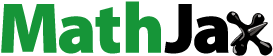
ABSTRACT
Our study specifically delves into the synthesis of AgNPs utilizing Tinospora malabarica leaf extract fractions (Tm-LMEFs) and explore their efficacy in combating the larvae have progressed to the synthesized AgNPs (Tm-AgNPS) and the leaf methanol extract fractions (LMEFs), our investigation focused on their impact on the targeted larvae species over a 24-h exposure period. Significantly noteworthy mortality rates were observed across all Spodoptera litura and Helicoverpa armigera post-treatment, indicating pronounced efficacy. Notably, the Tm-AgNPS exhibited higher toxicity compared to the LMEFs these selected polyphagous insect pests. Quantitative analysis yielded the highest mortality (98.99%/90.44% and 55.33%/49.60%) values for the synthesized Tm-AgNPs against S. litura and H. armigera, with no observed mortality in the control group. This study marks the first instance of exploring both Tm-LMEFs, Tm-AgNPs and enzyme activity have toxicity properties against selected agriculture pests, highlighting their promising role in pest management and environmental sustainability.
Introduction
Globally, Spodoptera litura is a predominant polyphagous pest, a strong flier and disperses long distance annually during the summer months, and which is occupied a wide range of hosting around 200 species floral and 74 species showed from India, also it is economically important crops throughout tropical and subtropical Asia [Citation1]. However, due to the larvae’s consumption of various host flora sections, including rhizomes, which severely damaged them and lost over 60% their revenue, India’s agriculture has recently suffered significant economic losses [Citation2]. Agronomically speaking, Helicoverpa armigera is a polyphagous pest that has been shown to devour over 500 different plant communities globally based on a variety of environmental conditions [Citation3]. Moreover, it is gaining resistance power in almost all chemical pesticides damaging the cash crops like tobacco, groundnut, pulses, castor, etc., and also developed resistance in almost all commercially available chemical pesticides [Citation4].
In India, agriculture is the backbone of the economy, supporting nearly 75% of rural areas. The green revolution in our country, while ushering in the much-needed self-sufficiency in food production, also paved the way for intensive use of harmful chemical pesticides [Citation5]. Scientists and researchers have been working extremely hard to develop novel control tactics and newer synthetic chemical insecticides (SCIs), which are typically derived from naturally occurring phyto-compounds (PCs) [Citation6,Citation7]. Additionally, PCs have little to no negative effects on the biological system and other relevant non-target animals and vegetation [Citation8–10]. Moreover, PCs have multifaceted applications globally [Citation11,Citation12]. Tinospora malabarica (Lam.) belongs to the family Menispermaceae and is endemic to India. It is common throughout tropical and subtropical zones in terminal zones and available in the areas of Karnataka, Assam, Orissa Andhra Pradesh, Arunachal Pradesh, Kerala and Bihar in India. According to ayurvedic nighantus, it is mainly indicated in fever, poisonous conditions, wrinkles, premature greying of hair, etc. [Citation13]. The insecticidal activity of T. malabarica leaf methanol fractions (Tm-LMF) and Tm-AgNPs against agricultural pests, such as H. armigera and S. litura, has been documented firsthand. We performed a number of spectral and microscopic investigations on Tm-LMF and Tm-AgNPs.
Materials and methods
Flora collection and extraction
The green leaves and well-cleaned Tinospora malabarica (Menispermacea) leaves () were collected from at various places of Poompuhar village (Melaiyur, Keelaiyur and Sri Ankalamman Temple) (Latitude: 11°14 to 11°15 N; Longitude: 79°84 to 79°85 E), Mayiladuthurai district, Tamil Nadu, India. The voucher specimen was assigned a number and stored for future use at our research facility. The green leaves (T. malabarica) were finely pulverised in an electric grinder after being dried in the shade (). An aqueous extract was prepared by combining 500 mL of cooled, heated distilled water with 50 g of dried leaf powder. The mixture was continuously agitated using a magnetic stirrer [Citation14]. During a 3-h incubation stage, the dry leaf powder suspension was filtered using Whatman no. 1 filter paper, the filtrate was subsequently stoned in an amber-coloured airtight bottle at a temperature of 10°C until it needs to be used ().
Phytochemical screening
Utilising the methods outlined by Senthil Kumar et al. [Citation15], as a framework, we examined the quality of various organic components in the effective LME.
TLC and CC analysis
PCs with insecticidal and enzyme activity source were searched for using the Tm-LME. The CC was packed with a 50 cm height by 50 mL capacity silica gel column and a pre-coated TLC sheet was used for Tm-LME. The column was also diluted with different solvent system containing ethyl acetate and diethyl ether [Citation16].
