Abstract
Food consumption of healthier products has become an essential trend in the food sector. This is also the case in beer, a biochemical process of transformation performed by yeast cells. More and more studies proclaim the need to reduce ethanol content in alcoholic drinks, certainly the most important health issue of beer consumption. In this review we gather key health issues related to beer consumption and the last advances regarding the use of yeast to attenuate those health problems. Furthermore, we have included the latest findings about the general positive impact of yeast in health as a consequence of its ability to biotransform polyphenolic compounds present in the wort, producing healthy compounds as hydroxytyrosol or melatonin, and its ability to perform as a probiotic driver. Besides, a group of population with chronic diseases as diabetes or celiac disease could take advantage of low carbohydrate or gluten-free beers, respectively. The role of yeast in beer production has been traditionally associated to its fermentative power. But here we have found a change in this dogma in the last years toward yeasts being a main driver to enhance healthy aspects of beer. The key findings are discussed and possible future directions are proposed.
Introduction
Beer is associated to human culture since ancient times. Archaeologist researchers have found evidence of the production of a cereal grain-based drink in remains dated 13000 years ago (Liu et al. Citation2018). For ancient societies and even indigenous actual cultures, beer has been considered more as a food than a beverage for the daily caloric intake. Beer has also been important for human social development. It helps to reinforce social communality and hospitality during ceremonial and everyday activities. Beer is everyone’s drink, showing no differences in terms of sex or socioeconomic level.
In the last years, consumers have focused in improve their health from a more holistic and personized point of view, considering food as a key element. Nowadays, consumers select beverages and food based on their definition of health, specially focusing on healthy adjectives as diet, organic, natural, functional of high in proteins. Regarding alcoholic drinks, half of the consumers worry about health impact and search for innovative products that does not produce negative effects of alcohol consumption. This trend is also present, and the consumers show a reasonable interest in nutrition fact labels, shared by the population in general (Achón et al. Citation2017).
Yeasts are the main driver in the transformation of hundreds of compounds during the alcoholic fermentation, the main step in the preparation of beer products. Since ancient times, we have taken advantage of some yeast metabolic abilities that they exhibit during this fermentative transformation. Now is the time to exploit other hidden abilities that yeasts can show in order to change beer toward a healthier product. That also includes the attenuation of the production of non-desirable compounds as off-flavors, biogenic amines, toxic compounds as ethyl carbamate and fusel oil compounds. In this work we will revise the recent research approach regarding this idea. Starting with the ability of yeast to ferment beer without producing high levels of ethanol, continuing with studies regarding the role of yeasts in beers indicated to patients of chronic illnesses as diabetes or celiac disease and ending with the revision of works centered in positive and healthy yeasts attributes as the synthesis of bioactive compounds derived from the aromatic amino acids or the yeast cells probiotic effects.
The role of yeast in the production of low alcoholic content beers
Ethanol is the most important compound produced by yeast though the alcoholic fermentation during beer production. Indeed, it could be the production of this primary metabolite what presumably woke up the interest of humans for the fermented beverages in the first time. It was proposed that Saccharomyces yeasts tends to generate elevated amounts of ethanol, a compound to which they are very resistant, to outcompete other microorganisms naturally present in their ecological niche (Piškur et al. Citation2006; Thomson et al. Citation2005). On top of that, industrial brewing yeasts have been domesticated by humans by selecting the most powered fermentative strains (Gallone et al. Citation2018; Saada et al. Citation2022). The production of beer involves the alcoholic fermentation performed by different yeasts which, in the end, determines the style of this drink. There are mainly two beer related species: Saccharomyces cerevisiae which is tasked to ferment Ale beers and Saccharomyces pastorianus (an interspecific hybrid between S. cerevisiae and S. eubayanus), implicated in the production of Lager beers (Baker et al. Citation2015). Although most beers are produced by inoculation of a selected starter, there are some beer styles as sour beers (e.g. Belgium lambic beer and American Coolship Ales) performed by spontaneous fermentation of the microbiota present in the fermenters, including species as Meyerozyma guilliermondii, Debaryomyces spp., Pichia spp., Wickerhamomyces anomalus, Brettanomyces anomalus, Brettanomyces custersii, Brettanomyces bruxellensis, Candida krusei, Cryptococcus keutzingii, Naumovia castellii, Kazachstania servazzii and Rhodotorula mucilaginosa (Spitaels et al. Citation2014). In the last years, there is an interest to study other non-conventional yeasts for the development of starter cultures that increase variety and organoleptic diversity in the beer market. These species are also being studied for their potential to reduce the ethanol concentration (Krogerus et al. Citation2022).
Ethanol is produced by the conversion of pyruvate in a two-step alcoholic fermentation pathway: first, pyruvate is converted to acetaldehyde by a pyruvate decarboxylase (PDC), releasing carbon dioxide. Then, acetaldehyde is converted into ethanol by an alcohol dehydrogenase (ADH) (Dzialo et al. Citation2017). There are three genes encoding the PDC enzymes encoded in the Saccharomyces cerevisiae genome, which carries out a key point in the flux divergence between respiration and fermentation because it is in direct competition with the pyruvate dehydrogenase (Cherry et al. Citation2012). The ADH is a type of oxidoreductase that can catalyze the reversible interconversion between alcohols and their corresponding aldehydes o ketones. Eukaryotes have numerous ADH enzymes, in the case of Saccharomyces cerevisiae species, it has seven different types (Bakker et al. Citation2001; de Smidt, Du Preez, and Albertyn Citation2012; Leskovac, Trivić, and Peričin Citation2002). Some of these enzymes are heavily regulated with an overarching goal: to channel the carbon flux that comes from the sugar consumption to produce high amounts of ethanol.
Negative effects of ethanol consumption in beer
Although the early civilizations developed spontaneous fermentation methods to exploit the benefits of ethanol, nowadays we have noticed about the negative effects that it can produce (). As with other substances, the positive or negative effects will depend on the dose in which it is consumed. A sector of the scientific community argues that a moderate consumption (< 30 gr/day) can have beneficial effects (de Gaetano et al. Citation2016). Although there are experiments that clearly supports the cardiovascular benefit of low-level alcohol intake, these cardioprotective effects are likely to be outweighed by other risks as addiction, liver disease, cancer, cardiovascular diseases (CVDs) and by the cumulative toxic effects of alcohol (Shield, Parry, and Rehm Citation2013). In fact, it has been shown that even low levels of alcohol intake are related with more than 10% of the cancer cases attributable to it (Rumgay et al. Citation2021). Excessive alcohol consumption has negative health consequences, in the short term after a large one-time intake or in the long term because of continued drinking causing chronic diseases.
Table 1. Summary of negative effects produced by ethanol consumption.
A huge intake of alcohol can produce indirect injuries due to collisions with vehicles, falls, drowning or violence, such as murder or sexual assaults. For example, in the United States, around six people per day die from alcohol poisoning caused by high levels of alcohol in blood (Kanny et al. Citation2015). Another important risk derived from alcohol intake is the occurrence of spontaneous abortions, showing higher odds in the first trimester of the pregnancy (Kesmodel et al. Citation2002).
A continued consumption of alcohol may end up in different chronic diseases. The presence and easy accessibility to alcohol results in an extensive personal and social use, with consequent widespread organ damage (Shield, Parry, and Rehm Citation2013). The liver and digestive tract can be highly affected by ethanol toxicity, but the neurological and cardiovascular systems are also important targets (Fernández-Solà Citation2015; Orman, Odena, and Bataller Citation2013).
The vascular system and the heart are susceptible to be harmed by the effects of alcohol. Alcohol intake induces a spectrum of cardiovascular damage: including cardiovascular-related mortality, coronary heart disease (CHD), subclinical ventricular dysfunction, alcoholic cardiomyopathy with congestive heart failure, peripheral artery disease (PAD), and ischemic and hemorrhagic cerebrovascular events (Fernández-Solà Citation2015).
Cardiac myocytes are excitable cells that have a complex signaling and contractile structures. They are very sensitive to the toxic effects of ethanol since it can affect at different levels. The alcohol consumption can modify different aspects of their plasma membrane: composition, permeability, signaling and activation of apoptosis (Oliveira et al. Citation2010). There is a strong association between alcohol and arrhythmias, especially the atrial extrasystoles and atrial fibrillations. Alcohol is also positively correlated with ventricular extrasystoles and tachycardia in heavier drinker, and it can result in sudden death (CitationGeorge and Figueredo 2010). Sustained alcohol consumption can also have chronic cardiac effects. Cardiac dysfunction associated with excessive alcohol intake is a specific cardiac disease known as alcoholic cardiomyopathy (ACM). ACM is associated with several adverse histological, cellular, and structural changes within the myocardium. (Guzzo-Merello et al. Citation2014).
Alcohol consumption is also associated with atherosclerosis. There are studies that show that increased alcohol consumption is associated with increased arterial stiffness and with decreased intima-media wall thickness (Kim et al. Citation2014). Heavy ethanol intake (>60 g/day) induces widespread arterial damage in brain, coronary and peripheral limbs arteries. On the other hand, moderate alcohol intake is associated with decreased risk of cardiovascular disease (CVD) events like myocardial infarction and stroke since it decreases the levels of thrombogenic and coagulation factors (including fibrinogen) (Rimm et al. Citation1999). The cardiovascular benefits of moderate alcohol intake (<30 g/day) are also based on changes in the levels of plasma lipoproteins. Moderate alcohol consumption is related with high concentrations of HDL cholesterol and apolipoprotein AI, and low concentration of LDL cholesterol (Brinton Citation2010).
Hypertension is associated with long-term ethanol consumption. Preclinical studies have demonstrated a positive correlation between the duration of ethanol consumption and the development of hypertension, mainly at high doses (>40 g/day). Mechanisms of alcohol-induced arterial hypertension are based on an increase in central adrenergic activity and vascular smooth-muscle tone, an activation of the renin angiotensin aldosterone system, and a decreased release of nitric oxide (Crestani et al. Citation2014).
Alcohol consumption also affects glucose metabolism and can imping on the appearance of certain types of diabetes. Light-to-moderate intake of alcohol (10-30 g/day) inhibits gluconeogenesis and glycogenolysis, and enhances the insulin sensitivity. These effects seem to decrease the incidence of type 2 diabetes mellitus by 30%. But, with excessive alcohol intake these favorable effects are not observed, and heavy drinkers have the same or higher risk than total abstainers (Pietraszek, Gregersen, and Hermansen Citation2010).
Ethanol content reduction in beers
Low-alcohol beer (LAB) and non-alcohol beer (NAB) are beers with an increasing interest due to the valuable contribution to enhance healthy diets. The ethanol content by volume limits depends on laws in different countries. In most of the EU countries it is considered LAB when the alcohol content is < 1.2% v/v and NAB when the alcohol content is < 0.5% v/v (Blanco, Andrés-Iglesias, and Montero Citation2016). The interest and the trend to consume these healthy beers is increasing and a growth in the market of low-alcohol beer is expected at 2.8% and non-alcohol at 8.8% in next five years (IWSR 2019).
The reduction of the alcoholic content in beer can be carried out by two types of methods: One relies on the removal ethanol from the final product (dealcoholisation) from a completely fermented alcoholic beer using several separation processes, the other strategy relies on restriction of alcohol formation during fermentation process.
Dealcoholisation can be achieved by different techniques such as reverse osmosis, dialysis, or osmotic distillation (Blanco, Andrés-Iglesias, and Montero Citation2016). These methods have in common that they produce a significant loss of organoleptic characteristics of the final product due to the removal of volatile compounds such as higher alcohols and esters. Thus, it drastically alters the final product, and it is expensive from the economic and energetic point of view.
