and heat tolerance will potentially avoid adverse effects on early vegetative, pre-, and post-anthesis on sorghum growth, which lead to reductions in yield (Akman, Zhang, and Ejeta Citation2021; Chiluwal et al. Citation2018).
The other approach is to use or incorporate sorghum into a range of food products, including most staple foods, especially in developing countries where sorghum has a comparative advantage. This can potentially help to improve the nutritional values of the product and play a preventive role against several diseases such as diabetes (Sharma and Arora Citation2020).
The other recent approach is to use sorghum to make degradable packaging and films to replace synthetic polymers. Mehboob et al. (Citation2020) reported research on cross-linking and/or acetylation of sorghum starch that can create edible films to pack food. Sorghum starch is also used as shell material in combination with gum arabic to suppresses the degradation of flavoring ingredients in nutmeg oleoresin (Arshad, Ali, and Hasnain Citation2020).
The most challenging approach is the commercialization of sorghum-based food products for nontraditional consumers in Western countries. Understanding the supply chain limitations and the reason behind poor collaborations between key stakeholders (i.e., researchers, food manufacturers, marketers, government agencies, dietitians and consumers) is necessary to increase sorghum awareness (Stefoska-Needham and Tapsell Citation2020).
Conclusions
Global climate variability, water scarcity, increased frequency of heat waves and longer periods of drought will require recognizing sorghum as a valuable and resilient crop alternative for sustainable food production. Progress has already been made to use sorghum whole grain in functional foods, as well as incorporating sorghum whole grain or components in foods to improve quality and functionality for nontraditional consumers. However, the work is still in the early stages and further research is required.
With the collaboration of researchers, farmers, food manufacturers, and other stakeholders, a successful new era for sorghum-based food products is achievable.
Disclosure statement
No potential conflict of interest was reported by the authors.
Additional information
Funding
References
- Adebo, O. A., P. B. Njobeh, and E. Kayitesi. 2018. Fermentation by Lactobacillus fermentum strains (singly and in combination) enhances the properties of ting from two whole grain sorghum types. Journal of Cereal Science 82:49–56. doi: 10.1016/j.jcs.2018.05.008.
- Adebowale, O., J. Taylor, and H. de Kock. 2020. Stabilization of wholegrain sorghum flour and consequent potential improvement of food product sensory quality by microwave treatment of the kernels. LWT - Food Science and Technology 132:109827. doi: 10.1016/j.lwt.2020.109827.
- Ahmed, S. B., S. a Mahgoub, and B. E. Babiker. 1996. Changes in tannin and cyanide contents and diastic activity during germination and the effect of traditional processing on cyanide content of sorghum cultivars. Food Chemistry 56 (2):159–62. doi: 10.1016/0308-8146(95)00157-3.
- Ai, Y. 2013. Structures, properties and digestibility of resistant starch. PhD Thesis., Iowa State University.
- Ajiboye, T. O., G. A. Iliasu, A. O. Adeleye, F. A. Abdussalam, S. A. Akinpelu, S. M. Ogunbode, S. O. Jimoh, and O. B. Oloyede. 2014. Nutritional and antioxidant dispositions of sorghum/millet-based beverages indigenous to Nigeria. Food Science & Nutrition 2 (5):597–604. doi: 10.1002/fsn3.140.
- Akinseye, F. M., H. A. Ajeigbe, P. C. S. Traore, S. O. Agele, B. Zemadim, and A. Whitbread. 2020. Improving sorghum productivity under changing climatic conditions: A modelling approach. Field Crops Research 246:107685. doi: 10.1016/j.fcr.2019.107685.
- Akman, H., C. Zhang, and G. Ejeta. 2021. Physio-morphological, biochemical, and anatomical traits of drought-tolerant and susceptible sorghum cultivars under pre- and post-anthesis drought. Physiologia Plantarum 172 (2):912–21. doi: 10.1111/ppl.13242.
- Ali, S., M. Merra, and N. Malleshi. 2003. :169–87. Processing of sorghum and pearl millet for promoting wider utilization for food. In Alternative uses of sorghum and pearl millet in Asia. Patacheru, AP: ICRISAT.
- Al-Rabadi, G. J., P. J. Torley, B. A. Williams, W. L. Bryden, and M. J. Gidley. 2011. Particle size of milled barley and sorghum and physico-chemical properties of grain following extrusion. Journal of Food Engineering 103 (4):464–72. doi: 10.1016/j.jfoodeng.2010.11.016.
- Amoako, D., and J. M. Awika. 2016. Polyphenol interaction with food carbohydrates and consequences on availability of dietary glucose. Current Opinion in Food Science 8:14–8. doi: 10.1016/j.cofs.2016.01.010.
- Amoura, H., H. Mokrane, and B. Nadjemi. 2020. Effect of wet and dry milling on the functional properties of whole sorghum grain flour and kafirin. Journal of Food Science and Technology 57 (3):1100–9. doi: 10.1007/s13197-019-04145-2.
- Anglani, C. 1998. Sorghum for human food–a review. Plant Foods for Human Nutrition (Dordrecht, Netherlands) 52 (1):85–95. doi: 10.1023/A:1008065519820.
- Anukam, K. C., and G. Reid. 2009. African traditional fermented foods and probiotics. Journal of Medicinal Food 12 (6):1177–84. doi: 10.1089/jmf.2008.0163.
- Arbab, M. E., and A. H. El Tinay. 1997. Effect of cooking and treatment with sodium bisulphite or ascorbic acid on the in vitro protein digestibility of two sorghum cultivars. Food Chemistry 59 (3):339–43. doi: 10.1016/S0308-8146(96)00106-9.
- Arshad, H., T. M. Ali, and A. Hasnain. 2020. Comparative study on efficiency of nutmeg microencapsulation (freeze-drying method) using native and OSA sorghum starch as wall materials in combination with gum arabic. Cereal Chemistry 97 (3):589–600. doi: 10.1002/cche.10275.
- Awika, J. M. 2003. Antioxidant properties of sorghum. PhD Thesis., Texas A & M University: College Station, TX.
- Awika, J. M. 2011. Sorghum flavonoids: Unusual compounds with promising implications for health. ACS Symposium Series, 1089, 171–200. doi: 10.1021/bk-2011-1089.ch009.
- Awika, J. M. 2017. Sorghum: Its unique nutritional and health-promoting attributes. Gluten-free ancient grains: Cereals, pseudocereals, and legumes: Sustainable, nutritious, and health-promoting foods for the 21st Century. Cambridge: Elsevier Ltd. doi: 10.1016/B978-0-08-100866-9.00003-0.
- Awika, J. M., L. Dykes, L. Gu, L. W. Rooney, and R. L. Prior. 2003. Processing of sorghum (Sorghum bicolor) and sorghum products alters procyanidin oligomer and polymer distribution and content. Journal of Agricultural and Food Chemistry 51 (18):5516–21. doi: 10.1021/jf0343128.
- Awika, J. M., and L. W. Rooney. 2004. Sorghum phytochemicals and their potential impact on human health. Phytochemistry 65 (9):1199–221. doi: 10.1016/j.phytochem.2004.04.001.
- Axtell, J. D., A. W. Kirleis, M. M. Hassen, N. D'Croz Mason, E. T. Mertz, and L. Munck. 1981. Digestibility of sorghum proteins. Proceedings of the National Academy of Sciences of the United States of America 78 (3):1333–5. doi: 10.1073/pnas.78.3.1333.
- Bai, M., and J. Liu. 2010. Sorghum, coarse-grain healthy food [in Chinese]. Oriental Diet-Therapy and Health Care 60:10.
- Barros, F., J. Awika, and L. W. Rooney. 2014. Effect of molecular weight profile of sorghum proanthocyanidins on resistant starch formation. Journal of the Science of Food and Agriculture 94 (6):1212–7. doi: 10.1002/jsfa.6400.
