Abstract
Rheumatoid arthritis is a chronic autoimmune disease, with the features of recurrent chronic inflammation of synovial tissue, destruction of cartilage, and bone erosion, which further affects joints tissue, organs, and systems, and eventually leads to irreversible joint deformities and body dysfunction. Therapeutic drugs for rheumatoid arthritis mainly reduce inflammation through regulating inflammatory factors. Transdermal administration is gradually being applied to the treatment of rheumatoid arthritis, which can allow the drug to overcome the skin stratum corneum barrier, reduce gastrointestinal side effects, and avoid the first-pass effect, thus improving bioavailability and relieving inflammation. This paper reviewed the latest research progress of transdermal drug delivery in the treatment of rheumatoid arthritis, and discussed in detail the dosage forms such as gel (microemulsion gel, nanoemulsion gel, nanomicelle gel, sanaplastic nano-vesiclegel, ethosomal gel, transfersomal gel, nanoparticles gel), patch, drug microneedles, nanostructured lipid carrier, transfersomes, lyotropic liquid crystal, and drug loaded electrospinning nanofibers, which provide inspiration for the rich dosage forms of transdermal drug delivery systems for rheumatoid arthritis.
Introduction
Rheumatoid arthritis (RA) is a chronic autoimmune disease accompanied by infiltration of inflammatory cells and proliferation of synovial fibroblasts, which can lead to synovial damage, cartilage damage, and joint deformities (Deng et al., Citation2021). The incidence rate of RA is about 1% of the total population, and it is higher in females than in males. RA is accompanied by a trend from mild injury to severe disability, which can reduce the life expectancy of patients from 10 to 15 years (Scott et al., Citation2010).
The physical and chemical properties, genetic factors, and environmental factors involved in RA are complicated, so the specific pathogenesis of RA is still not completely clear. However, the pathogenic inflammatory factors of RA have been extensively studied (Brzustewicz & Bryl, Citation2015). The etiology of RA includes the complex role of genetic factors, external environmental factors and specific risk factors (smoking, periodontitis, gut microbiome) on individuals. The complex role resulted in the over-expression of autoantibodies rheumatoid factor (RFs) and anti-citrullinated protein antibodies (ACPAs), which would activate B cells, T cells and macrophages, then resulting in the produce of inflammatory factors (mainly consist of tumor necrosis factor-α (TNF-α), interleukin-6 (IL-6), and interleukin-1 (IL-1)) (McInnes & Schett, Citation2017; Gorantla et al., Citation2020; Petrovska et al., Citation2021). These inflammatory factors destroy cartilage and bone, and finally result in joint erosion (). TNF-α can activate the abnormal proliferation of fibroblast-like synoviocytes (FLS), which leads to overexpression of cathepsin and matrix metalloproteinases (MMPs). Then collagen and proteoglycan breakdown, finally leading cartilage and bone destruction (Yoshitomi, Citation2019). After binding to the receptor, IL-6 can trigger a dimerization signal of the cell surface molecule glycoprotein p130, which activates the Janus kinase (JAK)/activator of transcription (STAT) pathway, thus promote inflammation (Simon et al., Citation2021; Weng et al., Citation2021). IL-1 and TNF-α could activate Nuclear factor Kappa-B (NF-κB) pathway, which upregulation cyclooxygenase 2 (COX-2) and proinflammatory cytokine MMP, finally resulting in cartilage degeneration and synovial erosion (Wang et al., Citation2017; Guo et al., Citation2019).
The dosage forms of traditional arthritis treatment drugs, such as tablets, capsules, and injections, have certain therapeutic effects. But these dosage forms have limiting factors. For example, oral agents have disadvantages of low bioavailability, first-pass effect, and liver and kidney toxicity, and injections have skin irritation and infection. The American college of rheumatology and European league against rheumatism recommend oral methotrexate as the preferred recommendation for patients with moderate or high disease severity. For patients with oral intolerance of methotrexate, subcutaneous injection of methotrexate within 24 h or every week is recommended. The transdermal drug delivery system (TDDS), also known as transdermal preparation, enables drugs to enter the blood circulation through the skin barrier at a relatively low dose and finally reach the action site (Jain et al., Citation2021). Compared with traditional dosage forms, TDDS can avoid first-pass effects and reduce gastrointestinal side effects, thereby improving bioavailability and having high compliance. This paper reviews the latest research progress of transdermal drug delivery in RA treatment, and discusses the transdermal drug delivery types in detail, which provides innovative ideas to the transdermal drug delivery for RA treatment.
Therapeutic drugs for rheumatoid arthritis
In the treatment of mild and moderate RA, drugs can be used to relieve the disease or improve joint function, but severe arthritis can only be controlled by surgical replacement of joints combined with drug treatment. Therapeutic drugs for RA mainly include non-steroidal anti-inflammatory drugs (NSAIDs), glucocorticoids drugs (GCs), disease-modifying anti-rheumatic drugs (DMARDs) (Bacelo et al., Citation2019). The DMARDs are divided into traditional conventional synthetic DMARDs (csDMARDs), biological DMARDs (bDMARDs) and targeted synthetic DMARDs (tsDMARDs) (Kohler et al., Citation2019).
NSAIDs are widely used to reduce the symptoms of RA, which are a class of anti-inflammatory drugs that do not contain steroidal skeletons. Most of NSAIDs have the basic structure of aromatic acid with pKa values of 3–5 (Badri et al., Citation2016). According to different aryl structures and substituents, the hydrophilic and lipophilic properties of drugs will be different (Miranda et al., Citation2021). For example, the partition coefficient values (logP) for NSAIDs ketoprofen, celecoxib, indomethacin, flurbiprofen, and diclofenac are 0.97, 3.53, 3.8, 4.16 and 4.51, respectively (Subongkot & Sirirak, Citation2020; Arunprasert et al., Citation2021; Miranda et al., Citation2021). NSAIDs have antipyretic, analgesic, and anti-inflammatory effects, which can inhibit the conversion of arachidonic acid to prostaglandin by inhibiting COX-2, and then reducing the production of pain-causing prostaglandin (Bazan et al., Citation2002; Abbasi et al., Citation2019). In addition, the decrease of prostaglandin can inhibit the activation of inflammatory T helper lymphocytes 17, then inhibiting the transformation of T cells into an inflammatory state, and further reducing the secretion of inflammatory factors (Jia et al., Citation2014; Samuels et al., Citation2018).
GCs are a class of hormone drugs with cyclopentanoperhydro-phenanthrene nucleus, which have anti-inflammatory and immunosuppressive effects. Through interacting with the cytoplasmic glucocorticoid receptor and regulating gene transcription, GC can inhibit the pro-inflammatory effect of M1 macrophages, thereby effectively decreasing the production of inflammatory cytokines (Ehrchen et al., Citation2019). Short-term use of the GCs drug prednisone is an effective way to quickly relieve the symptoms of RA patients (Cutolo et al., Citation2021).
CsDMARDs are slow-acting drugs, with no common mechanism of action, and the side effects of different drugs are also different. Methotrexate (MTX) and leflunomide (LEF) are commonly used csDMARDs. MTX is a small molecular drug with the logP of −1.8 and melting point of 195 °C, which has a negative charge at physiological pH (Vora et al., Citation2022). MTX monotherapy is characterized by established efficacy and safety, which is the first-line medication for treating RA. MTX can induce the release of adenosine. The combination of adenosine with adenosine A2 and A3 receptor subtypes can inhibit lymphocyte proliferation and reduce the production of proinflammatory cytokines (IL-1, IL-6, and TNF-α) (Cutolo et al., Citation2001). LEF is a synthetic isoxazole derivative used clinically as an immunomodulator for the treatment of RA, with a molecular weight of 270.20 and a logP value of 2.5 (Pund et al., Citation2015). LEF limits the synthesis of pyrimidine by inhibiting dihydroorotate dehydrogenase, so that the proliferation of T cells can be inhibited, further resulting in reducing the secretion of inflammatory cytokines (Alamri et al., Citation2021).
BDMARDs can achieve specificity and efficacy anti-inflammatory effects by targeting specific inflammatory factors or inflammatory cells, which have gradually been widely used in the treatment of RA. BDMARDs include TNF-α inhibitors, IL-6 receptor inhibitors, T cell costimulatory inhibitor, anti-CD20 antibody (Zhang et al., Citation2020; Fraenkel et al., Citation2021). TNF-α inhibitors include Etanercept, Infliximab, Adalimumab, Golimumab, Certolizumab, which can binds and inactivates TNF-α to reduce the production of inflammatory factors (Salem et al., Citation2021). IL-6 receptor inhibitors include tocilizumab and Sarilumab. The monoclonal anti-body tocilizumab can specifically targeted the IL-6 receptor on cell surfaces for the treatment of RA (Nozawa et al., Citation2019). The T cell costimulatory inhibitor Abatacept could inhibit the binding of costimulatory molecules CD80/CD86 on the surface of antigen-presenting cells, thereby inhibiting the activation of T cells, thus reducing the occurrence of subsequent inflammatory reactions (Blair & Deeks, Citation2017). Anti-CD20 antibody Rituximab is a monoclonal antibody that could specifically target CD20 molecules expressed on the surface of B cells, which could cause apoptosis of B cells and reduce the immune response of these B cells (Tavakolpour et al., Citation2019).
TsDMARDs includes Tofacitinib, Baricitinib, Upadacitinib and Peficitinib, which are selective JAK inhibitors that can target the JAK pathway, reducing the conduction of inflammatory signaling and the production of inflammatory cytokines (Dhillon, Citation2017; Angelini et al., Citation2020). TsDMARDs are small molecular drugs, in which Tofacitinib is the most commonly used, with the molecular weight of 312.37 and a logP value of 1.8 (Kathuria et al., Citation2020).
Drug dosage form for treating RA
Oral agents and injections are common dosage forms of drugs to treat RA (Guo & Pratap Singh, Citation2019). Oral agents have the advantages of various dosage forms and convenient administration, so they are the most common way of drug administration. Injections, as a delivery form that can directly inject drugs into the body, has some advantages such as rapid onset, avoiding the first-pass effect, and accurate dosage. Although oral agents and injections are the main ways to treat RA, they still have a few limitations.