Restriction of alcohol formation can be achieved by either shortening the fermentation process – interrupted fermentation, or by control of alcohol production – cold contact process. In recent years, brewers focus on experimenting with non-conventional yeasts in brewing, which produce less ethanol during the fermentation process (Blanco, Andrés-Iglesias, and Montero Citation2016). This strategy does not require adding stages to the industrial process and is based in the use of new yeasts that show lower alcohol production to use them in combination with commercial yeasts or to substitute the yeasts that are normally used in the industry (). Thus, important research and development effort is focused on searching and optimizing yeast starters by different strategies (Varela and Varela Citation2019) ().
Table 2. Summary of significant studies evaluating yeast strains with reduced ethanol production.
The use of genetic modification techniques
The initial idea to produce “low-ethanol” yeasts was focused primarily on generating new S. cerevisiae strains by genetic modification technologies (GM). These techniques have been used to divert carbon metabolism away from ethanol formation, by redirecting carbon to others final points, trying not to vary the fermentative capacity of the yeasts and to achieve a final product that maintains its organoleptic characteristics (Kutyna et al. Citation2010; Varela et al. Citation2015).
Different GM strategies to decrease the ethanol production in S. cerevisiae have been described although the introduction of gene modifications can affect the formation of other important compounds that alter the beer quality (Varela et al. Citation2015). Some studies have shown successful strategies that produce the decrease of ethanol production as, for example, diverting carbon to the tricarboxylic acids (TCA) cycle, increasing glycerol production or increasing lactic acid production.
The most straightforward GM approach is the deletion of genes encoding the ADH enzymes to eliminate the production of ethanol. This was assayed decades ago (US Patent No. 4814188) and the results were a higher glycerol production, but also a production of acetaldehyde, an off-flavor that invalidates this method.
An interesting strategy was based in the generation of strains deficient in the tricarboxylic acid cycle, lacking the genes FUM1 (fumarase) and KGD1/KGD2 (alpha-ketoglutarate dehydrogenase) which produced nonalcoholic beers with less than 0.5% v/v of alcohol (Selecký, Šmogrovičová, and Sulo Citation2008). These genetic changes produce a considerably higher concentrations of residual saccharides in the beer that could be a problem. An increase in lactic acid observed in this strain helps to mask the unacceptable worthy flavor. However, a considerable amount of diacetyl and absence of many essential esters, such as ethyl acetate and amyl acetate, can negatively affect the taste of the product.
Another possibility is to manipulate the glycerol/ethanol ratio by enhancing the glycerol production, generating a reduction in the ethanol yield. One strategy is the over-expression of GPD1 or GPD2 genes, that encode the Gpd1p and Gpd2p, isozymes that convert dihydroxyacetone phosphate (DHAP) to glycerol 3-phosphate (G3P), which is dephosphorylated to glycerol by glycerol 3-phosphatase. Although the increasing of glycerol production can lead to reduced ethanol yield, it is not sufficient to restore the steady state of NAD+/NADH ratio. Another consequence is the elevated concentration of succinate, acetaldehyde, acetoin and 2,3-butanediol (Kutyna et al. Citation2010).
In summary, the GM approaches provide the opportunity to decrease alcohol production with respect to their parent strains and the use of new technologies as the CRISPR/Cas9 system opens the potential to generate new yeast strains. Although further GM work in this area is likely to overcome some side effects, the underlying problem of negative public perception toward GMOs in food and beverage restrict their application. As long as it is not viable to generalize commercialization of GMO industrial yeast strains, we can take advantage of the knowledge gained from GMO-based studies to generate beer yeasts with reduced ethanol yields using non-GMO approaches to manipulate yeast metabolism, such as hybridization or evolutionary engineering.
The use of adaptive evolution
Another methodology to optimize yeast to reduce ethanol production in the beer fermentations is a technique called adaptive evolution or evolutionary engineering. The adaptative evolution at the laboratory consists of generating an artificial selection process, in an accelerated way, promoting the selection of the mutations that are most useful to our purpose (Gonzalez et al. Citation2021). The method is based on applying a selection pressure that favors sufficient genetic variation for this to generate a diversion away from ethanol production. The spontaneous emerging of this genetic variation is improbable, thus, to enhance the possibility of generating new yeasts, mutagens such as ethyl methane sulfonate (EMS), nitroso guanidine (NTG), diethyl sulfonate (DES), or UV and X-ray irradiation are sometimes used (Chambers et al. Citation2009). Yeasts are grown in a selective environment in which the desired metabolic changes would favor their growth. These cultures in specific selective medium can direct adaptations toward the desired phenotypes. This approach has been used to produce yeasts that can use xylose, have enhanced maltose utilization, and improved their alcohol tolerance (Chambers et al. Citation2009).
An interesting example of adaptively evolved strain has been shown to decrease the ethanol production (Tilloy, Ortiz-Julien, and Dequin Citation2014). The yeast culture was serially transferred under hyperosmotic conditions during 450 generations using KCl as osmotic and salt stress agent. The resulting strains, that became a commercial product, showed reduced ethanol yield, increased glycerol yield, and did not produce acetic acid. Another example has been described with the aim to channel carbon flux toward the pentose phosphate pathway (PPP). The selective pressure was established using gluconate as the only carbon source. Gluconate is a substrate poorly assimilated by S. cerevisiae and metabolized by the PPP which doesn’t contribute to ethanol production. The selected strain showed an increase in flux through the PPP compared to the ancestral strains, corresponding to a reduction in ethanol (Cadière et al. Citation2011).
In essence, this methodology is perfectly viable to optimize yeast that produce less ethanol, thus it is really plausible that new beer strains obtained with this method will appear in the market of industrial beer starters in the next years. The mayor limitation at the moment is that the amount of ethanol yield reduction of the strains obtained with this method is still considerable so they could be used to produce LAB but not NAB.
The use of non-conventional yeasts
S. cerevisiae and S. pastorianus species are well adapted to the beer fermentation process and some strains of these species had been selected for its use in inoculated fermentations. Classically, other yeast species present during the initial stages of spontaneous fermentations were traditionally considered to be undesirable microorganisms. Nowadays, the called ‘non-conventional’ yeasts species have been reevaluated and they have shown interesting characteristics for specific uses.
Screening yeasts for natural variants that produce less alcohol is an interesting way to obtain “low-alcohol” yeast strains. The use of this approach has been recently growing as a result of an increased interest in innovating and generate new beers. Furthermore, these yeasts can positively contribute during the production of alcoholic beverages with different aroma and flavors. Several studies show that non-Saccharomyces yeasts used in sequential or co-inoculation with S. cerevisiae can develop a lower ethanol yield during wines or beer fermentations (Contreras et al. Citation2014).
One of the most important points for ethanol production by brewing yeasts is the consumption of the main sugars present at the wort. These sugars present in brewer’s wort are mainly maltose (50-60%), maltotriose (15-20%) and glucose (10-15%). The difference in ethanol production between strains mostly depends on the ability to efficiently ferment these sugars. Strains producing less than 5% alcohol by volume only fermented 50–60% of the available maltotriose, whereas strains producing more than 5% ethanol fermented up to 70% of the maltotriose (Krogerus et al. Citation2017). The use of maltose negative is therefore a useful way to produce low-alcohol beers that still retain some of the aromatic complexity of the normal beers. Theses yeasts also reduce wort aldehydes, removing the “worty” taste that is often found in low-alcohol beers (Gibson et al. Citation2017).
The search of new ways to produce low-alcohol beer has inspired brewers to experiment with these non-conventional yeast species and strains that are unable to ferment the most abundant sugars present in the wort, but that maintain enough metabolic activity from the utilization of the simple sugars to generate the volatile components of the beer (Bellut et al. Citation2018; Johansson et al. Citation2021). Maltose-negative yeasts have been acquired from different environmental niches, but fermented food systems are of special interest due to the presence of nonpathogenic yeast strains that are considered acceptable for food applications. For example, the potential of sourdough cultures as sources of new yeast strains for low-alcohol brewing has been studied. These studies have shown the isolation of maltose-positive S. cerevisiae strains with good beer fermentation properties (Catallo et al. Citation2020), but also the isolation of non-Saccharomyces yeast species (de Vuyst et al. Citation2016). Ten different ascomycetes yeast species were isolated from different sourdough types, seven of them were maltose-negative and produce less than 1% (v/v) alcohol from a 12° Plato wort (Johansson et al. Citation2021)
Brewing strains of S. cerevisiae are usually able to use both maltose and maltotriose efficiently, for this reason the production of ethanol is higher. But S. eubayanus can only use maltose and that can carry a lower ethanol yield (Krogerus et al. Citation2017). Besides, the cryophilic species S. uvarum has been described as low ethanol, low acetate, and high glycerol producer yeast (Coloretti, Zambonelli, and Tini Citation2006). There are studies that compared the ethanol yield of several strains of S. uvarum, Candida zemplinina and Candida stellata. All these species showed a lower ethanol yield than S. cerevisiae (Magyar and Tóth Citation2011). Moreover, strains of the species Hanseniaspora uvarum, Zygosaccharomyces sapae and Zygosaccharomyces bisporus showed a lower ethanol yield compared to a control strain of S. cerevisiae (Gobbi et al. Citation2014). It is also highlighted the potential of strains of the species Schizosaccharomyces malidevorans and M. pulcherrima for sequential fermentations with S. cerevisiae, achieving an ethanol reduction (Contreras et al. Citation2014).
As we have shown, the use of non-conventional yeasts in the beer fermentation is being explored to obtain beers with reduced ethanol content but also for other applications related to beer quality. In this case, the reduction of ethanol content in beer can be higher, selecting strains that cannot assimilate most of the wort sugars. This generate other organoleptic problems as too sweet taste or worty like flavor that must be solved in the next years but the potential of this approach is among the highest to generate NAB/LAB products.
The generation of interspecific hybrid yeasts
There is an increasing interest in new brewing yeast hybrids generated by de novo hybridization. The breeding of brewing yeasts has been carried out for years in attempts to generate new strains and improve different fermentation aspects. It must be considered that the most commonly used yeast in brewing worldwide S. pastorianus is an interspecific hybrid (Krogerus et al. 2015).
Due to the domestication of most industrial brewing yeasts, a lot of them have lost the ability to sporulate and sexually reproduce. Different methods have been developed to overcome the limitation of low fertility in traditional brewing yeasts, the most commons are rare mating and protoplast fusion, although the later generates GMO’s as it has fell into disuse. Rare-mating is based on the spontaneous loss of heterozygosity at the mating type locus that occurs at low frequencies, resulting in the formation of diploid (or potentially higher ploidy) cells with a single mating type (Peris et al. Citation2020). The hybridization frequencies are typically low, and the parent strains require selection markers, the most used are auxotrophies, that allow to isolate the hybrids from the population of parent cells (Krogerus et al. Citation2017). The construction of artificial hybrids has opened up a new era to generate new yeast starters with innovative characteristics provided by the parental (Lairón-Peris et al. Citation2020)
De novo yeast hybrids have been used to successfully improve beer fermentation, including faster fermentations rates, increased aroma formation and higher stress tolerance (Giannakou et al. Citation2021). For example, new de novo lager yeast hybrids by mating Saccharomyces cerevisiae ale strain and Saccharomyces eubayanus have been developed (Krogerus et al. 2015). These hybrids inherit beneficial properties from both parental strains (cryotolerance, maltotriose utilization and strong flocculation) and ferment faster than the parents, producing beer with higher alcohol content. Nonetheless, this technique can be used to generate new strains using parents with a lower ethanol yield.
Overall, observing the increase in the last years in the use of yeast hybrids in the beer but also in the wine industry we can conclude that this is probably the most promising method to optimize commercial yeast strains and the generation of strains with low ethanol production is a perfect example. Beer hybrid strains with reduced ethanol yield will be commercialized in the next years although, similarly as the in the use of adaptive evolution, is unlikely to generate yeast hybrids that are able to produce beers with very low or no alcohol content.