- Barros, F., J. M. Awika, and L. W. Rooney. 2012. Interaction of tannins and other sorghum phenolic compounds with starch and effects on in vitro starch digestibility. Journal of Agricultural and Food Chemistry 60 (46):11609–17. doi: 10.1021/jf3034539.
- Belton, P. S., I. Delgadillo, N. G. Halford, and P. R. Shewry. 2006. Kafirin structure and functionality. Journal of Cereal Science 44 (3):272–86. doi: 10.1016/j.jcs.2006.05.004.
- Benhur, D. R., G. Bhargavi, K. Kalpana, A. D. Vishala, K. N. Ganapathy, and J. V. Patil. 2015. Development and standardization of sorghum pasta using extrusion technology. Journal of Food Science and Technology 52 (10):6828–33. doi: 10.1007/s13197-015-1801-8.
- Beta, T., L. W. W. Rooney, L. T. T. Marovatsanga, and J. R. N. R. N. Taylor. 2000. Effect of chemical treatments on polyphenols and malt quality in sorghum. Journal of Cereal Science 31 (3):295–302. doi: 10.1006/jcrs.2000.0310.
- Birt, D. F., T. Boylston, S. Hendrich, J.-L. Jane, J. Hollis, L. Li, J. McClelland, S. Moore, G. J. Phillips, M. Rowling, et al. 2013. Resistant starch: Promise for Improving Human health. Advances in Nutrition (Bethesda, Md.) 4 (6):587–601. doi: 10.3945/an.113.004325.
- Boling, M., and N. Eisener. 1981. Bogobe: Sorghum porridge of Botswana. In International Symposium on Sorghum Quality. Patancheru, India: ICRISAT Center.
- Boz, H. 2016. Ferulic acid in cereals - A review. Czech Journal of Food Sciences 33 (No. 1):1–7. doi: 10.17221/401/2014-CJFS.
- Bralley, E., P. Greenspan, J. L. Hargrove, and D. K. Hartle. 2008. Inhibition of hyaluronidase activity by select sorghum brans. Journal of Medicinal Food 11 (2):307–12. doi: 10.1089/jmf.2007.547.
- Buitimea-Cantúa, N. E., P. I. Torres-Chávez, A. I. Ledesma-Osuna, B. Ramírez-Wong, R. M. Robles-Sánchez, and S. O. Serna-Saldívar. 2013. Effect of defatting and decortication on distribution of fatty acids, phenolic and antioxidant compounds in sorghum (Sorghum bicolor) bran fractions. International Journal of Food Science and Technology 48 (10):2166–75. doi: 10.1111/ijfs.12201.
- Burdette, A., P. L. Garner, E. P. Mayer, J. L. Hargrove, D. K. Hartle, and P. Greenspan. 2010. Anti-inflammatory activity of select sorghum (Sorghum bicolor) brans. Journal of Medicinal Food 13 (4):879–87. doi: 10.1089/jmf.2009.0147.
- Bvochora, J. M., H. Danner, H. Miyafuji, R. Braun, and R. Zvauya. 2005. Variation of sorghum phenolic compounds during the preparation of opaque beer. Process Biochemistry 40 (3-4):1207–13. doi: 10.1016/j.procbio.2004.04.005.
- Bvochora, J. M., J. D. Reed, J. S. Read, and R. Zvauya. 1999. Effect of fermentation processes on proanthocyanidins in sorghum during preparation of Mahewu, a non-alcoholic beverage. Process Biochemistry 35 (1-2):21–5. doi: 10.1016/S0032-9592(99)00027-8.
- Cardoso, L. d M., T. A. Montini, S. S. Pinheiro, V. A. V. Queiroz, H. M. Pinheiro-Sant'Ana, H. S. D. Martino, and A. V. B. Moreira. 2014. Effects of processing with dry heat and wet heat on the antioxidant profile of sorghum. Food Chemistry 152:210–7. doi: 10.1016/j.foodchem.2013.11.106.
- Carnovale, E., E. Lugaro, and G. Lombardi-Boccia. 1988. Phytic acid in faba bean and pea: Effect on protein availability. Cereal Chemistry 65:114–7.
- Carr, T. P., C. L. Weller, V. L. Schlegel, S. L. Cuppett, D. M. Guderian, and K. R. Johnson. 2005. Grain sorghum lipid extract reduces cholesterol absorption and plasma non-HDL cholesterol concentration in Hamsters. The Journal of Nutrition 135 (9):2236–40. doi: 10.1093/jn/135.9.2236.
- Cecil, J. E. 1992. Semiwet milling of red sorghum: A review. In Utilization of sorghum and millets, edited by M. Gomez, L. House, L. Rooney, and D. Dendy, 23–6. Patancheru, India: ICRISAT.
- Celis, L., W. L. Rooney, and C. M. McDonough. 1996. A ready to eat breakfast cereal from food grade sorghum. Cereal Chemistry 73:108–14.
- Chávez, D., J. Ascheri, A. Martins, C. Carvalho, C. Bernardo, and A. Teles. 2018. Sorgo, un cereal alternativo para productos sin gluten. Revista Chilena De Nutrición 45 (2):169–77. doi: 10.4067/S0717-75182018000300169.
- Chen, X.-F., H.-T. Wu, G.-G. Tan, Z.-Y. Zhu, and Y.-F. Chai. 2011. Liquid chromatography coupled with time-of-flight and ion trap mass spectrometry for qualitative analysis of herbal medicines. Journal of Pharmaceutical Analysis 1 (4):235–45. doi: 10.1016/j.jpha.2011.09.008.
- Chiluwal, A., R. Bheemanahalli, R. Perumal, A. R. Asebedo, E. Bashir, A. Lamsal, D. Sebela, N. J. Shetty, and S. V. Krishna Jagadish. 2018. Integrated aerial and destructive phenotyping differentiates chilling stress tolerance during early seedling growth in sorghum. Field Crops Research 227:1–10. doi: 10.1016/j.fcr.2018.07.011.
- Chiremba, C., J. R. N. Taylor, and K. G. Duodu. 2009. Phenolic content, antioxidant activity, and consumer acceptability of sorghum cookies. Cereal Chemistry Journal 86 (5):590–4. doi: 10.1094/CCHEM-86-5-0590.
- Chirinos, R., I. Betalleluz-Pallardel, A. Huamán, C. Arbizu, R. Pedreschi, and D. Campos. 2009. HPLC-DAD characterisation of phenolic compounds from Andean oca (Oxalis tuberosa Mol.) tubers and their contribution to the antioxidant capacity. Food Chemistry 113 (4):1243–51. doi: 10.1016/j.foodchem.2008.08.015.
- Chung, I.-M., M.-a. Yeo, S.-J. Kim, M.-J. Kim, D.-S. Park, and H.-I. Moon. 2011. Antilipidemic activity of organic solvent extract from Sorghum bicolor on rats with diet-induced obesity. Human & Experimental Toxicology 30 (11):1865–8. doi: 10.1177/0960327110390066.
- Chung, I.-M., M.-J. Kim, D.-S. Park, and H.-I. Moon. 2011. Inhibition effects of the classical pathway complement of three Sorghum bicolor from South Korea. Immunopharmacology and Immunotoxicology 33 (3):447–9. doi: 10.3109/08923973.2010.532804.
- Collar, C. 2017. Significance of heat-moisture treatment conditions on the pasting and gelling behaviour of various starch-rich cereal and pseudocereal flours. Food Science and Technology International = Ciencia y Tecnologia de Los Alimentos Internacional 23 (7):623–36. doi: 10.1177/1082013217714671.
- Contreras-Jiménez, B., A. Del Real, B. M. Millan-Malo, M. Gaytán-Martínez, E. Morales-Sánchez, and M. E. Rodríguez-García. 2019. Physicochemical changes in barley starch during malting. Journal of the Institute of Brewing 125 (1):10–7. doi: 10.1002/jib.547.
