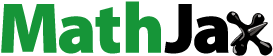
Abstract
The oral delivery of amphotericin B (AmB) has remained a challenge due to its low solubility, permeability, and instability in gastric acidic pH. To solve these issues, herein, we reported a novel approach of using nanostructured lipid carriers (NLCs) and NLCs coating with Eudragit®L100-55 (Eu-NLCs) for the oral delivery of AmB. This study aimed to compare their ability in protecting the drug from degradation in gastrointestinal fluids and permeation enhancement in Caco-2 cells. Uncoated NLCs and Eu-NLCs possessed a mean particle size of ∼180 and ∼550 nm, with a zeta potential of ∼−30 and ∼−50 mV, respectively. Both NLCs demonstrated an AmB entrapment efficiency up to ∼75%. They possessed significantly greater AmB water solubility than the free drug by up to 10-fold. In fasted state simulated gastric fluid, Eu-NLCs provided significantly greater AmB protection from acidic degradation than uncoated NLCs. In fasted state simulated intestinal fluid, both uncoated and Eu-NLCs showed a fast release characteristic. Caco-2 cells permeation studies revealed that uncoated NLCs provided significantly higher apparent permeation coefficient (Papp) value than Eu-NLCs. Moreover, after 6 months of storage at 4 °C in the absence of light, the physicochemical stabilities of the lyophilized uncoated and Eu-NLCs could be maintained. In conclusion, the developed NLCs and Eu-NLCs could be a potential drug delivery system in improving the oral bioavailability of AmB.
1. Introduction
There is an alarming increase in the incidence of systemic fungal infections that are rapidly rising with the prevalent proliferation of HIV-AIDS, which is also causing critical concern among other immune-compromised patients with cancer, organ transplant recipients, and diabetics (Hamill, Citation2013). In these patients, invasive fungal infections may account for as many as 30% of deaths (Wasan et al., Citation2009). Amphotericin B (AmB), a polyene antifungal antibiotic, possesses a broad-spectrum activity with relatively less resistance against most of the fungal infections. Thus, it is considered as one of the gold standards for the treatment of systemic fungal infections.
AmB, a molecular weight of 924 Da, is classified as a Biopharmaceutical Classification System as a class IV with limited solubility and permeability properties. Thus, it is considered poorly absorbed from the gastrointestinal (GI) tract leading to low oral bioavailability of 0.3% (Ouellette et al., Citation2004). Moreover, AmB is notorious for its unstable in an acidic environment. As a consequence, no oral AmB product is available in market, only intravenous (IV) administration has been available. However, IV route is not desirable because of the inconvenience, complexities, and need for hospitalization. On the other hand, oral administration is the most accepted and would reduce the requirement for hospitalization during treatment and cost-effectiveness. This route is simplicity, convenience, lower cost, and painless that offer greater patient compliance. Therefore, it remains a challenge task to develop an orally delivery carrier for AmB.
Recently, nanostructured lipid carriers (NLCs) attract more attention due to their safety and ability to enhance oral bioavailability of poorly water-soluble drugs via promoting intestinal absorption (Müller et al., Citation2002; Wissing et al., Citation2004; Pardeike et al., Citation2009). Compared to other forms of nanoparticles such as polymeric or inorganic ones, NLCs prove their superior in terms of human biocompatibility and biodegradability as they compose of physiological lipids and can be prepared without the use of organic solvent. In addition, due to their nano-sized, NLCs offer many advantages, including promote residence time at the target site and increase drug water solubility. As a solid state, NLCs could increase drug chemical stability. Thus, NLCs are the formulation-of-choice to improve AmB solubility, stability, and cellular absorption.
In a previous study, AmB-loaded NLCs were successfully developed with potentially used for parenteral administration. They showed the ability to reduce AmB adverse side effects, especially nephrotoxicity and hematotoxicity, while maintained antifungal activity against Candida albicans with a minimum inhibitory concentration of 0.25 μgmL−1 (Pataranapa et al., Citation2019). However, AmB is soluble in a medium of pH < 2, but also unstable under such extreme conditions (Lemke et al., Citation2005). As a consequence, the released AmB in gastric fluid will lead to AmB degradation. Therefore, any protection afforded by the lipid components of the formulation could be a significant benefit toward increasing the oral bioavailability of AmB. In addition, we proposed enteric polymer-coated NLCs as an orally delivery system for AmB that could bypass the stomach and release the loaded drug into the intestine. Eudragits are pharmaceutical excipients which have been widely used for protecting the active ingredient from the gastric fluid and to improve drug effectiveness (Wang & Zhang, Citation2012). Eudragits are non-biodegradable, non-absorbable, and nontoxic. Among the Eudragit family, an enteric pH-dependent Eudragit L100-55® (Methacrylic acid-ethyl acrylate copolymer type A, 1:1) has been commonly used for the preparation of enteric-coated tablet as it is soluble at pH > 5.5 (Khan et al., Citation1999; Moustafine et al., Citation2008). Thus, in this study, we further explore its possibility for delivery AmB orally by comparing between uncoated NLCs and NLCs coating with Eudragit®L100-55 (Eu-NLCs). Their ability to protect the drug from degradation in GI fluids and drug permeation across caco-2 cells was compared. The physicochemical properties including size, size distribution, zeta potential, entrapment efficiency, and long-term storage stability were investigated. In addition, the AmB solubility and patterns of drug release in GI fluids were also investigated in bio-relevant media simulating the fasting stomach and intestine fluid. Finally, in vitro permeation study in Caco-2 cell monolayer was also examined.