Potential role of yeasts to reduce negative effects of beer in diabetic and celiac diseases
In response to the increased consumer demands and from the perspective of health, the brewing industry has devoted intensive effort into the development of new low carbohydrate special beers, introducing into the market the so-called low calorie, low carbohydrates, light or diabetic beers. Beer can be considered “low carb” when it shows less than 20 g/L of carbohydrates.
Type 2 diabetes mellitus (T2DM) is an epidemic disease that could be developed even at younger ages. It is becoming a cause of concern due to its global prevalence and mortality rate. In addition to genetic susceptibility, several factors exist that can accelerate T2DM: overweight and obesity, sedentary lifestyles, smoking, alcohol consumption and the diet. Particularly, the quality of carbohydrates and fats has an essential role in its development (Sievenpiper Citation2020) because of its impact on glucose homeostasis, being the high dietary glycemic load (GL) and the trans fats the factors that increase the risk the most. The first one increases the insulin demand and may lead to pancreatic B-cell exhaustion and the second may play a role in the development of insulin resistance (Petersen and Shulman Citation2018).
There is a synergic beneficial effect between two compounds that can be added in alcohol-free beers to modulate postprandial glycemia and improve glucose metabolism in subjects with T2DM and overweight or obesity (Mateo-Gallego et al. Citation2020). One is the sugar isomaltulose, a disaccharide composed of α-1,6 bonds of glucose and fructose. This sugar decreases glucose and insulin concentration and increases fat oxidation (Koshinaka, Ando, and Sato Citation2018). The other compound is resistant dextrin, which is not completely hydrolyzed and absorbed in the small intestine while almost all is fermented in the colon. Used as a prebiotic, improves insulin resistance and inflammation in patients with T2DM (Hu et al. Citation2020).
The uptake of the fermentable sugars by yeasts in a normal fermentation is well established. During the first days, sucrose is quickly hydrolyzed to glucose and fructose to be taken up by the yeast cells. Then, yeast adapt to and starts maltose and maltotriose fermentation. These polysaccharides are further hydrolyzed by industrial beer strains of S. cerevisiae. However, higher oligomers are not fermented because of the absence of amylolytic activity of the brewing yeast (Bamforth Citation2002). One of them is the dextrin, the main non-fermentable sugar (20-25% of total wort carbohydrates) based in α-1,4 and α-1,6 bonds. In order to produce low carb beers, the dextrin content should be reduced or completely removed. This reduction can be done adding exogenous enzymes that hydrolyze dextrin to glucose, such as the amyloglucosidase (AMG) of Aspergillus niger. This enzyme is also able to degrade maltose and maltotriose to glucose. However, the complete elimination of dextrin remains difficult. When dextrins are degraded to the point where α-1,6 linked glucose unit constitute the non-reducing end of a dextrin, it becomes almost resistant to further amyloglucosidase attacks. Thus, the net result is a reduction of dextrins and a concomitant formation of glucose. On top of that, some added enzymes have shown that they could generate allergenic symptoms such as rhinitis and/or bronchial asthma produced by the α-amylase of A. oryzae (Baur, Chen, and Sander Citation1994).
Some yeast strains have the potential to be used to produce low carb beers. Diastatic strains of S. cerevisiae produce an extracellular glucoamylase that hydrolyzes dextrins, maltotriose and starch (Krogerus et al. Citation2019). These strains have traditionally been associated with beer contamination, because that capability causes super-attenuation: increased carbon dioxide and ethanol levels, production of off-flavors (particularly the clove-like 4-vinyl guaiacol) and a drier mouthfeel (Meier-Dörnberg et al. Citation2018). In extreme cases, this can lead to exploding packages, endangering the consumer. However, under controlled conditions, they may have many positive traits to be exploited profitably in developing new beers, such as diabetic beers (Krogerus and Gibson Citation2020). Specifically, three STA genes (STA1, STA2 and STA3) encode the I, II and III glucoamylase isozymes. This property has been transferred into brewing yeasts by somatic hybridization via protoplast fusion but trying to keep the same properties of the industrial yeast strains (Hansen, Röcken, and Emeis Citation1990). In this case, the glucoamylase lacks the ability to hydrolyze α-1,6 bonds, but adding the pullulanase enzyme the fermentation could be completed (Xu et al. Citation2021). Furthermore, the S. diastaticus DBVPG 6580 strain, isolated from a bioethanol plant, was successfully used to produce beer under different conditions (wort densities and fermentation temperatures) in a conventional manner (Troilo et al. Citation2020).
Other strategy involves the use of genetically modified yeast strains that express amylolytic enzymes. Despite recombinant yeast strains have limited perception by some costumers, safer industrial recombinant strains have been recently constructed. For example, the Debaryomyces occidentalis glucoamylase gene (GAM1) or Aspergillus awamori glucoamylase gene (GA1) have been used to develop brewer’s yeasts to reduce the caloric content of beer (Park et al. Citation2014). However, there is a drawback since phenotypes produced by plasmid containing strains are not stable because of the probability of the plasmid to be lost over generations without selection pressure. A more promising and an acceptable method was used in the work of Ogata et al. where they generated a brewing strain that secretes glucoamylase by mating an industrial beer strain with a S. diastaticus strain (Ogata, Iwashita, and Kawada Citation2017) ().
Celiac disease is directly related to the food content of gluten, a complex mixture of hundreds of related but distinct proteins, mainly gliadin and glutenin. Collectively, the gliadin and glutenin proteins are referred to as prolamins, which represent seed proteins insoluble in water, but extractable in aqueous ethanol and are characterized by high levels of glutamine (38%) and proline residues (20%). Similar proteins as gliadin in wheat, can be found in other cereal such as hordein in barley, secalin in rye and avenin in oats and are all referred to as gluten (Gumienna and Górna Citation2020). Triticale, malt and other ancient wheat varieties (kamut and spelt) are derivatives of these grains and can also contain gluten. Celiac disease can be triggered by gluten found in all these grains. Gluten is heat stable and it is known for its use as the binding and extending agent that also improves moisture retention, texture and flavor as an additive in processed foods.
This global disease is affecting about 0.7% of the population worldwide (Glissen Brown and Singh Citation2019). As of now, the only known treatment is a strict gluten free diet, that must be practiced until the end of the patient’s life. Individuals who carry the human leukocyte antigen (HLA)-DQ2 or DQ8 have genetic disposition that can trigger the disease. With consumption of gluten by celiac disease patients, the damage in the small intestine produces distension, altered bowel habits and other common gastrointestinal symptoms. Most of the diet without gluten is based on the food that lacks gluten naturally and is unlikely to be contaminated. Market also offers specially formulated products, consisting of gluten-free grains and labeled as »gluten free« which are normally highly processed and provide poor nutritional value. Strict gluten free diet improves most of the patients’ histological lesions, biochemistry of blood, clinical manifestations and the risk of complications related to celiac disease (Bascuñán, Vespa, and Araya Citation2017) ().
Figure 3. Gluten assimilation. Healthy people with undamaged villi have a good absorption of nutrients while celiac patient with damaged villi have a bad absorption of nutrients.
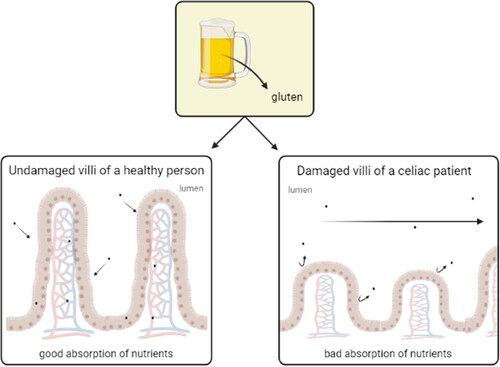
Some studies have proposed the use of brewing strains that could help to degrade gluten to generate gluten-free beers. In the study of Sakandar et al., some bacterial and three yeast isolates from sourdough samples were screened for the presence of gluten-degrading ability (Sakandar, Usman, and Imran Citation2018). They isolated gluten degrading microbiota from sourdough samples using specific gluten limited agar (GA) that was described by Zamakhchari et al. (Zamakhchari et al. Citation2011). They also showed that amongst yeast strains the maximum degradation was done by three strains of Wickerhamomyces anomalus. Another proposed method can be the co-culture fermentations of Pediococcus acidilactici and two yeasts (S. cerevisiae and Torulaspora delbrueckii) in regards of degradation of wheat allergens (Fu et al. Citation2021). The co-culture fermentation quickened the degradation of protein and clearly lowered the allergen content. Authors confirmed the synergistic effect between used microorganisms on degrading wheat allergens and see the potential use of multi-strain leavening agent for production of hypoallergenic wheat products.
Potential role of brewing yeast in the production of healthy aromatic amino acid-derived compounds
Beer is made up of a large variety of healthy molecules, among which the phenolic compounds (PC) stand out derived mainly from plant origin (hops and barley malt) but also produced from the alcoholic fermentation during the brewing process by yeasts (Ruiz-Ruiz et al. Citation2019). PC are hydroxylated aromatic ring-containing compounds in which the hydroxyl group is directly attached to a phenyl, substituted phenyl, or another aryl group and they are the main antioxidant compounds in beer (Martinez-Gomez, Caballero, and Blanco Citation2020) ().
Figure 4. Schematic representation of the yeast synthesis of bioactive compounds present in beer derived from L-tryptophan. The aromatic amino acids tyrosine (Tyr), 2-phenylalanine (Phe), and tryptophan (Trp) are produced through the shikimate (SKP) pathway that begins with the condensation of erythrose-4-phosphate (E4P) and phosphoenolpyruvate (PEP) from the pentose phosphate pathway (PPP) and glycolysis, respectively.The formation of higher alcohols is leaded by the Ehrlich pathway (EP). Tryptofol (TOL), phenylethanol (2-PE), and tyrosol (TYROH) are phenolic compounds or fusel alcohols formed from Trp, Phe, and Tyr, respectively. Hydroxytyrosol (HT), indole-3-acetic acid (IAA), kynurenine (KYN), kynurenic acid (KA), serotonin (SER), melatonin (MEL).
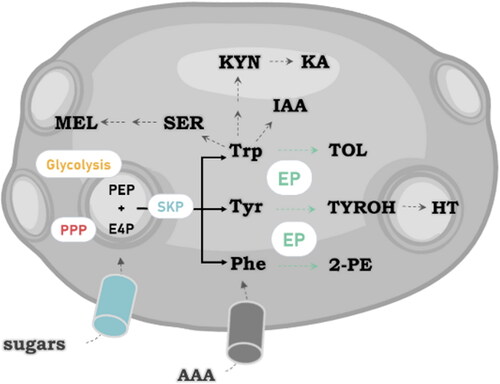
The role of yeasts in the transformation of PC has been studied in more detail in relation with flavor and aroma, such as fruity and floral aromas (isoamyl alcohol, 2-phenylethanol and esters) or as phenolic off-flavors (bioconversion of ferulic acid into 2-methoxy-4-vinylphenol or 4-vinylguaiacol) (Mertens et al. Citation2017). However, there is an increasing interest in the presence of bioactive compounds beneficial to health in foods derived from the aromatic amino acid metabolism during alcoholic fermentation. In this context, beer is a relevant source of tyrosol, one of the major phenolic compounds reported in this drink, with concentrations similar to those in wine (Boronat et al. Citation2020). Tyrosol is an aromatic alcohol produced by yeast during the alcoholic fermentation from the aromatic amino acid precursor tyrosine through the Ehrlich pathway (Ehrlich Citation1906; Muñiz-Calvo et al. Citation2020). However, this is not the only route for this polyphenol since, in a previous work, the formation of tyrosol was reported during the fermentation of a medium lacking amino acids. In fact, recent works describe that higher alcohol precursors originate from the catabolism of sugars rather than from the catabolism of consumed amino acids (Gallardo-Fernández et al. Citation2022). Thus, either by conversion of sugars or by catabolism of the amino acids present in the wort, yeasts play a key role in the presence of this nonvolatile alcohol during alcoholic fermentation. Tyrosol production in yeast has been shown to be promoted by nitrogen limitation whereas low glucose generally inhibited it (González et al. Citation2018). Tyrosol in beer ranges in concentrations from 3 to 40 mg/L and it has been found to play a role in the beer aroma and taste (Sánchez-Estébanez et al. Citation2018). Besides, tyrosol has been a target to increase during the brewing process (Soejima et al. Citation2012) and several methods have been described to obtain strains with an increased amount of tyrosol. It is clear that the wort composition and brewing conditions together with the choice of yeast strains to carry out the alcoholic fermentation contribute to the final concentration of higher alcohols. Metabolic interactions have been evidenced in the assimilation of amino acids between S. cerevisiae and T. delbrueckii during beer fermentation. With specific inoculum ratios and co-culture of both yeast species, beers displayed significantly higher amino acid assimilation as well as aromatic alcohol production compared to the beers fermented with monoculture. Banach and Ooi (Citation2014) studied the potential of increase of tyrosol and other aromatic alcohols during wort fermentation by increasing the levels of aromatic amino acids through kiwi fruit puree addition. The authors evidenced the importance of the yeast used to produce more fusel alcohols and attributed this greater production capacity to a highly active and efficient Ehrlich pathway.