Silver nanoparticle (AgNP) synthesis
Green reduction was used in a synthetic process to produce Tm-AgNPs. Twenty grams of finely chopped, thoroughly cleaned leaves and one hundred millilitres of sterile, double-distilled water were added to a 300 millilitre Erlenmeyer flask to create the broth solution. Following a five-minute boil, the mixture was decanted. First, the extract was filtered using Whatman filter paper. The filtrate in an Erlenmeyer flask was mixed with an aqueous 1 mM AgNO3 (21.2 mg of AgNO3 powder in 125 mL Milli-Q water) and left to incubate at room temperature. The mixture could be utilised within a week and stored at 15°C. Using 12 millilitre of leaf extract, an 88-millilitre aqueous solution containing 1 millimole silver nitrate was reduced for 10 min at room temperature. The outcome demonstrated the creation of Tm-AgNPs in a green solution [Citation17].
Characterisation of AgNps
UV–vis spectroscopy makes it simple to observe the synthesis of AgNPs solution leaf extract. By measuring the UV–vis spectra and frequently sampling 1 mL aliquots of the aqueous components after 20 times dilution, the bioreduction of Ag+ ions in solutions was observed. The ultraviolet-visible spectra of these aliquots were monitored as a function of reaction time using a Shimadzu 1601 spectrophotometer that operated in the 200–700 nm range with a resolution of 1 nm. Following a 40-min centrifugation at 60,000 × g of the reaction mixture, the pellet was dissolved in distilled water and filtered using a Millipore filter (0.45 μm). A JASCO FT/IR-6600 spectrum one instrument was employed to analyse the sample’s FTIR spectra utilising an aliquot of this filtrate that included silver nanoparticles in a diluted form. The images of the nanoparticles were examined using scanning electron microscopy (SEM; 3TESCAN, Czech Republic, Model Vega: Beam Energy; 200 eV to 30 kV) to observe the particle’s shape. The pellet of silver nanoparticles was redispersed into 10 millilitres of deionised water after the silver nanoparticle solution was centrifuged repeatedly for 20 min at 5000 rpm. Following the purified copper particle freeze-drying process, the structure and composition were examined using X-ray diffraction (XRD), a Pro X-ray diffractometer and a voltmeter.
Polyphagous pests rearing
The polyphagous pests H. armigera and S. litura, their egg/eggs masses, larvae, pupae and adults () were collected during dawn and dusk from groundnut and bendi field in and around Sirkazhi Taluk (Latitude: 11°23’78‘N; Longitude: 79°71’39’ E), Mayiladuthurai District of Tamil Nadu in India. Both S. lituraand H. armigera were cultivated on castor and bendi fruits, respectively, under laboratory circumstances. The rearing parameters included a 12-h photoperiod at 28 ± 2°C and 75 ± 5% relative humidity. In the neighbourhood of agricultural fields of Sirkazhi, Tamil Nadu, wild moths caught with a light trap were used to regularly replenish a pest culture. Generally, hale, healthy and uniform sized fourth instar larvae of (F1) selected species were used in the experiments.
Insect toxicity
The toxicity of Tm-AgNPs and Tm-LMF (Leaf Methanol Fractions) to larvae and pupae was assessed using this method [Citation18]. In a 100 × 15 mm petri dish, five batches of 25 well-active, hale, healthy and fourth-instar larvae of H. armigera and S. litura () were introduced independently at varying times, ranging from 0 to 6 h. Tm-AgNPs (1.0–2.5 μg/mL) and Tm-LMF (50–400 μg/mL) were sprayed at varying doses on a variety of host plants, such as castor leaves for S. litura and bendi fruits for H. armigera, to determine their larval toxicity ().
Where
% LMT = % larval mortality in the treatment
% LMC = % larval mortality in the control
The same protocol was used to assess the toxicity of the pupae; healthy, evenly proportioned and hale larvae in their fifth instar of the chosen polyphagous pests were given varying dosages (10–40 μg/mL for Tm-LMF and 1.0–2.5 μg/mL for Tm-AgNPs) sprayed on their respective host leaves. To assess the toxicity of the pupae, control and Tm-LMF and Tm-AgNPs treated larvae were allowed to pupate individually. For S. litura, this resulted in 15 pupae and for H. armigera, in 12 pupae (). Both narrow and broad range tests were used to evaluate the toxicity to larvae and pupae.
Enzyme bioassays
Bioassay was performed with instars of test organisms using fractions. Two-day-old fourth instars larvae of treated S. litura and H. armigera were used to quantify the enzyme activities, such as estimation of acid phosphatase (ACP) and alkaline phosphatases (ALP) [Citation19], estimation of adenosine triphosphatase (ATPase) [Citation20] and estimation of lactate dehydrogenase (LDH). Fresh castor and cotton leaves were sprayed with fractions on both surfaces and let to air dry, whereas control leaves were treated with isopropanol and left to air dry. Using a spray sprayer with a regulator, the fractions were sprayed on the leaves. Larvae in their fifth instar were fed separately with their corresponding host plant after being starved for 4 h. Every 24 h, the uneaten leaves were taken out and replaced with newly treated ones. Each treatment employed a minimum of 20 larvae/concentration, which were reproduced five times (n = 100).
