Abstract
A slit die rheometer fitted to a twin-screw extruder was used to measure the viscosity and expansion characteristics of starch-based melts plasticized with water and supercritical CO2. Experiments were conducted for four intermediate moisture starch-water mixtures with varying degree of starch gelatinization (DG), achieved by blends of pregelatinized and native common corn starch. SC-CO2 was shown to be an effective plasticizer for starch-water mixture at 0.45 g SC-CO2/100 g sample, lowering the viscosity of the melt by an average of 14%. Apparent viscosity was measured for shear rate ranging from 100–200 s−1. The viscosity reduction factors based on DG and SC-CO2 indicated that the free volume added to starch-water mixtures with SC-CO2 is an effective mechanism for viscosity reduction. In addition, the higher water affinity of pregelatinized starch increased the water induced plasticization effect in the mixtures. SC-CO2 was a good blowing agent producing distinct expanded microcellular morphology for the starch-water mixtures with DG at or above 80%. The expansion and cellular characterization of these starch-based extrudates produced by SC-CO2 injection may be governed by the viscosity-dependent parameters including gas retention capability, CO2 diffusivity and the pressure drop rate.
INTRODUCTION
Generation of extrusion-based foams from starch melt has received considerable attention in recent years for both food and non-food applications. It typically involves low-moisture (13–20 g water/100 g sample) and high-temperature (130–170°C), short-time (HTST) cooking of starch to produce steam-induced expanded products.[Citation1–3] The ability of starch polymer to form thermoplastic melt upon water plasticization as well as its abundant supply, cheap cost, and high biodegradability makes it a competitive alternative to polystyrene (PS) and other popular foaming polymers. Steam expanded starch foams of conventional process, however, have its limitations in that they have a coarse and very non-uniform cellular structure with cell sizes in the range of 1–3 mm.[Citation4] Moreover, there is little or no control over cell size, density, and therefore the resultant mechanical properties of the end product.
Conventional plastic foam processing by extrusion involves melting of polymer resins and incorporation of blowing agents ranging from hydrocarbon, chlorofluorocarbon to environmental friendly nitrogen and carbon dioxide. Recognizing the potential dissolution and expansion capability of supercritical carbon dioxide (SC-CO2) in starch melt, Rizvi and Mulvaney[Citation5] proposed a novel patented extrusion technology combined with injection of SC-CO2 as a blowing agent and/or an active carrier for production of highly expanded starch foams.[Citation6,Citation7] In this high pressure (> 7.38 MPa), low temperature (< 100°C) process termed supercritical fluid extrusion (SCFX), the conventional roles of water as a plasticizer as well as a blowing agent are decoupled. It obviates the need for steam expansion and high mechanical energy inputs by increasing the moisture content of the feed to reduce the viscous dissipation of heat. Hence, low product temperature becomes attainable, which offers major advantages over steam puffing such as incorporation of heat sensitive ingredients and actives.[Citation8] A key criterion to achieve the good cellular expansion during the SCFX processing of starch-water-CO2 mixtures is to maintain both low temperature (< 100°C) and high moisture content of the mixtures (35–45 g water/100 g sample), which are conditions necessary to attain high CO2 solubility limit in the starch-water mixtures as the main driving force of expansion. In addition, the highly gelatinized matrix is required to achieve good gas retention capacity. It was found from our previous study,[Citation9] by differential scanning calorimeter (DSC), that complete starch gelatinization was not needed to achieve gas retention capacity for cellular expansion during SCFX processing. This has brought forth the hypothesis that there exists a minimum required degree of gelatinization in the starch-based mixtures to produce good microcellular expansion. Such design of partial starch gelatinization not only has the ability to achieve various final product textures, but it also has its application in resolving product texture loss problem typically among the quick-cooking and microwavable food novelty such as noodles, pastas, snacks, and bread products.[Citation10]