The existence of natural diversity between yeast strains gives strain selection and hybridization a possible alternative to modify the production of tyrosol during brewing. Another strategy that leads to obtain strains that produce an increase in higher alcohols includes the use of toxic analogues of aromatic amino acids (Cordente et al. Citation2018). Through this approach, deregulation of allosteric checkpoints in amino acid biosynthesis is achieved. Using the phenylalanine analogs o-fluoro-DL-phenylalanine (OFP) and p-fluoro-DL-phenylalanine (PFP), wine yeasts strains have been obtained that are capable of overproducing the higher alcohol 2-phenylethanol. When the authors sequenced the obtained strains, they found that mutations in the key genes for the phenylalanine biosynthetic pathway, ARO4 and TYR1, were responsible for the high production of the higher alcohol (Cordente et al. Citation2018). Furthermore, in a subsequent work, several variants with the 2-phenylethanol overproduction phenotype were employed for Chardonnay juice fermentation. Among the five variants previously obtained (Cordente et al. Citation2018), the one carrying ARO4 mutations produced a 9-fold increase in tyrosol titer compared to the parental strain (Cordente et al. Citation2021). By this approach, but using specific tyrosine analogs, such as 2-fluoro-L-tyrosine, resistant mutants accumulating higher levels of tyrosol can be obtained. Also, breeding strategy with high tyrosol production has been achieved by combining tryptophan auxotrophy (trp3) and ethanol resistance in diploid industrial sake yeast strains (Soejima et al. Citation2012). Effective metabolic engineering strategies to generate yeast for high tyrosol titer has been reported recently (Bisquert et al. Citation2022; Liu et al. Citation2021). Finally, the use of genetic modification to produce strains that overproduce tyrosol by using CRISPR-based technologies, may be the most feasible strategy. This technology has been used to facilitate efficient breeding of brewer’s yeast or to improve the flavor and safety of wine (Mertens et al. Citation2019). In the same way and taking the advantage of the previous knowledge in bibliography for the key genes involved in tyrosine and tyrosol synthesis, it would be an easily affordable strategy introducing punctual mutations that release the enzymes from retro-inhibition or make them nonfunctional when they participate on competing pathways. In fact, Liu et al. (Citation2021) have achieved the highest tyrosol levels from glucose to date (9.90 g/L) using this strategy in 5 L fed-batch bioreactors.
The presence of tyrosol in beer is of great interest for several reasons related to the beneficial properties of this polyphenol. Due to its own characteristics, tyrosol has a high stability against autoxidation compared to other polyphenols, which contributes to its cellular antioxidant activity (Marković et al. Citation2019). Furthermore, tyrosol is the direct precursor to one of the greatest antioxidant compounds to date, hydroxytyrosol. In the human body, hydroxytyrosol is formed by hydroxylation of dietary tyrosol through two isoforms of cytochrome P450: CYP2A6 and CYP2D6 (Rodríguez-Morató et al. Citation2017). The main source of dietary hydroxytyrosol intake is virgin olive oil, and it is considered a bioactive health-promoting molecule in the Mediterranean diet. Furthermore, the presence of hydroxytyrosol in wine is also considerable and has recently been detected in beer (Boronat et al. Citation2020). In a randomized controlled clinical trial conducted by Soldevila-Domenech and colleagues, the authors evidenced that tyrosol present in beer was absorbed and biotransformed into hydroxytyrosol. Furthermore, nonalcoholic beers also led to an increase in hydroxytyrosol (Soldevila-Domenech et al. Citation2019). Although more studies should be carried out to evaluate the relevance of hydroxytyrosol biotransformation associated with responsible beer consumption, the results obtained open the doors to greater knowledge of the antioxidant composition of beer and its implication in health benefits.
Currently, there is a growing interest in the role that yeasts play in the production of hydroxytyrosol during alcoholic fermentation. The capability of several winemaking Saccharomyces and non-Saccharomyces yeast to produce hydroxytyrosol has been confirmed in both natural and synthetic must (Gallardo-Fernández et al. Citation2022; Rebollo-Romero et al. Citation2020) and in the intracellular compartment (Álvarez-Fernández et al. Citation2018). Due to the bioactive properties of hydroxytyrosol, the presence of this molecule in fermented beverages is very attractive. In fact, the effect of factors that can influence hydroxytyrosol levels during alcoholic fermentation such as the concentration of aromatic amino acids in the must, the temperature and the alcoholic degree have been studied in the wine context (Rebollo-Romero et al. Citation2020). However, the differences in the production capacity of different yeast strains seem to have a greater impact on the final concentration of this bioactive compound than the mentioned factors (Rebollo-Romero et al. Citation2020). Nevertheless, further research is required to explore the role of yeast and brewing conditions in the occurrence of this interesting compound during wort fermentation since it has been poorly investigated.
Improved analytical techniques coupled with an increased interest in what consumers eat and drink has led to the identification of molecules other than PCs that are present in smaller amounts but with much interest in their potential bioactivity. Among some of these molecules, neuroactive compounds derived from tryptophan include kynurenine, kynurenic acid, serotonin and melatonin. Several studies have focused their attention on the role of yeast in the transformation of tryptophan into all these bioactive molecules during alcoholic fermentation (Gallardo-Fernández et al. Citation2022; Muñiz-Calvo et al. Citation2019; Yılmaz and Gökmen Citation2018). Regarding metabolites involved in the kynurenine pathway, only few years ago it was shown for the first time the biosynthesis of kynurenine and kynurenic acid by two different brewer yeasts, belonging to the species S. cerevisiae and S. pastorianus, during beer fermentation (Yılmaz and Gökmen Citation2019). The amounts of kynurenine and kynurenic acid reported from beer analyses are within the range of 0.14 − 0.41 and 0.02 − 0.36 µM (Yılmaz and Gökmen Citation2018, Citation2019). On the other hand, melatonin concentrations in fermented products vary in the range from pg to ng per mL (Fernández-Cruz et al. Citation2017; Gallardo-Fernández et al. Citation2022;). In beer, amounts ranging 58-169 pg/mL of melatonin and also relatively higher amounts of the melatonin isomer (14.3 ng/mL) have been found (Garcia-Moreno, Calvo, and Maldonado Citation2013; Maldonado, Moreno, and Calvo Citation2009). Although these amounts may seem small, it has been seen that after drinking melatonin-containing beers, both melatonin and the total antioxidant status in human serum increased (Maldonado, Moreno, and Calvo Citation2009). Unlike what can occur with other antioxidants, melatonin (and also hydroxytyrosol) is able to cross the blood-brain barrier (Gallardo-Fernández et al. Citation2022). Melatonin has an antioxidant role and it has evidenced a protective effect on yeast against different fermentation-associated stresses such as oxidative stress and ethanol (Bisquert, Muñiz-Calvo, and Guillamón Citation2018). The first work in which melatonin produced by yeast was reported by Sprenger and colleagues (Sprenger et al. Citation1999). After that, several studies have evidenced that different yeast strains, from both Saccharomyces and non-Saccharomyces, synthesized melatonin at different levels (Fernández-Cruz et al. Citation2017; Muñiz-Calvo et al. Citation2017; Rodriguez-Naranjo et al. Citation2012). The production of melatonin seems to be linked to the growth phase (Rodriguez-Naranjo et al. Citation2012). Besides, melatonin has been shown to interact with different glycolytic proteins in both Saccharomyces and non-Saccharomyces yeasts in a fermentative capacity-dependent manner. All these results reinforce a possible role of this molecule in the process of adaptation of yeast to the changing environment that is alcoholic fermentation.
During beer fermentation, melatonin biosynthesis was monitored throughout the brewing process to elucidate the role of yeast in the beer enrichment. Yeast was found to be a major contributor to melatonin enrichment during alcoholic fermentation of this beverage. In a recent study, the occurrence of not only melatonin but other indolic compounds derived from L-tryptophan, such as 5-hydroxytryptophan, N-acetylserotonin or 3-indole acetic acid, along wort alcoholic fermentation and also in commercial beers has been reported (Fernández-Cruz et al. Citation2020). Although there are several recent studies focused on the production of melatonin in a fermentative context, research on the pathways and steps leading melatonin biosynthesis in yeast remains a partially unknown topic compared to other organisms such as plants or animals (Muñiz-Calvo et al. Citation2020). To shed light on this, two different works have been performed very recently. The first one consisted in the addition of melatonin intermediates to assess the bioconversion into new indolic compounds by S. cerevisiae cells in different media and growth stages (Muñiz-Calvo et al. Citation2019). In the second work, the authors used labeled tryptophan (15-N2 L-tryptophan) in order to more easily follow the new formation of serotonin, melatonin and related molecules (Gallardo-Fernández et al. Citation2022). From the results obtained from both studies, a hypothetical biosynthetic pathway for yeast melatonin has been proposed in which tryptophan is the initial substrate for both and serotonin a key intermediate. A better understanding of the aromatic amino acid metabolism will provide the potential tool to modulate all this set of molecules that can yield health beneficial effects and increase the levels of these molecules in final beers.
Yeast can also produce negative compounds from aminoacids as biogenic amines
Biogenic amines are toxic small nitrogenous bases generally produced by contaminant or spoilage bacteria in foods. Among the most important we can highlight cadaverine, tyramine, spermine, putrescine and histamine, which is the most toxic one causing migraines, nausea and digestive issues. During the catabolism of aminoacids, yeast can also produce those biogenic amines, through decarboxylation and transamination of aldehydes and ketones. Thus, the presence of these compounds in a yeast related food as beer must be studied and monitored, maintaining concentrations below to 100 mg/L. In several studies evaluating their content in commercial beers (Nalazek-Rudnicka, Wojnowski, and Wasik Citation2021; Lorencová et al. Citation2020), they observed several samples containing levels that exceeded the safety threshold, and an elevated variability among the levels of different biogenic amines has been found (Koller and Perkins Citation2022). Having all this information into account, the evaluation or the development of new yeast strains to produce and commercialize beer must include the evaluation of their ability to produce biogenic amines. For example, new strains from different species studied to produce nonalcoholic beer where evaluated for the production of biogenic amines and levels below detection limits were found (Methner et al. Citation2022). It has been shown that some species tend to produce higher levels, for example Brettanomyces sp. (Matukas et al. Citation2022). But more research regarding the factors that influence and promote the production of biogenic amines during the production of beer should be done in the next years to avoid the presence of this compound in certain beer products.