Oral NSAIDs, such as ibuprofen sustained-release tablets and ibuprofen sustained-release capsules, are nonselective cyclooxygenase (COX) enzyme inhibitors, which can simultaneously inhibit COX-1 and COX-2 (Thakur et al., Citation2018). However, the inhibition of COX-1 could cause gastrointestinal discomfort (Solomon et al., Citation2017). The selective COX-2 inhibitor Celecoxib has low solubility (3–7 μg/ml), strong first-pass metabolism and rapid plasma clearance rate, so Celecoxib capsules have only 22–40% bioavailability when administered orally (Quinones & Pierre, Citation2019). Although low-dose oral administration of GCs is an effective route of administration, it needs optimal time selection when used, so it has poor compliance, while it has large systemic adverse reactions with long-term usage (Cutolo et al., Citation2021). CsDMARDs oral drugs, for instance, MTX, not only have the disadvantages of first-pass effect and low bioavailability, but also cause loss of appetite (Wohlrab et al., Citation2015). The long-term use of LEF capsules can also bring about the side-effects of gastrointestinal disorders, such as diarrhea, nausea, and abdominal pain (Alamri et al., Citation2021). Additionally, TsDMARDs Tofacitinib can be taken orally to treat RA. Occasionally oral Tofacitinib will bing side effort gastrointestinal disorders (nausea, diarrhea) to patient (Yamaoka, Citation2016).
In Injection of CsDMARDs, the use of subcutaneous injection of MTX could overcome the limitations of oral MTX. Meanwhile, subcutaneous injection of MTX has linear and predictable pharmacokinetic characteristics, which can increase the bioavailability of MTX (Vena et al., Citation2018). However, the methotrexate injection would quickly eliminate from the body, and frequent injections will cause damage to the injection site (Prabhu et al., Citation2012; Garg et al., Citation2016). At present, bDMARDs injection is an effective way for RA clinical treatment. The main feature of the bDMARDs injection is that it takes effect quickly, and bDMARDs have good therapeutic specificity against specific targets of immune response (Law & Taylor, Citation2019). However, during the long-term injection administration of bDMARDs, it was easy to cause problems such as infection at the injection site, muscle pain and edema. These problems reduce the patient’s compliance with medication (Isabwe et al., Citation2017; Ito, Citation2018; Cao et al., Citation2019).
Oral and injection dosage forms of commonly used drugs for the treatment of RA are shown in .
Table 1. Commonly used oral and injectable drug formulations for RA.
Transdermal administration can avoid the first-pass effect and gastrointestinal side effects of oral preparations and injections, and can also be independently administered with high medication compliance. There are fewer kinds and dosage forms of transdermal drug delivery for rheumatoid arthritis, mainly including Ibuprofen Gel and Ketoprofen Gel. With the optimization of the preparation process, the improvement of prescription technology, the emergence of new technology, and the development of new excipients, transdermal formulations for RA have been increasingly developed.
Transdermal administration
As the largest organ in the human body, skin is a natural barrier to protect the body from external invasion. The skin area is about 1.5–2.0 m2, accounting for 15% of the total weight of adults (Dąbrowska et al., Citation2018). Transdermal drug delivery first crosses the skin barrier. The skin consists of three layers: epidermis, dermis, and subcutaneous hypodermis () (Kalluri & Banga, Citation2011). The epidermis includes the upper layer of non-viable stratum corneum and the lower layer of viable epidermis. The stratum corneum (10–20 μm) is composed of 10–15 layers of inanimate keratinocytes, which are closely arranged so that drug molecules have difficulty in penetrating (Andrews et al., Citation2013). In addition, the non-viable stratum corneum contains multiple lipid bilayers, which form an enormous barrier to the penetration of hydrophilic molecules. The viable epidermal layer does not contain blood vessels, so it also has a blocking effect on drug absorption (Pan et al., Citation2020). The dermis is located in the lower part of the epidermis, which contains fibroblasts, adipocytes, blood vessels, and lymphatic capillaries, as well as a large number of hair follicles, sebaceous glands, and sweat glands. Drugs are mainly absorbed in the dermis (Romgens et al., Citation2015). The hypodermis, below the dermis, is mainly composed of loose connective tissue and fat, which has the function of preventing heat dissipation and storing energy (Wong et al., Citation2016).
The drug absorption route of transdermal administration can be divided into the transepidermal route and skin appendage route. As the main absorption route, the transepidermal route can be divided into the transcellular route and intercellular route () (Kotla et al., Citation2017). In the transcellular route, the drug needs to pass through the stratum corneum lipid membrane structure, and keratin that filled with highly hydrated cells also limit drug penetration. The transcellular route requires multiple hydrophilic/oleophilic distribution balances, so fewer drugs can pass through it (Rabiei et al., Citation2020). In the intercellular pathway, drug molecules enter the dermis through the lipid matrix between keratinocytes, and then the abundant capillaries in the dermis effectively absorb the drug, allowing it to enter the circulation. So, the intercellular pathway is the main route of drug absorption (Iachina et al., Citation2019).
Figure 3. Transdermal route of drug absorption. Copyright 2017, American Chemical Society (Kotla et al., Citation2017).
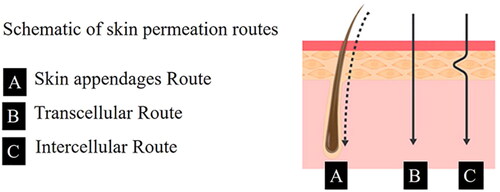
The absorption route of skin appendages refers to the absorption of drugs through hair follicles, sweat glands, and sebaceous glands. The drug penetration rate in this route is faster than that in the transepidermal route. However, the absorption area of skin appendages is small (about 0.1% of the total skin area), so the skin appendages is not the main way of drug transdermal absorption (Alkilani et al., Citation2015).
Currently, there are only about 20 transdermal therapeutic preparations approved by the FDA. The tight arrangement of the skin and the rich structure of the lipid membrane restrict the percutaneous absorption of exogenous drugs, and only a small number of drugs with ideal physical and chemical properties could pass through the stratum corneum (Prausnitz & Langer, Citation2008). Transdermal delivery usually requires the drug molecular weight less than 600 Da. The logP should be in the range of 1.0–3.0, and the low melting point less than 200 °C (Larrañeta et al., Citation2016).
It is essential to overcome the inherent barrier of the skin and develop a safe and efficient transdermal drug delivery system. The research shows that the transdermal performance of drugs can be improved by development of drug formulations and combining with new preparation technology, which could approve the use of more and more conventional RA treatment drugs and natural medicine active ingredients for transdermal delivery.
RA transdermal dosage form
RA transdermal delivery has become a research hotspot. The Gels, patches, and microneedles dosage form are commonly transdermal delivery systems. Gels including microemulsion gel, nanoemulsion gel, nanomicelle gel, sanaplastic nano-vesiclegel, ethosomal gel, transfersomal gel and nanoparticles gel. The patch has good administration compliance, nanocarriers could be compounded into the patch to enhance the transdermal penetration. Microneedles, nanostructured lipid carrier, transfersomes, lyotropic liquid crystal, drug loaded electrospinning nanofibers are novel transdermal delivery systems, which have great application potential for RA transdermal drug delivery.
Gel
Gel is a three-dimensional polymer network formed by chemical or physical crosslinking of polymers. The gel polymer matrix, such as carbomer and cellulose derivatives, has hydrophilicity and can absorb large amounts of water, so that the gel can hydrate the skin stratum corneum and facilitate the penetration of drugs through the skin. Therefore, gel can be used as an effective semi solid drug delivery vehicle (Zhu et al., Citation2021). Constructing gel systems with different structures (microemulsion gel, nanoemulsion gel, nanomicellar gel, sanaplastic nano-vesicle gel, ethosomal gel, transferosomal gel, and nanoparticles gel) is a excellent way to improve the ability of drug penetration and drug bioavailability.
Microemulsion gel
Microemulsion, which is formed by water phase, oil phase, and surfactant, is a thermodynamically stable system. Microemulsion has high fluidity and low viscosity, and drug-loaded microemulsion ha the advantages of large drug loading and easy drug penetration through the skin. Microemulsion gel is prepared by adding drug-loaded microemulsion to the gel medium, which has both characteristics of microemulsion and gel. Not only can it maintain the advantages of drug loaded microemulsion, but it can also reduce the fluidity of the emulsion and realize drug sustained-release (Zhang et al., Citation2017).
Seok firstly mixed the surfactant Tween 80, cosurfactant diethylene glycol monoethyl ether, propylene glycol monocaprylate, and celecoxib (CLX) to prepare CLX microemulsion, then added carbomer to form CLX microemulsion gel. The rheological measurement results of the CLX microemulsion gel showed that it had good elasticity, which is beneficial to transdermal drug delivery. In vitro skin penetration experiments showed that the permeation flux of the CLX microemulsion gel was 9.79 ± 2.83 μg/cm2/h for 24 h, which reduced its permeation flux by 32.20% compared with the free drug group. The results revealed that the CLX microemulsion gel can delay the release of CLX and achieve the purpose of continuous treatment of arthritis (Seok et al., Citation2018). Shewaiter prepared the leflunomide(LEF)/diclofenac(DS) microemulsions by water titration method, then added carbopol to construct LEF-DS dual-drugs microemulsion gel. The drug loading capacity is high in this microemulsion gel, with the drug loading of 89.2% ± 1.42% w/v for LEF and 98.69% ± 0.31% w/v for DS. In vitro permeability experiments of excised rat skin showed that the in vitro permeation of LEF and DS conformed to the zero-order permeation model in PBS buffer, and the cumulative permeation rates of LEF and DS were 76.114% and 60.714% (24 h) respectively. Hematological parameters and histopathological evaluation of ankle joints results suggested that LEF-DS ME gel treatment can effectively inhibit foot swelling, and the anti-rheumatoid activity of LEF-DS ME gel is better than that of a single-drug gel (LEF ME gel and DS ME gel).A 28-day anti-arthritic evaluation assessment in arthritic rats confirmed that LEF-DS ME gel is effective in relieving arthritis with a good sustained release effect () (Shewaiter et al., Citation2021).
Figure 4. Transdermal delivery of leflunomide and diclofenac microemulsion gel with good sustained-release effect in the treatment of RA. Copyright 2021, Elsevier, (Shewaiter et al., Citation2021).
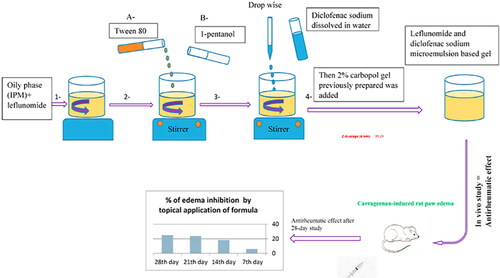
Nanoemulsion gel
Nanoemulsion is a thermodynamically unstable system formed by mixing oil phase, water phase, surfactant, and co-surfactant to an appropriate ratio. Nanoemulsion has abundant surface charge, smaller droplet size, and excellent kinetic stability against flocculation, which can improve the solubility and stability of loaded drugs (Rai et al., Citation2018). However, when the drug-loaded nanoemulsion is directly transdermally administered, there are limitations such as evaporation of the water phase, low viscosity, and high fluidity. Nanoemulsion gel was prepared by combining nanoemulsions with a gel matrix to expand its application for transdermal drug delivery (Yang et al., Citation2017).