- Dadnia, M. R., M. Mojaddam, and A. Ayran. 2018. Role of copper on physiological parameters and salt tolerance in sweet sorghum (Sorghum bicolor L.) Cultivars. Journal of Crop Nutrition Science 4 (1):1–14.
- Dahir, M., K.-X. Zhu, X.-N. Guo, W. Aboshora, and W. Peng. 2015. Possibility to utilize sorghum flour in a modern bread making industry. Journal of Academia and Industrial Research 4 (4):128–35.
- Dai, J., and R. J. Mumper. 2010. Plant phenolics: Extraction, analysis and their antioxidant and anticancer properties. Review. Molecules (Basel, Switzerland) 15 (10):7313–−52. doi: 10.3390/molecules15107313.
- Devi, N. L., S. Shobha, X. Tang, S. A. Shaur, H. Dogan, and S. Alavi. 2013. Development of protein-rich sorghum-based expanded snacks using extrusion technology. International Journal of Food Properties 16 (2):263–76. doi: 10.1080/10942912.2011.551865.
- Diaz Napal, G. N., M. T. Defago, G. R. Valladares, and S. M. Palacios. 2010. Response of Epilachna paenulata to two flavonoids, pinocembrin and quercetin, in a comparative study. Journal of Chemical Ecology 36 (8):898–904. doi: 10.1007/s10886-010-9823-1.
- Dicko, M. H., H. Gruppen, O. C. Zouzouho, A. S. Traoré, W. J. H. Van Berkel, and A. G. J. Voragen. 2006. Effects of germination on the activities of amylases and phenolic enzymes in sorghum varieties grouped according to food end-use properties. Journal of the Science of Food and Agriculture 86 (6):953–63. doi: 10.1002/jsfa.2443.
- Djameh, C., F. K. Saalia, E. Sinayobye, A. Budu, G. Essilfie, H. Mensah-Brown, … S. Sefa-Dedeh. 2015. Optimization of the sorghum malting process for pito production in Ghana. Journal of the Institute of Brewing 121 (1):106–12. doi: 10.1002/jib.191.
- Dlamini, N. R., J. R. N. Taylor, and L. W. Rooney. 2007. The effect of sorghum type and processing on the antioxidant properties of African sorghum-based foods. Food Chemistry 105 (4):1412–9. doi: 10.1016/j.foodchem.2007.05.017.
- Dunn, K. L., L. Yang, A. Girard, S. Bean, and J. M. Awika. 2015. Interaction of sorghum tannins with wheat proteins and effect on in vitro starch and protein digestibility in a baked product matrix. Journal of Agricultural and Food Chemistry 63 (4):1234–41. doi: 10.1021/jf504112z.
- Duodu, K. G., A. Nunes, I. Delgadillo, M. L. Parker, E. N. C. Mills, P. S. Belton, and J. R. N. Taylor. 2002. Effect of grain structure and cooking on sorghum and maize in vitro protein digestibility. Journal of Cereal Science 35 (2):161–74. doi: 10.1006/jcrs.2001.0411.
- Duodu, K. G., J. R. N. Taylor, P. S. Belton, and B. R. Hamaker. 2003. Factors affecting sorghum protein digestibility. Journal of Cereal Science 38 (2):117–31. doi: 10.1016/S0733-5210(03)00016-X.
- Duressa, D., D. Weerasoriya, S. R. Bean, M. Tilley, and T. Tesso. 2018. Genetic basis of protein digestibility in grain sorghum. Crop Science 58 (6):2183–99. doi: 10.2135/cropsci2018.01.0038.
- Dykes, L., and L. W. Rooney. 2006. Sorghum and millet phenols and antioxidants. Journal of Cereal Science 44 (3):236–51. doi: 10.1016/j.jcs.2006.06.007.
- Dykes, L., G. C. Peterson, W. L. Rooney, and L. W. Rooney. 2011. Flavonoid composition of lemon-yellow sorghum genotypes. Food Chemistry 128 (1):173–9. doi: 10.1016/j.foodchem.2011.03.020.
- Dykes, L., L. Seitz, W. Rooney, and L. Rooney. 2009. Flavonoid composition of red sorghum genotypes. Food Chemistry 116 (1):313–7. doi: 10.1016/j.foodchem.2009.02.052.
- Dykes, L., W. L. Rooney, and L. W. Rooney. 2013. Evaluation of phenolics and antioxidant activity of black sorghum hybrids. Journal of Cereal Science 58 (2):278–83. doi: 10.1016/j.jcs.2013.06.006.
- Earp, C. F., C. M. McDonough, and L. W. Rooney. 2004. Microscopy of pericarp development in the caryopsis of Sorghum bicolor (L.) Moench. Journal of Cereal Science 39 (1):21–7. doi: 10.1016/S0733-5210(03)00060-2.
- Egbujie, A., and J. Okoye. 2019. Quality characteristics of complementary foods formulated from Sorghum, African Yam Bean and Crayfish flours. Science World Journal 14 (2):16–22.
- Elkhalifa, A., A. Ali, and A. El-Tinay. 2007. Fermented sorghum foods of Sudan – A review. Journal of Food Science and Technology 44:343–9.
- Elmaki, H. B., E. E. Babiker, A. H. E. Tinay, and A. H. El Tinay. 1999. Changes in chemical composition, grain malting, starch and tannin contents and protein digestibility during germination of sorghum cultivars. Food Chemistry 64 (3):331–6. doi: 10.1016/S0308-8146(98)00118-6.
- Emmambux, N. M., and J. R. N. Taylor. 2003. Sorghum kafirin interaction with various phenolic compounds. Journal of the Science of Food and Agriculture 83 (5):402–7. doi: 10.1002/jsfa.1379.
- Eze, C., G. Okafor, E. Omah, and C. Azuka. 2020. Micronutrients, antinutrients composition and sensory properties of extruded snacks made from sorghum and charamenya flour blends. African Journal of Food Science 14 (1):25–31. doi: 10.5897/AJFS2019.1882.
- Ezeogu, L. I., K. G. Duodu, and J. R. N. Taylor. 2005. Effects of endosperm texture and cooking conditions on the in vitro starch digestibility of sorghum and maize flours. Journal of Cereal Science 42 (1):33–44. doi: 10.1016/j.jcs.2005.02.002.
- FAO. 1995. Food and Agriculture Organization. Sorghum and millet in human nutrition. FAO Food and Nutrition Series. ISBN 92-5-103381-1.
- Farrar, J., D. Hartle, J. Hargrove, and P. Greenspan. 2008. A novel nutraceutical property of select sorghum (Sorghum bicolor) brans: Inhibition of protein glycation. Phytotherapy Research: PTR 22 (8):1052–6. doi: 10.1002/ptr.2431.
- Fu, L., B. T. Xu, X. R. Xu, R. Y. Gan, Y. Zhang, E. Q. Xia, and H. B. Li. 2011. Antioxidant capacities and total phenolic contents of 62 fruits. Food Chemistry 129 (2):345–50. doi: 10.1016/j.foodchem.2011.04.079.
- Glew, R. H., D. J. Vanderjagt, C. Lockett, L. E. Grivetti, G. C. Smith, A. Pastuszyn, and M. Millson. 1997. Amino acid, fatty acid, and mineral composition of 24 indigenous plants of Burkina Faso. Journal of Food Composition and Analysis 10 (3):205–17. doi: 10.1006/jfca.1997.0539.
- Gomez, M. I. 1993. Comparative evaluation and optimization of a milling system for small grains. In Cereal science and technology: Impact on a changing Africa, edited by J. R. N. Taylor, P. Randall, and J. Viljoen, 463–74. Pretoria: The CSIR.
- Gomez-Cordoves, C., B. Bartolome, W. Vieira, and V. M. Virador. 2001. Effects of wine phenolics and sorghum tannins on tyrosinase activity and growth of melanoma cells. Journal of Agricultural and Food Chemistry 49 (3):1620–4. doi: 10.1021/jf001116h.