The beneficial potential of probiotic brewer’s yeast
Microorganism can have beneficial impact on human health due to the interactions with cells and among them. In this sense, (Zendeboodi et al. Citation2021) define probiotics as “viable or non-viable microbial cell (vegetative or spore; intact or broken) that is potentially healthy for the host”. In the last years, a lot of effort has been implemented to search and clarify which are the beneficial species and their effects. It has been shown that most probiotic microorganism with a confirmed beneficial effect belong to lactic acid bacteria as Bifidobacterium or Lactobacillus (Lucatto et al. Citation2020). But also yeast strains have proven their probiotic potential, as some S. cerevisiae strains known as S. boulardii (Panghal et al. Citation2018), although other yeast species have been claimed to have probiotic potential as Kluyveromyces marxianus or Pichia pastoris (Arevalo-Villena et al. Citation2017). The health-benefiting actions that had been associated to probiotics include the enhancement of the immune system, the prevention of constipation and the antibiotic-associated diarrhea (Kim et al. Citation2019). It seems that human gut microbiome-associated diseases as microbial dysbiosis, antibiotic-associated diarrhea, inflammatory bowel disease, Crohn’s disease and colorectal cancer are the health problems were the treatment with probiotics have a more promising impact.
Yeast are key players in the production of nutraceuticals and functional foods (Rai, Pandey, and Sahoo Citation2019). They can provide several valuable characteristics in the functional food industry: contribute with cell wall constituents (as β-glucan) or secreted extracellular fractions with bioactive metabolites (folate, carotenoids, γ-amino butyric acid), or specific enzymes responsible implicated in food components biotransformation and finally as live cells used as probiotics. In the case of S. boulardii, several potential benefits were proposed related to his probiotic action: a trophic effect on enterocytes due to polyamines activation (Jahn et al. Citation1996); a general immune stimulation attributed to the interaction with specific receptors of dendritic or innate immune cells (CitationBarreto-Bergter and Figueiredo 2014); an effect of physical barrier and colonization resistance (Swidsinski et al. Citation2009); an anti-inflammatory and antisecretory action (Pothoulakis Citation2009); an elimination of bacterial toxins and pathogen bindings (Tiago et al. Citation2012); and a nonspecific prebiotic effect due to the spillage of glucans, mannoproteins or chitin (Schneider et al. Citation2005).
All these health benefits of the S. boulardii strains have attracted the attention to different researches that have proposed its use as a brewing strain to produce probiotic beer with the aim of generate a functional food that vehicles these advantages to consumers (). The idea was proposed in 2015 (Spanish patent ES2583178B1) and since then, several studies have evaluated the use of S. boulardii in different types of brewing styles. The concern that these strains were not resistant enough to ethanol content was discarded (Ramírez-Cota et al. Citation2021) and different physicochemical, and metabolic analyses were performed showing acceptable fermentative capabilities (Mulero-Cerezo, Briz-Redón, and Serrano-Aroca Citation2019; Reitenbach, Iwassa, and Barros Citation2021; Senkarcinova et al. Citation2019; Silva et al. Citation2020, Citation2021). Interestingly, the sensory analyses showed satisfactory results with a slight decrease in some parameters (Mulero-Cerezo, Briz-Redón, and Serrano-Aroca Citation2019; Reitenbach, Iwassa, and Barros Citation2021; Senkarcinova et al. Citation2019) which promotes the production of these probiotic beer, considering the potential beneficial that these beers could provide.
Table 3. Summary of studies evaluating S. boulardii probiotic strains in brewing.
Conclusions
We have described the massive impact that yeast confers to beer production and the potential positive and negative effects that it can provide to this product. Although beer by itself contributes with a myriad of healthy nutrients, the negative impact of ethanol to multiple levels in the human body is limiting their future as a healthy food. In this sense, yeast can solve this limitation by fermenting beer with low ethanol content. Also selecting new yeasts with low levels of biogenic amines production will be important.
On top of that, we have described that yeast can contribute to other aspects related with healthy beers as the ones related with consumers with chronical illness as diabetes or celiac disease. Yeasts that produce low carbohydrate or gluten free beers can provide an appropriated product for these particular consumers.
Apart from reducing this negative health problems, yeasts were suggested to participate in the generation of positive healthy effects. The production of bioactive molecules by yeasts has claimed their role as a producer of very interesting compounds with important health benefits as tyrosol, hydroxytyrosol, serotonin or melatonin. Furthermore, the possibly of yeast to participate as a probiotic, besides to produce the beer fermentation, enhances the possibility to generate a positive impact in human health.
Most of these yeast healthy applications are still in development but some of them are being tested at industrial levels in brewing companies so it will not be surprising that some of them appear in the market in the next years to increase the healthy product range and enhance the people well-being.
Disclosure statement
No potential conflict of interest was reported by the authors.
Additional information
Funding
References
- Achón, M., M. Serrano, Á. García-González, E. Alonso-Aperte, and G. Varela-Moreiras. 2017. Present food shopping habits in the Spanish adultss population: A cross-sectional study. Nutrients 9 (5):508. doi: 10.3390/nu9050508.
- Álvarez-Fernández, M. A., E. Fernández-Cruz, E. Cantos-Villar, A. M. Troncoso, and M. C. García-Parrilla. 2018. Determination of hydroxytyrosol produced by winemaking yeasts during alcoholic fermentation using a validated UHPLC–HRMS method. Food Chemistry 242:345–51. doi: 10.1016/j.foodchem.2017.09.072.
- Arevalo-Villena, M., A. Briones-Perez, M. R. Corbo, M. Sinigaglia, A. Bevilacqua, and C. A. Bevilacqua. 2017. Biotechnological application of yeasts in food science: Starter cultures, probiotics and enzyme production. Journal of Applied Microbiology 123 (6):1360–72. doi: 10.1111/jam.13548.
- Baker, E. C., B. Wang, N. Bellora, D. Peris, A. B. Hulfachor, J. A. Koshalek, M. Adams, D. Libkind, and C. T. Hittinger. 2015. The genome sequence of saccharomyces eubayanus and the domestication of lager-brewing yeasts. Molecular Biology and Evolution 32 (11):2818–31. doi: 10.1093/molbev/msv168.
- Bakker, B. M., K. M. Overkamp, A. J. A. Van Maris, P. Kötter, M. A. H. Luttik, J. P. Van Dijken, and J. T. Pronk. 2001. Stoichiometry and compartmentation of NADH metabolism in Saccharomyces cerevisiae. FEMS Microbiology Reviews 25 (1):15–37. doi: 10.1016/S0168-6445(00)00039-5.
- Bamforth, C. W. 2002. Nutritional aspects of beer-a review. Nutrition Research 22 (1–2):227–37. doi: 10.1016/S0271-5317(01)00360-8.
- Banach, A. A., and B. G. Ooi. 2014. Enhancing the yields of phenolic compounds during fermentation using Saccharomyces cerevisiae strain 96581. Food and Nutrition Sciences 5 (21):2063–70. doi: 10.4236/fns.2014.521218.
- Barreto-Bergter, E., and R. T. Figueiredo. 2014. Fungal glycans and the innate immune recognition. In Frontiers in cellular and infection microbiology, 4, 145. doi: 10.3389/fcimb.2014.00145.
- Bascuñán, K. A., M. C. Vespa, and M. Araya. 2017. Celiac disease: Understanding the gluten-free diet. European Journal of Nutrition 56 (2):449–59. doi: 10.1007/S00394-016-1238-5.
- Baur, X., Z. Chen, and I. Sander. 1994. Isolation and denomination of an important allergen in baking additives: A -amylase from Aspergillus oryzae (Asp o II). Clinical and Experimental Allergy: Journal of the British Society for Allergy and Clinical Immunology 24 (5):465–70. doi: 10.1111/j.1365-2222.1994.tb00935.x.
- Bellut, K., M. Michel, M. Zarnkow, M. Hutzler, F. Jacob, D. P. Schutter, L. de Daenen, K. M. Lynch, E. Zannini, E. K. Arendt, et al. 2018. Application of non-saccharomyces yeasts isolated from Kombucha in the production of alcohol-free beer. Fermentation 4 (3):66. ( doi: 10.3390/fermentation4030066.
- Bindesboll Nielsen, N., and F. Schmidt. 1985. The fate of carbohydrates during fermentation of low calorie beer. Carlsberg Research Communications 50 (6):325–32. doi: 10.1007/BF02907155.
- Bisquert, R., S. Muñiz-Calvo, and J. M. Guillamón. 2018. Protective role of intracellular Melatonin against oxidative stress and UV radiation in Saccharomyces cerevisiae. Frontiers in Microbiology 9 (FEB):318. doi: 10.3389/fmicb.2018.00318.
- Bisquert, R., A. Planells‐Cárcel, E. Valera‐García, J. M. Guillamón, and S. Muñiz‐Calvo. 2022. Metabolic engineering of Saccharomyces cerevisiae for hydroxytyrosol overproduction directly from glucose. Microbial Biotechnology 15 (5):1499–510. doi: 10.1111/1751-7915.13957.
- Blanco, C. A., C. Andrés-Iglesias, and O. Montero. 2016. Low-alcohol beers: Flavor compounds, defects, and improvement strategies. Critical Reviews in Food Science and Nutrition 56 (8):1379–88. doi: 10.1080/10408398.2012.733979.
- Boronat, A., N. Soldevila-Domenech, J. Rodríguez-Morató, M. Martínez-Huélamo, R. M. Lamuela-Raventós, and R. de La Torre. 2020. Beer phenolic composition of simple phenols, prenylated flavonoids and alkylresorcinols. Molecules 25 (11):2582. doi: 10.3390/molecules25112582.
- Brinton, E. A. 2010. Effects of ethanol intake on lipoproteins and atherosclerosis. Current Opinion in Lipidology 21 (4):346–51. doi: 10.1097/MOL.0b013e32833c1f41.
- Cadière, A., A. Ortiz-Julien, C. Camarasa, and S. Dequin. 2011. Evolutionary engineered Saccharomyces cerevisiae wine yeast strains with increased in vivo flux through the pentose phosphate pathway. Metabolic Engineering 13 (3):263–71. doi: 10.1016/j.ymben.2011.01.008.
- Cambon, B., V. Monteil, F. Remize, C. Camarasa, and S. Dequin. 2006. Effects of GPD1 overexpression in Saccharomyces cerevisiae commercial wine yeast strains lacking ALD6 genes. Applied and Environmental Microbiology 72 (7):4688–94. doi: 10.1128/AEM.02975-05.
- Catallo, M., J. Nikulin, L. Johansson, K. Krogerus, M. Laitinen, F. Magalhães, M. Piironen, A. Mikkelson, C. L. Randazzo, L. Solieri, et al. 2020. Sourdough derived strains of Saccharomyces cerevisiae and their potential for farmhouse ale brewing. Journal of the Institute of Brewing 126 (2):168–75. doi: 10.1002/jib.608.
- Chambers, P. J., J. R. Bellon, S. A. Schmidt, C. Varela, and I. S. Pretorius. 2009. Non-genetic engineering approaches for isolating and generating novel yeasts for industrial applications. In Yeast biotechnology: Diversity and applications, 433–57. Netherlands: Springer. doi: 10.1007/978-1-4020-8292-4_20.
- Cherry, J. M., E. L. Hong, C. Amundsen, R. Balakrishnan, G. Binkley, E. T. Chan, K. R. Christie, M. C. Costanzo, S. S. Dwight, S. R. Engel, et al. 2012. Saccharomyces genome database: The genomics resource of budding yeast. Nucleic Acids Research 40 (Database issue):D700–D705. doi: 10.1093/nar/gkr1029.