Statistical analysis
The SPSS 25.0 version software was used to determine the chi-square values and lethal concentration. ANOVA of arcsine transformed percentages was used to evaluate the enzyme activity data, and Tukey’s multiple range test was then applied [Citation21] (Snedecor and Cochran, 1989).
Results and discussion
Phytochemical screening
The maximum number of phytochemicals (PCs), including saponin, terpenoids, tannins, steroids, phlobatannin, flavanoids, reducing sugar, glycosides and alkaloids, that were extracted from higher polarity solvents like Tm-LME and evaluated by PCs of Tm-LE (hexane, diethyl ether, dichloromethane, ethyl acetate and methanol) are listed in . Similarly, diethyl ether extract was found to contain glycosides, flavonoids, steroids, reducing sugar, terpenoids and alkaloids. Dichloromethane extract was also found to include alkaloids, reducing sugar, glycosides, terpenoids and flavonoids. Reducing sugar, flavonoids, terpenoids, tannins and alkaloids were observed in ethyl acetate extract. From the methanol extract flavonoids, terpenoids, saponin, steroids, reducing sugar and tannin were found. The PCs are highly effective bioresource phyto-pesticidal compounds that can be isolated from different portions of medicinal plants and species [Citation22–24].
Table 1. Phytochemical screening of hexane, diethyl ether, dichloromethane, ethyl acetate and methanol extracts T. malabarica.
TLC and CC analysis
The effective Tm-LME was run on pre-coated TLC with a solvent mixture including ethyl acetate:methanol in a 1:9 ratio in order to conduct additional analysis aimed at determining the number of chemicals responsible for their effectiveness. In the same solvent solution, the maximum of five fractions-five-was then attained (). Tm-extract’s active phytochemical group was further separated using CC packed with silica gel, and it was subsequently eluted with 50 ml of several solvent system, including a 9:1 mixture of methanol and ethyl acetate. In all, five fractions were obtained, gathered and examined in relation to specific agricultural field pests (). Likewise, the experiment using a TLC plate to detect five fractions in a solvent system ratio of 9:1 (ethanol:diethyl ether) was conducted. Additionally, CC was tightly packed with silica gel and the 50 mL solvent ratio of 9:1 (ethanol:diethyl ether) was obtained from Acacia catechu-LEE [Citation4].
Tm-AgNPs synthesis
Studies in the literature indicate that the colour of the silver nanoparticle solution is either dark brown or dark reddish. Before AgNO3 was added, T. malabarica had a translucent yellow colour; however, AgNO3 treatment caused the colour to shift to dark brown, indicating the synthesis of AgNPs. The colour shift is caused by the quantum confinement feature, a size dependent characteristic of nanoparticles that influences their optical characteristics. Numerous reports deeply relate to the work we are doing now [Citation17,Citation25–27].
UV–Vis spectra analysis
UV-visible spectrum regular sampling of the reaction mixture, scanning of the absorption maxima using UV–vis spectra and measurement of the solution’s UV–vis spectra were used to confirm the bio-reduction of silver nanoparticles. The extract colour began to change when it was combined with a silver nitrate solution. The solution’s colour changed from brown upon mixing the Tm-LE with the silver nitrate aqueous solution, signifying the formation of AgNPs. Observation of the T. malabarica extract during its incubation with AgNO3 solution revealed a change in colour. The absence of AgNO3 in T. malabarica extract resulted in no colour shift. During the first hour of incubation, the extract’s colour turned light brown, and after 6 h, it turned dark brown. Six hours passed with no notable change. The extract of T. malabarica showed a peak at 245 nm in its absorption spectrum at various wavelengths between 200 and 700 nm (). Similarly, it has been demonstrated that Argemone mexicana-LAE green produced AgNPs can be experimentally observed by UV–vis spectrum analysis by changing from yellow to brown in hue [Citation28]. Numerous studies have been conducted recently that bolster our current researches [Citation29–31].