Gokhale firstly prepared QCT nanoemulsion, and then dispersed it into Carbopol to obtain QCT nanoemulsion gel. In vitro permeation experiments, the drug permeability of QCT-NE gel and free QCT gel (QCT directly compounded with Carbomer940) after 24 h were 62.51% ± 0.34% and 35.87% ± 0.21%, respectively. The drug is more likely to cross the skin barrier of the stratum corneum due to the small particle size of the nanoemulsion. At the same time, the gel matrix hydrates with the skin, which swells the stratum corneum cells, further widening the drug channel, resulting in improving the drug permeability in QCT-NE gel. In the rat model of arthritis induced by Complete Freund’s adjuvant (CFA), the paw circumference of the blank control group was 71.21 ± 0.33 mm on the 28th day, while the QCT-NE gel group was reduced to 51.13 ± 1.35 mm, demonstrating that QCT-NE gel could effectively inhibit inflammation and alleviate the pathological changes of arthritis (Gokhale et al., Citation2019). Tacrolimus (TL) is an immunosuppressant that inhibits calcineurin. It has good anti-inflammatory activity and inhibits expression of TNF-α and IL-1 (Thao le et al., Citation2016). Aziz prepared TL nanoemulsion by high-speed shear homogenization, and mixed carbomer with TL nanoemulsion to construct tacrolimus nanoemulsion gel (TL-NG). The Zeta potential test showed that the repulsion between adjacent molecules of TL-NE was weak and flocculation did not easily occur. After preparing TL-NE into TL-NG, TL-NG can be stored at room temperature for 1.59 years with good stability. In vitro permeability experiments showed that the drug permeation flux of TL-NG is 68.88 μg/cm2/h and the cumulative drug permeation was 1621.46 μg/24 h. TL-NG obviously improved transdermal permeability and promoted the transdermal absorption of TL compared with the commercially ointment (Protope®), (drug permeation flux was 33.43 μg/cm2/h, the cumulative drug penetration was 781.54 μg/24 h). As a result of in vivo experiments in rats, the local application of TL-NG in inflammatory sites for 24 h could reduce the edema volume of 41.8% of rat paws with arthritis induced by bovine type II collagen and CFA. Based on the above conclusions, TL-NG has a good anti-inflammatory effect (Aziz et al., Citation2017). Yang designed magnesium oil-MTX nanoemulsion (MTX-MONE) to improve the permeability of MTX, and added carbomer 940 to MTX-MONE to construct the MTX-loaded nanoemulsion gel (MTX-MONEG). In vitro drug release and in vitro permeation experiments showed that the drug release and the penetration of MTX-MONE after 24 h were 93.60% ± 3.20% and 100%, respectively, while the drug release of MTX-MONEG after 24 h was 82.6% ± 8.50% and 93.5%, therefore MTX - MONEG can achieve sustained release of MTX and prolong the action time of the drug. The anti-inflammatory experiment in rats showed that MTX-MONEG can significantly inhibit expression of TNF-α, IL-1, and IL-6 inflammatory factors, which can effectively decrease the volume of inflammation and swelling (Yang et al., Citation2020). Bashir designed diflunisal (DIF-IC) loaded nanoemulsions, and combining xanthan gum (XG) with it to prepare DIF-IC-XG nanoemulsion gel. The in vivo experiment in mice showed that DIF-IC-XG nanoemulsion gel could significantly reduce the paw edema volume compared with DIF-IC nanemulsion and commercially available emulgel (diclofenac diethylamine) in the mouse paw swelling model induced by carrageenan, histamine, and formalin (Bashir et al., Citation2021). Resveratrol (RSV) can reduce expression of pro-inflammatory cytokines IL-1, IL-6, and TNF-α (Mittal et al., Citation2021). In the treatment of RA, the combination of MTX and RSV has a synergistic effect (Jia et al., Citation2016). Poonia developed the MTX and RSV co-loaded MTX-RSV nanoemulsion gel. In vitro drug permeation studies on rat skin showed that the permeation of MTX and RSV in the MTX-RSV nanoemulsion gel for 48 h was 64.54% and 73.91%, respectively which had high drug penetration. In a rat model of carrageenan-induced foot swelling, the MTX-RSV nanoemulsion gel can reduce 69.61% of the foot swelling volume after 3 h of topical application. In the model of formaldehyde-induced arthritis in rats, compared with the blank treatment group, the MTX-RSV nanoemulsion gel group could significantly reduce joint swelling and narrow the joint diameter of swollen joints after 10 days (Poonia et al., Citation2020).
Nanomicelle gel
Nanomicelle gel is formed by loading drugs into nanomicelles, and then adding it to the gel matrix. Nanomicelles have amphiphilic characteristics, which can load lipophilic and hydrophilic drugs. The hydrophilic structure can resist recognition by the reticuloendothelial system (RES), thus prolonging the drug action time in vivo and improving drug bioavailability. In order to reduce the barrier ability of the stratum corneum and promote drug permeation, nanomicelles are added to the gel matrix to prepare nanomicelle gels. The addition of penetration enhancers can help the nanomicelle gels to achieve an effective transdermal drug delivery effect (Pitorre et al., Citation2017).
Qindeel successfully prepared MTX self-assembled nanomicelles (MTX-NMs) by nanoprecipitation technique using MTX and polycaprolactone-polyethyleneglycol- polycaprolactone (PCL-PEG-PCL), then mixed MTX-NMs, ethylene oxide (EO) and carbopol to prepare the MTX-NMs gel. The external hydrophilic subunit structure of the triblock copolymer PCL-PEG-PCL can prevent MTX in the MTX-NMS gel from being recognized and captured by RES, which can prolong the drug action time and achieve 72 h sustained drug release. An in vitro permeation study showed that the drug permeation amounts of free MTX gel (carbomer was dissolved in water and MTX directly added) and MTX-NMs gel after 24 h were 59 μg/cm2 and 946 μg/cm2, respectively. The results of fluorescence microscopy showed that the fluorescence intensity of the MTX-NMs gel was strong, which illustrated that the MTX-NMs gel has high permeability in the skin. EO as a permeation enhancer, can decompose the lipid structure between keratinocytes and change the conformation of the intercellular protein domains, thereby promoting the penetration of MTX through the skin. The results of pharmacokinetics in mice showed that the plasma half-life of transdermal administration of MTX-NMs gel was 4.34 times higher than that of the oral free MTX group. In the CFA inflammation-induced model, obvious swelling and erythema was observed in the blank control group, and the swelling of the claw foot could be significantly reduced in the MTX-NMs gel group. The proinflammatory cytokines in the plasma of inflammation model mice were monitored, the results indicated that the release of inflammatory cytokines (TNF-α, IL-1 and IL-6) in the MTX-NMS gel-treated group was significantly reduced compared with that in those free MTX gel group (Qindeel et al., Citation2020).
Sanaplastic nano-vesicle gel
Sanaplastic nano-vesicles are formed by edge activators arranged in a bilayer vesicle membrane composed of surfactant molecules. Edge activators can lower the interfacial tension of the bilayer of vesicles, so sanaplastic nano-vesicles have good deformability. Sanaplastic nano-vesicle gels are prepared by adding drug-loaded sanaplastic nano-vesicles to the gel matrix, and then the bioavailabilit and penetration are promoted (Farghaly et al., Citation2017).
Alaaeldin firstly used Brij (aedge activators) to prepare celecoxib loaded sanaplastic nano-vesicles (SP) by modified injection method, and then added SP into carbopol solution to obtain SP gel. The edge activator in the SP-gel can increase the elasticity and deformability of vesicles, and the surfactant can reduce the barrier effect of the lipid bilayer in skin cells. The results of in vitro skin penetration showed that the percentages of celecoxib permeation of the SP-gel and the celecoxib suspension was 85.2% ± 3.4% and 12% ± 4.2%, respectively. In arthritic mice induced by CFA, the SP-gel can significantly reduce inflammation and edema, decrease the edema rate by 73.45% ± 2.6% after 7 days, and can effectively down-regulate the relative expression of inflammatory factor markers TNF-α, NF-κB, and COX-2 (Alaaeldin et al., Citation2021).
Ethosomal gel
Ethosomes are a bilayer structure with loose arrangement and good fluidity, which is prepared by adding ethanol or dibasic low-chain alcohols to the liposome phospholipid bilayer on the basis of traditional liposomes. Ethosomes can interfere with the lipid matrix of the cell membrane, fuze with the lipid matrix to activate the stratum corneum, and then promote the penetration of drugs into the skin. Ethosomal gel is obtained by adding ethosomes into the gel, endowing the ethosomes with ideal bio-adhesion and spreadability, which is more conducive to transdermal drug delivery (Elnaggar et al., Citation2014).
Heba prepared dapoxetine (DH) loaded transethosome nanovesicles (DH-TENVs) by the injection ultrasonic method, and then adds it to carbomer to obtain DH-TENVs gel. An in vitro drug release study showed that the release of the DH free aqueous solution for 3 h was 95%, while that of DH-TENVs gel after 8 h was 61.69%, and that the DH-TENVs gel has good sustained drug release properties. The results of histopathological analysis between DH-TENVs gel treated skin and untreated skin were indistinguishable, indicating that the DH-TENVs gel is safe and nonirritant. The PC of the DH-TENVs gel contacts and fuses with the lipid layer of the skin, and ethanol interacts with the polar head groups of stratum corneum lipids. These effects can increase the amount of DH permeating through the skin and effectively increase the bioavailability of DH. The pharmacokinetic study of rats showed that the bioavailability of the DH-TENVs gel was 2.42, 4.16 times higher than that of the DH oral group and the DH gel group (DH was added to Carbomer971 aqueous solution, stirred and dissolved). The in vivo analysis of a rat RA model induced by CFA showed that the DH-TENVs gel had a significant anti-RA effect, which can reduce inflammation and tissue lesions by inhibiting expression of the RA specific marker anti-cyclophosphamide antibody (anti-CCP), cartilage destruction marker cartilage oligomeric matrix protein (COMP), and inflammation marker IL-6 (Salem et al., Citation2020).
Transfersomal gel
The transfersomal gel is prepared by adding TFSs to the gel matrix, which can improve the stability of the TFSs and the permeability of drugs (Tawfeek et al., Citation2020).