2. Materials and methods
2.1. Materials
AmB (USP grade) was purchased from Biobasic Inc. (Markham ON, Canada). Standard AmB (80% Pure HPLC grade) and 4-nitroaniline (Purity >99%) were purchased from Sigma-Aldrich (St. Louis, MO, USA). Dimethyl sulfoxide, methanol, and acetonitrile (HPLC grade) were purchased from LabScan (Bangkok, Thailand). Glyceryl monosterate (GMS) was purchased from Union Science (Chiangmai, Thailand). Stearic acid, castor oil, lexol, polyethylene glycol 400 (PEG400), Cremophor® RH40, and hydrogenated castor oil were purchased from Naming trading Co., Ltd. (Bangkok, Thailand). Emulmetik 900 was purchased from Cargill Texturizing Solution (Hamburg, Germany). Tween 80 was purchased from Ajax Finechem (NSW, Australia). Labrasol® was purchased from GATTEFOSSE (Saint-Priest, France). Mannitol, sodium dihydrogen phosphate dihydrate, sodium acetate, and sodium chloride were purchased from Ajax Finechem (NSW, Australia). Eudragit®L100-55 was kindly gifted by Evonik Industries (North Rhine-Westphalia, Germany). Simulated intestinal fluid (SIF) powder was purchased from Biorelevant (London, UK). All other chemicals and reagents used were of analytical grade. Regenerated cellulose membrane, diameter 76 mm, with a molecular weight cut off (MWCO) 100 kDa (Milipore® YM100) was purchased from Millipore Corporation (Bedford, MA, USA). Polycarbonate (PC) membrane 0.1 µm, diameter 13 mm was purchased from Merck KGaA (Darmstadt, Germany).
Caco-2 cells (HTB-37™, Passage no. 18) were purchased from the American Type Culture Collection (Manassas, VA, USA). Dulbecco’s modified Eagle’s medium (DMEM F-12) and all the materials for cell culture were purchased from Sigma chemical (St. Louis, MO, USA). Cell culture plate and Transwell plate (12 mm diameter, 0.4 mm-pore-size polycarbonate membrane) were purchased from Corning Costar (Oneonta, NY, USA). 3-(4,5-Dimethylthiazol-2-yl)-2,5-Diphenyltetrazolium Bromide (MTT) was purchased was obtained from Amresco (Solon, OH, USA).
2.2. Preparation of AmB-NLCs
Briefly, the water phase consisted of 40 mg of AmB dissolved in 1 mL of DMSO, 2% (w/w) of Tween 80, 2.5% (w/w) of Cremophor® RH40, 1% (w/w) of PEG400, 2.5% (w/w) of Labrasol® and 82% (w/w) of deionized water. The water phase was heated to ∼75 °C before adding to the oil phase. The oil phase, consisting of 1% (w/w) of stearic acid, 1% (w/w) of GMS, 5% (w/w) of lexol, 1% (w/w) of Emulmetik 900 and 1% (w/w) of castor oil was heated to ∼75 °C. Then, the obtained warm microemulsion was prepared under high pressure homogenization at 1000 bars for five cycles (M-110P, Microfluidics, Newton, MA, USA). Uncoated NLCs dispersion was washed three times with deionized water using an ultrafiltration cell system fitted with a membrane molecular weight cutoff of 100 kDa.
Apart from uncoated NLCs, NLCs were coated with varying concentrations of Eu (v/v) at 1, 2.5, 5, and 7.5%. The polymer solution was dissolved in a mixture of water and 1 N NaOH at a volume ratio of 6:1. The Eu-NLCs were prepared by adding polymer solution to the uncoated NLCs dispersion, at a volume ratio of 1:1, with constant stirring at 24 × g for 30 min (Model C-MAG HS7, IKA, China). Finally, AmB-NLCs were lyophilized using a programmable freeze-dryer (Gamma 2-16 LSC plus, Christ, Germany). 10% (w/v) mannitol was added to the uncoated NLCs dispersion before freezing. Both uncoated and Eu-NLCs dispersion were slow freezing at temperature −20 °C and lyophilized at 0.05 mbar and −40 °C for 72 h.
2.3. Physicochemical characterization of AmB-NLCs
Mean particle size and polydispersity index (PI) were measured by dynamic light scattering (DLS) using a ZetaPALS® analyzer (Brookhaven Instruments Corporation, Holtsville, NY, USA). All samples were prepared by re-dispersing the NLCs in DI water (50 mg of lyophilized particles in 1 mL of DI water). Then, all samples were diluted with DI water in a volume ratio of 1:10. The mean particle size and PI were measured at a detection angle of 90° and run for 10 measurement cycles. All the measurements were performed in triplicate. The morphology of AmB-NLCs was characterized by a transmission electron microscope (TEM, Philips Tecnai 12, Hillsboro, OR, USA).