Numerous studies on the rheology of polymer/CO2 systems have been previously evaluated. Gerhardt et al.[Citation11] investigated the viscosity-shear rate relationships for polydimethylsiloxane (PDMS)/CO2 mixtures up to 20 g CO2/100 g sample at 50 and 80°C. Kwag et al.[Citation12] studied the viscosity of PS melts containing CO2 from 0 to 5 g/100 g sample up to 16 MPa and 150–175°C. Areerat et al.[Citation13] studied the melt viscosity of low density polyethylene (LDPE)/CO2 solutions up to 5 g CO2/100 g sample and temperature in the range of 150–175°C. Maio et al.[Citation14] examined the viscosity of polycaprolactone(PCL)/CO2 system up to 10 g CO2/100 g sample in the range of 70–85°C. In all, the rheology results concluded that the free volume added to the melt by dissolved CO2 is the principal mechanism for viscosity reduction. Furthermore, the rheology of CO2/polymer melt system has been modeled successfully in terms of classical viscoelastic scaling theory using a shift factor approach, reflecting both dilution effect and free volume increase effect contributed by the dissolved CO2. The extent of viscosity reduction has been attributed to the degree of molecular mobility influenced by the additional free volume, reflected upon the proximity of melt temperature from the glass transition of the CO2/polymer system at operating pressure and temperature condition.[Citation11]
Many SCFX applications ranging from bread dough[Citation15] to masa-based chips,[Citation9] and protein-fortified snacks[Citation6] have been investigated. In this article, we used blends of pregelatinized and native corn starches as the model system to determine the minimum degree of gelatinization (DG) required for good cellular expansion, and to characterize the rheology of starch-water-CO2 mixture at SCFX operating pressure and temperature using an online slit-die rheometer system. The expansion characteristics were measured and correlated with the melt viscosity of varying concentrations of SC-CO2 and the DG in the starch-water mixtures.
MATERIALS AND METHODS
Preparation of Materials
Native (C∗Gel) and pregelatinized (C∗Instant) corn starch (∼23% amylose) were obtained from Cerestar (Hammond, IN). Four starch-water mixtures containing a 60:40, 80:20, 90:10, and 100:0 ratio of pregelatinized to native starch were prepared by dry blending. Water was added during SCFX processing to achieve 42 g water/100 g extrudate. High purity (∼ 99%) CO2 from Air Gas (Ithaca, New York) was used in all SCFX trials.
Extrusion and Rheometer System
The viscosities of the SC-CO2/starch-water mixtures were measured with an online slit die rheometer attached to a co-rotating twin screw extruder (Wenger TX-57 Magnum, Sabetha, KS). This extruder with a L/D ratio of 28.5 was specially configured for the SCFX process and SC-CO2 was injected into the barrel at L/D of 24. The screw configuration, pressure profile and temperature control zones are shown in . The slit die rheometer used in this study had length (L) = 325 mm, width (W) = 50.8 mm, and height (H) = 3.175 mm as illustrated in . This die dimension with (W/H) ratio of 16 and (L/H) ratio of 102 had the ability to produce fully developed flow and to minimize the wall slippage and entrance effects. Two precalibrated pressure transducers were inserted at two ports along the slit die rheometer at a distance of 170 and 300 mm from the die entrance. Immediately downstream 38 mm from the die entrance, a bypass line with a valve was included to vary the rate of main flow traveling across the slit die rheometer.
Figure 1 Typical screw configuration and corresponding pressure profile developed along the extruder barrel during SCFX processing.
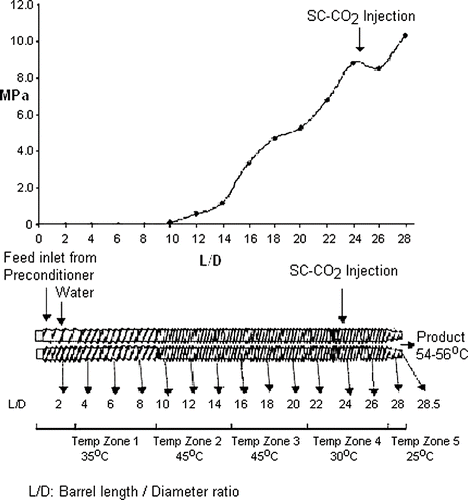
A SC-CO2 generation and metering system with a PID control loop was used to stabilize and maintain the mass flow to the set point at high operating pressure range between 10 and 20 MPa and target temperature. By activating a back-pressure type regulator, this system can rapidly and smoothly correct for any changes in the extruder conditions without becoming unstable. Hence, the concentration of injected SC-CO2 into the starch-water mixture can be ensured during viscosity measurement.