Dudhipa obtained the aceclofenac(AF)-loaded transfersomes (AF-TS) by film hydration method using soybean lecithin, AF and the edge activator span 80, and AF-TS converted into AF-loaded transfersomal gel (AF-TG) by the addition of Carbopol 934. In vitro drug release studies within 24 h showed that the release rate of AF-TS was 99.1% ± 2.7% and AF-TG was 83.5% ± 3.6%, because the gel matrix in AF-TG allows the drug to be released slowly. In in vitro permeation experiments on rats for 24 h, the AF permeation rates of AF-TG and commercially available Haifenac gel were 71% ± 2.8% and 47.4% ± 1.9%, respectively, which indicated that AF-TG combined with the advantages of transfersomes and gel, showing higher drug penetration through the skin (Dudhipala et al., Citation2020). Sana prepared the curcumin transfersomal gel (Cur-TF) by thin-film hydration method, and then added Cur-TF to carbomer 934 to construct the Cur-TF gel. The in vitro drug release curve showed that when the pH = 5.5 and pH = 7.4, the cur release rate of the Cur-TF gel after 48 h was 66.1% ± 1.8% and 20.2% ± 1.9% respectively. The result proved that the release rate of the Cur-TF gel at pH = 5.5 (inflamed joint tissue pH) was higher than that at pH 7.4 (blood), and the Cur-TF gel had a good effect to target inflamed joints. An in vitro permeability study showed that the cumulative permeation amount of the Cur-TF gel in 24 h was 134.8 ± 6.9 μg/cm2, which was higher than that of curcumin used alone (47.06.6 μg/cm2), indicating that the Cur-TF gel obviously improved the poor permeability of Cur. In the model of inflammation induced by CFA in rats, histopathological analysis showed that the CUR-TF gel treatment group could significantly reduce bone erosion and tissue inflammation compared with the Cur-gel group (prepared by directly adding Cur into Carbomer 934) and the inflammation-induced non-treatment group. The results of immunohistochemistry showed that the Cur-TF gel can inhibit the activation of NF-κB, thus preventing the occurrence of subsequent inflammation. From results of enzyme (linked immunosorbent assay) ELISA showed, the Cur-TF gel could effectively reduce expression of inflammatory cytokines TNF- α and IL-6 (Sana et al., Citation2021).
Nanoparticles gel
Nanoparticles (NPs) are particles with a size of 1–1000 nm. The drug-loaded behavior of nanoparticles can be adjusted by adjusting the nanoparticles type, size, and surface charge (Nair et al., Citation2019). Nanoparticles gel, which is beneficial to the transdermal delivery of nanoparticles and improvement of the bioavailability of drugs, can be obtained by combining the drug-loaded nanoparticles with the gel matrix (Jana et al., Citation2014).
Nagai prepared indomethacin (IMC)-loaded nanoparticles (N-IMC) by the bead mill method, then N-IMC was dispersed into carbopol with or without l-menthol to prepare N-IMC/MT gel or N-IMC gel. An in vitro permeation experiment of rat skin showed that the AUC0-24h and penetration rate of the N-IMC/MT gel were 2.8 times and 3.8 times higher than that of the N-IMC gel, respectively. The addition of MT can enlarge the intercellular space in the stratum corneum and promote the permeability of IMC. In addition, the drug permeability of the N-IMC/MT gel and N-IMC gel at 4 °C was lower than that at 37 °C, indicating that energy-dependent endocytosis is the skin permeation mechanism of drug-loaded nanoparticles (Nagai et al., Citation2019).
Gul firstly prepared ketoprofen and chondroitin sulfate (CS) co-loaded nanoparticles by ion coagulation method, then the nanoparticles and argan oil were added into HPMC gel matrix to form the nanoparticles gel (emulgel). An in vitro permeation test of mouse skin showed that the drug permeation of drug co-loaded nanoparticles and emulgel increased by 1.92 times and 2.49 times that of the commercially available Fastum gel. The reason of emulgel promoting penetration is as follows: CHS can hydrate the stratum corneum, further reversibly changing the conformation of keratin to form a channel to promote the penetration of ketoprofen, and the oleic and linoleic components of argan oil can interact with the lipids of the stratum corneum to promote the permeation by the pericellular permeation pathway. Among the prepared emulgels, ketoprofen, CS, and argan oil have a synergistic effect in treating RA. Ketoprofen is a typical non-steroidal anti-inflammatory drug, CS can down-regulate expression of inflammatory factors and promote cartilage protein synthesis, and essential oils have natural anti-inflammatory activity (Gul et al., Citation2018).
Patch
Patch refers to a flake preparation made of drugs and suitable materials that can be pasted on the skin for topical administration. Transdermal patches are usually composed of four layers, the impermeable backing layer, drug containing matrix layer, adhesive layer, and peelable anti adhesion protective layer (Pastore et al., Citation2015). The patch can slowly release the drug, allowing it to penetrate the skin and maintain a constant plasma concentration (Nalamachu & Gudin, Citation2020). Optimizing the patch preparation technology and adjusting the structural components of the auxiliary materials can improve the shape and function of the patch and facilitate the effective transdermal delivery of drugs.
Transdermal patch directly loaded with drug
Kandil prepared galanthamine (GH) patch by solvent casting method, which pour viscous solution of hydroxypropyl methylcellulose 4000, plasticizer propylene glycol (PG), dimethyl sulfoxide (DMSO), GH into the backing layer. An in vitro penetration experiments of rat skin showed that the drug permeation rates of the GH aqueous solution and GH patch were 32.58% and 89.97% respectively. Eudragit RS in the patch can promote skin hydration, DMSO and limonene can interact with lipids of the stratum corneum to increase the disorder of their arrangement, and thus increasing the permeability of GH. In a rat inflammatory model induced by CFA, the results of ELISA showed that the GH patch can reduce the number of C-reactive protein (biomarker of RA), and inhibit expression of inflammatory cytokines TNF-α and IL-1. The GH patch can continuously penetrate for 48 h, which solved the problem of frequent medication caused by oral GH (twice daily), and significantly reduce the side effects of diarrhea and weight loss caused by oral GH. In summary, the GH patch is a safe and efficient transdermal dosage form for the treatment of RA (Kandil et al., Citation2020). Vaidya prepared MTX patch by solvent casting method. The prepared MTX patch has high drug loading (87.04% ± 0.0564%), strong strength, and elasticity. The physical penetration enhancement technology (sonophoresis, electroporation, cold laser) can be applied to promote the release of MTX. Sonophoresis can create a gaseous cavity in the stratum corneum, which can promote drug passage of the skin. Electroporation technology can create water holes in the stratum corneum to promote the passage of hydrophilic moieties. Cold laser technology can ablate part of the stratum corneum, resulting in reducing the barrier ability of the stratum corneum. In vitro drug release studies have shown that the order of the cumulative drug release rate at 24 h is sonophoresis (73%) > electroporation (55%) > cold laser dialysis (54%)> MTX patch without penetration enhancement technology (45%). In the CFA-induced inflammation model of rats, the reduction rate of paw swelling diameter and the degree of pain reduction of the ultrasound-permeated MTX patch group were higher than those of the nonphysical permeable MTX patch group on day 5 and day 21 (Vaidya & Shende, Citation2020).
Liu developed a compound transdermal patch containing ketoprofen (KTP) and tefnomide (TEF) through solvent volatilization, and azone (AZ) was added as penetration enhancer. The in vitro drug release curve showed that the KTP release of the KTP-TE-AZ patch was 67% after 24 h, while the KTP release of the KTP-TEF patch (without adding AZ, the rest is the same as the preparation of the KTP-TEF-AZ patch) was only 47%, indicating the KTP-TEF-AZ patch can increase the release of KTP. AZ can strengthen the hydrogen bonding between KTP and TEF, which improves the ability of the drug in a compound patch to pass through the skin. In an in vitro permeability test of rabbit skin, the penetration of KTP at 24 h in the KTP-TEF-AZ patch was 502.92 ± 24.0 μg/cm2 and the TEF penetration was 594.2 ± 46.8 μg/cm2, which is 1.2 times and 1.9 times of the corresponding drug penetration of the KTP-AZ patch (only the drug KTP was added, the rest is the same as the preparation of the KTP-TEF-AZ patch) and the TEF-AZ patch (only the drug TEF was added, the rest is the same as the preparation of the KTP-TEF-AZ patch). The results of the CFA-induced anti-inflammatory test and acetic acid-induced writhing analgesia test in mice showed that the TEF-KTP-AZ patch can effectively reduce foot swelling and inhibit pain (Liu et al., Citation2019).
Nanoparticle transdermal patch
Nanoparticle transdermal patches are prepared by encapsulating drugs into nanoparticles, and then loading the drug-loaded nanoparticles on the patch.
Khan prepared flurbiprofen (FB) loaded pH responsive nanoparticles by nanoprecipitation method, then constructed FB-loaded nanoparticles patches (FB NP-based patch) by the solvent evaporation method. The in vitro drug release experiment showed that the FB NP-based patch released 92% of the drug at pH = 7.4 after 72 h; however, the drug release amount was less than 10% for the same time at pH = 5.5. In vivo studies of the acute carrageenan-induced inflammation model and chronic CFA-induced inflammation model in mice showed that the FB NP-based patch significantly reduced the paw volume of swollen paws and increased the response to thermal and mechanical pain compared with the commercial Froben ®gel group (Khan et al., Citation2020).
Hydrogel patch
Huang fabricated ultrasound responsive microcapsules-loaded hydrogel patches ((DS@PEG-PLGA) @ PEG) by embedding DS-loaded microcapsules into a hydrogel patch. The prepared patch has good self-adhesiveness, which can attach to pigskin to twist and bend without peeling. In vitro cytotoxicity experiments showed that the survival rate of MC3T3-E1 cells detected by the CCK-8 method was above 90%, indicating that the (DS@PEG-PLGA)@PEG hydrogel patch is highly safe. An in vitro drug release study revealed that the microcapsules reached the release plateau at 48 h, and the (DS@PEG-PLGA)@PEG hydrogel patch can continue to release the drug for 96 h, indicating that the prepared hydrogel patch has a DS sustained release effect. The prepared hydrogel patch has DS release rate of 90% after 8 min of continuous ultrasound action, and the release rate of the patch without ultrasound treatment is less than 20%, indicating that the stretching vibrations caused by the ultrasound action induce microcapsule rupture, which can promote the release of DS. An in vitro penetration study of rat skin showed that the cumulative release of the patch after ultrasound action reached 47% at 10 min, and DS was not detected without ultrasound at the same time. These results highlighted that ultrasound destroys the lipid cell structure of the stratum corneum, thus promoting the skin penetration of the drug. This ultrasound response type (DS@PEG-PLGA)@PEG hydrogel patch can not only trigger the release of DS under ultrasound, but also increases the penetration of DS, which is an efficient and safe transdermal delivery formulation (Huang et al., Citation2019).