FTIR spectra analysis
FTIR spectroscopy was used to determine which biomolecules were responsible for capping the bio-reduced AgNPs that were synthesised with a particular plant extract. The silver nanoparticle solution was spun for 20 min at 15,000 rpm in order to prepare it for FTIR analysis. Two millilitres of distilled water were used five times to clean the pellet in order to get rid of any proteins or free enzymes that were not covering the silver nanoparticles. Major peaks at 3882.71, 3865.35, 3828.70, 3792.05, 3782.41, 3724.54, 3423.65, 2924.09, 2858.51, 2357.01, 2260.57, 2083.12, 1639.49, 1415.75, 1247.94, 1111.00, 1068.56, 771.53, 663.51 and 617.22 cm−1 were found when the purified nanoparticles were exposed to FTIR analysis (). Strong and wide peak values were associated with functional groups such as alcohols, phenols (O-H stretch, H-bonded), carboxylic acids, alkanes (O-H stretch and C-H stretch (medium) 2924.09 cm−1, 2858.51 cm−1), nitriles (C≡N stretch (very strong) 2260.57 cm−1), 1* amines (N-H bend (medium) 1639.49 cm−1), aromatics (C-C stretch (in-ring) (medium) 1415.75 cm−1), aliphatic amines (C-N stretch (medium) 1247.94 cm−1), alcohols, carboxylic acids, esters, ethers (C-O stretch (strong) 1111.00 cm−1, 1068.56 cm−1), 1*, 2* amines (N-H wag (strong and broad) 771.53 cm−1), alkynes (-C≡C-H: C-H bend (broad and strong) 663.51 cm−1, 617.22 cm−1) group present in Tm-LME involved in Ag+ ions reduction to Ag0 NPs (). Numerous studies with comparable findings have been noted in previously published experiments [Citation7,Citation32,Citation33]
Table 2. FTIR spectra analysis of AgNPs obtained from Tm-LME.
SEM and EDX analysis
The scanning electron microscope utilises a high-energy electron beam to produce a range of signals on the surface of the used specimens. The signals display details about the material, such as its orientation, external morphology (texture) and crystalline structure, as well as its chemical composition. Since the X-rays produced by SEM analysis do not cause the sample to lose volume, it is typically regarded as non-destructive and can be used to repeatedly analyse the same materials. The SEM image results for synthesised AgNPs, which are measured, ranged from 48.4 nm to 22.7 nm and are magnified at 4.65 Kx times, are displayed in . The structures of triangles, pentagons and hexagons are evident. The synthetic AgNPs chemical purity is confirmed by energy-dispersive X-ray spectroscopy (EDX), which also clearly shows their hexagonal, pentagonal and triangular structures. Information on the chemical analysis of the fields under investigation or the composition at particular locations can be obtained with the help of the EDX attachment that comes with the SEM. For silver, there were noticeable signals and high atomic percent values. The synthetic AgNPs chemical purity is validated by EDX (). Similar results were found: FE-SEM and HR-TEM analyses revealed a spherical form with a size range of 20 to 53 nm, while EXD indicates the chemical composition of synthesised AgNP. Particle size study revealed the size and distribution of Annona muricata-AgNPs (103 nm) [Citation34]. The same type of propensity was recorded in many earlier results [Citation34–36].
Insect toxicity
Agricultural pests S. litura and H. armigera showed larval and pupal toxicity values of Tm-LME and Tm-AgNPs, as expressed in . The highest mortality, fraction 5, showed the LC50 values of 15.18 ppm (S. litura) and 17.44 ppm (H. armigera), respectively, and LC90 values of 43.92 ppm and 46.67 ppm, followed by fraction 4 (LC50 values 18.37 ppm/21.78 ppm), fraction 3 (LC50 values 20.34 ppm/24.32 ppm), fraction 2 (LC50 values 22.67 ppm/26.89 ppm) and fraction 1 (LC50 values 23.12 ppm/28.50 ppm), respectively (). The data unmistakably showed that the S. litura pupae treated with fraction 5 had the highest mortality, with LC50 values of 25.08 ppm. The H. armigera pupae treated with fraction 1 had the lowest mortality, with LC50 values of 36.46 ppm (). The highest larval mortality of S. litura was found to be 98.99%, 82.44%, 56.99%, 38.44%, followed by H. armigera, which were found to be 90.44%, 69.66%, 48.46%, 30.33% treated Tm-AgNPs with different concentrations (2.5, 2.0, 1.5 and 1.0 ppm) (). Pupicidal toxicity of Tm-AgNPs showed the S. litura (55.33%, 52.8%, 32.13% and 19.86%) and H. armigera (49.6%, 41.63%, 26.10% and 15.66%) (). The present investigation revealed that the results are attributed to the findings that entomopathogenic nematodes are effective at controlling S. litura, which may partially substitute the use of chemical insecticides, thus reduce the overuse of chemical insecticides [Citation37,Citation38]. Ocimum basilicum-AgNPs were found to be eco-friendly but the most compared to the selected synthetic chemicals which may be hazardous for the environment as well as human health [Citation39]. Additionally, the metabolite demonstrated strong larvicidal action against S. litura (58.22%) and H. armigera (63.11%); the LC50 values were 645.25 ppm and 806.54 ppm, respectively [Citation40]. The detrimental effects of Pseudomonas sp. EN4 on S. litura biological characteristics suggest that this soil bacterial strain could be exploited as an efficient bio-control agent against insect pests [Citation41]. From Leonotis nepetifolia-AgNPs, the highest larval mortality rates of 78.49% for S. litura and 72.70% for H. armigera, as well as the highest pupal mortality rates of 84.66% for S. litura and 77.44% for H. armigera, were noted [Citation42]. The maximum mortality of S. litura (92.83%) and H. armigera (91.41%) occurred at 72 h treatment with Trigonella foenum-graecum leaf extract and fenvalerate [Citation43]. The MPCs were methyl chavicol for Ocimumselloi, α-bisabolol for E. erythropappus, bicyclogermacrene for Hyptissuaveolens and β-thujone for Hyptismarrubioides [Citation44]. The maximum LC50 values were 10.20 ppm (H. armigera) and 12.49 ppm (S. litura) from Neem gum nano formulation at 100 ppm [Citation45].