For each set of extrusion treatments, three to four different flow rates of extrudate were used by manipulating the bypass valve. Flow rates of the extrudate were measured by weighing material outflow during a 30 second time interval at steady extrusion conditions. Moisture of the extrudates was determined by oven drying method. Simultaneously, corresponding pressure drop data from the pressure transducers were recorded automatically every 10 seconds.
Experimental Procedures
Starch melt viscosity was measured for the starch-water mixtures with four different blends of pregelatinized and native corn starches with and without injected SC-CO2. The temperature of the extruder zone was set respectively at 35, 45, 45, 30, and 25°C from zone 1 to 5 (). The product temperature at the exit was at 55°C. Screw speed was set at 180 rpm. Dry feed rate was set at 9.7 × 10−3 kg/s. The moisture contents of the extrudate melt were adjusted to 42% by adding water to the barrel at 5.82 × 10−3 kg/s (60% dry basis). Specific mechanical energy (SME) input of the extruder was 240 kJ/kg. SC-CO2 injection was set at 6.79 × 10−5 kg/s (0.7% dry basis) for expanded samples. At this injection rate, the SC-CO2 concentration is at 0.45 g SC-CO2/100 g CO2-starch-water mixture.
Experiments started with maximum flow rate of the extrudate while keeping the bypass flow rate to minimum. Next, the flow rate of extrudate was decreased stepwise by adjustment to the bypass valve. Total of 4 flow rates through the die were obtained for each starch blend formulation tested. The raw data of pressure readings and measured mass flow rates were collected in triplicates and used to calculate shear stress and shear rate. Data reported were the results of extrusion runs for these 4 starch blends with and without SC-CO2 injection.
Extrudate Expansion and Microstructure Analysis
The expansion ratio (ER) was calculated as the cross-section area of the extrudate divided by the cross-section area of the circular shaping orifice attached at the end of the slit die. From each formulation, extrudate samples were dried at 75°C convection oven for 8 hours. Each ER value was an average of 30 measurements. A scanning electron microscope (Leica 440 SEM) was used for examining the cellular structure of extrudates and measuring the average cell size. Samples were cut into 5mm-long cylinders and mounted on aluminum stubs with double side conductive carbon tape, and sputter coated with palladium gold before mounting in the vacuum chamber of SEM for imaging. Image processing software was used to measure the average cell diameters from the SEM analysis. The cell density for each sample was calculated by:
where is the weight average of cell sizes, and
is the number average of cell sizes.
Apparent Viscosity Calculations
The shear stress at the walls of the slit die was determined from the slit height (H) and the pressure drop (ΔP) data across the first and second transducers separated by a distance of ΔL:
The apparent shear rate at the walls was calculated from the measured mass flow rate
(kg/s) and density ρ (kg/m3) of the mixture estimated by their weight fraction contribution from the density of starch-water mixtures and of CO2 at measured pressure range and 55°C using the following equation:
where W is the slit width. The rheological parameters, K’(consistency index) and n (flow behavior index) were calculated from linear regression of the log-log plot of shear stress versus apparent shear rate using the power law equation to obtain the best fitting values:
The actual shear rate at the wall was then calculated using n as follows:
Apparent viscosity of the mixture (ηapp) was calculated as a function of the wall shear rate:
where the consistency index, K (Pa-s) was given as:
Viscosity Reduction Factors Calculation
To quantitatively determine the effects of added SC-CO2 and native starch on the viscosity of a molten polymer-like starch-water mixture, a fundamental understanding of the plasticization is required. Several research groups have attempted to address the relationship of free volume and plasticization on the molten polymer rheology. The SC-CO2/polymer mixtures are generally regarded as polymer diluted by a solvent, where entangled polymer chain dynamics prevail and where the polymer contribution to viscosity predominates over solvent contribution. Thus, the viscosities of the SC-CO2/polymer mixtures are expected to be reduced, relative to pure molten polymer, by the dilution of the concentration of entangled polymer chains and by the increase in mixture free volume due to dissolved SC-CO2. Han and Ma[Citation16,Citation17] reported that the viscosity curves for mixtures of polystyrene and polyethylene with fluorocarbon blowing agents can be reduced to a master curve by applying a shift factor to only the viscosity values, defined as the viscosity reduction factor (VRF):
Their data were taken in the shear-thinning power-law portions of the viscosity curves. For this work, two viscosity reduction factors: VRFc and VRFg to describe for the viscosity change due to SC-CO2 concentration and DG were determined respectively. Hence, the viscosity reduction contributions would facilitate us in better understanding the high pressure rheology behavior of SC-CO2 plasticized starch-water mixtures during SCFX process.