Microneedles (MNs)
MNs are usually a painless and less invasive drug delivery form with a size of less than 1000 μm, which can facilitate self-administration and improve drug delivery compliance. MNs can cause drugs to directly penetrate the stratum corneum, which helps the drugs to be directly absorbed by the capillaries and lymphoid tissues in the dermis. MNs are an effective way to deliver macromolecular drugs (Ma & Wu, Citation2017). MNs include solid MNs, coated MNs, hollow MNs, hydrogel MNs, and dissolving MNs. Due to the outstanding advantages of hydrogel MNs and dissolving MNs, the related research has gradually become a hot spot in the field of MNs (Hong et al., Citation2014).
Hydrogel microneedles (hydrogel MNs)
Hydrogel MNs are prepared by crosslinking and swelling of the polymer matrix. Hydrogel MNs are inserted into the skin and pierce the stratum corneum, then the swellable polymer of hydrogel MNs absorbs body fluids to form a hydrogel tube, which can transport the drug in the hydrogel microneedles to the dermis and achieve drug diffusion (McAlister et al., Citation2021). Hydrogel MNs safety has been evaluated by Rehan. The repeated application of hydrogel microneedles on the skin of 11 healthy volunteers in a clinical trial showed that no prolonged skin irritation or disruption of skin barrier function during the study period (5 days). The favorable safety of microneedle is beneficial to ensure its practical application (Al-Kasasbeh et al., Citation2020).
DTA is a DEK-targeting nucleic acid aptamer for the treatment of RA. DTA6 modified from DTA can improve the stability of the drug. Cao developed a hyaluronic acid based hydrogel microneedles to deliver DTA, which prepared by micro-molding method. DTA6-HMN was applied to the leg of the CFA-induced inflammation model mice. In an in vivo anti-arthritis study, CT in vivo imaging results of small animals (MicroCT) showed that compared with the inflammatory model group and the intravenous injection DTA6 group, the ratio of bone surface area to bone volume (BS/BV) in the DTA6-HMN group was lower, indicating that DTA6-HMN made the bone surface smoother. The results of ELISA kit showed that the DTA6-HMN group was more effective than the inflammatory model group in reducing the concentration of TNF-α and IL-6 in the serum, thus inhibiting the secretion of inflammatory factors. The results of hematoxylin and eosin(H&E)- staining sections revealed that the inflammatory model group of mice and the DTA6 treatment group had severe cartilage destruction and immune cell infiltration, while the DTA6-HMN group had normal joint structure and mild cartilage erosion () (Cao et al., Citation2021).
Figure 6. DTA6-HMN hydrogel microneedle transdermal treatment of RA. Copyright 2021, American Chemical Society (Cao et al., Citation2021).
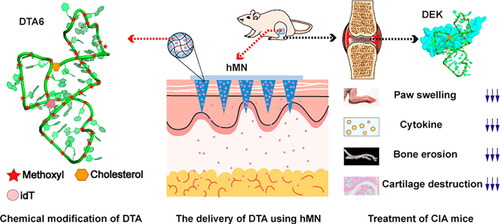
Tekko constructed methotrexate reservoir hydrogel microneedle (MTX-RV-HFMN), which combined patch-like reservoir of MTX (MTX-RV) with hydrogel microneedle array (HFMN). HFMN can absorb skin interstitial fluid after piercing the skin to swell hydrogel conduits, and the MTX in the reservoir continuously diffuses to the dermis through the swelled hydrogel conduits. The MTX can be steadily released for 24 hours and the steady-state flux of it can reach 506.8 + 136.9 µg/cm2/h. A pharmacokinetic study in rats showed that the highest plasma concentration (Cmax) (57.4 ± 20.0 nM) of the oral methotrexate group can be reached at 1 h and the MTX-RV-HFMN group reached Cmax (35.1 ± 5.1 nM) at 24 h, indicating that MTX-RV-HFMN has a sustained-release effect and significantly improves the shortcomings of rapid oral MTX release. The area under the curve of MTX-RV-HFMN (AUC0-48h, 685.9 ± 157.9 nM.h) was 1.2 times that of the oral group, illustrating that MTX-RV-HFMN is an efficient transdermal drug delivery system. The strong mechanical properties of HFMN make the hydrogel microneedles could be completely removed from skin after 24 h use without tip residue and irritation at the application site, which exhibit its usage security (Tekko et al., Citation2020).
Dissolving microneedles (dissolving MNs)
Dissolving MNs are prepared using the solvent casting method by dispersing or dissolving drugs in water-soluble matrix materials or biodegradable materials (Yang et al., Citation2021). When the microneedle acts on the skin, the needle gradually dissolves after absorbing the skin interstitial liquid, and then the drug is directly released from the needle into the deep layer of the skin to exert a therapeutic effect. The dissolving microneedle has high safety in usage, and no medical waste is generated after use (He et al., Citation2021). The clinical trial showed that the dissolving microneedle would not trigger adverse reaction related to the treatment, which approve their good acceptance and tolerance (Rouphael et al., Citation2017).
Sinomenine (SIN), an alkaloid extracted the from plant Sinomenium acutum, can decrease the secretion of TNF-α, IL-6, and IL-1 and reduce inflammation (Liu et al., Citation2018). Shu fabricated SIN loaded dissolved microneedles (SIN-MN) using SIN, PVP and chondroitin sulfate (CS) as raw materials by casting method. After the matrix materials PVP and CS of SIN-MN absorb tissue fluid, the tip of MN is gradually dissolved and the drug is released. The drug penetration thickness can subcutaneously reach 200 μm. The results of in vitro permeation experiments demonstrated that the cumulative permeation and the permeation rates of SIN-MN were 5.31 times and 5.06 times that of sinomenine gel (SIN-G) respectively. Transdermal pharmacokinetic studies in mice showed that the area under the curve (AUC)0-800 min of the skin and blood of the SIN-MN group were 1.43 times and 1.63 times higher than that of the SIN-G group, indicating that SIN-MN has the ability to enhance the transdermal absorption of the drug (Shu et al., Citation2020).
Nanostructured lipid carriers (NLCs)
NLCs are unstructured solid lipid matrix composed of an oil phase mixture of solid lipid/liquid lipid and an aqueous phase mixture containing surfactants. The stability of NLCs can be increased by adjusting the ratio of the solid lipid/liquid lipid and the content of the surfactants. Compared with the ‘brick arrangement’ structure of solid lipid nanoparticles (SLNs), the unstructured matrix structure of NLCs can load more drugs (), and the solid lipid core of NLCs can wrap more lipophilic drugs, and protect them from degradation to improve the biological activity of drugs (Beloqui et al., Citation2016).
Gu prepared triptolide-loaded nanostructured lipid carriers (TPL-NLCs) by emulsification technology using triptolide (TPL), Docosanoic acid, 1, 2, 3- propanetriyl ester (Compritol 888 ATO, solid lipid), propylene glycol caprylate (Capryol 90, liquid lipid), and surfactants as material. The liquid lipid (Capryol 90) in TPL-NLCs was inserted into the two-layer structure of the solid oil lipid (Compritol 888 ATO) to form a three-layer crystal structure, which can improve the drug loading capacity. The encapsulation efficiency of TPL-NLCs can reach 97.15% ± 9.46 and the drug loading can reach 10.35% ± 1.12. In vitro penetration experiments of rat skin showed that the cumulative penetration quality of TPL-NLCs within 12 h was 73.51 ± 17.29 μg/cm2, while the TPL nanoemulsion was 23.94 ± 0.78 μg/cm2, so the penetration of TPL in TPL-NLCs was significantly improved. The protective effect of the liposome core and the outer aqueous phase of TPL-NLCs gives the drug sustained-release characteristics. In the rat arthritis model induced by CFA, the joint swelling in the TPL-NLCs group was more obviously reduced than that in the untreated group. Compared with diclofenac sodium gel that can only reduce TNF- α inflammatory factors, TPL-NLCs can significantly inhibit the expression of TNF- α, IL-1, and IL-6 inflammatory factors and reduce synovial hyperplasia and synovial fibrosis (Gu et al., Citation2019).
Transfersomes (TFSs)
TFSs are edge activators modified liposome (Balata et al., Citation2020). Edge activators confer higher elasticity to liposome vesicles by interfering with liposome bilayer membranes, resulting in good deformability of TFSs. Because TFSs can squeeze through small holes in the stratum corneum that are less than one-tenth of its own diameter, they have better skin permeability, thereby increasing the concentration of active drugs (Fernandez-Garcia et al., Citation2020).
Zheng prepare the mixed monoterpenes edge-activated PEGylated transfersomes (MMPTs) by injecting the organic phase into the aqueous phase. The results of confocal laser scanning microscopy (CLSM) showed that after 2 h of applying MMPTS to the skin, weak and continuous red fluorescence can be detected in the stratum corneum, and more obvious red fluorescence can be detected in the dermis or deep skin layers after 12 h. However, after using conventional liposomes (LPSs) for 2 h, almost no fluorescence was observed in the skin, and only bright red fluorescence was observed in the stratum corneum after 12 h. CLSM results proved that LPSs can only penetrate the stratum corneum, while MMPTS has stronger permeability. Limonene and citral as edge activators increase the elasticity and fluidity of MMPTS, and make up for the rigidity defect of the lipid bilayer of LPSs. Applying MMPTS to rabbit knee joint arthritis, in vivo pharmacokinetic studies showed that the steady state concentration (Css, 74.5 ± 9.65 ng/mL) and AUC0- 600 min of SIN of MMPTS in the articular cavity were 2.1 times and 2.5 times those of LPSs, indicating that MMPTS can deliver SIN to deep skin. Moreover, the Css and AUC0- 600 min of SIN in MMPTs applied to the knee joint cavity of rabbits were 12.9 and 14.4 times higher than that in the blood respectively, which indicated that MMPTS possesses the ability of targeted drug delivery and can alleviate systemic side effects (Zheng et al., Citation2020).
Lyotropic liquid crystal (LLC)
LLC is a self-assembled intermediate between liquids and solids with a two-dimensional or three-dimensional structure formed by amphiphilic lipids in solvents, which has a structure with good order and symmetry (Silvestrini et al., Citation2020). The internal structures of LLCs can be classified into lamellar, cubic, hexagonal, sponge phase and so on. Cubic phase (V2) and hexagonal phase (H2) are more commonly used for transdermal drug delivery because of their similar structures of cell membranes. They have specific biological adhesion and skin retention properties, which can cause LLC loaded drugs to easily penetrate the stratum corneum. The aqueous and lipid domain of LLC enable LLC to be used not only as a carrier for hydrophilic drugs and hydrophobic drugs, but also as a carrier for drug molecules such as proteins, nucleic acids, and polypeptides (Liu et al., Citation2021).