- Gomez-Martinez, R., and A. Culham. 2000. Phylogeny of the subfamily Panicoideae with emphasis on the tribe Paniceae: Evidence from the trnL-F cpDNA region: in Grasses: Systematics and evolution, edited by S. Jacobs and J. Everett, 136–40. Victoria: CSIRO Publishing.
- Goyal, M., R. S. Sohu, R. Bhardwaj, B. S. Gill, and M. Goyal. 2020. Genetic variance and predicted response for three types of recurrent selection procedures in forage sorghum [Sorghum bicolor (L.) moench]. Range Management and Agroforestry 41 (1):43–51.
- Guindo, D., F. Davrieux, N. Teme, M. Vaksmann, M. Doumbia, G. Fliedel, D. Bastianelli, J.-L. Verdeil, C. Mestres, M. Kouressy, et al. 2016. Pericarp thickness of sorghum whole grain is accurately predicted by NIRS and can affect the prediction of other grain quality parameters. Journal of Cereal Science 69:218–27. doi: 10.1016/j.jcs.2016.03.008.
- Hamaker, B. R., A. A. Mohamed, J. E. Habben, C. P. Huang, and B. A. Larkins. 1995. An efficient procedure for extracting maize and sorghum kernels protein. Cereal Chemistry 72 (6):583–8.
- Hargrove, J. L., P. Greenspan, D. K. Hartle, and C. Dowd. 2011. Inhibition of aromatase and α-amylase by flavonoids and proanthocyanidins from Sorghum bicolor bran extracts. Journal of Medicinal Food 14 (7-8):799–807. doi: 10.1089/jmf.2010.0143.
- Hibberd, C. A., D. G. Wagner, R. L. Schemm, E. D. Mitchell, D. E. Weibel, and R. L. Hintz. 1982. Digestibility characteristics of isolated starch from sorghum and corn grains. Journal of Animal Science 55 (6):1490–7. doi: 10.2527/jas1982.5561490x.
- Hoseney, R. 1994. Principles of cereal science and technology, 2nd ed. St. Paul, MN: American Association of Cereal Chemistry.
- Hussain, S. A., F. C. Garg, and D. Pal. 2014. Effect of different preservative treatments on the shelf-life of sorghum malt based fermented milk beverage. Journal of Food Science and Technology 51 (8):1582–7. doi: 10.1007/s13197-012-0657-4.
- Iqbal, Z., M. Munir, M. Khan, M. Akhtar, I. Javed, and O. Of. 2001. In Vitro Inhibitory Effects of Sorghum bicolor on Hatching and Moulting of Haemonchus contortus Eggs. Prospects 3:451–3.
- Itagi, H. N., B. V. R. S. Rao, P. A. Jayadeep, and V. Singh. 2012. Functional and antioxidant properties of ready-to-eat flakes from various cereals including sorghum and millets. Quality Assurance and Safety of Crops & Foods 4 (3):126–33. doi: 10.1111/j.1757-837X.2012.00136.x.
- Izquierdo, I., and I. D. Godwin. 2005. Molecular characterization of a novel methionune-rich δ-kafirin seed storage protein gene in sorghum (Sorghum bicolor L.). Cereal Chemistry Journal 82 (6):706–10. doi: 10.1094/CC-82-0706.
- Jambunathan, R., E. Mertz, and J. Axtell. 1975. Fractionation of soluble proteins of high lysine and normal sorghum grain. Cereal Chemistry 52:119–21.
- Joe White Malting System. 2002. Newmalt micromalting operations manual. Adelaide: Joe White Maltings Pty. Limited.
- Kalui, C., J. Mathara, and P. Kutima. 2010. Probiotic potential of spontaneously fermented cereal based foods – A review. African Journal of Biotechnology 9:2490–8.
- Kaufman, R. C., T. J. Herald, S. R. Bean, J. D. Wilson, and M. R. Tuinstra. 2013. Variability in tannin content, chemistry and activity in a diverse group of tannin containing sorghum cultivars. Journal of the Science of Food and Agriculture 93 (5):1233–41. doi: 10.1002/jsfa.5890.
- Kayodé, A. P. P., J. D. Hounhouigan, and M. J. R. Nout. 2007. Impact of brewing process operations on phytate, phenolic compounds and in vitro solubility of iron and zinc in opaque sorghum beer. LWT - Food Science and Technology 40 (5):834–41. doi: 10.1016/j.lwt.2006.04.001.
- Kennedy, D. O., and E. L. Wightman. 2011. Herbal extracts and phytochemicals: Plant secondary metabolites and the enhancement of human brain function. Advances in Nutrition (Bethesda, MD) 2 (1):32–50. doi: 10.3945/an.110.000117.
- Khan, I., A. M. Yousif, S. K. Johnson, and S. Gamlath. 2014. Effect of sorghum flour addition on in vitro starch digestibility, cooking quality, and consumer acceptability of durum wheat pasta. Journal of Food Science 79 (8):S1560–S1567. doi: 10.1111/1750-3841.12542.
- Khan, I., A. Yousif, S. K. Johnson, and S. Gamlath. 2013. Effect of sorghum flour addition on resistant starch content, phenolic profile and antioxidant capacity of durum wheat pasta. Food Research International 54 (1):578–86. doi: 10.1016/j.foodres.2013.07.059.
- Khoddami, A. 2016. Phenolic compounds in grains of Australian-Grown sorghums : Quantitative analyses including impacts of malting and effects on broiler nutrition. Sydney: University of Sydney.
- Khoddami, A., H. H. Truong, S. Y. Liu, T. H. Roberts, and P. H. Selle. 2015. Concentrations of specific phenolic compounds in six red sorghums influence nutrient utilisation in broiler chickens. Animal Feed Science and Technology 210:190–9. doi: 10.1016/j.anifeedsci.2015.09.029.
- Khoddami, A., M. Mohammadrezaei, and T. H. Roberts. 2017. Effects of sorghum malting on colour, major classes of phenolics and individual anthocyanins. Molecules 22 (10):1713–7. doi: 10.3390/molecules22101713.
- Kingamkono, R., E. Sjögren, U. Svanberg, and B. Kaijser. 1995. Inhibition of different strains of enteropathogens in a lactic-fermenting cereal gruel. Doktorsavhandlingar Vid Chalmers Tekniska Hogskola 11 (3):299–303. doi: 10.1007/BF00367103.
- Knudsen, K. E. B., A. W. Kirleis, B. O. Eggum, and L. Munck. 1988. Carbohydrate composition and nutritional quality for rats of sorghum tô prepared from decorticated white and whole grain red flour. The Journal of Nutrition 118 (5):588–97. doi: 10.1093/jn/118.5.588.
- Kobue-Lekalake, R. I., J. R. Taylor, and H. De-Kock. 2007. Effects of phenolics in sorghum grain on its bitterness, astringency and other sensory properties. Revista de Fitoterapia 87:1940–8. doi: 10.1002/jsfa.
- Lakshmi, K. B., and V. Vimala. 1996. Hypoglycemic effect of selected sorghum recipes. Nutrition Research 16 (10):1651–8. doi: 10.1016/0271-5317(96)00184-4.
- Lawan, H. K., M. Badau, E. C. Chibuzo, and F. M. Adam. 2020. Nutritional analysis of varied processing and complementary food formulations with sorghum, cowpea and carrot. American Journal of Food and Nutrition 8:47–53. doi: 10.12691/ajfn-8-2-5.
- Lemilioglu-Austin, D. 2014. Sorghum: Obliging alternative and ancient grain. Cereal Foods World 59:12–20.
- Lemlioglu-Austin, D., N. D. Turner, C. M. McDonough, and L. W. Rooney. 2012. Effects of sorghum [Sorghum bicolor (L.) moench] crude extracts on starch digestibility, estimated glycemic index (EGI), and resistant starch (RS) contents of porridges. Molecules (Basel, Switzerland) 17 (9):11124–38. doi: 10.3390/molecules170911124.