2.4. Determination of drug entrapment efficiency
The amount of AmB was determined by using HLPC (Shimadzu, Japan), SPD-20A UV-Visible detector at 405 nm which has been performed from our previous study (Pataranapa et al., Citation2019). Chromatographic separation was performed on a HALO C18 column (5 µm, 4.6 × 150 mm, Wilmington, DE, USA) with a flow rate of 0.8 mLmin−1. The mobile phase was a mixture of 10 mM acetate buffer (pH 5) and acetonitrile at the volume ratio of 67:33 (v/v). A linear calibration curve was plotted with concentrations ranging from 0.04 to 4.0 µgmL−1, with the addition of an internal standard (4-nitroaniline). Briefly, 10 milligrams of lyophilized particles were dispersed in 1 mL of methanol and further dissolved using ultrasonic probe at 40% amplitude, and centrifuged at 31,514 × g for 30 min. The extraction process was done two times. Then, the supernatant from each extraction was diluted with the mobile phase to establish a final concentration in the range for HPLC quantification. The percentage of drug entrapped was calculated, as shown in EquationEquation (1)(1)
(1) .
(1)
(1)
2.5. Solubility of free AmB and AmB-NLCs
To simulate conditions in GI tract, the solubility of AmB was examined in water, 0.1 N HCl pH 1.2, phosphate buffer saline (PBS) pH 6.8, fasted state simulated gastric fluid pH 1.6 (FaSSGF) and fasted state simulated intestinal fluid pH 6.5, (FaSSIF). Ten milligrams of AmB power (pure drug) and an excess amount of lyophilized NLCs was dispersed in 5 mL of these media. Then, the mixture was stirred at 24 × g and maintained at 37 ± 0.5 °C for 24 h. After that, the mixture was centrifuged at 31,514 × g for 30 min. Finally, the concentration of AmB in the supernatants was analyzed by HPLC.
2.6. In vitro dissolution study
The in vitro dissolution study was performed by USP II paddle apparatus (Model UDT-804, Logan Instrument Corp., Somerset, NJ, USA). Two dissolution media were used, FaSSGF pH 1.6, represents stomach pH conditions FaSSIF pH 6.5, represents intestinal pH conditions. Lyophilized particles containing 0.9 and 0.3 mg of AmB were introduced directly into the 300 mL of FaSSGF and FaSSIF, respectively. The experiments were carries out at 37 ± 0.5 °C in the dark with a paddle speed of 75 rpm. At each time point, 2 mL of the sample was withdrawn, and the volume was replaced with an equivalent volume of fresh dissolution medium pre-warmed to 37 ± 0.5 °C. After each withdrawal, each sample was immediately filtered with a 0.1 µm PC membrane (Whatman® Cyclopore®, Whatman International Ltd., Germany) and the released drug in the rest of the filtrate was determined by HPLC as described earlier.
2.7. Cytotoxicity study
Caco-2 cells (ATCC, Manassas, VA, USA, passage number 35-45) were grown in DMEM F-12 supplemented with 10% fetal bovine serum and 1% penicillin/streptomycin. Cell cultures were maintained at 37 °C, 5% CO2. Cells were seeded in 96-well plate at a density of 1 × 104 cells per well and allow attachment overnight. Then, the cells were treated with AmB-NLCs diluted in serum-free medium to prepare samples of 1–30 µgmL−1, followed by incubation for 6 h at 37 °C in a CO2 incubator. The untreated cell was used as a control. Then, the medium containing sample was removed and incubated with a combined solution of 3-(4, 5-Dimethylthiazol-2-yl)-2, 5-Diphenyltetrazolium Bromide (MTT) at 37 °C for 2 h. After that, MTT was removed and ethanol–DMSO (1:1) was added to dissolve the formazan crystals. The absorbance of dissolved formazan was measured at a wavelength of 570 nm by microplate reader.