RESULTS AND DISCUSSION
Rheological Parameters
shows the consistency index (K), and the flow behavior index (n) of starch-water mixtures determined in the shear rate range of 100 to 200 s−1 using EquationEqs. (5) and Equation(8). The data for all starch-water and starch-water-CO2 mixtures show the consistency index, K, increased as DG increased from 60 to 100% level. In addition, the K values of starch-water mixtures were always higher than their respective SC-CO2 plasticized mixtures.
Table 1 Consistency index and flow behavior index as a function of degree of gelatinization and SC-CO2 addition for starch-water mixtures with 42% water content
This trend of K value is well supported by the work of Willet et al.,[Citation18] in which the K values of thermoplastic starch melts were shown to decrease with increasing moisture content and addition of other plasticizer-like low molecular weight additives. The flow behavior index, n, increased with the addition of SC-CO2 but decreased as DG increased from 60 to 100% level. For the starch-water mixtures, n decreases from 0.46 to 0.21, and for starch-water-CO2 mixtures from 0.71 to 0.31. These results correspond to the works of Singh and Smith[[Citation19] and of Willet et al.,Citation20] in which the flow behavior index of thermoplastic wheat starch and pre-extruded corn starch increased with moisture content, temperature, and with the addition of plasticizer such as wheat germ oil.
These results are in line with the relationship between the flow behavior index and the polymer entanglement density in the mixture, in which a smaller n value reflects an increased polymer entanglement density.[Citation19,Citation21] In their work, Della Valle et al.[Citation22] observed a decrease in the power law index with increasing amylose content and came to conclude that linear amylose would more likely contribute to entanglements whereas the highly branched amylopectin would not form much entanglements. In our study, the lowest n value was observed for the starch-water mixtures with 100% DG with no CO2 added. Such result would suggest the mixtures with 100% DG to have the highest polymer entanglement density due to the least dilution effect contributed by either CO2 molecules or native starch concentration in the mixtures.
Apparent Viscosity of Starch-Water Mixtures
Apparent viscosity of the starch-water mixture was measured with K and n values respective of each starch-water mixture and the corresponding shear rate using EquationEq. (7). The viscosity versus shear rate relationship for starch-water mixtures only and with 0.45 g SC-CO2 /100 g sample added at 55°C are shown respectively, in and . For the starch-water only mixtures, the viscosity shows a decrease as DG lowered from 100 to 60%. Such viscosity dilution induced by the lowering of DG can be attributed to the higher water affinity of pregelatinized starch over native starch fraction. At the same water content, the starch-water mixture with lower DG or lower concentration of pregelatinized starch would take more water away from the native starch, inducing a stronger water plasticization effect to produce a lower viscosity. Moreover, the viscosity of starch-water mixture in shows to decrease consistently with addition of 0.45 g SC-CO2/100 g sample, suggesting that the free volume added to starch-water mixtures with SC-CO2 is an effective mechanism for viscosity reduction.
Figure 3 Viscosity of starch-water mixtures with starch of various degrees of gelatinization as a function of shear rate at 55°C.