- Licata, R., J. Chu, S. Wang, R. Coorey, A. James, Y. Zhao, and S. Johnson. 2014. Determination of formulation and processing factors affecting slowly digestible starch, protein digestibility and antioxidant capacity of extruded sorghum-maize composite flour. International Journal of Food Science & Technology 49 (5):1408–19. doi: 10.1111/ijfs.12444.
- Lim, T. K. 2012. Edible medicinal and non-medicinal plants. Edible Medicinal and Non-Medicinal Plants 2:867–78.
- Lim, T. K. 2013. Sorghum. In Edible medicinal and non-medicinal plants, Vol. 5, 359–84. Dordrecht: Springer. doi: 10.1007/978-94-007-5653-3.
- Liu, S., G. Fox, A. Khoddami, K. Neilson, H. Truong, A. Moss, and P. Selle. 2015. Grain Sorghum: A conundrum for chicken-meat production. Agriculture 5 (4):1224–51. doi: 10.3390/agriculture5041224.
- Llopart, E. E., Drago, S. R. Greef, D. M. De Luis, R. González, R. J. E. Jose E. … R. 2014. Effects of extrusion conditions on physical and nutritional properties of extruded whole grain red sorghum (Sorghum spp). International Journal of Food Sciences and Nutrition 65 (1):34–41. doi: 10.3109/09637486.2013.836737.
- Lubadde, G., J. Ebiyau, C. Aru, J. Andiku, M. Wandulu, and M. Ugen. 2019. Sorghum production handbook for Uganda. Uganda: Resources Research Institute of the National Agricultural Research Organisation (NaSARRI-NARO).
- Mace, E. S., A. W. Cruickshank, Y. Tao, C. H. Hunt, and D. R. Jordan. 2021. A global resource for exploring and exploiting genetic variation in sorghum crop wild relatives. Crop Science 61 (1):150–62. doi: 10.1002/csc2.20332.
- MacLean, W. C., G. López de Romaña, a Gastañaduy, and G. G. Graham. 1983. The effect of decortication and extrusion on the digestibility of sorghum by preschool children. The Journal of Nutrition 113 (10):2071–7. doi: 10.1093/jn/113.10.2071.
- Marston, K., H. Khouryieh, and F. Aramouni. 2016. Effect of heat treatment of sorghum flour on the functional properties of gluten-free bread and cake. LWT - Food Science and Technology 65:637–44. doi: 10.1016/j.lwt.2015.08.063.
- Martino, H. S. D., Tomaz, P. A. Moraes, É. A. Conceicao, L. L. da Oliveria, D. da, S. Queiroz, V. A. V. … Ribeiro S. M. R. 2012. Chemical characterization and size distribution of sorghum genotypes for human consumption Caracterização química e distribuição granulométrica de genótipos de sorgo para alimentação humana. Rev Inst Adolfo Lutz 71 (2):337–44.
- Massey, A. R., L. Reddivari, and J. Vanamala. 2014. The dermal layer of sweet sorghum (Sorghum bicolor) stalk, a byproduct of biofuel production and source of unique 3-Deoxyanthocyanidins, has more antiproliferative and proapoptotic activity than the Pith in p53 variants of HCT116 and colon cancer stem cells. Journal of Agricultural and Food Chemistry 62 (14):3150–9. doi: 10.1021/jf405415u.
- Maxson, E. D., W. B. Fryar, L. W. Rooney, and M. N. Krishnaprasad. 1971. Milling properties of sorghum grain with different proportions of corneous to floury endosperm. Cereal Chemistry 48:478–89.
- Mehboob, S., T. M. Ali, M. Sheikh, and A. Hasnain. 2020. Effects of cross linking and/or acetylation on sorghum starch and film characteristics. International Journal of Biological Macromolecules 155:786–94. doi: 10.1016/j.ijbiomac.2020.03.144.
- Mellen, P. B., T. F. Walsh, and D. M. Herrington. 2008. Whole grain intake and cardiovascular disease: A meta-analysis. Nutrition, Metabolism, and Cardiovascular Diseases: NMCD 18 (4):283–90. doi: 10.1016/j.numecd.2006.12.008.
- Mertz, E. T., M. M. Hassen, C. Cairns-Whittern, a W. Kirleis, L. Tu, and J. D. Axtell. 1984. Pepsin digestibility of proteins in sorghum and other major cereals. Proceedings of the National Academy of Sciences of the United States of America 81 (1):1–2. doi: 10.1073/pnas.81.1.1.
- Mishra, G., D. C. Joshi, D. Mohapatra, and V. B. Babu. 2015. Varietal influence on the microwave popping characteristics of sorghum. Journal of Cereal Science 65:19–24. doi: 10.1016/j.jcs.2015.06.001.
- Mkandawire, N. L., S. A. Weier, C. L. Weller, D. S. Jackson, and D. J. Rose. 2015. Composition, in vitro digestibility, and sensory evaluation of extruded whole grain sorghum breakfast cereals. LWT - Food Science and Technology 62 (1):662–7. doi: 10.1016/j.lwt.2014.12.051.
- Modha, H., and D. Pal. 2011. Optimization of Rabadi-like fermented milk beverage using pearl millet. Journal of Food Science and Technology 48 (2):190–6. doi: 10.1007/s13197-010-0146-6.
- Mohamed, S. K., A. A. A. Ahmed, S. M. Yagi, and A. E. W. Abd Alla. 2009. Antioxidant and antibacterial activities of total polyphenols isolated from Pigmented Sorghum (Sorghum bicolor) Lines. Journal of Genetic Engineering and Biotechnology 7 (1):51–8.
- Moraes, É. A., D. I. G. Natal, V. A. V. Queiroz, R. E. Schaffert, P. R. Cecon, S. O. de Paula, L. d A. Benjamim, S. M. R. Ribeiro, and H. S. D. Martino. 2012. Sorghum genotype may reduce low-grade inflammatory response and oxidative stress and maintains jejunum morphology of rats fed a hyperlipidic diet. Food Research International 49 (1):553–9. doi: 10.1016/j.foodres.2012.07.029.
- Moraes, É. A., V. A. V. Queiroz, R. E. Shaffert, N. M. B. Costa, J. D. Nelson, S. M. R. Ribeiro, and H. S. D. Martino. 2012. In vivo protein quality of new sorghum genotypes for human consumption. Food Chemistry 134 (3):1549–55. doi: 10.1016/j.foodchem.2012.03.079.
- Mukuru, S., J. Mushonga, and D. Murthy. 1981. Sorghum Ugali. In International Symposium on Sorghum Grain Quality. Patacheru, AP.
- Muriu, J. I., E. N. Njoka-Njiru, J. K. Tuitoek, and J. N. Nanua. 2002. Evaluation of sorghum (Sorghum bicolor) as replacent for maize in the diet of growing rabbits. Asian-Australasian Journal of Animal Sciences 15 (4):565–9. doi: 10.5713/ajas.2002.565.
- Nawar, I., H. Clark, R. Pickett, and D. Hegsted. 1970. Protein quality of selected lines of sorghum vulgare for the growing rat. Nutrition Reports International 1:75–81.
- Niernberger, F., and J. R. N. Taylor. 2001. Development of simple, common grain quality standards for sorghum, to faciliate grain trade in Southern Africa.
- Nout, M. J. R. 2009. Rich nutrition from the poorest - Cereal fermentations in Africa and Asia. Food Microbiology 26 (7):685–92. doi: 10.1016/j.fm.2009.07.002.
- Nout, M. J. R., J. G. A. J. Hautvast, F. Van der Haar, W. E. W. Marks, and E. M. Rombouts. 1988. Formulation and microbiological safety of cereal-based weaning foods. In Improving young child feeding in Eastern and Southern Africa: Household-level food technology, edited by D. Alnwick, S. Moses, and O. G. Schmidt, 245–60. Ottawa: International Development Research Centre.