Table 3. Larval toxicity induced by Tm-LMF on the larvae of selected polyphagous insect pests.
Table 4. Pupicidal toxicity induced by Tm-LMF on the larvae of selected polyphagous insect pests.
Table 5. Larval toxicity induced by Tm-AgNPs on the larvae of selected polyphagous insect pests.
Table 6. Pupicidal toxicity induced by Tm-AgNPs on the larvae of selected polyphagous insect pests.
Table 7. Enzyme activities (in µmol/mg/h−1) of fourth instar larvae of S. litura, H. armigera and A. janata fed on their respective host plant treated with different fractions.
Enzyme bioassays
The enzyme bioassay of different fractions from Tm-LME was tested fourth instar larvae of S. litura and H. armigera. Acid phosphatase activity (ACP) in the fourth instar larvae of S. litura was greatly reduced by the action of fraction 5 (6.34 ± 1.26 µmol/mg/h−1), followed by alkaline phosphatase (ALP) (8.82 ± 1.26 µmol/mg/h−1), adenosine triphosphatase (ATPase) (46.57 ± 3.21 µmol/mg/h−1) and lactate dehydrogenase (LDH) (15.79 ± 1.34 µmol/mg/h−1). ACP activity of fraction 4 (8.43 ± 1.81 µmol/mg/h−1), followed by ALP (10.11 ± 1.81 µmol/mg/h−1), ATPase (53.58 ± 3.79 µmol/mg/h−1) and LDH (17.63 ± 2.42 µmol/mg/h−1) tested larvae of S. litura. It was imperative to note that fraction 1, 2 and 3 had no effective influence over the ACP, ALP, ATPase and LDH activities in S. litura and H. armigera with the lowest ACP (6.21 ± 2.60 µmol/mg/h−1), ALP (8.23 ± 2.81 µmol/mg/h−1), ATPase (37.23 ± 3.57 µmol/mg/h−1) and LDH (12.40 ± 1.78 µmol/mg/h−1) from fraction 5, while other fractions showed statistically insignificant activities (). The inhibitor was stable and actively inhibited the proteolysis of both HGP and SGP enzymes for up to 72 h. Incorporation of Murraya koenigii miraculin-like protein into artificial diet adversely affected the growth and development of pests in a dose-dependent manner [Citation46]. Archidendronellipticum-TI, the protein, inhibited trypsin in a 1:1 stoichiometric ratio but lacked similar stoichiometry against chymotrypsin. Additionally, S. litura larval gut physiology indicated a sevenfold decrease in trypsin-like protease activity and a fivefold increase in chymotrypsin-like protease activity after being fed a diet supplemented with 150 microM [Citation47,Citation48]. It is imminent to notice, that the observed increased detoxification enzyme activity (α esterase, β esterase and glutathione-S-transferase) of S. litura exposed to different concentrations of biosynthesised TiO2NPs [Citation49].
Conclusion
The findings of the study showed that Tm-LME green-synthesised Tm-AgNPs offer promise as potential bio-control agents against insect pest, S. litura and H. armigera, due to their markedly insect toxicity and enzyme activity. Based on the current investigation, phytocompound from T. malabarica can be used as a pesticidal product to combat and protect against pests.
Acknowledgments
The authors are thankful to the Researchers Supporting Project number (RSPD2024R728), King Saud University, Riyadh, Saudi Arabia.
Disclosure statement
No potential conflict of interest was reported by the author(s).
Data availability statement
The authors confirm that the data supporting the findings of this study are available within the article.
References
- Elanchezhiyan K, Gokulakrishnan J, Deepa J, et al. Botanical extracts of Tinosporacrispa (Menispermaceae) and Psidium guajava (Myrtaceae) against important agricultural polyphagous field pest armyworm, Spodoptera litura (Fab.) (Lepidoptera: Noctuidae). Int J Rec Sci Res. 2015;6(2):2703–12.