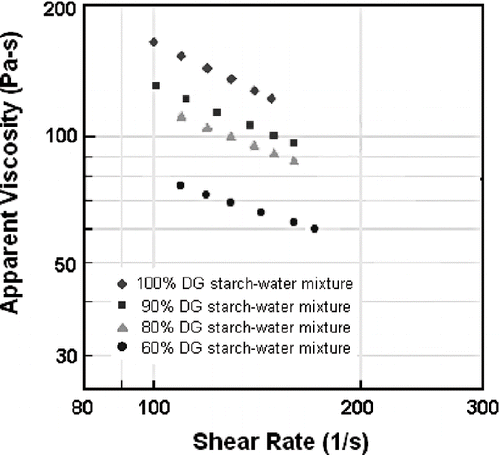
Figure 4 Viscosity of starch-water-CO2 mixtures with 0.45% wt CO2 and starch with various degrees of gelatinization as a function of shear rate at 55°C.
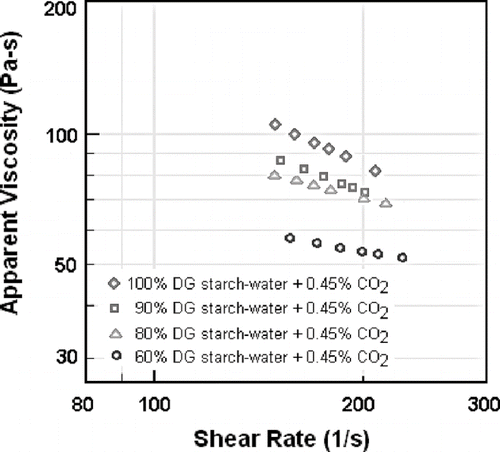
From the viscosity data measured between 100–200 s−1 in and , the gelatinization and concentration dependent viscosity reduction factors, VRFg and VRFc, were calculated using EquationEq (9) and determined for the various combinations of starch blends and SC-CO2 concentration at 55°C. VRF's showed to be slightly dependent on shear rate, yielding about 10% more reduction from 100 s−1 to 200 s−1.
illustrates the VRF results with respect to DG levels and SC-CO2 addition at the shear rate of 150 s−1. For the starch-water mixtures with 0.45 g SC-CO2 /100 g sample addition, the VRFc has a mean value of 0.86, indicating that mixture with 0.45 g SC-CO2/100 g sample added consistently achieves a 14% viscosity reduction. Moreover, the VRFg without the effect of SC-CO2 addition yields 17%, 24%, and 46% viscosity reduction for 90%, 80%, and 60% DG mixtures, respectively.
Figure 5 Variation of viscosity reduction factors with respect to SC-CO2 and DG levels for starch-water mixtures at the shear rate of 150/s.
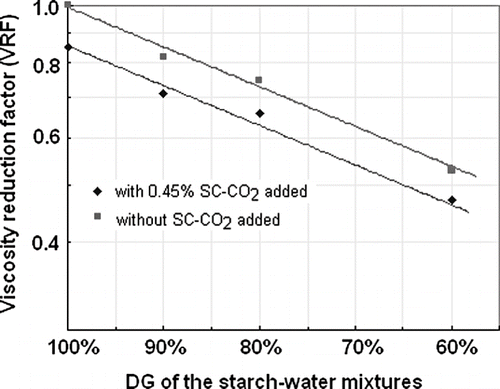
In comparison of the two diluents, SC-CO2 appears to be a more effective diluent on a weight basis to reduce melt viscosity. In their rheology evaluation of starch-water mixtures containing native and pregelatinized wheat starch blends, Alavi et al.[Citation23] indicated that native starch granules act as an inert diluent by increasing the free volume of the starch-water mixtures and decreasing the volume fraction of starch polymers in the starch-water system. Moreover, the higher water affinity of pregelatinized starch increased the water induced plasticization effect in the mixtures with higher native starch concentration. It is important to note that the mixture viscosity would play a key process control for the extrudate expansion and microstructure development during SCFX processing.