2.8. In vitro permeation studies in caco-2 cell monolayer
Caco-2 cells (passage number 35-45) were seeded onto the polycarbonate 12-well Transwell® inserts (0.4 μm pore, 1.12 cm2 surface area, Corning Costar Inc., Oneonta, NY, USA) at a density of 1 × 105 cells per well and the confluent monolayers (18–21 days) were used for permeability studies. Culture medium was replaced every 2 days and the integrity of the cells was checked by measuring the trans-epithelial electrical resistance (TEER) using a volt-ohm meter equipped (Millicell® ERS-2, Millipore Corporation, Burlington, MA, USA). Only cell monolayer with TEER value of 400 ± 100 Ωcm2 was used. For permeation studies, 0.5 mL of the sample dispersed in serum-free medium (containing 10 µg drug) was added to the apical side, and 1.5 mL of serum-free medium was added to the basolateral side. The treated cells were incubated at 37 ± 0.5 °C, 5% CO2. The amount of drug in three parts: apical side, basolateral side, and cells, were determined by collecting samples at time interval of 1, 2, 4, and 6 h. Cell monolayer was washed twice with 0.5 mL of ice-cold PBS pH 7.4 to eliminate the drug on the surface of monolayer. Then, cell monolayer was scraped and collected in 1 mL of PBS before centrifuged at 9744×g for 5 min. The cells were collected, dispersed in methanol, and lysed using ultrasonic probe. After that, the samples were centrifuged at 31,514×g for 10 min. Then, the supernatant was evaporated using centrifugal vacuum concentrators (Labconco Corporation, Kansas, MO, USA) to increase drug concentration. Finally, the concentration of the drug from each compartment was determined by HPLC analysis. The accumulation of AmB in Caco-2 cells monolayer and basolateral were expressed as an accumulation amount of AmB versus time. The AmB apparent permeability coefficient (Papp) was calculated according to the following EquationEquation (2)(2)
(2) .
(2)
(2)
where dQ/dt is the slope of the cumulative drug permeated versus time curve (μgh−1). A is the diffusion area (1.12 cm2), C0 is the initial concentration of AmB in the donor compartment.
2.9. Stability study of AmB-NLCs
Uncoated NLCs and Eu-NLCs were stored in darkness at 4 °C and room temperature for 6 months. The physical stability in terms of the mean particle size and zeta potential were investigated by ZetaPALS® analyzer as described earlier. The chemical stability was determined in terms of the percentage drug remaining. Ten milligrams of lyophilized NLCs were accurately weighed and dissolved with 1 mL of methanol. The sample was then sonicated and centrifuged and drug in the supernatant determined by HPLC analysis. Each of the samples was determined in triplicate.
2.10. Statistical analysis
The results are expressed as mean ± SD. Differences between the groups were compared by one-way ANOVA followed by Tukey's post hoc test. A value of p < .05 was considered statistically significant.
3. Results and discussions
3.1. Physicochemical characterization of AmB-NLCs
In this study, AmB-loaded Eu-NLCs were further developed by coating NLCs with various concentration of Eu. TEM micrographs of both prepared uncoated and Eu-NLCs showed spherical shape with some particle agglomeration (). The particle size of Eu-NLCs (400–500 nm) was found to be larger than uncoated-NLCs (200 nm), which was in accordance with the results of DLS (). However, NLCs dispersions could result in premature drug release and susceptible to drug degradation during storage. Therefore, all NLCs were further prepared in a dry state using lyophilization technique, which offers chemical and physical stability by preventing hydrolysis and Ostwald ripening, respectively (Mehnert & Mäder, Citation2001; Shete & Patravale, Citation2013). Generally, cryoprotectant has been used to prevent particle aggregation during the freeze-drying process (Shahgaldian et al., Citation2003; Lee et al., Citation2009). Therefore, 10% mannitol was used as a cryoprotectant for uncoated NLCs based on previous studies (Pataranapa et al., Citation2019), while no cryoprotectant was used for 5 and 7.5%Eu-NLCs as Eu could act as a cryoprotectant. Before lyophilization, uncoated NLCs showed a mean size of ∼130 nm with a zeta potential of ∼−30 mV, while Eu-NLCs showed a larger mean size of ∼450 nm with a higher zeta potential of ∼−50 mV. A high negative charge of Eu-NLCs suggested that carboxyl groups of Eu presented on the particle surface. After lyophilization, all obtained lyophilized products appeared as a fluffy cake () with high re-dispersibility in water and demonstrated a narrow size distribution with a PI of ∼0.3. The mean size of uncoated and Eu-NLCs showed slightly larger than those before lyophilization, ∼170 nm, ∼500–600 nm, respectively, while the zeta potential was not changed, . This observation suggesting the lyophilization process could damage the surfactant film on the particle due to freezing effect and led to particle aggregation during the re-dispersion process. The reduction in mean size by increasing the Eu concentration could be explained by the fact that Eu, a polymer, can act as a cryoprotectant by physically protect the particle from aggregation. Therefore, the more Eu content, the more effectiveness in maintaining the particle size during freezing and lyophilization process.
Figure 1. TEM micrographs of freshly prepared AmB-NLCs (A) uncoated-NLCs; (B) 5%Eu-NLCs, and (C) 7.5%Eu-NLCs.
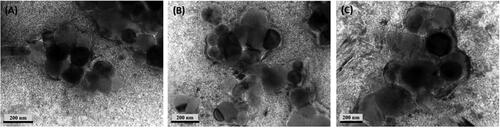
Figure 2. Photographs of uncoated NLCs and Eu-NLCs after lyophilization; (A) uncoated-NLCs; (B) 5%Eu-NLCs, and (C) 7.5%Eu-NLCs.
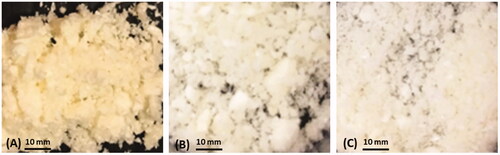
Table 1. Physicochemical characteristics of AmB-NLCs.