Shan obtained triptolide-loaded cubic liquid crystals (V2 liquid crystals) and triptolide-loaded hexagonal liquid crystals (H2 liquid crystals) by self-emulsification method using amphiphilic lipid material phytantriol and triptolide. In vitro permeation experiments showed that the cumulative permeability of V2 liquid crystals and H2 liquid crystals after 48 h was 1.65 times and 1.76 times higher than that of the TP gel, which was attributed to the similar lipid structure of liquid crystals with the stratum corneum. The V2 liquid crystals, H2 liquid crystals, and TP gels were smeared on the abdomen of rats for the skin pharmacokinetics study, and the AUC0-700 min of the V2 liquid crystals and H2 liquid crystals groups were 1.89 times and 1.34 times higher than that the TP gel group. The result indicated that liquid crystals can significantly improve the bioavailability of TP. The pharmacokinetic curves of rat plasma illustrated that both TP residence time (MRT) and peak time (Tmax) of V2 and H2 liquid crystals groups were longer than the TP gel, indicating that lyotropic liquid crystals had a drug sustained release effect. The curves also showed that the drug bioavailability of the H2 liquid crystal group was stronger, and V2 liquid crystals had stronger sustained release, which was attributed to their different internal structure and viscosity. The Cmax of V2 and H2 liquid crystals in the pharmacokinetic curve of skin was significantly higher than the curve of plasma, which illustrated that the transdermal treatment of lyotropic liquid crystal has strong targeting ability, thereby reducing side effects by decreasing the amount of drug reaching the system. In the rat arthritis mode induced by CFA, V2 and H2 liquid crystals could more obviously inhibit expression of TNF-α and IL-1 than the TP gel group, and thus V2 and H2 liquid crystals can effectively alleviate inflammatory phenomena such as synovial hyperplasia, neutrophil infiltration, and synovial lining cell hyperplasia (Shan et al., Citation2019). Wan prepared TCA loaded cubic lyotropic liquid crystals (TCA loaded V2 liquid crystals) and TCA loaded hexagonal lyotropic liquid crystals (TCA loaded H2 liquid crystals) by the self-emulsification method. In vitro transdermal metabolism and permeation experiments, the cumulative infiltration capacity (QN) and penetration rate (Js) of TCA loaded V2 liquid crystals were 1.26 and 1.31 times higher than that of TCA gel, and the QN and Js of TCA loaded H2 liquid crystals were 1.80 times and 1.83 times of that of the TCA gel respectively, indicating that liquid crystalline had better skin permeability than gels. With the different structure of H2 and V2, TCA loaded H2 liquid crystals had better skin permeability. Pharmacokinetic studies in rats showed that the AUC0-50 h of TCA loaded V2 and H2 liquid crystals increased by 1.53 and 1.92 times compared with the TCA gel group, and the MRT was also significantly prolonged by V2 and H2 liquid crystals, indicating that crystalline liquid improve the bioavailability and drug release. The experimental results of rat paw edema induced by CFA showed that compared with the control group injected with normal saline, TCA loaded H2 liquid crystals could effectively reduce foot swelling, improve synovial hyperplasia, and inflammatory cell infiltration, and inhibit expression of IL-1 and TNF-α (). The pharmacodynamic studies showed that TCA loaded H2 liquid crystals has similar anti-inflammatory effects to the commercially available product Voltaren® emulgel®, which support the potential application of TCA liquid crystals for transdermal delivery (Wan et al., Citation2018).
Figure 8. In vitro and in vivo skin permeability experiments of TCA liquid crystals and preliminary pharmacodynamic studies in rats show liquid crystal was considered as an effective transdermal delivery system for TCA. Copyright 2018, Elsevier (Wan et al., Citation2018).
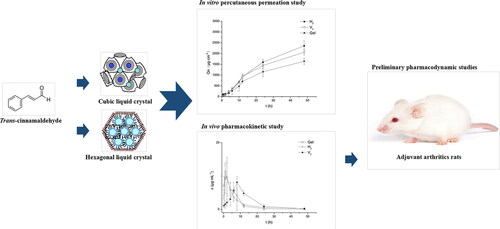
Drug-loaded electrospun nanofibers
Drug-loaded electrospun nanofibers are prepared by electrospinning drug polymer solution, and continuously stretching and volatilizing the solvent by electrostatic repulsion of jet surface charge (Goyal et al., Citation2016). Drug-loaded electrospun nanofibers have high specific surface areas and large drug loading. The drug release performance and penetration through the skin can be improved through adjusting the fiber type, structure, and porosity (Goh et al., Citation2013).
Xiang designed a novel ibuprofen self-nanoemulsifying electrospun fibrous membrane (IBU-SNE-FM), which was fabricated by electrospinning self-nanoemulsifying drug delivery system (SNEDDS). When IBU-SNE-FM contacts body fluids, various components of SNEDDS will self-assemble into lamellar liquid crystals due to hydrogen bonding and hydrophobic forces, and further spontaneously form nanoemulsions (NE), which can facilitate the transdermal penetration of the drug (). In vitro permeation experiments of rat skin showed that the drug permeability coefficient of IBU-SNE-FM was 1.79 and 1.67 times higher than that of IBU’s phosphate buffer, and the IBM nanofibers (IBU was directly dissolved in PLLA ethanol solution for electrospinning) after 4 h. In the acute inflammation model in rats induced with CFA, the IBU-SNE-FM group significantly reduced the inflammation infiltration and the swelling thickness of rat paws, which can effectively exert the anti-inflammatory effect (Xiang et al., Citation2019).
Figure 9. IBU-SNE-FM transdermal delivery process. Copyright 2019, American Chemical Society (Xiang et al., Citation2019).

Outlook
RA is a chronic disease triggered by the imbalance of the autoimmune system. The immune imbalance releases inflammatory factors that cause damage to articular cartilage and bone erosion. TDDS is an effective drug delivery system that can overcome the shortcomings of traditional oral administration and injection administration. This paper reviewed the TDDS of drugs for the treatment of RA by regulating inflammatory factors. TDDS can not only have sustained drug release and improve drug solubility, but also specifically achieve targeted drug delivery, reduce systemic adverse reactions, so as to improve the bioavailability of therapeutic drugs, and effectively inhibit TNF- α, IL-1, and IL-6 inflammatory factors. The biological safety of TDDS of RA should be strictly evaluated in clinical application, but existing researches have rarely evaluated the biological safety of nanocarriers, excipients, and composite materials. The toxic reactions caused by TDDS after short-term and long-term contact with damaged skin or intact skin, and the comprehensive evaluation of the metabolic pathway, metabolites, and cytotoxicity of the active groups in TDDS after entering the skin are challenges faced by RA transdermal drug delivery. In addition, the effectiveness evaluation of TDDS in RA is another focus to realize its clinical application. Although RA animal models could be established to test the efficacy of transdermal agents, the pathological characteristics of RA in humans are much more complex than those in animal models. Therefore, more clinical data are needed to test its validity in the future.
Currently, the design of transfersomes, transfersomal gel, lyotropic liquid crystals, and other dosage forms can enhance the targeting of TDDS, so that TDDS shows excellent efficacy for RA treatment. The E-selectin, vasoactive intestinal peptide, alphaV, and beta3 integrins are highly expressed in inflammatory cells, when TDDS targeted them, new RA-targeted transdermal preparations would be developed. In addition, the emergence of new penetration enhancement technologies (focused ultrasound technology, smart electrical responsive microneedles, and cold atmospheric plasma technology) and development of alternative methods such as electrophoresis or needle free injection also provide broad opportunities for the construction of sustained and efficient transdermal drug delivery formulations for RA.
Disclosure statement
The authors report no declarations of interest.
Additional information
Funding
References
- Abbasi M, Mousavi MJ, Jamalzehi S, et al. (2019). Strategies toward rheumatoid arthritis therapy; the old and the new. J Cell Physiol 234:10018–31.
- Alaaeldin E, Abou-Taleb HA, Mohamad SA, et al. (2021). Topical nano-vesicular spanlastics of celecoxib: enhanced anti-inflammatory effect and down-regulation of tnf-alpha, nf-small ka, cyrillicb and cox-2 in complete freund’s adjuvant-induced arthritis model in rats. Int J Nanomed 16:133–45.
- Alamri RD, Elmeligy MA, Albalawi GA, et al. (2021). Leflunomide an immunomodulator with antineoplastic and antiviral potentials but drug-induced liver injury: a comprehensive review. Int Immunopharmacol 93:107398.
- Al-Kasasbeh R, Brady AJ, Courtenay AJ, et al. (2020). Evaluation of the clinical impact of repeat application of hydrogel-forming microneedle array patches. Drug Deliv Transl Res 10:690–705.
- Alkilani AZ, McCrudden MT, Donnelly RF. (2015). Transdermal drug delivery: innovative pharmaceutical developments based on disruption of the barrier properties of the stratum corneum. Pharmaceutics 7:438–70.
- Andrews SN, Jeong E, Prausnitz MR. (2013). Transdermal delivery of molecules is limited by full epidermis, not just stratum corneum. Pharm Res 30:1099–109.
- Angelini J, Talotta R, Roncato R, et al. (2020). Jak-inhibitors for the treatment of rheumatoid arthritis: a focus on the present and an outlook on the future. Biomolecules 10:1002.
- Arunprasert K, Pornpitchanarong C, Rojanarata T, et al. (2021). Development and evaluation of novel water-based drug-in-adhesive patches for the transdermal delivery of ketoprofen. Pharmaceutics 13:789.
- Aziz K, Ahmad N, Kohli K. (2017). Enhanced anti arthritic potential of tacrolimus by transdermal nanocarrier system. J Nanosci Nanotechnol 17:4573–83.
- Bacelo E, Alves da Silva M, Cunha C, et al. (2019). Biofunctional nanofibrous substrate for local tnf-capturing as a strategy to control inflammation in arthritic joints. Nanomaterials 9:567.
- Badri W, Miladi K, Nazari QA, et al. (2016). Encapsulation of nsaids for inflammation management: overview, progress, challenges and prospects. Int J Pharm 515:757–73.
- Balata GF, Faisal MM, Elghamry HA, et al. (2020). Preparation and characterization of ivabradine hcl transfersomes for enhanced transdermal delivery. J Drug Deliv Sci Technol 60:101921.
- Bashir M, Ahmad J, Asif M, et al. (2021). Nanoemulgel, an innovative carrier for diflunisal topical delivery with profound anti-inflammatory effect: in vitro and in vivo evaluation. Int J Nanomed 16:1457–72.