- Coloretti, F., C. Zambonelli, and V. Tini. 2006. Characterization of flocculent Saccharomyces interspecific hybrids for the production of sparkling wines. Food Microbiology 23 (7):672–6. doi: 10.1016/j.fm.2005.11.002.
- Contreras, A., C. Hidalgo, P. A. Henschke, P. J. Chambers, C. Curtin, and C. Varela. 2014. Evaluation of non-Saccharomyces yeasts for the reduction of alcohol content in wine. Applied and Environmental Microbiology 80 (5):1670–8. doi: 10.1128/AEM.03780-13.
- Cordente, A. G., D. E. Nandorfy, M. Solomon, A. Schulkin, R. Kolouchova, I. L. Francis, and S. A. Schmidt. 2021. Aromatic higher alcohols in wine: Implication on aroma and palate attributes during chardonnay aging. Molecules 26 (16):4979. doi: 10.3390/molecules26164979.
- Cordente, A. G., M. Solomon, A. Schulkin, I. Leigh Francis, A. Barker, A. R. Borneman, and C. D. Curtin. 2018. Novel wine yeast with ARO4 and TYR1 mutations that overproduce ‘floral’ aroma compounds 2-phenylethanol and 2-phenylethyl acetate. Applied Microbiology and Biotechnology 102 (14):5977–88. doi: 10.1007/s00253-018-9054-x.
- Cordier, H., F. Mendes, I. Vasconcelos, and J. Francois. 2007. A metabolic and genomic study of engineered Saccharomyces cerevisiae strains for high glycerol production. Metabolic Engineering 9 (4):364–78. doi: 10.1016/j.ymben.2007.03.002.
- Crestani, C. C., A. Lopes da Silva, A. A. Scopinho, S. G. Ruginsk, E. T. Uchoa, F. M. A. Correa, L. L. K. Elias, J. Antunes-Rodrigues, and L. B. M. Resstel. 2014. Cardiovascular alterations at different stages of hypertension development during ethanol consumption: Time-course of vascular and autonomic changes. Toxicology and Applied Pharmacology 280 (2):245–55. doi: 10.1016/j.taap.2014.08.012.
- de Gaetano, G., S. Costanzo, A. Castelnuovo, L. di Badimon, D. Bejko, A. Alkerwi, G. Chiva-Blanch, R. Estruch, C. Vecchia, S. la Panico, et al. 2016. Effects of moderate beer consumption on health and disease: A consensus document. Nutrition, Metabolism, and Cardiovascular Diseases: NMCD 26 (6):443–67. doi: 10.1016/j.numecd.2016.03.007.
- Dequin, S., E. Baptista, and P. Barre. 1999. Acidification of grape musts by Saccharomyces cerevisiae wine yeast strains genetically engineered to produce lactic acid. American Journal of Enology and Viticulture 50:45–50.
- de Smidt, O., J. C. Du Preez, and J. Albertyn. 2012. Molecular and physiological aspects of alcohol dehydrogenases in the ethanol metabolism of Saccharomyces cerevisiae. FEMS Yeast Research 12 (1):33–47. doi: 10.1111/j.1567-1364.2011.00760.x.
- de Vuyst, L., H. Harth, S. van Kerrebroeck, and F. Leroy. 2016. Yeast diversity of sourdoughs and associated metabolic properties and functionalities. International Journal of Food Microbiology 239:26–34. doi: 10.1016/j.ijfoodmicro.2016.07.018.
- Dzialo, M. C., R. Park, J. Steensels, B. Lievens, and K. J. Verstrepen. 2017. Physiology, ecology and industrial applications of aroma formation in yeast. FEMS Microbiology Reviews 41 (Supp_1):S95–S128. doi: 10.1093/femsre/fux031.
- Ehrlich, F. 1906. Ehrlich pathway. Biochem Ztschr 2:1027–47.
- Fernández-Cruz, E., M. A. Álvarez-Fernández, E. Valero, A. M. Troncoso, and M. C. García-Parrilla. 2017. Melatonin and derived L-tryptophan metabolites produced during alcoholic fermentation by different wine yeast strains. Food Chemistry 217:431–7. doi: 10.1016/j.foodchem.2016.08.020.
- Fernández-Cruz, E., F. Carrasco-Galán, A. B. Cerezo-López, E. Valero, M. Á. Morcillo-Parra, G. Beltran, M. J. Torija, A. M. Troncoso, and M. C. García-Parrilla. 2020. Occurrence of melatonin and indolic compounds derived from L-tryptophan yeast metabolism in fermented wort and commercial beers. Food Chemistry 331:127192. doi: 10.1016/j.foodchem.2020.127192.
- Fernández-Solà, J. 2015. Cardiovascular risks and benefits of moderate and heavy alcohol consumption. Nature Reviews. Cardiology 12 (10):576–87. doi: 10.1038/nrcardio.2015.91.
- Fu, W., C. Liu, X. Meng, S. Tao, and W. Xue. 2021. Co-culture fermentation of Pediococcus acidilactici XZ31 and yeast for enhanced degradation of wheat allergens. International Journal of Food Microbiology 347:109190. doi: 10.1016/J.IJFOODMICRO.2021.109190.
- Gallardo-Fernández, M., J. Valls-Fonayet, E. Valero, R. Hornedo-Ortega, T. Richard, A. M. Troncoso, and M. C. Garcia-Parrilla. 2022. Isotopic labelling-based analysis elucidates biosynthesis pathways in Saccharomyces cerevisiae for Melatonin, Serotonin and Hydroxytyrosol formation. Food Chemistry 374:131742. doi: 10.1016/j.foodchem.2021.131742.
- Gallone, B., S. Mertens, J. L. Gordon, S. Maere, K. J. Verstrepen, and J. Steensels. 2018. Origins, evolution, domestication and diversity of Saccharomyces beer yeasts. Current Opinion in Biotechnology 49:148–55. doi: 10.1016/j.copbio.2017.08.005.
- Garcia-Moreno, H., J. R. Calvo, and M. D. Maldonado. 2013. High levels of melatonin generated during the brewing process. Journal of Pineal Research 55 (1):26–30. doi: 10.1111/jpi.12005.
- George, A., and V. M. Figueredo. 2010. Alcohol and arrhythmias: A comprehensive review. Journal of Cardiovascular Medicine (Hagerstown, MD) 11 (4):221–8. doi: 10.2459/JCM.0b013e328334b42d.
- Giannakou, K., F. Visinoni, P. Zhang, N. Nathoo, P. Jones, M. Cotterrell, U. Vrhovsek, and D. Delneri. 2021. Biotechnological exploitation of Saccharomyces jurei and its hybrids in craft beer fermentation uncovers new aroma combinations. Food Microbiology 100:103838. doi: 10.1016/j.fm.2021.103838.
- Gibson, B., J. M. A. Geertman, C. T. Hittinger, K. Krogerus, D. Libkind, E. J. Louis, F. Magalhães, and J. P. Sampaio. 2017. New yeasts-new brews: Modern approaches to brewing yeast design and development. FEMS Yeast Research 17 (4):1–13. doi: 10.1093/femsyr/fox038.
- Glissen Brown, J. R., and P. Singh. 2019. Coeliac disease. Paediatrics and International Child Health 39 (1):23–31. doi: 10.1080/20469047.2018.1504431.
- Gobbi, M., L. de Vero, L. Solieri, F. Comitini, L. Oro, P. Giudici, and M. Ciani. 2014. Fermentative aptitude of non-saccharomyces wine yeast for reduction in the ethanol content in wine. European Food Research and Technology 239 (1):41–8. doi: 10.1007/s00217-014-2187-y.
- González, B., J. Vázquez, M. Á. Morcillo-Parra, A. Mas, M. J. Torija, and G. Beltran. 2018. The production of aromatic alcohols in non-Saccharomyces wine yeast is modulated by nutrient availability. Food Microbiology 74:64–74. doi: 10.1016/j.fm.2018.03.003.
- Gonzalez, R., A. M. Guindal, J. Tronchoni, and P. Morales. 2021. Biotechnological approaches to lowering the ethanol yield during wine fermentation. Biomolecules 11 (11):1569–16. doi: 10.3390/biom11111569.
- Gumienna, M., and B. Górna. 2020. Gluten hypersensitivities and their impact on the production of gluten-free beer. European Food Research and Technology 246 (11):2147–60. doi: 10.1007/s00217-020-03579-9.
- Guzzo-Merello, G., M. Cobo-Marcos, M. Gallego-Delgado, and P. Garcia-Pavia. 2014. Alcoholic cardiomyopathy. World Journal of Cardiology 6 (8):771–81. doi: 10.4330/wjc.v6.i8.771.
- Hansen, M., W. Röcken, and C. Emeis. 1990. Construction of yeast strains for the production of low-carbonhydrate beer. Journal of the Institute of Brewing 96 (3):125–9. doi: 10.1002/j.2050-0416.1990.tb01022.x.
- Hu, F., Y. Niu, X. Xu, Q. Hu, Q. Su, and H. Zhang. 2020. Resistant dextrin improves high-fat-high-fructose diet induced insulin resistance. Nutrition & Metabolism 17 (1), 36. doi: 10.1186/s12986-020-00450-2.
- Jahn, H. U., R. Ullrich, T. Schneider, R. M. Liehr, H. L. Schieferdecker, H. Holst, and M. Zeitz. 1996. Immunological and trophical effects of saccharomyces boulardii on the small intestine in healthy human volunteers. Digestion 57 (2):95–104. doi: 10.1159/000201320.
- Johansson, L., J. Nikulin, R. Juvonen, K. Krogerus, F. Magalhães, A. Mikkelson, M. Nuppunen-Puputti, E. Sohlberg, G. de Francesco, G. Perretti, et al. 2021. Sourdough cultures as reservoirs of maltose-negative yeasts for low-alcohol beer brewing. Food Microbiology 94:103629. doi: 10.1016/j.fm.2020.103629.
- Kanny, D., R. D. Brewer, J. B. Mesnick, L. J. Paulozzi, T. S. Naimi, and H. Lu. 2015. Vital signs: Alcohol poisoning deaths - United States, 2010-2012. MMWR. Morbidity and Mortality Weekly Report 63 (53):1238–42.
- Kesmodel, U., K. Wisborg, S. F. Olsen, T. B. Henriksen, and N. J. Secher. 2002. Moderate alcohol intake in pregnancy and the risk of spontaneous abortion. Alcohol and Alcoholism (Oxford, Oxfordshire) 37 (1):87–92. doi: 10.1093/alcalc/37.1.87.
- Kim, M. K., J. Shin, S. S. Kweon, D. H. Shin, Y. H. Lee, B. Y. Chun, and B. Y. Choi. 2014. Harmful and beneficial relationships between alcohol consumption and subclinical atherosclerosis. Nutrition, Metabolism and Cardiovascular Diseases 24 (7):767–76. doi: 10.1016/j.numecd.2014.02.004.
- Kim, S. K., R. B. Guevarra, Y. T. Kim, J. Kwon, H. Kim, J. H. Cho, H. B. Kim, and J. H. Lee. 2019. Role of probiotics in human gut microbiome-associated diseases. Journal of Microbiology and Biotechnology 29 (9):1335–40. doi: 10.4014/jmb.1906.06064.
- Koller, H., and L. B. Perkins. 2022. Brewing and the chemical composition of amine-containing compounds in beer: A review. Foods 11 (3):257. doi: 10.3390/foods11030257.
- Koshinaka, K., R. Ando, and A. Sato. 2018. Shorttterm replacement of starch with isomaltulose enhances both insulinndependent and independent glucose uptake in rat skeletal muscle. Journal of Clinical Biochemistry and Nutrition 63 (2):113–22. doi: 10.3164/jcbn.17798.