- Nwanguma, B. C., and M. O. Eze. 1996. Changes in the concentrations of the polyphenolic constituents of sorghum during malting and mashing. Journal of the Science of Food and Agriculture 70 (2):162–6. doi: 10.1002/(SICI)1097-0010(199602)70:2<162::AID-JSFA464>3.0.CO;2-2.
- Nwinyi, F. C., and H. O. Kwanashie. 2009. Evaluation of Sorghum bicolor leaf base extract for gastrointestinal effects. African Journal of Biotechnology 8 (21):5985–94.
- Obilana, A. T. 1981. Traditional sorghum foods in Nigeria: Their preparation and quality parameters. In International Symposium on Sorghum Grain Quality. Patacheru, AP.
- Oboh, G., T. L. Akomolafe, and A. O. Adetuyi. 2010. Inhibition of cyclophosphamide-induced oxidative stress in brain by dietary inclusion of red dye extracts from sorghum (Sorghum bicolor) stem. Journal of Medicinal Food 13 (5):1075–80. doi: 10.1089/jmf.2009.0226.
- Ojha, P., R. Adhikari, R. Karki, A. Mishra, U. Subedi, and T. B. Karki. 2018. Malting and fermentation effects on antinutritional components and functional characteristics of sorghum flour. Food Science & Nutrition 6 (1):47–53. doi: 10.1002/fsn3.525.
- Okpala, L. C., and E. C. Okoli. 2014. Development of cookies made with cocoyam, fermented sorghum and germinated pigeon pea flour blends using response surface methodology. Journal of Food Science and Technology 51 (10):2671–7. doi: 10.1007/s13197-012-0749-1.
- Oladiji, A. T., T. O. Jacob, and M. T. Yakubu. 2007. Anti-anaemic potentials of aqueous extract of Sorghum bicolor (L.) Moench stem bark in rats. Journal of Ethnopharmacology 111 (3):651–6. doi: 10.1016/j.jep.2007.01.013.
- Oria, M. P., B. R. Hamaker, and J. M. Shull. 1995. Resistance of sorghum α-, β- and γ-kafirins to pepsin digestion. Journal of Agricultural and Food Chemistry 43 (8):2148–53. doi: 10.1021/jf00056a036.
- Oseguera-Toledo, M. E., B. Contreras-Jiménez, E. Hernández-Becerra, and M. E. Rodriguez-Garcia. 2020. Physicochemical changes of starch during malting process of sorghum grain. Journal of Cereal Science 95:103069. doi: 10.1016/j.jcs.2020.103069.
- Park, J. H., P. Darvin, E. J. Lim, Y. H. Joung, D. Y. Hong, E. U. Park, S. H. Park, S. K. Choi, E.-S. Moon, B. W. Cho, et al. 2012. Hwanggeumchal sorghum induces cell cycle arrest, and suppresses tumor growth and metastasis through JAK2/STAT pathways in breast cancer xenografts. PLoS One 7 (7):e40531. doi: 10.1371/journal.pone.0040531.
- Pawar, S. G., I. L. Pardeshi, P. A. Borkar, and M. R. Rajput. 2014. Optimization of process parameters of microwave puffed sorghum based Ready- to-Eat (RTE) Food. Journal of Ready to Eat Food 1 (2):59–68.
- Pelembe, L. A., C. Erasmus, and J. R. Taylor. 2002. Development of a protein-rich composite sorghum–cowpea instant porridge by extrusion cooking process. LWT - Food Science and Technology 35 (2):120–7. doi: 10.1006/fstl.2001.0812.
- Pontieri, P., G. Mamone, S. De Caro, M. R. Tuinstra, E. Roemer, J. Okot, P. De Vita, D. B. M. Ficco, P. Alifano, D. Pignone, et al. 2013. Sorghum, a healthy and gluten-free food for celiac patients as demonstrated by genome, biochemical, and immunochemical analyses. Journal of Agricultural and Food Chemistry 61 (10):2565–71. doi: 10.1021/jf304882k.
- Porter, L. J., L. N. Hrstich, and B. G. Chan. 1985. The conversion of procyanidins and prodelphinidins to cyanidin and delphinidin. Phytochemistry 25 (1):223–30. doi: 10.1016/S0031-9422(00)94533-3.
- Price, M. L., and L. G. Butler. 1977. Rapid visual estimation and spectrophotometric determination of tannin content of sorghum grain. Journal of Agricultural and Food Chemistry 25 (6):1268–73. doi: 10.1021/jf60214a034.
- Proietti, I., C. Frazzoli, and A. Mantovani. 2015. Exploiting nutritional value of staple foods in the world’s semi-arid areas: risks, benefits, challenges and opportunities of sorghum. Healthcare 3 (2):172–93. doi: 10.3390/healthcare3020172.
- Przybylska-Balcerek, A., J. Frankowski, and K. Stuper-Szablewska. 2019. Bioactive compounds in sorghum. European Food Research and Technology 245 (5):1075–80. doi: 10.1007/s00217-018-3207-0.
- Ratnavathi, C. V., N. Seetharama, and U. D. Chavan. 2008. Dough and roti making qualities of sorghum genotypes. Jowar Samachar 4 (2):2–4.
- Ratnavathi, C. V., and J. V. Patil. 2013. Sorghum utilization as food. Journal of Nutrition & Food Sciences 4 (1):1–8. doi: 10.4172/2155-9600.1000247.
- Ratnavathi, C. V., V. Sailaja, V. V. Komala, and B. Dayakar Rao. 2008. “Delicious sorghum foods'. National Research Centre for Sorghum, Rajendranagar, India. pp 56. ISBN-81-89335-19-7.
- Reichert, R. D., C. G. Youngs, and B. D. Oomah. 1982. Measurement of grain hardness and dehulling quality with a multisample, tangential abrasive dehulling device. In Proceedings of the International Symposium on sorghum grain quality, 186. Patancheru, India: ICRISAT.
- Rhodes, M., and K. Price. 1997. Identification and analysis of plant phenolic antioxidants. European Journal of Cancer Prevention: The Official Journal of the European Cancer Prevention Organisation (ECP) 6 (6):518–21. doi: 10.1097/00008469-199712000-00005.
- Rooney, L. W., and F. Miller. 1982. Variation in the structure and kernel characteristics of sorghum. In International symposium on sorghum grain quality, edited by L. W. Rooney, D. Murty, and J. Mertin, 143–62. Patacheru, AP: ICRISAT.
- Rooney, L., and R. Waniska. 2000. Sorghum food and industrial utilization. In Sorghum: Origin, history, technology and production, edited by C. Smith and R. Frederiksen. New York: John Wiley and Sons, Inc.
- Rooney, L., W. L. Rooney, and S. Serna-Saldivar. 2016. Sorghum. Reference Module in Food Science:1–6. doi: 10.1016/B978-0-08-100596-5.02986-3.
- Rose, D. J., E. Williams, N. L. Mkandawire, C. L. Weller, and D. S. Jackson. 2014. Use of whole grain and refined flour from tannin and non-tannin sorghum (Sorghum bicolor (L.) Moench) varieties in frybread. Food Science and Technology International = Ciencia y Tecnologia de Los Alimentos Internacional 20 (5):333–9. doi: 10.1177/1082013213488380.
- Sang, Y., S. Bean, P. a Seib, J. Pedersen, and Y. Shi. 2008. Structure and functional properties of sorghum starches differing in amylose content. Journal of Agricultural and Food Chemistry 56 (15):6680–5. doi: 10.1021/jf800577x.
- Sawadogo, W. R., Maciuk, A. Banzouzi, J. T. Champy, P. Figadere, B. Guissou, I. Nacoulma P., and O. G. 2012. Mutagenic effect, antioxidant and anticancer activities of six medicinal plants from Burkina Faso. Natural Product Research 26 (6):575–9. doi: 10.1080/14786419.2010.534737.