- Bazan NG, Colangelo V, Lukiw WJ. (2002). Prostaglandins and other lipid mediators in Alzheimer’s disease. Prostaglandins Other Lipid Mediat 68-69:197–210.
- Beloqui A, Solinis MA, Rodriguez-Gascon A, et al. (2016). Nanostructured lipid carriers: promising drug delivery systems for future clinics. Nanomedicine 12:143–61.
- Blair HA, Deeks ED. (2017). Abatacept: a review in rheumatoid arthritis. Drugs 77:1221–33.
- Brzustewicz E, Bryl E. (2015). The role of cytokines in the pathogenesis of rheumatoid arthritis-practical and potential application of cytokines as biomarkers and targets of personalized therapy. Cytokine 76:527–36.
- Cao J, Su J, An M, et al. (2021). Novel dek-targeting aptamer delivered by a hydrogel microneedle attenuates collagen-induced arthritis. Mol Pharm 18:305–16.
- Cao J, Zhang N, Wang Z, et al. (2019). Microneedle-assisted transdermal delivery of etanercept for rheumatoid arthritis treatment. Pharmaceutics 11:235.
- Cutolo M, Paolino S, Gotelli E. (2021). Glucocorticoids in rheumatoid arthritis still on first line: the reasons. Exp Rev Clin Immunol 17:417–20.
- Cutolo M, Sulli A, Pizzorni C, et al. (2001). Anti-inflammatory mechanisms of methotrexate in rheumatoid arthritis. Ann Rheum Dis 60:729–35.
- Dąbrowska AK, Spano F, Derler S, et al. (2018). The relationship between skin function, barrier properties, and body-dependent factors. Skin Res Technol 24:165–74.
- Deng C, Zhang Q, He P, et al. (2021). Targeted apoptosis of macrophages and osteoclasts in arthritic joints is effective against advanced inflammatory arthritis. Nat Commun 12:2174.
- Dhillon S. (2017). Tofacitinib: a review in rheumatoid arthritis. Drugs 77:1987–2001.
- Dudhipala N, Phasha Mohammed R, Adel Ali Youssef A, et al. (2020). Effect of lipid and edge activator concentration on development of aceclofenac-loaded transfersomes gel for transdermal application: in vitro and ex vivo skin permeation. Drug Dev Ind Pharm 46:1334–44.
- Ehrchen JM, Roth J, Barczyk-Kahlert K. (2019). More than suppression: glucocorticoid action on monocytes and macrophages. Front Immunol 10:2028.
- Elnaggar YS, El-Refaie WM, El-Massik MA, et al. (2014). Lecithin-based nanostructured gels for skin delivery: an update on state of art and recent applications. J Control Release 180:10–24.
- Farghaly DA, Aboelwafa AA, Hamza MY, et al. (2017). Topical delivery of fenoprofen calcium via elastic nano-vesicular spanlastics: optimization using experimental design and in vivo evaluation. AAPS PharmSciTech 18:2898–909.
- Fernandez-Garcia R, Lalatsa A, Statts L, et al. (2020). Transferosomes as nanocarriers for drugs across the skin: quality by design from lab to industrial scale. Int J Pharm 573:118817.
- Fraenkel L, Bathon JM, England BR, et al. (2021). 2021 american college of rheumatology guideline for the treatment of rheumatoid arthritis. Arthritis Rheumatol 73:1108–23.
- Garg NK, Singh B, Tyagi RK, et al. (2016). Effective transdermal delivery of methotrexate through nanostructured lipid carriers in an experimentally induced arthritis model. Colloids Surf B Biointerfaces 147:17–24.
- Goh Y-F, Shakir I, Hussain R. (2013). Electrospun fibers for tissue engineering, drug delivery, and wound dressing. J Mater Sci 48:3027–54.
- Gokhale JP, Mahajan HS, Surana SJ. (2019). Quercetin loaded nanoemulsion-based gel for rheumatoid arthritis: in vivo and in vitro studies. Biomed Pharmacother 112:108622.
- Gorantla S, Singhvi G, Rapalli VK, et al. (2020). Targeted drug-delivery systems in the treatment of rheumatoid arthritis: recent advancement and clinical status. Ther Deliv 11:269–84.
- Goyal R, Macri LK, Kaplan HM, et al. (2016). Nanoparticles and nanofibers for topical drug delivery. J Control Release 240:77–92.
- Gu Y, Tang X, Yang M, et al. (2019). Transdermal drug delivery of triptolide-loaded nanostructured lipid carriers: preparation, pharmacokinetic, and evaluation for rheumatoid arthritis. Int J Pharm 554:235–44.
- Gul R, Ahmed N, Ullah N, et al. (2018). Biodegradable ingredient-based emulgel loaded with ketoprofen nanoparticles. AAPS PharmSciTech 19:1869–81.
- Guo JT, Cao XQ, Wu LL, et al. (2019). Effect of il-1β on apoptosis of synovial cells in rheumatoid arthritis rats via the nf-κb pathway. Eur Rev Med Pharmacol Sci 23:10211–7.
- Guo Y-g, Pratap Singh A. (2019). Emerging strategies for enhancing buccal and sublingual administration of nutraceuticals and pharamaceuticals. J Drug Deliv Sci Technol 52:440–51.
- He J, Zhang Z, Zheng X, et al. (2021). Design and evaluation of dissolving microneedles for enhanced dermal delivery of propranolol hydrochloride. Pharmaceutics 13:579.
- Hong X, Wu Z, Chen L, et al. (2014). Hydrogel microneedle arrays for transdermal drug delivery. Nano-Micro Lett 6:191–9.
- Huang D, Sun M, Bu Y, et al. (2019). Microcapsule-embedded hydrogel patches for ultrasound responsive and enhanced transdermal delivery of diclofenac sodium. J Mater Chem B 7:2330–7.
- Iachina I, Antonescu IE, Dreier J, et al. (2019). The nanoscopic molecular pathway through human skin. Biochim Biophys Acta Gen Subj 1863:1226–33.
- Isabwe GAC, de Las Vecillas Sanchez L, Castells M. (2017). Management of adverse reactions to biologic agents. Allergy Asthma Proc 38:409–18.
- Ito S. (2018). Weekly subcutaneous injection of tocilizumab in patients with rheumatoid arthritis. Mod Rheumatol 28:17–9.
- Jain S, Tripathi S, Tripathi PK. (2021). Invasomes: potential vesicular systems for transdermal delivery of drug molecules. J Drug Delivery Sci Technol 61:102166.
- Jana S, Manna S, Nayak AK, et al. (2014). Carbopol gel containing chitosan-egg albumin nanoparticles for transdermal aceclofenac delivery. Colloids Surf B Biointerfaces 114:36–44.
- Jia XY, Chang Y, Sun XJ, et al. (2014). The role of prostaglandin e2 receptor signaling of dendritic cells in rheumatoid arthritis. Int Immunopharmacol 23:163–9.
- Jia Y, Liu Z, Wang C, et al. (2016). P-gp, mrp2 and oat1/oat3 mediate the drug-drug interaction between resveratrol and methotrexate. Toxicol Appl Pharmacol 306:27–35.
- Kalluri H, Banga AK. (2011). Transdermal delivery of proteins. AAPS PharmSciTech 12:431–41.
- Kandil LS, Hanafy AS, Abdelhady SA. (2020). Galantamine transdermal patch shows higher tolerability over oral galantamine in rheumatoid arthritis rat model. Drug Dev Ind Pharm 46:996–1004.
- Kathuria H, Nguyen DTP, Handral HK, et al. (2020). Proposome for transdermal delivery of tofacitinib. Int J Pharm 585:119558.
- Khan D, Qindeel M, Ahmed N, et al. (2020). Development of novel ph-sensitive nanoparticle-based transdermal patch for management of rheumatoid arthritis. Nanomedicine (Lond) 15:603–24.
- Kohler BM, Gunther J, Kaudewitz D, et al. (2019). Current therapeutic options in the treatment of rheumatoid arthritis. J Clin Med 8:938.
- Kotla NG, Chandrasekar B, Rooney P, et al. (2017). Biomimetic lipid-based nanosystems for enhanced dermal delivery of drugs and bioactive agents. ACS Biomater Sci Eng 3:1262–72.
- Larrañeta E, Lutton REM, Woolfson AD, et al. (2016). Microneedle arrays as transdermal and intradermal drug delivery systems: materials science, manufacture and commercial development. Mater Sci Eng R: Rep 104:1–32.
- Law ST, Taylor PC. (2019). Role of biological agents in treatment of rheumatoid arthritis. Pharmacol Res 150:104497.
- Liu J, Cheng R, Heimann K, et al. (2021). Temperature-sensitive lyotropic liquid crystals as systems for transdermal drug delivery. J Mol Liq 326:115310.
- Liu C, Guan Y, Tian Q, et al. (2019). Transdermal enhancement strategy of ketoprofen and teriflunomide: the effect of enhanced drug-drug intermolecular interaction by permeation enhancer on drug release of compound transdermal patch. Int J Pharm 572:118800.
- Liu W, Zhang Y, Zhu W, et al. (2018). Sinomenine inhibits the progression of rheumatoid arthritis by regulating the secretion of inflammatory cytokines and monocyte/macrophage subsets. Front Immunol 9:2228.
- Ma G, Wu C. (2017). Microneedle, bio-microneedle and bio-inspired microneedle: a review. J Control Release 251:11–23.
- McAlister E, Dutton B, Vora LK, et al. (2021). Directly compressed tablets: a novel drug-containing reservoir combined with hydrogel-forming microneedle arrays for transdermal drug delivery. Adv Healthc Mater 10:e2001256.
- McInnes IB, Schett G. (2017). Pathogenetic insights from the treatment of rheumatoid arthritis. Lancet 389:2328–37.
- Miranda GM, Santos V, Bessa JR, et al. (2021). Inclusion complexes of non-steroidal anti-inflammatory drugs with cyclodextrins: a systematic review. Biomolecules 11:361.
- Mittal M, Mehta P, Rajput S, et al. (2021). The pharmacological assessment of resveratrol on preclinical models of rheumatoid arthritis through a systematic review and meta-analysis. Eur J Pharmacol 910:174504.
- Nagai N, Ogata F, Yamaguchi M, et al. (2019). Combination with l-menthol enhances transdermal penetration of indomethacin solid nanoparticles. IJMS 20:3644.
- Nair RS, Morris A, Billa N, et al. (2019). An evaluation of curcumin-encapsulated chitosan nanoparticles for transdermal delivery. AAPS PharmSciTech 20:69.
- Nalamachu S, Gudin J. (2020). Characteristics of analgesic patch formulations. J Pain Res 13:2343–54.
- Nozawa T, Nishimura K, Ohara A, et al. (2019). Primary varicella infection in children with systemic juvenile idiopathic arthritis under tocilizumab therapy. Mod Rheumatol 29:558–62.