- Mahesh Babu S, Baranitharan M, Dhanasekaran S, et al. Chemical compositions, antifeedant and larvicidal activity of Pongamia pinnata (L.) against polyphagous field pest, Spodoptera litura. Int J Zool Invest. 2016;2(1):48–57.
- Backiyaraj M, Elumalai A, Kasinathan D, et al. Bioefficacy of Caesalpinia bonducella extracts against Tobacco cutworm, Helicoverpa armigera (Hub.) (Lepidoptera: Noctuidae). J Coast Life Med. 2014;2:685–693.
- Baranitharan M, Alarifi S, Alkahtani S, et al. Phytochemical analysis and fabrication of silver nanoparticles using Acacia catechu: an efficacious ecofriendly control tool against selected polyphagous insect pests. Saudi J Biol Sci. 2021;28(1):148–156. doi: 10.1016/j.sjbs.2020.09.024
- Baranitharan M, Dhanasekaran S, Murugan K, et al. Chemical composition and laboratory investigation of Melissa officinalis essential oil against human malarial vector mosquito, Anopheles stephensi, L. (Diptera: Culicidae). J Coast Life Med. 2016;114:1817–1827. doi: 10.12980/jclm.4.2016J6-174
- Benelli G. Plant-mediated synthesis of nanoparticles: a newer and safer tool against mosquito-borne diseases? Asian Pac J Trop Biomed. 2016;6(4):353–354. doi: 10.1016/j.apjtb.2015.10.015
- Mathiyazhagan M, Baranitharan M, Rajaganesh K, et al. Acute toxicity of Cistanche tubulosa methanol extract: a potential novel insecticidal compounds against malaria-transmitting mosquito. Biochem Cell Archiv. 2023;23(2). doi: 10.51470/bca.2023.23.2.1163
- Thushimenan BJ, Baranitharan M. Laboratory investigation of Terminalia arjuna and Trachyspermum roxburghianum against groundnut pest, Helicoverpa armigera. Asia J Pharmaceut Clinic Res. 2016;9(1):232–236.
- Thushimenan BJ. Baranitharan M. Antifeedant and toxicity of indigenous medicinal plants extracts against Spodoptera litura (Fab) (Lepidoptera: Noctuidae). Int J Zool Appl Bioscien. 2016;1(1):106–110.
- Benelli G, Kadaikunnan S, Alharbi NS, et al. Biophysical characterization of Acacia caesia-fabricated silver nanoparticles: effectiveness on mosquito vectors of public health relevance and impact on non-target aquatic biocontrol agents. Environ Sci Pollut Res. 2018;25(11):10228–10242. doi: 10.1007/s11356-017-8482-y
- El-Wakeil NE. Botanical pesticides and their mode of action. Gesunde Pflanzen. 2013;65(4):125–149. doi: 10.1007/s10343-013-0308-3
- Dhanasekaran S, Baranitharan M, Muthulingam M, et al. Studies on the impact of medicinal plants in relation to malaria vector control against Anopheles stephensi. Innoriginal Int J Scien. 2018;5(3):12–14. Available from: https://www.innoriginal.com/index.php/iijs/article/view/131
- Ahana AK, Prakash LH. Conservation of ret medicinal plants W.S.R to Tinospora Malabarica (Lam.) Hook.F. & Thoms. J Pharma Cog Phyto Chem. 2018;P3:409–412.
- Minjas JN, Sarda RK. Laboratory observations on the toxicity of Swartzia madagascariensis (Leguminosae) extract to mosquito larvae. Trans R Soc Trop Med. 1986;80(3):460–461. doi: 10.1016/0035-9203(86)90345-7
- Senthilkumar PK, Reetha D. Screening of antimicrobial properties of certain Indian medicinal plants. J Phytol. 2009;1:193–198.
- Baranitharan M, Dhanasekaran S, Murugan K, et al. Coleus aromaticus leaf extract fractions: A source of novel ovicides, larvicides and repellents against Anopheles, Aedes and Culex mosquito vectors? Process Saf Environ Prot. 2017;106:23–33. doi: 10.1016/j.psep.2016.12.003
- Subash B, Vijayan P, Baranitharan M. Biosynthesis of silver nanoparticles using Hygrophila auriculata: A novel route of malarial fever vector mosquito control. Int J Scient Technol Res. 2019;8(12):4010–4018. ISSN: 2277-8616.