- Scalbert, A., C. Manach, C. Morand, C. Rémésy, and L. Jiménez. 2005. Dietary polyphenols and the prevention of diseases. Critical Reviews in Food Science and Nutrition 45 (4):287–306. doi: 10.1080/1040869059096.
- Scalbert, A., S. Déprez, I. Mila, A. M. Albrecht, J. F. Huneau, and S. Rabot. 2000. Proanthocyanidins and human health: Systemic effects and local effects in the gut. BioFactors (Oxford, England) 13 (1-4):115–20. doi: 10.1002/biof.5520130119.
- Schwab, C., M. Mastrangelo, A. Corsetti, and M. Gänzle. 2008. Formation of oligosaccharides and polysaccharides by Lactobacillus reuteri LTH5448 and Weissella cibaria 10M in sorghum sourdoughs. Cereal Chemistry Journal 85 (5):679–84. doi: 10.1094/CCHEM-85-5-0679.
- Sedghi, M., A. Golian, P. Soleimani-Roodi, A. Ahmadi, and M. Aami-Azghadi. 2012. Relationship between color and tannin content in sorghum grain: Application of image analysis and Artificial Neural Network. Revista Brasileira de Ciência Avícola 14 (1):57–62. doi: 10.1590/S1516-635X2012000100010.
- Sekwati-Monang, B., and M. G. Gänzle. 2011. Microbiological and chemical characterisation of ting, a sorghum-based sourdough product from Botswana. International Journal of Food Microbiology 150 (2-3):115–21. doi: 10.1016/j.ijfoodmicro.2011.07.021.
- Serna-Saldivar, S., and L. W. Rooney. 1995. Structure and chemistry of sorghum and millets. In Sorghum and millets: Chemistry and technology, edited by D. Dendy, 69–124. St Paul, MN: American Association of Cereal Chemists, Inc.
- Sharanagat, V., R. Suhag, P. Anand, G. Deswal, R. Kumar, A. Chaudhary, L. Singh, O. Singh Kushwah, S. Mani, Y. Kumar, et al. 2019. Physico-functional, thermo-pasting and antioxidant properties of microwave roasted sorghum [Sorghum bicolor (L.) Moench]. Journal of Cereal Science 85:111–9. doi: 10.1016/j.jcs.2018.11.013.
- Sharma, A., and M. Arora. 2020. Development of low glycemic index food products incorporating sorghum. Moringa Oliefera, Nigella Sativa for Type II Diabetic Patients 20 (2):7588–94.
- Sharma, V., P. S. Champawat, and V. D. Mudgal. 2014. Process development for puffing of Sorghum. International Journal of Current Research and Academic Review 2 (1):164–70.
- Shim, T.-J., T. M. Kim, K. C. Jang, J.-Y. Ko, and D. J. Kim. 2013. Toxicological evaluation and anti-inflammatory activity of a golden gelatinous sorghum bran extract. Bioscience, Biotechnology, and Biochemistry 77 (4):697–705. doi: 10.1271/bbb.120731.
- Shin, L. 1986. Sorghum based breakfast cereal. Lincoln, NE: University of Nebraska-Lincoln.
- Shull, J. M., J. J. Watterson, and a W. Kirleis. 1992. Purification and immunocytochemical localization of kafirins in Sorghum bicolor (L. Moench) endosperm. Protoplasma 171 (1-2):64–74. doi: 10.1007/BF01379281.
- Shull, J., A. Chandrashekar, A. Kirleis, and G. Ejeta. 1990. Development of sorghum (Sorghum bicolor) endosperm in varieties of varying hardness. Food Structure 9 (3):253–67.
- Sikabbubba, R. 1989. The effect of alcohol soluble proteins on the digestibility of sorghum., Etats-Unis. Manhattan, KS: Kansas State University.
- Soto-Vaca, A., A. Gutierrez, J. N. Losso, Z. Xu, and J. W. Finley. 2012. Evolution of phenolic compounds from color and flavor problems to health benefits. Journal of Agricultural and Food Chemistry 60 (27):6658–77. doi: 10.1021/jf300861c.
- Spangler, R., B. Zaitchik, E. Russo, and E. Kellogg. 1999. Evolution and Generic Limits in Sorghum (Poaceae) Using ndhF Sequences. Systematic Botany 24 (2):267–81. doi: 10.2307/2419552.
- Starzyńska-Janiszewska, A., B. Stodolak, R. Socha, B. Mickowska, and A. Wywrocka-Gurgul. 2019. Spelt wheat tempe as a value-added whole-grain food product. LWT 113:108250.doi: 10.1016/j.lwt.2019.108250.
- Stefoska-Needham, A., and L. Tapsell. 2020. Considerations for progressing a mainstream position for sorghum, a potentially sustainable cereal crop, for food product innovation pipelines. Trends in Food Science & Technology 97:249–53. doi: 10.1016/j.tifs.2020.01.012.
- Svanberg, U., E. Sjögren, W. Lorri, A.-M. Svennerholm, and B. Kaijser. 1992. Inhibited growth of common enteropathogenic bacteria in lactic-fermented cereal gruels. World Journal of Microbiology & Biotechnology 8 (6):601–6. doi: 10.1007/BF01238797.
- Svensson, L., B. Sekwati-Monang, D. L. Lutz, R. Schieber, and M. G. Gänzle. 2010. Phenolic acids and flavonoids in nonfermented and fermented red sorghum (Sorghum bicolor (L.) Moench). Journal of Agricultural and Food Chemistry 58 (16):9214–20. doi: 10.1021/jf101504v.
- Taylor, J. R. N., and K. G. Duodu. 2019. Sorghum and millets: Chemistry, technology, and nutritional attributes. 2nd ed. Cambridge: Woodland Publishing.
- Taylor, J. R. N., and J. Dewar. 2001. Developments in sorghum food technologies. Advances in Food and Nutrition Research 43:217–64. doi: 10.1016/s1043-4526(01)43006-3.
- Taylor, J. R. N., and K. G. Duodu. 2015. Effects of processing sorghum and millets on their phenolic phytochemicals and the implications of this to the health-enhancing properties of sorghum and millet food and beverage products. Journal of the Science of Food and Agriculture 95 (2):225–37. doi: 10.1002/jsfa.6713.
- Taylor, J. R. N., and N. M. Emmambux. 2010. Understanding the physicochemical and functional properties of wheat starch in various foods. Cereal Chemistry 87 (4):305–14. doi: 10.1094/CCHEM-86-3-0290.
- Taylor, J. R. N., T. J. Schober, and S. R. Bean. 2006. Novel food and non-food uses for sorghum and millets. Journal of Cereal Science 44 (3):252–71. doi: 10.1016/j.jcs.2006.06.009.
- Taylor, J. R., and N. M. Emmambux. 2010. Developments in our understanding of sorghum polysaccharides and their health benefits. Cereal Chemistry 87 (4):263–71. doi: 10.1094/CCHEM-87-4-0263.
- Taylor, J., S. R. Bean, B. P. Ioerger, and J. R. N. Taylor. 2007. Preferential binding of sorghum tannins with gamma-kafirin and the influence of tannin binding on kafirin digestibility and biodegradation. Journal of Cereal Science 46 (1):22–31. doi: 10.1016/j.jcs.2006.11.001.
- Taylor, P. J. R. N., and N. M. Emmambux. 2008. Products containing other speciality grains: Sorghum, the millets and pseudocereals. In Technology of functional cereal products, 281–335. Cambridge: Woodhead Publishing.
- Thompson, L. U., J. H. Yoon, D. J. a Jenkins, T. M. S. Wolever, and a L. Jenkins. 1984. Relationship between polyphenol intake and blood-glucose response of normal and diabetic individuals. The American Journal of Clinical Nutrition 39 (5):745–51. doi: 10.1093/ajcn/39.5.745.
- Towo, E. E., U. Svanberg, and G. D. Ndossi. 2003. Effect of grain pre-treatment on different extractable phenolic groups in cereals and legumes commonly consumed in Tanzania. Journal of the Science of Food and Agriculture 83 (9):980–6. doi: 10.1002/jsfa.1435.