- Oray M, Abu Samra K, Ebrahimiadib N, et al. (2016). Long-term side effects of glucocorticoids. Expert Opin Drug Saf 15:457–65.
- Pan Q, Yu Y, Chen D, et al. (2020). Enhanced penetration strategies for transdermal delivery. Front Chem Sci Eng 14:378–88.
- Pastore MN, Kalia YN, Horstmann M, et al. (2015). Transdermal patches: history, development and pharmacology. Br J Pharmacol 172:2179–209.
- Petrovska N, Prajzlerova K, Vencovsky J, et al. (2021). The pre-clinical phase of rheumatoid arthritis: from risk factors to prevention of arthritis. Autoimmun Rev 20.
- Pitorre M, Gonde H, Haury C, et al. (2017). Recent advances in nanocarrier-loaded gels: which drug delivery technologies against which diseases? J Control Release 266:140–55.
- Poonia N, Lather V, Kaur B, et al. (2020). Optimization and development of methotrexate- and resveratrol-loaded nanoemulsion formulation using box-behnken design for rheumatoid arthritis. Assay Drug Dev Technol 18:356–68.
- Prabhu P, Shetty R, Koland M, et al. (2012). Investigation of nano lipid vesicles of methotrexate for anti-rheumatoid activity. Int J Nanomed 7:177–86.
- Prausnitz MR, Langer R. (2008). Transdermal drug delivery. Nat Biotechnol 26:1261–8.
- Pund S, Pawar S, Gangurde S, et al. (2015). Transcutaneous delivery of leflunomide nanoemulgel: mechanistic investigation into physicomechanical characteristics, in vitro anti-psoriatic and anti-melanoma activity. Int J Pharm 487:148–56.
- Qindeel M, Khan D, Ahmed N, et al. (2020). Surfactant-free, self-assembled nanomicelles-based transdermal hydrogel for safe and targeted delivery of methotrexate against rheumatoid arthritis. ACS Nano 14:4662–81.
- Quinones OG, Pierre MBR. (2019). Cutaneous application of celecoxib for inflammatory and cancer diseases. Curr Cancer Drug Targets 19:5–16.
- Rabiei M, Kashanian S, Samavati SS, et al. (2020). Nanomaterial and advanced technologies in transdermal drug delivery. J Drug Target 28:356–67.
- Rai VK, Mishra N, Yadav KS, et al. (2018). Nanoemulsion as pharmaceutical carrier for dermal and transdermal drug delivery: formulation development, stability issues, basic considerations and applications. J Control Release 270:203–25.
- Romgens AM, Bader DL, Bouwstra JA, et al. (2015). Diffusion profile of macromolecules within and between human skin layers for (trans)dermal drug delivery. J Mech Behav Biomed Mater 50:215–22.
- Rouphael NG, Paine M, Mosley R, et al. (2017). The safety, immunogenicity, and acceptability of inactivated influenza vaccine delivered by microneedle patch (tiv-mnp 2015): a randomised, partly blinded, placebo-controlled, phase 1 trial. The Lancet 390:649–58.
- Salem RM, El-Deeb AE, Elsergany M, et al. (2021). Intra-articular injection of etanercept versus glucocorticoids in rheumatoid arthritis patients. Clin Rheumatol 40:557–64.
- Salem HF, Nafady MM, Kharshoum RM, et al. (2020). Novel enhanced therapeutic efficacy of dapoxetine hcl by nano-vesicle transdermal gel for treatment of carrageenan-induced rat paw edema. AAPS PharmSciTech 21:113.
- Samuels JS, Holland L, Lopez M, et al. (2018). Prostaglandin e2 and il-23 interconnects stat3 and RoRγ pathways to initiate Th17 CD4+ T-cell development during rheumatoid arthritis . Inflamm Res 67:589–96.
- Sana E, Zeeshan M, Ul Ain Q, et al. (2021). Topical delivery of curcumin-loaded transfersomes gel ameliorated rheumatoid arthritis by inhibiting NF-κβ pathway. Nanomedicine (Lond) 16:819–37.
- Scott DL, Wolfe F, Huizinga TWJ. (2010). Rheumatoid arthritis. Lancet 376:1094–108.
- Seok SH, Lee S-A, Park E-S. (2018). Formulation of a microemulsion-based hydrogel containing celecoxib. J Drug Deliv Sci Technol 43:409–14.
- Shan QQ, Jiang XJ, Wang FY, et al. (2019). Cubic and hexagonal liquid crystals as drug carriers for the transdermal delivery of triptolide. Drug Deliv 26:490–8.
- Shewaiter MA, Hammady TM, El-Gindy A, et al. (2021). Formulation and characterization of leflunomide/diclofenac sodium microemulsion base-gel for the transdermal treatment of inflammatory joint diseases. J Drug Deliv Sci Technol 61:102110.
- Shu Z, Cao Y, Tao Y, et al. (2020). Polyvinylpyrrolidone microneedles for localized delivery of sinomenine hydrochloride: preparation, release behavior of in vitro & in vivo, and penetration mechanism. Drug Deliv 27:642–51.
- Silvestrini AVP, Caron AL, Viegas J, et al. (2020). Advances in lyotropic liquid crystal systems for skin drug delivery. Exp Opin Drug Deliv 17:1781–805.
- Simon LS, Taylor PC, Choy EH, et al. (2021). The jak/stat pathway: a focus on pain in rheumatoid arthritis. Semin Arthritis Rheum 51:278–84.
- Solomon DH, Husni ME, Libby PA, et al. (2017). The risk of major nsaid toxicity with celecoxib, ibuprofen, or naproxen: a secondary analysis of the precision trial. Am J Med 130:1415–22 e4.
- Subongkot T, Sirirak T. (2020). Development and skin penetration pathway evaluation of microemulsions for enhancing the dermal delivery of celecoxib. Colloids Surf B Biointerfaces 193:111103.
- Tavakolpour S, Alesaeidi S, Darvishi M, et al. (2019). A comprehensive review of rituximab therapy in rheumatoid arthritis patients. Clin Rheumatol 38:2977–94.
- Tawfeek HM, Abdellatif AAH, Abdel-Aleem JA, et al. (2020). Transfersomal gel nanocarriers for enhancement the permeation of lornoxicam. J Drug Deliv Sci Technol 56:101540.
- Tekko IA, Chen G, Dominguez-Robles J, et al. (2020). Development and characterisation of novel poly (vinyl alcohol)/poly (vinyl pyrrolidone)-based hydrogel-forming microneedle arrays for enhanced and sustained transdermal delivery of methotrexate. Int J Pharm 586:119580.
- Thakur S, Riyaz B, Patil A, et al. (2018). Novel drug delivery systems for nsaids in management of rheumatoid arthritis: an overview. Biomed Pharmacother 106:1011–23.
- Thao le Q, Byeon HJ, Lee C, et al. (2016). Pharmaceutical potential of tacrolimus-loaded albumin nanoparticles having targetability to rheumatoid arthritis tissues. Int J Pharm 497:268–76.
- Vaidya J, Shende P. (2020). Potential of sonophoresis as a skin penetration technique in the treatment of rheumatoid arthritis with transdermal patch. AAPS PharmSciTech 21:180.
- Vena GA, Cassano N, Iannone F. (2018). Update on subcutaneous methotrexate for inflammatory arthritis and psoriasis. Ther Clin Risk Manag 14:105–16.
- Vora D, Garimella HT, German CL, et al. (2022). Microneedle and iontophoresis mediated delivery of methotrexate into and across healthy and psoriatic skin. Int J Pharm 618:121693.
- Wan J, Wang SM, Gui ZP, et al. (2018). Phytantriol-based lyotropic liquid crystal as a transdermal delivery system. Eur J Pharm Sci 125:93–101.
- Wang Q, Jiang H, Li Y, et al. (2017). Targeting nf-kb signaling with polymeric hybrid micelles that co-deliver sirna and dexamethasone for arthritis therapy. Biomaterials 122:10–22.
- Weng C, Xue L, Wang Q, et al. (2021). Comparative efficacy and safety of Janus kinase inhibitors and biological disease-modifying antirheumatic drugs in rheumatoid arthritis: a systematic review and network meta-analysis. Ther Adv Musculoskelet Dis 13:1759720X21999564.
- Wohlrab J, Neubert RH, Michael J, et al. (2015). Methotrexate for topical application in an extemporaneous preparation. J Dtsch Dermatol Ges 13:891–901.
- Wong R, Geyer S, Weninger W, et al. (2016). The dynamic anatomy and patterning of skin. Exp Dermatol 25:92–8.
- Xiang Y, Liang J, Liu L, et al. (2019). Self-nanoemulsifying electrospun fiber enhancing drug permeation. ACS Appl Mater Interfaces 11:7836–49.
- Yamaoka K. (2016). Benefit and risk of tofacitinib in the treatment of rheumatoid arthritis: a focus on herpes zoster. Drug Saf 39:823–40.
- Yang D, Chen M, Sun Y, et al. (2021). Microneedle-mediated transdermal drug delivery for treating diverse skin diseases. Acta Biomater 121:119–33.
- Yang C, Daoping Z, Xiaoping X, et al. (2020). Magnesium oil enriched transdermal nanogel of methotrexate for improved arthritic joint mobility, repair, and reduced inflammation. J Microencapsul 37:77–90.
- Yang M, Gu Y, Yang D, et al. (2017). Development of triptolide-nanoemulsion gels for percutaneous administration: physicochemical, transport, pharmacokinetic and pharmacodynamic characteristics. J Nanobiotechnol 15:88.
- Yoshitomi H. (2019). Regulation of immune responses and chronic inflammation by fibroblast-like synoviocytes. Front Immunol 10:1395.
- Zhang YT, Li Z, Zhang K, et al. (2017). Co-delivery of evodiamine and rutaecarpine in a microemulsion-based hyaluronic acid hydrogel for enhanced analgesic effects on mouse pain models. Int J Pharm 528:100–6.
- Zhang N, Wang Z, Zhao Y. (2020). Selective inhibition of tumor necrosis factor receptor-1 (tnfr1) for the treatment of autoimmune diseases. Cytokine Growth Factor Rev 55:80–5.
- Zheng H, Xu C, Fei Y, et al. (2020). Monoterpenes-containing pegylated transfersomes for enhancing joint cavity drug delivery evidenced by clsm and double-sited microdialysis. Mater Sci Eng C Mater Biol Appl 113:110929.
- Zhu C, Ji Z, Ma J, et al. (2021). Recent advances of nanotechnology-facilitated bacteria-based drug and gene delivery systems for cancer treatment. Pharmaceutics 13:940.