Effect of Viscosity on Extrudate Expansion
illustrated the expansion ratio (ER) for the starch-water mixtures with respect to their DG level and SC-CO2 injection. With 0.45 g SC-CO2/100 g sample injection, ER of the extrudates increased with DG from 2.7 to 7.1 times the cross sectional area of the circular shaped die. The extrudates without SC-CO2 injection only produced ER of 1.1 to 1.7 times, reflecting the sole contribution effect of die swelling based on the material property of the starch-water mixtures with 60 to 100% DG levels. Based on the ER results and expansion observation, the starch-water mixture with the lowest DG of 60% did not possess sufficient gelatinized gas holding capability and could not produce distinct expanded cellular morphology. Since all tests with SC-CO2 addition had 0.45 g SC-CO2/100 g sample, it would indicate that considerable amount of CO2 had escaped from the extrudate for the starch-water mixtures with lower viscosity corresponding to the 60% DG samples. Moreover, the lower viscosity produced by the starch-water mixtures with 60% DG would also more likely promote product collapse due to its weaker structural integrity.
Figure 6 Expansion of starch-water mixtures with 0.45 wt% SC-CO2 injection using SCFX system with die diameter of 4.50 mm.
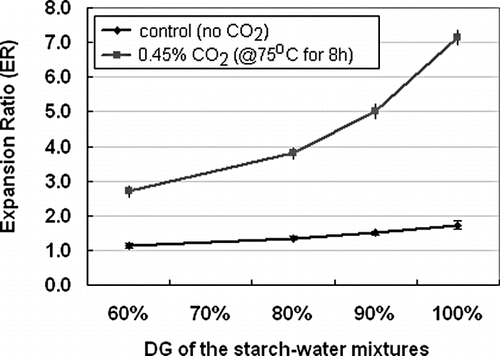
In general, SC-CO2 showed to be a good blowing agent at 0.45 g/100 g sample level where the starch-water mixtures with highest viscosity corresponding to 100% DG samples produced the maximum expansion. The extent of extrudate expansion is likely governed by the gas retention capability which correlates well with the measured apparent viscosity in the DG range examined. As expected, the higher pressure observed in the slit die rheometer was obtained by more viscous starch-water mixtures with higher DG levels. The formation of a less expanded product by the less viscous mixture was likely due to larger loss of CO2 gas since the pressure near die may fall below the saturated solubility of injected SC-CO2 to prematurely nucleate and more likely to escape from the extrudate surface. Hence, with prudent manipulation of formulation, SC-CO2 injection rate, and other processing conditions, we can achieve the target expansion and desired texture for novel food product applications using SCFX process.
Effect of Viscosity on Extrudate Microstructure
showed the effect of DG and SC-CO2 injection on the expanded microstructure in terms of their average cell size, average cell density, and cell size distribution for the various starch-water mixtures with 60–100% DG levels. Based on the scanning electron microscopy (SEM) at 32 times magnification, the average cell size decreased from 410 to 310 μm as DG increased from 80 to 100% while average cell density increased an order of magnitude to 1.4 × 105 cells/cm3. These SCFX extrudates demonstrated its uniformity in cell size distribution with the polydispersity index (PDI) values calculated between 0.81–0.84, much higher compared to the PDI value of 0.30 of typical steam puffed extrudates.[Citation4]
Table 2 Microstructure characteristics of starch-water mixtures with 0.45 wt% SC-CO2 injection
showed the expanded starch-based extrudate with 100% DG to produce microstructure with more smaller bubbles and higher cell density. This result was attributed to the lower gas diffusivity found in the more viscous starch-water mixtures corresponding to the higher DG level.[Citation24] In addition, cell coalescence and ruptures were more frequently observed for the starch-water mixtures with lower DG levels, indicating that the lower mixture viscosity generated a weaker structural integrity unable to withstand the dynamics of adjacent growing nuclei. As expected, the smaller pressure drop rate observed by the starch-water mixtures with lower DG would experience a smaller thermodynamic instability to induce fewer nuclei formation. In all, the final expanded cellular microstructure would be governed by the viscosity-dependent CO2 diffusivity into the nuclei and the competition between nuclei formation and bubble growth affected by the pressure drop rate experienced at the die. CO2/polymer system with higher pressure drop rate and lower diffusion rate would favor more nucleation activity, while CO2/polymer system with lower pressure drop rate and higher diffusion rate would favor more bubble growth activity. These interpretations are similar to those proposed by Goel and Beckman[Citation25] about CO2-induced microcellular formation.