3.2. Determination of entrapment efficacy
The results revealed that all formulations showed high drug entrapment efficiency of ∼75%. The amount of Eu had no effect on drug entrapment efficiency. To confirm the incorporation of drug in the NLCs, the polarized light microscopy was used to investigate the presence of unincorporated drug crystals that might be suspended in the external aqueous phase of NLCs dispersion. This technique is well established method to examine the unincorporated drug (Chang & Bodmeier, Citation1997; Das et al., Citation2011). In our preliminary study, the prepared NLCs, containing 40 mg of AmB, did not show any unincorporated drug crystals in the external aqueous phase, and only dark background was observed suggesting that almost all of drug was incorporated into NLCs.
3.3. Solubility of free AmB and AmB-NLCs
It is well established that AmB is a poorly water-soluble drug of less than 1 µgmL−1. However, the drug is soluble at a pH below 2 or above 11, but under such harsh conditions, the molecule is not stable and less antimycotic activity (Lemke et al., Citation2005). In this study, AmB powder possessed highly solubility in pH 1.2 HCl, and FaSSGF of 8.85 ± 0.25 and 11.01 ± 1.61 µgmL−1, respectively, while in water, pH 6.8 PBS and FaSSIF showed poorly solubility of 0.70 ± 0.02, 0.32 ± 0.06, and 0.43 ± 0.04 µgmL−1, respectively. Interestingly, both uncoated NLCs and Eu-NLCs showed enhancement in drug solubility, the AmB water solubility is significantly greater than the free drug by 4-fold and up to 10-fold, respectively (). Moreover, drug solubility of uncoated NLCs and Eu-NLCs is also significantly greater than the free drug by 6-fold in FaSSIF. These results suggested that AmB-NLCs can improve the solubility of the drug. The increase in drug solubility could be attributed to two critical factors. Firstly, small particle size could increase the surface area and wettability of the drug, which would lead to an increase in the solubility of drug in the dissolution medium (Williams et al., Citation2013). Secondly, most of the drug loaded in NLCs were in an amorphous state. Several studies reported that during the formation of solid lipid particles by rapid quenching in a cold aqueous medium, the drug molecules in the nanoemulsion droplet could not nucleate and form the crystal lattice (Venkateswarlu & Manjunath, Citation2004; Kalam et al., Citation2013; Niamprem et al., Citation2018). Thus, the drug molecule dispersed in the lipid matrix remains in an amorphous state which has a higher solubility than crystalline form (Hancock & Parks, Citation2000).
Table 2. Solubility of AmB-NLCs in different dissolution medium.
3.4. In vitro dissolution studies
The in vitro drug release profile of uncoated NLCs and Eu-NLCs in FaSSGF and FaSSIF are shown in . The results showed that the Eu-NLCs were more effective in preventing AmB degradation in stomach than uncoated NLCs. In FaSSGF, uncoated NLCs showed burst release of AmB, ∼60% within 5 min, implying ∼40% of AmB was retained in NLCs core due to the hydrophobic interaction between AmB and lipid NLC components. After 2 h, ∼30% of the released drug was degraded possibly due to hydrolysis of the ester bonds and/or oxidation of the polyene chains in the lactone ring (Volmer et al., Citation2010) (). Nevertheless, the uncoated NLCs show greater AmB protection effect in an acidic medium as compared to free amB, ∼60% degraded in 2 h. On the other hand, when coated with Eu, no drug release was observed, indicating 100% of AmB remained in the particles. These results could be explained by Eu, a pH-sensitive polymer, is not dissolved in acidic condition. Therefore, it is capable of protecting the drug being degraded in the stomach (Sauer & McGinity, Citation2009; Wulff & Leopold, Citation2016). Eu has been successfully used for increasing the therapeutic effects of many drugs substances, such as curcumin, insulin, and omeprazole (Li et al., Citation2006; Hao et al., Citation2013; Manconi et al., Citation2019). Therefore, this polymer is effective in preserving intact AmB to reach the absorption site in the intestine. In summary, compared to the free AmB, both uncoated NLCs and Eu-NLCs could protect the drug from degradation in the gastric fluid, with the latter was more effective than the former.
Figure 3. %Cumulative drug released from (
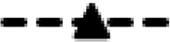
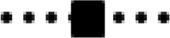
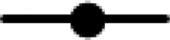
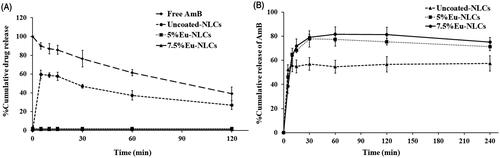
In FaSSIF, the drug release profile of uncoated and Eu-NLCs was similar (). They showed a fast release characteristic with a burst release in the first 10 min. The fast release could be explained by the NLCs small particle size and amorphous AmB, as discussed before. Moreover, the rapid initial drug release was attributed to most of the drugs associated near the NLCs surface. However, Eu-NLCs exhibited ∼80% maximum release within 30 min, while uncoated NLCs showed ∼60% maximum release. The higher drug release of Eu-NLCs could be explained by the solubility of the drug in the dissolution medium. In FaSSIF, Eu-NLCs showed a higher AmB solubility than uncoated NLCs () thus, leading to a significantly higher drug release than uncoated NLCs.