- Towo, E., E. Matuschek, and U. Svanberg. 2006. Fermentation and enzyme treatment of tannin sorghum gruels: Effects on phenolic compounds, phytate and in vitro accessible iron. Food Chemistry 94 (3):369–76. doi: 10.1016/j.foodchem.2004.11.027.
- Trappey, E. F., H. Khouryieh, F. Aramouni, and T. Herald. 2015. Effect of sorghum flour composition and particle size on quality properties of gluten-free bread. Food Science and Technology International = Ciencia y Tecnologia de Los Alimentos Internacional 21 (3):188–202. doi: 10.1177/1082013214523632.
- USDA-FAS. 2021. World agricultural production. doi: 10.32317/2221-1055.201907059.
- USDA-FGIS. 2009. Inspecting grain: Practical procedures for grain handlers. Grain inspection, packers and stockyards administration. USA.
- Vinje, M. A., S. H. Duke, and C. A. Henson. 2015. Comparison of factors involved in starch degradation in barley germination under laboratory and malting conditions. Journal of the American Society of Brewing Chemists 73 (2):195–205. doi: 10.1094/ASBCJ-2015-0318-01.
- Wagaw, K. 2019. Review on mechanisms of drought tolerance in Sorghum (Sorghum bicolor (L.) Moench) basis and breeding methods. Academic Research Journal of Agricultural Science and Research 7:87–99.
- Wahyuningsih, K., L. Rismayani, E. Y. Purwani, and I. Herawati. 2019. The effect of milling method on protein profile in sorghum (Sorghum bicolor L.) KD-4 variety. Agrointek 13 (2):109–20. doi: 10.21107/agrointek.v13i2.5034.
- Wang, Y.-Y D., and M. L. Fields. 1978. Germination of corn and sorghum in the home to improve nutritive value. Journal of Food Science 43 (4):1113–24. doi: 10.1111/j.1365-2621.1978.tb15247.x.
- Waniska, R., and L. Rooney. 2000. Structure and chemistry of the sorghum caryopsis. New York: John Wiley and Sons, Inc.
- Welch, R. W. 2011. Nutrient composition and nutritional quality of oats and comparisons with other cereals. In Oats: Chemistry and technology, 2nd ed., 95–108. St Paul, MN: AACC International.
- Wijngaard, H. H., C. Rößle, and N. Brunton. 2009. A survey of Irish fruit and vegetable waste and byproducts as a source of polyphenolic antioxidants. Food Chemistry 116 (1):202–7. − doi: 10.1016/j.foodchem.2009.02.033.
- Wong, J. H., T. Lau, N. Cai, J. Singh, J. F. Pedersen, W. H. Vensel, W. J. Hurkman, J. D. Wilson, P. G. Lemaux, and B. B. Buchanan. 2009. Digestibility of protein and starch from sorghum (Sorghum bicolor) is linked to biochemical and structural features of grain endosperm. Journal of Cereal Science 49 (1):73–82. doi: 10.1016/j.jcs.2008.07.013.
- Worku, B. B., Woldegiorgis, A. Z. Gemeda H., and F. 2015. Indigenous processing methods of cheka: A traditional fermented beverage in southwestern Ethiopia. Journal of Food Processing & Technology 7:1–7.
- Wu, L., Z. Huang, P. Qin, and G. Ren. 2013. Effects of processing on phytochemical profiles and biological activities for production of sorghum tea. Food Research International 53 (2):678–85. doi: 10.1016/j.foodres.2012.07.062.
- Wu, L., Z. Huang, P. Qin, Y. Yao, X. Meng, J. Zou, K. Zhu, and G. Ren. 2011. Chemical characterization of a procyanidinrich extract from sorghum bran and its effect on oxidative stress and tumor inhibition in vivo. Journal of Agricultural and Food Chemistry 59 (16):8609–15. doi: 10.1021/jf2015528.
- Wu, Y., X. Li, W. Xiang, C. Zhu, Z. Lin, Y. Wu, J. Li, S. Pandravada, D. Ridder, G. Bai, et al. 2012. Presence of tannins in sorghum grains is conditioned by different natural alleles of Tannin. Proceedings of the National Academy of Sciences of the United States of America 109 (26):10281–6. doi: 10.1073/pnas.1201700109.
- Xie, M., J. Liu, R. Tsao, Z. Wang, B. Sun, and J. Wang. 2019. Whole grain consumption for the prevention and treatment of breast cancer. Nutrients 11 (8):1769–21. doi: 10.3390/nu11081769.
- Xiong, Y., P. Zhang, R. D. Warner, and Z. Fang. 2019. Sorghum Grain: From genotype, nutrition, and phenolic profile to its health benefits and food applications. Comprehensive Reviews in Food Science and Food Safety 18 (6):2025–46. doi: 10.1111/1541-4337.12506.
- Yanagida, A., A. Shoji, Y. Shibusawa, H. Shindo, M. Tagashira, M. Ikeda, and Y. Ito. 2006. Analytical separation of tea catechins and food-related polyphenols by high-speed counter-current chromatography. Journal of Chromatography. A 1112 (1-2):195–201. doi: 10.1016/j.chroma.2005.09.086.
- Yang, L., K. F. Allred, L. Dykes, C. D. Allred, and J. M. Awika. 2015. Enhanced action of apigenin and naringenin combination on estrogen receptor activation in non-malignant colonocytes: Implications on sorghum-derived phytoestrogens. Food & Function 6 (3):749–55. doi: 10.1039/c4fo00300d.
- Young, R., M. H. Gomez, C. M. McDonough, R. D. Waniska, and L. W. Rooney. 1993. Changes in sorghum starch during parboiling. Cereal Chemistry 70:179–83.
- Yousif, A., D. Nhepera, and S. Johnson. 2012. Influence of sorghum flour addition on flat bread in vitro starch digestibility, antioxidant capacity and consumer acceptability. Food Chemistry 134 (2):880–7. doi: 10.1016/j.foodchem.2012.02.199.
- Zhang, G., and B. R. Hamaker. 1998. Low α-amylase starch digestibility of cooked sorghum flours and the effect of protein. Cereal Chemistry Journal 75 (5):710–3. doi: 10.1094/CCHEM.1998.75.5.710.
- Zhang, G., and B. R. Hamaker. 2009. Slowly digestible starch: Concept, mechanism, and proposed extended glycemic index. Critical Reviews in Food Science and Nutrition 49 (10):852–67. doi: 10.1080/10408390903372466.
- Zhang, L., A. S. Ravipati, S. R. Koyyalamudi, S. C. Jeong, N. Reddy, P. T. Smith, J. Bartlett, K. Shanmugam, G. Münch, and M. J. Wu. 2011. Antioxidant and anti-inflammatory activities of selected medicinal plants containing phenolic and flavonoid compounds. Journal of Agricultural and Food Chemistry 59 (23):12361–7. doi: 10.1021/jf203146e.
- Zhang, Y.-J., R.-Y. Gan, S. Li, Y. Zhou, A.-N. Li, D.-P. Xu, and H.-B. Li. 2015. Antioxidant phytochemicals for the prevention and treatment of chronic diseases. Molecules (Basel, Switzerland) 20 (12):21138–56. doi: 10.3390/molecules201219753.
- Zhao, Y. 2016. Effect of different tempering methods on sorghum milling. Retrieved from https://docs.lib.purdue.edu/cgi/viewcontent.cgi?article=2206&context=open_access_theses
- Zhao, Y., and R. P. K. Ambrose. 2018. A laboratory-scale tempering and milling method for grain sorghum. Transactions of the ASABE 61 (2):713–21. doi: 10.13031/trans.12343.
- Zulak, K., D. Liscombe, H. Ashihara, and P. Facchini. 2006. Alkaloids: Plant secondary metabolism in diet and human health. Oxford, UK: Blackwell Publishing.