CONCLUSIONS
Apparent viscosity and expansion characteristics of starch-based melts plasticized with water and supercritical CO2 were evaluated using a slit die rheometer fitted to a twin-screw extruder for four intermediate moisture starch-water mixtures with varying DG levels, achieved by blends of pregelatinized and native common corn starch. Apparent viscosity of the starch-water mixtures measured between 100–200 s−1 was found to reduce by an average of 14% with the addition of 0.45 g SC-CO2/100 g sample independent of the DG level in the mixtures. The viscosity reduction factors (VRF) determined based on SC-CO2 and DG levels suggested that the free volume added to starch-water mixtures with SC-CO2 effectively reduced viscosity, and that the higher water affinity of pregelatinized starch increased the water induced plasticization effect in the mixtures. Observing from the extrudate expansion, the results indicated that the starch-water mixtures would require DG at or above 80% to achieve good gas-holding rheology to produce nonporous skin and distinct cellular interior morphology. Moreover, expansion and cellular characterization of these starch-based extrudates can be well justified by the viscosity-dependent gas retention capability, CO2 gas diffusivity in the starch-water mixtures, and the pressure drop rate experienced at the die. Using this knowledge of high pressure CO2-plasticized rheology of the starch-water mixtures, we may then achieve better controlled expansion and greater flexibility of innovation by supercritical fluid extrusion (SCFX).
ACKNOWLEDGMENTS
The authors would like to acknowledge the technical assistance support from Esref Dogan, and the pilot scale extruder system provided by Wenger Manufacturing, Inc.
REFERENCES
- Chinnaswamy , R. and Bhattacharya , K.R. 1986 . Characteristics of Gel-chromatographic Fractions of Starch in Relation to Rice and Expanded Rice-product Qualities . Starch , 2 : 51 – 57 .
- Mercier , C. and Feillet , P. 1975 . Modification of Carbohydrate Components by Extrusion-cooking of Cereal Products . Cereal Chemistry , 52 ( 3 ) : 283 – 297 .
- Gujral , H.S. and Singh , N. 2002 . Extrusion Behavior and Product Characteristics of Brown and Milled Rice Grits . International Journal of Food Properties , 5 ( 2 ) : 307 – 316 .
- Barrett , A.M. and Peleg , M. 1992 . Cell Size Distribution of Puffed Corn Extrudates . J. Food Sci , 57 : 146 – 148 .
- Rizvi , S.S.H. and Mulvaney , S.J. Extrusion Processing with Supercritical Fluids . US Patent 5,120,559 . filed October 3, 1991 and issued June 9, 1992
- Rizvi , S.S.H. , Mulvaney , S.J. and Sokhey , A.S. 1995 . The Combined Application of Supercritical Fluid and Extrusion technology . Trends in Food Science and Technology , 6 : 232 – 240 .
- Alavi , S. and Rizvi , S.S.H. 2005 . Strategies for Enhancing Expansion in Starch-based Microcellular Foams Produced by Supercritical Fluid Extrusion . International Journal of Food Properties , 8 : 23 – 24 .
- Alavi , S.H. , Gogoi , B.K. , Khan , M. , Bowman , B.J. and Rizvi , S.S.H. 1999 . Structural Properties of Protein-stabilized Starch-based Supercritical Fluid Extrudates . Food Research International , 32 : 107 – 118 .
- Chen , K.H. , Dogan , E. and Rizvi , S.S.H. 2002 . Supercritical Fluid Extrusion of Masa-based Snack Chips . Cereal Foods World , 47 ( 2 ) : 44 – 51 .
- Thammathongchat , S. , Fukuoka , M. and Watanabe , H. 2005 . An Innovative Noodle: Gelatinized at the Core, Leaving the Surface Ungelatinized . Journal of Food Engineering , 70 : 27 – 33 .