- Krogerus, K., R. Eerikäinen, H. Aisala, and B. Gibson. 2022. Repurposing brewery contaminant yeast as production strains for low-alcohol beer fermentation. Yeast 39 (1–2):156–69. doi: 10.1002/yea.3674.
- Krogerus, K., and B. Gibson. 2020. A re-evaluation of diastatic Saccharomyces cerevisiae strains and their role in brewing. Applied Microbiology and Biotechnology 104 (9):3745–56. doi: 10.1007/s00253-020-10531-0.
- Krogerus, K., F. Magalhães, J. Kuivanen, and B. Gibson. 2019. A deletion in the STA1 promoter determines maltotriose and starch utilization in STA1+ Saccharomyces cerevisiae strains. Applied Microbiology and Biotechnology 103 (18):7597–615. doi: 10.1007/s00253-019-10021-y.
- Krogerus, K., F. Magalhães, V. Vidgren, and B. Gibson. 2017. Novel brewing yeast hybrids: Creation and application. Applied Microbiology and Biotechnology 101 (1):65–78. doi: 10.1007/s00253-016-8007-5.
- Kutyna, D. R., C. Varela, P. A. Henschke, P. J. Chambers, and G. A. Stanley. 2010. Microbiological approaches to lowering ethanol concentration in wine. Trends in Food Science & Technology 21 (6):293–302. doi: 10.1016/j.tifs.2010.03.004.
- Lairón-Peris, M., L. Pérez-Través, S. Muñiz-Calvo, J. M. Guillamón, J. M. Heras, E. Barrio, and A. Querol. 2020. Differential contribution of the parental genomes to a S. cerevisiae × S. uvarum hybrid, inferred by phenomic, genomic, and transcriptomic analyses, at different industrial stress conditions. Frontiers in Bioengineering and Biotechnology 8 (129):129. doi: 10.3389/fbioe.2020.00129.
- Leskovac, V., S. Trivić, and D. Peričin. 2002. The three zinc-containing alcohol dehydrogenases from baker’s yeast, Saccharomyces cerevisiae. FEMS Yeast Research 2 (4):481–94. doi: 10.1016/S1567-1356(02)00157-5.
- Liu, H., Y. Tian, Y. Zhou, Y. Kan, T. Wu, W. Xiao, and Y. Luo. 2021. Multi-modular engineering of Saccharomyces cerevisiae for high-titre production of tyrosol and salidroside. Microbial Biotechnology 14 (6):2605–16. doi: 10.1111/1751-7915.13667.
- Liu, L., J. Wang, D. Rosenberg, H. Zhao, G. Lengyel, and D. Nadel. 2018. Fermented beverage and food storage in 13,000 y-old stone mortars at Raqefet Cave, Israel: Investigating Natufian ritual feasting. Journal of Archaeological Science: Reports 21:783–93. doi: 10.1016/j.jasrep.2018.08.008.
- Lorencová, E., R. H. Salek, M. Černíková, L. Buňková, A. Hýlková, and F. Buňka. 2020. Biogenic amines occurrence in beers produced in Czech microbreweries. Food Control 117:107335. doi: 10.1016/j.foodcont.2020.107335.
- Lucatto, J. N., R. A. da Silva-Buzanello, S. N. T. G. de Mendonça, T. C. Lazarotto, J. L. Sanchez, E. Bona, and D. A. Drunkler. 2020. Performance of different microbial cultures in potentially probiotic and prebiotic yoghurts from cow and goat milks. International Journal of Dairy Technology 73 (1):144–56. doi: 10.1111/1471-0307.12655.
- Magyar, I., and T. Tóth. 2011. Comparative evaluation of some oenological properties in wine strains of Candida stellata, Candida zemplinina, Saccharomyces uvarum and Saccharomyces cerevisiae. Food Microbiology 28 (1):94–100. doi: 10.1016/j.fm.2010.08.011.
- Maldonado, M. D., H. Moreno, and J. R. Calvo. 2009. Melatonin present in beer contributes to increase the levels of melatonin and antioxidant capacity of the human serum. Clinical Nutrition (Edinburgh, Scotland) 28 (2):188–91. doi: 10.1016/j.clnu.2009.02.001.
- Marković, A. K., J. Torić, M. Barbarić, and C. J. Brala. 2019. Hydroxytyrosol, tyrosol and derivatives and their potential effects on human health. Molecules 24 (10):2001–39. doi: 10.3390/molecules24102001.
- Martinez-Gomez, A., I. Caballero, and C. A. Blanco. 2020. Phenols and melanoidins as natural antioxidants in beer. Structure, reactivity and antioxidant activity. Biomolecules 10 (3):400. doi: 10.3390/biom10030400.
- Mateo-Gallego, R., S. Pérez-Calahorra, I. Lamiquiz-Moneo, V. Marco-Benedí, A. M. Bea, A. J. Fumanal, A. Prieto-Martín, M. Laclaustra, A. Cenarro, and F. Civeira. 2020. Effect of an alcohol-free beer enriched with isomaltulose and a resistant dextrin on insulin resistance in diabetic patients with overweight or obesity. Clinical Nutrition (Edinburgh, Scotland) 39 (2):475–83. doi: 10.1016/j.clnu.2019.02.025.
- Matukas, M., V. Starkute, E. Zokaityte, G. Zokaityte, D. Klupsaite, E. Mockus, J. M. Rocha, R. Ruibys, and E. Bartkiene. 2022. Effect of different yeast strains on biogenic amines, volatile compounds and sensory profile of beer. Foods 11 (15):2317. doi: 10.3390/foods11152317.
- Meier-Dörnberg, T., O. I. Kory, F. Jacob, M. Michel, and M. Hutzler. 2018. Saccharomyces cerevisiae variety diastaticus friend or foe?-spoilage potential and brewing ability of different Saccharomyces cerevisiae variety diastaticus yeast isolates by genetic, phenotypic and physiological characterization. FEMS Yeast Research 18 (4), foy023. doi: 10.1093/femsyr/foy023.
- Mertens, S., B. Gallone, J. Steensels, B. Herrera-Malaver, J. Cortebeek, R. Nolmans, V. Saels, V. K. Vyas, and K. J. Verstrepen. 2019. Reducing phenolic off-flavors through CRISPR-based gene editing of the FDC1 gene in Saccharomyces cerevisiae x Saccharomyces eubayanus hybrid lager beer yeasts. Plos ONE 14 (1):e0209124. doi: 10.1371/journal.pone.0209124.
- Mertens, S., J. Steensels, B. Gallone, B. Souffriau, P. Malcorps, and K. J. Verstrepen. 2017. Rapid screening method for phenolic off-flavor (POF) production in yeast. Journal of the American Society of Brewing Chemists 75 (4):318–23. doi: 10.1094/ASBCJ-2017-4142-01.
- Methner, Y., N. Weber, O. Kunz, M. Zarnkow, M. Rychlik, M. Hutzler, and F. Jacob. 2022. Investigations into metabolic properties and selected nutritional metabolic by-products of different non-saccharomyces yeast strains when producing non-alcoholic beer. FEMS Yeast Research, foac042. doi: 10.1093/femsyr/foac042.
- Mulero-Cerezo, J., Á. Briz-Redón, and Á. Serrano-Aroca. 2019. Saccharomyces cerevisiae var. boulardii: Valuable probiotic starter for craft beer production. Applied Sciences 9 (16):3250. doi: 10.3390/app9163250.
- Muñiz-Calvo, S., R. Bisquert, E. Fernández-Cruz, M. C. García-Parrilla, and J. M. Guillamón. 2019. Deciphering the melatonin metabolism in Saccharomyces cerevisiae by the bioconversion of related metabolites. Journal of Pineal Research 66 (3):e12554. doi: 10.1111/jpi.12554.
- Muñiz-Calvo, S., R. Bisquert, S. Puig, and J. M. Guillamón. 2020. Overproduction of hydroxytyrosol in Saccharomyces cerevisiae by heterologous overexpression of the Escherichia coli 4-hydroxyphenylacetate 3-monooxygenase. Food Chemistry 308:125646. doi: 10.1016/j.foodchem.2019.125646.
- Muñiz-Calvo, S., J. M. Guillamón, I. Domínguez, and A. Doménech-Carbó. 2017. Detecting and monitoring the production of melatonin and other related indole compounds in different saccharomyces strains by solid-state electrochemical techniques. Food Analytical Methods 10 (5):1408–18. doi: 10.1007/s12161-016-0699-8.
- Nalazek-Rudnicka, K., W. Wojnowski, and A. Wasik. 2021. Occurrence and levels of biogenic amines in beers produced by different methods. Foods 10 (12):2902. doi: 10.3390/foods10122902.
- Ogata, T., Y. Iwashita, and T. Kawada. 2017. Construction of a brewing yeast expressing the glucoamylase gene STA1 by mating. Journal of the Institute of Brewing 123 (1):66–9. doi: 10.1002/jib.394.
- Oliveira, G., A. E. Beezer, J. Hadgraft, and M. E. Lane. 2010. Alcohol enhanced permeation in model membranes. Part I. Thermodynamic and kinetic analyses of membrane permeation. International Journal of Pharmaceutics 393 (1–2):61–7. doi: 10.1016/j.ijpharm.2010.03.062.
- Orman, E. S., G. Odena, and R. Bataller. 2013. Alcoholic liver disease: Pathogenesis, management, and novel targets for therapy. Journal of Gastroenterology and Hepatology 28 (SUPPL 1):77–84. doi: 10.1111/jgh.12030.
- Panghal, A., S. Janghu, K. Virkar, Y. Gat, V. Kumar, and N. Chhikara. 2018. Potential non-dairy probiotic products – A healthy approach. Food Bioscience 21:80–9. doi: 10.1016/j.fbio.2017.12.003.
- Park, J. Y., J. Y. Lee, S. H. Choi, H. M. Ko, I. C. Kim, H. B. Lee, and S. Bai. 2014. Construction of dextrin and isomaltose-assimilating brewer’s yeasts for production of low-carbohydrate beer. Biotechnology Letters 36 (8):1693–9. doi: 10.1007/s10529-014-1530-5.
- Peris, D., W. G. Alexander, K. J. Fisher, R. v Moriarty, M. G. Basuino, E. J. Ubbelohde, R. L. Wrobel, and C. T. Hittinger. 2020. Synthetic hybrids of six yeast species. Nature Communications 11 (1):2085. doi: 10.1038/s41467-020-15559-4.
- Petersen, M. C., and G. I. Shulman. 2018. Mechanisms of insulin action and insulin resistance. Physiological Reviews 98 (4):2133–223. doi: 10.1152/physrev.
- Pietraszek, A., S. Gregersen, and K. Hermansen. 2010. Alcohol and type 2 diabetes. A review. Nutrition, Metabolism, and Cardiovascular Diseases: NMCD 20 (5):366–75. doi: 10.1016/j.numecd.2010.05.001.
- Piškur, J., E. Rozpedowska, S. Polakova, A. Merico, and C. Compagno. 2006. How did Saccharomyces evolve to become a good brewer? Trends in Genetics: TIG 22 (4):183–6. doi: 10.1016/J.TIG.2006.02.002.
- Pothoulakis, C. 2009. Review article: Anti-inflammatory mechanisms of action of Saccharomyces boulardii. Alimentary Pharmacology & Therapeutics 30 (8):826–33. doi: 10.1111/j.1365-2036.2009.04102.x.
- Rai, A. K., A. Pandey, and D. Sahoo. 2019. Biotechnological potential of yeasts in functional food industry. Trends in Food Science & Technology 83:129–37. doi: 10.1016/j.tifs.2018.11.016.