3.5. In vitro permeation studies in Caco-2 cell monolayer
Before the permeation experiments, the cytotoxicity test of the AmB-NLCs on Caco-2 cells were performed using MTT assay to ensure there was no effect on cell viability. According to International Organization for Standardization (ISO 10993-5) (ISO 2009), the cell viability of >70% was considered as nontoxic. The results showed that at high AmB concentrations (15 μgmL−1), the cell viability decreased to ∼50%, while all AmB-NLCs tested at lower AmB concentration (1, 5, and 10 μgmL−1) possessed cell viability >90% indicating no cytotoxicity (data not shown). According to the cell viability, the highest concentration used in the permeation study was equivalent to AmB 10 µgmL−1.
AmB is not a substrate for P-glycoprotein (P-gp) (Yang et al., Citation2014; Osei-Twum & Wasan, Citation2015), thus, only drug transport from apical to basolateral compartment was investigated. AmB-NLCs, Eu-NLCs, and free drug were added through the apical compartment and samples were collected accordingly from the basolateral compartment. showed the AmB distribution in basolateral compartment and cell monolayer. Interestingly, the absorption efficiency of AmB could be enhanced by incorporation into the NLCs. The results showed that both uncoated and Eu-NLCs could enhance the AmB permeation across Caco-2 cell monolayer compared to free drug. At the end of the incubation, the amount of AmB in the basolateral side of uncoated and Eu-NLCs was detected, indicating that AmB was permeated across the Caco-2 cell monolayers. In contrast, the amount of drug in the basolateral side was not detected in free AmB. However, free drug was more detected in the cell monolayers than those of NLCs formulations. These results could be explained by the interaction between drug and cell membrane. It is well known that the target of AmB is membrane sterols, leading to the formation of an AmB-sterol complex (Fujii et al., Citation1997). Furthermore, detected drug in the cell could be influenced by DMSO using for dissolving drugs resulting in enhanced cell membrane permeability of drugs into Caco-2 cell monolayer (de Ménorval et al., Citation2012). Thus, free drug showed retaining in the cell monolayer, but was not permeated into the basolateral compartment.
Table 3. The percentage of AmB in apical side, basolateral side, and cell monolayer after 6-h incubation.
The corresponding Papp values were calculated based on the ability of drug across the cell monolayers. The correlation between the absorbed fraction in humans and permeability across the Caco-2 cell monolayer has been evaluated in many studies. Yee et al. suggested that a compound with Papp less than 1 × 10−6 cms−1 showed low absorption in vivo (0–20%), while a Papp between 1–10 × 10−6 cms−1 corresponded to moderate absorption (20–70%), and Papp higher than 10 × 10−6 cms−1 was indicative of high absorption (70–100%) (Yee, Citation1997). As a result, uncoated NLCs provide significantly higher Papp value, ∼5.84 × 10−6 cms−1, than 5%Eu-NLCs and 7.5%Eu-NLCs, ∼1.55 × 10−6 and ∼1.84 × 10−6 cms−1, respectively, . According to the Papp values, AmB-NLCs were classified as a moderate permeability. The absorption efficiency of drug was enhanced in AmB-NLCs could be attributed to two critical reasons. Firstly, the nano-sized AmB-NLCs can improve the drug solubility which resulting in increased drug concentration gradient at the absorption site. As a consequence, this could improve drug permeation via transcellular transport. Secondly, AmB-NLCs could be directly transported across the cell barrier into the basolateral side via endocytosis. Several studies reported that smaller particles show greater cellular uptake than the larger ones (Florence, Citation2004; Kulkarni & Feng, Citation2013; Neves et al., Citation2016). Accordingly, uncoated NLCs with particle size ∼180 nm exhibited greater Papp value than Eu-NLCs with particle size ∼500–600 nm. Furthermore, the lipid-based formulations have been reported to stimulate chylomicron formation and facilitate lymphatic uptake, which can avoid the first-pass metabolism of drugs resulting in the increased therapeutic efficacy of drugs (Trevaskis et al., Citation2008; Ali Khan et al., Citation2013). Considering overall, the results suggested that the AmB-NLCs present a high potential to improve the oral bioavailability of drugs.
3.6. Stability study of AmB-NLCs
To assess the effect of temperature on physicochemical stability, all formulations were stored at different storage temperatures (4 °C and room temperature) over a period of 6 months. The changes in mean particle size, zeta potential, and percent drug remaining were evaluated. After 6 months of storage, the mean particle size and zeta potential of tested formulations showed no significant difference as compared to those of initial preparations ( and ). Zeta potential is a significant tool in predicting the physical stability of NLCs. Generally, zeta potential values of more than ǀ30ǀ mV indicate good physical stability, in which aggregation and agglomeration can be avoided by electrostatic barriers (Clogston & Patri, Citation2011). Therefore, the developed NLCs are likely to be stable.