- Gerhardt , L.J. , Garg , A. , Manke , C.W. and Gulari , E. 1998 . Concentration-dependent Viscoelastic Scaling Models for Polydimethysiloxane Melts with Dissolved Carbon Dioxide . Journal of Polymer Science: Part B: Polymer Physics , 36 : 1911 – 1918 .
- Kwag , C. , Manke , C.W. and Gulari , E. 1999 . Rheology of Molten Polystyrene with Dissolved Supercritical and Near-critical Gases . Journal of Polymer Science: Part B: Polymer Physics , 37 : 2771 – 2781 .
- Areerat , S. , Nagata , T. and Ohshima , M. 2002 . Measurement and Prediction of LDPE/CO2 Solution Viscosity . Polymer Engineering and Science , 42 ( 11 ) : 2234 – 2245 .
- Maio , E.D. , Mensitieri , G. , Iannace , S. , Nicolais , L. , Li , W. and Flumerfelt , R.W. 2005 . Structure Optimization of Polycaprolactone Foams by Using Mixtures of CO2 and N2 as Blowing Agents . Polymer Engineering and Science , 45 : 432 – 441 .
- Hicsasmaz , Z. , Dogan , E. , Chu , C. and Rizvi , S.S.H. 2003 . Leavened Dough Processing by Supercritical Fluid Extrusion (SCFX) . Journal of Agricultural and Food Chemistry , 51 : 6191 – 6197 .
- Han , D.H. and Ma , C.Y. 1983 . Rheological Properties of Mixtures of Molten Polymer and Fluorocarbon Blowing Agent. I. Mixtures of Low-density Polyethylene and Fluorocarbon Blowing Agent . Journal of Applied Polymer Science , 28 : 831 – 850 .
- Han , D.H. and Ma , C.Y. 1983 . Rheological Properties of Mixtures of Molten Polymer and Fluorocarbon Blowing Agent. II. Mixtures of Low-density Polystyrene and Fluorocarbon Blowing Agent . Journal of Applied Polymer Science , 28 : 851 – 860 .
- Willett , J.L. , Millard , M.M. and Jasberg , B.K. 1997 . Extrusion of Waxy Maize Starch: Melt Rheology and Molecular Weight Degradation of Amylopectin . Polymer , 38 ( 24 ) : 5983 – 5989 .
- Singh , N. and Smith , A.C. 1999 . Rhelologcial Behaviour of Different Cereals Using Capillary Rheometry . Journal of Food Engineering , 39 : 203 – 209 .
- Willett , J.L. , Jasberg , B.K. and Swanson , C.L. 1995 . Rheology of Thermoplastic Starch: Effects of Temperature, Moisture Content, and Additives on Melt Viscosity . Polymer Engineering Science , 35 : 202
- Della Valle , G. , Vergnes , B. , Colonna , P. and Patria , A. 1997 . Relations Between Rheological Properties of Molten Starches and Their Expansion Behavior in Extrusion . Journal of Food Engineering , 31 : 277 – 296 .
- Della Valle , G. , Colonna , P. , Patria , A. and Vergnes , B. 1996 . Influence of Amylose Content on the Viscous Behavior of Low Hydrated Molten Starches . Journal of Rheology , 40 : 347 – 362 .
- Alavi , S.H. , Chen , K.H. and Rizvi , S.S.H. 2002 . Rheological Characteristics of Intermediate Moisture Blends of Pregelatinized and Raw Wheat Starch . Journal of Agricultural and Food Chemistry , 50 : 6740 – 6745 .
- Chen , K.H.J. and Rizvi , S.S.H. 2006 . Measurement and Prediction of Solubilities and Diffusion Coefficients of Carbon Dioxide in Starch-water Mixtures at Elevated Pressures . Journal of Polymer Science: Part B , 44 : 607 – 621 .
- Goel , S.K. and Beckman , E.J. 1994 . Generation of Microcellular Polymeric Foams Using Supercritical Carbon Dioxide. II: Cell Growth and Skin Formation . Polymer Engineering and Science , 34 ( 14 ) : 1148 – 1156 .