- Abbott WS. A method of computing the effectiveness of an insecticide. J Econ Entomol. 1925;18(2):265–267. doi: 10.1093/jee/18.2.265a
- Bessey OA, Lowry OH, Brock MJ. A method for the rapid determination of alkaline phosphatase with five cubic millimeters of serum. J Biol Chem. 1946;164(1):321–329. doi: 10.1016/S0021-9258(18)43072-4
- Shiosaka T, Okuda H, Fujii S. Mechanism of the phosphorylation of thymidine by the culture filtrate of Clostridium perfringens and rat liver extract. Biochimica Biophys Acta. 1971;246(2):171–183. doi: 10.1016/0005-2787(71)90125-0
- Snedecor GW, Cochran WG. Statistical methods. Eighth ed. Ames (IA): Iowa State University Press; 1989.
- Ahmed M, Peiwen Q, Gu Z, et al. Insecticidal activity and biochemical composition of Citrullus colocynthis, Cannabis indica and Artemisia argyi extracts against cabbage aphid (Brevicoryne brassicae L.). Sci Rep. 2020;10(1). doi: 10.1038/s41598-019-57092-5
- Mungenge C, Zimudzi C, Zimba M, et al. Phytochemical screening, cytotoxicity and insecticidal activity of the fish poison plant Synaptolepis alternifoliaoliv (Thymelaeaceae). J Pharm Phytochem. 2014;2:15–19.
- Riaz B, Zahoor MK, Zahoor MA, et al. Toxicity, phytochemical composition, and enzyme inhibitory activities of some indigenous weed plant extracts in fruit fly, Drosophila melanogaster. Evid Based Complement Alternat Med. 2018;2018:1–11. doi: 10.1155/2018/2325659
- Morejón B, Pilaquinga F, Domenech F, et al. Larvicidal activity of silver nanoparticles synthesized using extracts of Ambrosia arborescens (Asteraceae) to control Aedes aegypti L. (Diptera: Culicidae). J Nanotechnol. 2018;2018:1–8. doi: 10.1155/2018/6917938
- Sutthanont N, Attrapadung S, Nuchprayoon S. Larvicidal activity of synthesized silver nanoparticles from Curcuma zedoaria essential oil against Culex quinquefasciatus. Insect. 2019;10(1):1–11. doi: 10.3390/insects10010027
- Mohammed SSS, Lawrance AV, Sampath S, et al. Facile green synthesis of silver nanoparticles from sprouted Zingiberaceae species: spectral characterisation and its potential biological applications. Mater Technol. 2020;37(8):533–546. doi: 10.1080/10667857.2020.1863571
- Kavitha G, Gokulakrishnan J, Baranitharan M. Biosynthesis of silver nanoparticles using Argemone mexicana: a novel route of malarial fever vector mosquito control. Int J Advanc Res Biol Scien. 2019;6(8):148–158. doi: 10.22192/ijarbs
- Elamawi RM, L-Harbi RE, Hendi AA. Biosynthesis and characterization of silver nanoparticles using Trichoderma longibrachiatum and their effect on phytopathogenic fungi. Egypt J Biol Pest Control. 2018;28(1):1–11. doi: 10.1186/s41938-018-0028-1
- Pilaquinga F, Morejón B, Ganchala D, et al. Green synthesis of silver nanoparticles using Solanum mammosum L. (Solanaceae) fruit extract and their larvicidal activity against Aedes aegypti L. (Diptera: Culicidae). PLoS One. 2019;14(10):e0224109. doi: 10.1371/journal.pone.0224109
- Harikrishnan A, Ramalingam B, Nadeem A, et al. Eco-friendly synthesis of zinc oxide nanoparticles (ZnOnps) from piper betel leaf extract: spectral characterization and its application on plant growth parameters in maize, fenugreek and red gram. Mat Technol. 2024;39(1):2298547. doi: 10.1080/10667857.2023.2298547
- Ajitha B, Reddy YAK, Reddy PS. Biogenic nano-scale silver particles by Tephrosia purpurea leaf extract and their inborn antimicrobial activity. Spectro Acta Part A. 2014;121:164–172. doi: 10.1016/j.saa.2013.10.077
- Suresh S, Karthikeyan S, Jayamoorthy K. FTIR and multivariate analysis to study the effect of bulk and nano copper oxide on peanut plant leaves. J Sci Adv Mater Dev. 2016;1(3):343–350. doi: 10.1016/j.jsamd.2016.08.004
- Santhosh SB, Yuvarajan R, Natarajan D. Annona muricata leaf extract-mediated silver nanoparticles synthesis and its larvicidal potential against dengue, malaria and filariasis vector. Parasitol Res. 2015;114(8):3087–3096. doi: 10.1007/s00436-015-4511-2