Table 4. Mean size of AmB-NLCs after 3 and 6 months at 4 °C and RT in the absence of the day light.
Table 5. Zeta potential of AmB-NLCs after 3 and 6 months at 4 °C and RT in the absence of the day light.
In addition, the percentage of drug remaining at a different time and temperature storage was shown in . From the results, all formulations showed a percent drug remaining of approximately 90–100% at both storage temperatures. Thus, the finding indicated that lyophilized AmB-NLCs were physical and chemical stability for at least 6 months of storage at 4 °C or room temperature.
4. Conclusion
The developed uncoated and Eu-NLCs were successfully prepared by an HPH with potential capability to enhance AmB oral bioavailability. They manifested the ability to increase AmB water solubility, stability in gastric fluid, and permeation across Caco-2 cell monolayer as compared to free drug. The particles coated with Eudragit®L100-55, an enteric polymer, possessed a larger particle size of ∼550 nm with a charge of ∼−50 mV, while uncoated NLCs possessed a mean particle size of ∼180 nm with a charge of ∼−30 mV. Both developed NLCs can significantly enhance AmB water solubility more than the free drug by 4-fold and up to 10-fold, respectively. Although, uncoated NLCs showed greater AmB permeation across Caco-2 cells than Eu-NLCs, but they suffered from some AmB degradation in acidic medium. Therefore, the use of enteric coating capsules or tablets could further increase its effectiveness in delivering AmB orally. In contrast, Eu-NLCs were advantageous in its simplicity in the preparation process and its ability to retain the particles in the nano-size range. In conclusion, uncoated and Eu-NLCs present a promise as an oral drug delivery system for AmB. Nevertheless, there is certainly good justification for further work, including performance in animal studies or possible human studies.
Acknowledgments
The authors thank Dr. Duy Toan Pham for helpful discussions. The authors acknowledge the Faculty of Pharmaceutical Sciences, Naresuan University for providing necessary facilities.
Disclosure statement
No potential conflict of interest was reported by the author(s).
Additional information
Funding
References
- Ali Khan A, Mudassir J, Mohtar N, et al. (2013). Advanced drug delivery to the lymphatic system: lipid-based nanoformulations. Int J Nanomed 8:2733–44.
- Chang CM, Bodmeier R. (1997). Swelling of and drug release from monoglyceride-based drug delivery systems. J Pharm Sci 86:747–52.
- Clogston JD, Patri AK. (2011). Zeta potential measurement. In: McNeil SE, ed. Characterization of nanoparticles intended for drug delivery. Totowa (NJ): Humana Press.
- Das S, Ng WK, Kanaujia P, et al. (2011). Formulation design, preparation and physicochemical characterizations of solid lipid nanoparticles containing a hydrophobic drug: effects of process variables. Colloids Surf B 88:483–9.
- de Ménorval MA, Mir LM, Fernández ML, et al. (2012). Effects of dimethyl sulfoxide in cholesterol-containing lipid membranes: a comparative study of experiments in silico and with cells. PLoS One 7:e41733.
- Florence AT. (2004). Issues in oral nanoparticle drug carrier uptake and targeting. J Drug Target 12:65–70.
- Fujii G, Chang JE, Coley T, et al. (1997). The formation of amphotericin B ion channels in lipid bilayers. Biochemistry 36:4959–68.
- Hamill RJ. (2013). Amphotericin B formulations: a comparative review of efficacy and toxicity. Drugs 73:919–34.
- Hancock BC, Parks M. (2000). What is the true solubility advantage for amorphous pharmaceuticals? Pharm Res 17:397–404.
- Hao S, Wang B, Wang Y, et al. (2013). Preparation of Eudragit L 100-55 enteric nanoparticles by a novel emulsion diffusion method. Colloids Surf B Biointerf 108:127–33.
- ISO, (2009). Biological evaluation of medical devices – part 5: testes for in vitro cytotoxicity. International Organization for Standardization.
- Kalam MA, Sultana Y, Ali A, et al. (2013). Part I: development and optimization of solid-lipid nanoparticles using Box–Behnken statistical design for ocular delivery of gatifloxacin. J Biomed Mater Res 101A:1813–27.
- Khan MZI, Prebeg Ž, Kurjaković N. (1999). A pH-dependent colon targeted oral drug delivery system using methacrylic acid copolymers: I. Manipulation of drug release using Eudragit® L100-55 and Eudragit® S100 combinations. J Control Release 58:215–22.
- Kulkarni SA, Feng SS. (2013). Effects of particle size and surface modification on cellular uptake and biodistribution of polymeric nanoparticles for drug delivery. Pharm Res 30:2512–22.
- Lee MK, Kim MY, Kim S, et al. (2009). Cryoprotectants for freeze drying of drug nano-suspensions: effect of freezing rate. J Pharm Sci 98:4808–17.
- Lemke A, Kiderlen AF, Kayser O. (2005). Amphotericin B. Appl Microbiol Biotechnol 68:151–62.