- Ramírez-Cota, G. Y., E. O. López-Villegas, A. R. Jiménez-Aparicio, and H. Hernández-Sánchez. 2021. Modeling the ethanol tolerance of the probiotic yeast saccharomyces cerevisiae var. boulardii CNCM I-745 for its possible use in a functional beer. Probiotics and Antimicrobial Proteins 13 (1):187–94. doi: 10.1007/s12602-020-09680-5.
- Rebollo-Romero, I., E. Fernández-Cruz, F. Carrasco-Galán, E. Valero, E. Cantos-Villar, A. B. Cerezo, A. M. Troncoso, and M. C. Garcia-Parrilla. 2020. Factors influencing the production of the antioxidant hydroxytyrosol during alcoholic fermentation: Yeast strain, initial tyrosine concentration and initial must. LWT 130 (109631):109631. doi: 10.1016/j.lwt.2020.109631.
- Reitenbach, A. F., I. J. Iwassa, and B. C. B. Barros. 2021. Production of functional beer with the addition of probiotic: Saccharomyces boulardii. Research, Society and Development 10 (2):e5010212211. doi: 10.33448/rsd-v10i2.12211.
- Rimm, E. B., P. Williams, K. Fosher, M. Criqui, and M. J. Stampfer. 1999. Moderate alcohol intake and lower risk of coronary heart disease: Meta-analysis of effects on lipids and haemostatic factors. BMJ (Clinical Research ed.) 319 (7224):1523–8. doi: 10.1136/bmj.319.7224.1523.
- Rodríguez-Morató, J., P. Robledo, J. A. Tanner, A. Boronat, C. Pérez-Mañá, C. Y. Oliver Chen, R. F. Tyndale, and R. de la Torre. 2017. CYP2D6 and CYP2A6 biotransform dietary tyrosol into hydroxytyrosol. Food Chemistry 217:716–25. doi: 10.1016/j.foodchem.2016.09.026.
- Rodriguez-Naranjo, M. I., M. J. Torija, A. Mas, E. Cantos-Villar, and M. D. C. Garcia-Parrilla. 2012. Production of melatonin by Saccharomyces strains under growth and fermentation conditions. Journal of Pineal Research 53 (3):219–24. doi: 10.1111/j.1600-079X.2012.00990.x.
- Ruiz-Ruiz, J. C., G. Aldana, C. E. del, A. I. C. Cruz, and M. R. Segura-Campos. 2019. Antioxidant activity of polyphenols extracted from hop used in craft beer. ACTA PORTUGUESA DE NUTRIÇÃO, 25:84–89. doi: 10.21011/apn.2021.2515.
- Rumgay, H., K. Shield, H. Charvat, P. Ferrari, B. Sornpaisarn, I. Obot, F. Islami, V. E. P. P. Lemmens, J. Rehm, and I. Soerjomataram. 2021. Global burden of cancer in 2020 attributable to alcohol consumption: A population-based study. The Lancet Oncology 22 (8):1071–80. doi: 10.1016/S1470-2045(21)00279-5.
- Ryan, J., S. C. Hutchings, Z. Fang, N. Bandara, S. Gamlath, S. Ajlouni, and C. S. Ranadheera. 2020. Microbial, physico-chemical and sensory characteristics of mango juice-enriched probiotic dairy drinks. International Journal of Dairy Technology 73 (1):182–90. doi: 10.1111/1471-0307.12630.
- Saada, O. A., A. Tsouris, C. Large, A. Friedrich, M. J. Dunham, and J. Schacherer. 2022. Phased polyploid genomes provide deeper insight into the multiple origins of domesticated Saccharomyces cerevisiae beer yeasts. Current Biology 32 (6):1350–61.e3. doi: 10.1016/j.cub.2022.01.068.
- Sakandar, H. A., K. Usman, and M. Imran. 2018. Isolation and characterization of gluten-degrading Enterococcus mundtii and Wickerhamomyces anomalus, potential probiotic strains from indigenously fermented sourdough (Khamir). LWT 91:271–7. doi: 10.1016/j.lwt.2018.01.023.
- Sánchez-Estébanez, C., S. Ferrero, C. M. Alvarez, F. Villafañe, I. Caballero, and C. A. Blanco. 2018. Nuclear Magnetic Resonance methodology for the analysis of regular and non-alcoholic lager beers. Food Analytical Methods 11 (1):11–22. doi: 10.1007/s12161-017-0953-8.
- Schneider, S. M., F. Girard-Pipau, J. Filippi, X. Hébuterne, D. Moyse, G. C. Hinojosa, A. Pompei, P. R. Elsevier, and P. Rampal. 2005. Effects of Saccharomyces boulardii on fecal short-chain fatty acids and microflora in patients on long-term total enteral nutrition. World Journal of Gastroenterology 11 (39):6165–9. doi: 10.3748/wjg.v11.i39.6165.
- Selecký, R., D. Šmogrovičová, and P. Sulo. 2008. Beer with reduced ethanol content produced using Saccharomyces cerevisiae yeasts deficient in various tricarboxylic acid cycle enzymes. Journal of the Institute of Brewing 114 (2):97–101. doi: 10.1002/j.2050-0416.2008.tb00312.x.
- Senkarcinova, B., I. A. G. Dias, J. Nespor, and T. Branyik. 2019. Probiotic alcohol-free beer made with Saccharomyces cerevisiae var. boulardii. LWT 100:362–7. doi: 10.1016/j.lwt.2018.10.082.
- Shield, K. D., C. Parry, and J. Rehm. 2013. Chronic diseases and conditions related to alcohol use. Alcohol Research: Current Reviews 35 (2):155–73.
- Sievenpiper, J. L. 2020. Low-carbohydrate diets and cardiometabolic health: The importance of carbohydrate quality over quantity. Nutrition Reviews 78 (Suppl 1):69–77. doi: 10.1093/NUTRIT/NUZ082.
- Silva, L. C., H. de Souza Lago, M. O. T. Rocha, V. S. de Oliveira, R. Laureano-Melo, E. T. G. Stutz, B. P. de Paula, J. F. P. Martins, R. H. Luchese, A. F. Guerra, et al. 2021. Craft beers fermented by potential probiotic yeast or lacticaseibacilli strains promote antidepressant-like behavior in Swiss webster mice. Probiotics and Antimicrobial Proteins 13 (3):698–708. doi: 10.1007/s12602-020-09736-6.
- Silva, L. C., G. B. Schmidt, L. G. O. Alves, V. S. Oliveira, R. Laureano-Melo, E. Stutz, J. F. P. Martins, B. P. Paula, R. H. Luchese, A. F. Guerra, et al. 2020. Use of probiotic strains to produce beers by axenic or semi-separated co-culture system. Food and Bioproducts Processing 124:408–18. doi: 10.1016/j.fbp.2020.10.001.
- Soejima, H., K. Tsuge, T. Yoshimura, K. Sawada, and H. Kitagaki. 2012. Breeding of a high tyrosol-producing sake yeast by isolation of an ethanol-resistant mutant from a TRP3 mutant. Journal of the Institute of Brewing 118 (3):264–8. doi: 10.1002/jib.46.
- Soldevila-Domenech, N., A. Boronat, J. Mateus, P. Diaz-Pellicer, I. Matilla, M. Pérez-Otero, A. Aldea-Perona, and R. de La Torre. 2019. Generation of the antioxidant hydroxytyrosol from tyrosol present in beer and red wine in a randomized clinical trial. Nutrients 11 (9):2241. doi: 10.3390/nu11092241.
- Spitaels, F., A. D. Wieme, M. Janssens, M. Aerts, H. M. Daniel, A. van Landschoot, L. de Vuyst, and P. Vandamme. 2014. The microbial diversity of traditional spontaneously fermented lambic beer. PLoS One. 9 (4):e95384. doi: 10.1371/journal.pone.0095384.
- Sprenger, J., R. Hardeland, B. Fuhrberg, and S.-Z. Han. 1999. Melatonin and other 5-methoxylated indoles in yeast. Presence in high concentrations and dependence on tryptophan availability. The Japan Mendel Society, Cytología, 64:209–13.
- Swidsinski, A., V. Ung, B. C. Sydora, V. Loening-Baucke, Y. Doerffel, H. Verstraelen, and R. N. Fedorak. 2009. Bacterial overgrowth and inflammation of small intestine after carboxymethylcellulose ingestion in genetically susceptible mice. Inflammatory Bowel Diseases 15 (3):359–64. doi: 10.1002/ibd.20763.
- Thomson, J. M., Gaucher, E. A. Burgan, M. F. de Kee, D. W., Li, T. Aris, J. P, and Benner, S. A. 2005. Resurrecting ancestral alcohol dehydrogenases from yeast. Nature Genetics 37 (6):630–5. doi: 10.1038/ng1553.
- Tiago, F. C. P., F. S. Martins, E. L. S. Souza, P. F. P. Pimenta, H. R. C. Araujo, I. M. Castro, R. L. Brandão, and J. R. Nicoli. 2012. Adhesion to the yeast cell surface as a mechanism for trapping pathogenic bacteria by Saccharomyces probiotics. Journal of Medical Microbiology 61 (Pt 9):1194–207. doi: 10.1099/jmm.0.042283-0.
- Tilloy, V., A. Ortiz-Julien, and S. Dequin. 2014. Reduction of ethanol yield and improvement of glycerol formation by adaptive evolution of the wine yeast saccharomyces cerevisiae under hyperosmotic conditions. Applied and Environmental Microbiology 80 (8):2623–32. doi: 10.1128/AEM.03710-13.
- Troilo, A., de Francesco, G., Marconi, O. Sileoni, V. Turchetti, B, and Perretti, G. 2020. Low carbohydrate beers produced by a selected yeast strain from an alternative source. Journal of the American Society of Brewing Chemists 78 (1):80–8. doi: 10.1080/03610470.2019.1682887.
- Varela, C., P. R. Dry, D. R. Kutyna, I. L. Francis, P. A. Henschke, C. D. Curtin, and P. J. Chambers. 2015. Strategies for reducing alcohol concentration in wine. Australian Journal of Grape and Wine Research 21:670–9. doi: 10.1111/ajgw.12187.
- Varela, J., and C. Varela. 2019. Microbiological strategies to produce beer and wine with reduced ethanol concentration. Current Opinion in Biotechnology 56:88–96. doi: 10.1016/j.copbio.2018.10.003.
- Xu, P., S. Y. Zhang, Z. G. Luo, M. H. Zong, X. X. Li, and W. Y. Lou. 2021. Biotechnology and bioengineering of pullulanase: State of the art and perspectives. In World journal of microbiology and biotechnology, Vol. 37. Springer Science and Business Media B.V. doi: 10.1007/s11274-021-03010-9.
- Yılmaz, C., and V. Gökmen. 2018. Determination of tryptophan derivatives in kynurenine pathway in fermented foods using liquid chromatography tandem mass spectrometry. Food Chemistry 243:420–7. doi: 10.1016/j.foodchem.2017.10.004.
- Yılmaz, C., and V. Gökmen. 2019. Kinetic evaluation of the formation of tryptophan derivatives in the kynurenine pathway during wort fermentation using Saccharomyces pastorianus and Saccharomyces cerevisiae. Food Chemistry 297 (124975):124975. doi: 10.1016/j.foodchem.2019.124975.
- Zamakhchari, M., G. Wei, F. Dewhirst, J. Lee, D. Schuppan, F. G. Oppenheim, and E. J. Helmerhorst. 2011. Identification of rothia bacteria as gluten-degrading natural colonizers of the upper gastro-intestinal tract. PLoS One 6 (9):e24455. doi: 10.1371/journal.pone.0024455.
- Zendeboodi, F., M. M. Gholian, E. Khanniri, S. Sohrabvandi, and A. M. Mortazavian. 2021. Beer as a vehicle for probiotics. Applied Food Biotechnology 8 (4):329–37. doi: 10.22037/afb.v8i4.35303.