- Elumalai K, Velmurugan S. Green synthesis, characterization and antimicrobial activities of zinc oxide nanoparticles from the leaf extract of Azadirachta indica (L.). Appl Surface Sci. 2015;345:329–336. doi: 10.1016/j.apsusc.2015.03.176
- Erdogan O, Abbak M, Demirbolat GM, et al. Green synthesis of silver nanoparticles via Cynara scolymus leaf extracts: The characterization, anticancer potential with photodynamic therapy in MCF7 cells. PLoS One. 2019;14(6):1–15. doi: 10.1371/journal.pone.0216496
- Rautela A, Rani J, Debnath M. Green synthesis of silver nanoparticles from Tectona grandis seeds extract: characterization and mechanism of antimicrobial action on different microorganisms. J Anal Sci Technol. 2019;10(1):1–10. doi: 10.1186/s40543-018-0163-z
- Xun Y, Muhammad Shahid A, Yinying L, et al. Efficacy of entomopathogenic nematodes against the Tobacco cutworm, Spodoptera litura (Lepidoptera: Noctuidae). J Econ Entomol. 2020;113(1):64–72. doi: 10.1093/jee/toz262
- Muhammad J, Nazeer Ahmad J, Jalal Arif M, et al. Characterization of Ocimum basilicum synthesized silver nanoparticles and its relative toxicity to some insecticides against tobacco cutworm, Spodoptera litura Feb. (Lepidoptera; Noctuidae). Ecotoxicol Environ Saf. 2021;218:112278. doi: 10.1016/j.ecoenv.2021.112278
- Valan Arasu M, Abdullah Al-Dhabi N, Saritha V, et al. Antifeedant, larvicidal and growth inhibitory bioactivities of novel polyketide metabolite isolated from Streptomyces sp. AP-123 against Helicoverpa armigera and Spodoptera litura. BMC Microbiol. 2013. doi: 10.1186/1471-2180-13-105
- Sunaina S, Devi M, Sharma G, et al. Larvicidal, growth inhibitory and biochemical effects of soil bacterium, Pseudomonas sp. EN4 against Spodoptera litura (Fab.) (Lepidoptera: Noctuidae). BMC Microbiol. 2023;23(1):95. doi: 10.1186/s12866-023-02841-w
- Manimegalai T, Raguvaran K, Kalpana M, et al. Green synthesis of silver nanoparticle using Leonotis nepetifolia and their toxicity against vector mosquitoes of Aedes aegypti and Culex quinquefasciatus and agricultural pests of Spodoptera litura and Helicoverpa armigera. Environ Sci Pollut Res Int. 2020;27(34):43103–43116. doi: 10.1007/s11356-020-10127-1
- Ranganathan M, Ramkumar G, Kumarasamy S, et al. Synergism and toxicity of iron nanoparticles derived from Trigonella foenum-graecum against pyrethroid treatment in S. litura and H. armigera (Lepidoptera: Noctuidae). Environ Res. 2023;231:116079. doi: 10.1016/j.envres.2023.116079
- Claubert Wagner Guimarães DM, Geraldo Andrade C, Dejane Santos A, et al. Biocontrol potential of methyl chavicol for managing Spodoptera frugiperda (Lepidoptera: Noctuidae), an important corn pest. Environ Sci Pollut Res Int. 2020;27(5):5030–5041. doi: 10.1007/s11356-019-07079-6
- Kamaraj C, Rajiv Gandhi P, Elango G, et al. Novel and environmental friendly approach; Impact of neem (Azadirachta indica) gum nano formulation (NGNF) on Helicoverpa armigera (Hub.) and Spodoptera litura (Fab.). Int J Biol Macromol. 2018;107:59–69. doi: 10.1016/j.ijbiomac.2017.08.145
- Gahloth D, Shukla U, Birah A, et al. Bioinsecticidal activity of Murraya koenigii miraculin-like protein against Helicoverpa armigera and Spodoptera litura. Arch Insect Biochem Physiol. 2011;78(3):132–144. doi: 10.1002/arch.20448
- Bhattacharyya A, Mazumdar S, Mazumdar Leighton S, et al. A Kunitz proteinase inhibitor from Archidendron ellipticum seeds: purification, characterization, and kinetic properties. Phytochem. 2006;67(3):232–241. doi: 10.1016/j.phytochem.2005.11.010
- Bhattacharyya A, Mazumdar Leighton S, Babu CR. Bioinsecticidal activity of Archidendron ellipticum trypsin inhibitor on growth and serine digestive enzymes during larval development of Spodoptera litura. Comp Biochem Physiol C Toxicol Pharmacol. 2007;145(4):669–677. doi: 10.1016/j.cbpc.2007.03.003
- Narayanan SS, Sengodan K, Sengottayan SN, et al. Eco-friendly biosynthesis of TiO2 nanoparticles using Desmostachya bipinnata extract: Larvicidal and pupicidal potential against Aedes aegypti and Spodoptera litura and acute toxicity in non-target organisms. Sci Total Environ. 2023;858:159512. doi: 10.1016/j.scitotenv.2022.159512