- Li MG, Lu WL, Wang JC, et al. (2006). Preparation and characterization of insulin nanoparticles employing chitosan and poly(methylmethacrylate/methylmethacrylic acid) copolymer. J Nanosci Nanotechnol 6:2874–86.
- Manconi M, Manca ML, Escribano-Ferrer E, et al. (2019). Nanoformulation of curcumin-loaded Eudragit-nutriosomes to counteract malaria infection by a dual strategy: improving antioxidant intestinal activity and systemic efficacy. Int J Pharm 556:82–8.
- Mehnert W, Mäder K. (2001). Solid lipid nanoparticles: production, characterization and applications. Adv Drug Deliv Rev 47:165–96.
- Moustafine R, Margulis E, Sibgatullina L, et al. (2008). Comparative evaluation of interpolyelectrolyte complexes of chitosan with Eudragit® L100 and Eudragit® L100-55 as potential carriers for oral controlled drug delivery. Eur J Pharm Biopharm 70:215–25.
- Müller RH, Radtke M, Wissing SA. (2002). Solid lipid nanoparticles (SLN) and nanostructured lipid carriers (NLC) in cosmetic and dermatological preparations. Adv Drug Deliv Rev 54:S131–S155.
- Neves AR, Queiroz JF, Costa Lima SA, et al. (2016). Cellular uptake and transcytosis of lipid-based nanoparticles across the intestinal barrier: relevance for oral drug delivery. J Colloid Interf Sci 463:258–65.
- Niamprem P, Srinivas S, Tiyaboonchai W. (2018). Development and characterization of indomethacin-loaded mucoadhesive nanostructured lipid carriers for topical ocular delivery. Int J Appl Pharm 10:91.
- Osei-Twum JA, Wasan KM. (2015). Does P-glycoprotein contribute to amphotericin B epithelial transport in Caco-2 cells? Drug Dev Ind Pharm 41:1130–6.
- Ouellette M, Drummelsmith J, Papadopoulou B. (2004). Leishmaniasis: drugs in the clinic, resistance and new developments. Drug Resist Updat 7:257–66.
- Pardeike J, Hommoss A, Müller RH. (2009). Lipid nanoparticles (SLN, NLC) in cosmetic and pharmaceutical dermal products. Int J Pharm 366:170–84.
- Pataranapa N, Waree T, Supaporn L. (2019). Amphotericin B loaded nanostructured lipid carriers for parenteral delivery: characterization, antifungal and in vitro toxicity assessment. Curr Drug Deliv 16:645–53.
- Sauer D, McGinity J. (2009). Properties of theophylline tablets dry powder coated with Eudragit E PO and Eudragit L 100-55. Pharm Dev Technol 14:632–41.
- Shahgaldian P, Gualbert J, Aïssa K, et al. (2003). A study of the freeze-drying conditions of calixarene based solid lipid nanoparticles. Eur J Pharm Biopharm 55:181–4.
- Shete H, Patravale V. (2013). Long chain lipid based tamoxifen NLC. Part I: preformulation studies, formulation development and physicochemical characterization. Int J Pharm 454:573–83.
- Trevaskis NL, Charman WN, Porter CJH. (2008). Lipid-based delivery systems and intestinal lymphatic drug transport: a mechanistic update. Adv Drug Deliv Rev 60:702–16.
- Venkateswarlu V, Manjunath K. (2004). Preparation, characterization and in vitro release kinetics of clozapine solid lipid nanoparticles. J Control Release 95:627–38.
- Volmer AA, Szpilman AM, Carreira EM. (2010). Synthesis and biological evaluation of amphotericin B derivatives. Nat Prod Rep 27:1329–49.
- Wang XQ, Zhang Q. (2012). pH-sensitive polymeric nanoparticles to improve oral bioavailability of peptide/protein drugs and poorly water-soluble drugs. Eur J Pharm Biopharm 82:219–29.
- Wasan EK, Bartlett K, Gershkovich P, et al. (2009). Development and characterization of oral lipid-based Amphotericin B formulations with enhanced drug solubility, stability and antifungal activity in rats infected with Aspergillus fumigatus or Candida albicans. Int J Pharm 372:76–84.
- Williams HD, Trevaskis NL, Charman SA, et al. (2013). Strategies to address low drug solubility in discovery and development. Pharmacol Rev 65:315–499.
- Wissing SA, Kayser O, Müller RH. (2004). Solid lipid nanoparticles for parenteral drug delivery. Adv Drug Deliv Rev 56:1257–72.
- Wulff R, Leopold CS. (2016). Coatings of Eudragit® RL and L-55 blends: investigations on the drug release mechanism. AAPS PharmSciTech 17:493–503.
- Yang Z, Chen M, Yang M, et al. (2014). Evaluating the potential of cubosomal nanoparticles for oral delivery of amphotericin B in treating fungal infection. Int J Nanomed 9:327–36.
- Yee SY. (1997). In vitro permeability across caco-2 cells (colonic) can predict in vivo (small intestinal) absorption in man—fact or myth. Pharn Res 14:763–6.