Abstract
The freezing point depression of mango and papaya pulps was measured by using a simple apparatus, consisting of two major sections: a freezing vessel and a data acquisition system. The thermal conductivity of both pulps as a function of frozen water fraction and temperature was also investigated by using a coaxial dual-cylinder apparatus. Thermal conductivity above the initial freezing point was well fitted by polynomial equations. Below the freezing point, the thermal conductivity was strongly affected by both the frozen water fraction and temperature. Simple equations in terms of frozen water fraction and temperature could be fitted to the experimental data of freezing point depression and thermal conductivity.
INTRODUCTION
The fruit juice industry has become one of the world's biggest agribusinesses. Although Brazil is the main exporter of concentrated juices, there are many other countries involved in the fruit juice and pulp market, principally in the domain of tropical fruits. The mango (Mangifera indica L.) is a tropical fruit, originally from the South of Asia, and it is available worldwide today. Mango is rich in vitamin A and contains reasonable amounts of vitamins B and C, beyond minerals, mainly iron.[Citation1] On the other hand, papaya (Carica papaya L.) cultivation is favored by suitable climatic conditions, and there is successful commercial production, primarily in Hawaii, tropical Africa, the Philippines, India, Ceylon, Malaya and Australia, apart from the widespread but smaller scale production in South Africa and Latin America. Papaya is regarded as a good source of vitamins A, B, and G and as an excellent source of vitamin C.[Citation2] Both fruits are mainly consumed in natural form, but can be also industrialized as pulps, jellies, juices, and dehydrated fruits. Some products such as pulps and concentrated juices are normally stored under freezing conditions.
Knowledge of thermal properties of frozen mango and papaya pulps are essential to calculate the temperature distribution during freezing and frozen storage, and to estimate the freezing time. Food engineers are interested in predicting freezing times in order to estimate the refrigeration requirements for freezing systems and to design the necessary equipment for effective processing. Minimization of the energy requirement, reliability, safety, and quality of the product must also be considered.[Citation3]
The freezing point temperature relative to 0°C, also called “freezing point depression” (FPD), which increases with increasing solute concentration, is an important thermophysical property in freezing processes. It is known that the freeze-drying process involves freezing of the fresh product, heating of the frozen foods at low temperature to induce sublimation, condensation of water vapor, and mechanical energy consumption to maintain the vacuum. The main disadvantage of this technique is the high capital and operating costs, limiting its use in the food industry and restricting freeze-drying to dehydration of only high-added value products, such as instant coffee or baby foods. However, it has recently been proposed to freeze-dry fruits and vegetables to be used as high quality ingredients in ready-to-prepare soups, snacks, and delicacies.[Citation4] With these considerations, knowledge of thermophysical properties related to freezing of fruit pulps is also important for freeze-drying of this kind of material.[Citation5,Citation6] The aim of this article was to determine freezing point depression and thermal conductivity of mango and papaya pulps as affected by frozen water fraction and temperatures from -26 to 74°C.
MATERIAL AND METHODS
Sample Preparation
Both fruits were acquired at a local market and kept in a cold room at 7°C prior to their use. Samples of mango (Mangifera indica L) fruit (variety Keith) presented the following composition: 84.4% of water (wet basis); 14.2°Brix; 17.5 g of total sugars/100 g; 2.4 g of fibers/100 g; 0.4 g of ashes/100 g.[Citation7] The main composition of papaya (Carica papaya L) fruit was determined with the following results: 88.2% of moisture (wet basis.); 9.8°Brix; 11.8 g of total sugars/100 g; 1.6 g of fibers/100 g; 0.5 g of ashes /100 g.[Citation7] The pulps were prepared in a pilot scale equipment with fruits of similar degree of ripeness (12°Brix) that were passed through finishers with a 1.6-mm screen. This finisher was chosen aiming to obtain the maximum yield in pulp extraction, as well as a pulp of high homogeneity.[Citation8] After processing, the pulps were quickly plate-frozen and stored at −20°C until used for the experiment.
Experimental Apparatus and Measurement Procedure
Freezing point depression
A schematic diagram of the apparatus used for experimental measurement of the freezing point depression, similar to that described by Chen and Chen,[Citation9] is shown in . It consists of two major components: a freezing vessel and a data acquisition system. The freezing vessel is a Dewar flask containing liquid nitrogen, closed with an inverted aluminum cone supported by a polystyrene cap. The aluminum cone has an angle of 90°, wall thickness of 1 mm and largest diameter of 4 cm. Each experiment was carried out by supplying the mango or papaya pulp to the bottom of the cone, which was then cooled by the evaporating nitrogen inside the Dewar flask. The temperature of the sample contained in the cone was measured by a thermocouple fixed on a lab stand. The thermocouple was placed at a distance of 5 mm from the cone bottom and was connected to a temperature transmitter (model TT302, SMAR, Sertãozinho, SP, Brazil).
Thermal Conductivity
The system used to measure thermal conductivity is a coaxial dual-cylinder apparatus and is schematically shown in . This method was originally presented by Bellet et al.[Citation10] and was already used by Telis-Romero et al.[Citation11,Citation12] for measuring thermal conductivity of orange juice above the freezing point. The heater is a uniformly distributed electric resistance inserted along the axis of the inner cylinder (220 mm long and 20 mm in diameter) to provide a radial heat flux. Samples were loaded into the annular space between the inner and outer cylinders (inner diameter of 42 mm and length of 220 mm), with both ends being fitted with nylon stoppers to prevent axial heat transfer. Before being loaded, samples were degassed under vacuum for 20 minutes to remove air bubbles. Only 95% of the available volume was filled with sample in order to allow for expansion during freezing. The apparatus was then immersed in a thermostatic bath (model MA-184, Marconi, São Paulo, Brazil) containing ethyl alcohol. The power input to the heater resistance was made by means of a laboratory DC power supply (model MPS-3006D, Minipa, São Paulo, Brazil), which permitted to adjust the current with a stability of 0.05%. Temperatures were monitored with an accuracy of 0.6°C by a HP data logger model 75.000-B, an interface HP-IB, and a HP PC running a data acquisition program written in IBASIC. In order to measure temperature, one and three copper-constantan thermocouples were embedded at the surfaces of the inner and outer cylinders, respectively. In the steady state, conduction inside the cell is described by the Fourier equation in cylindrical coordinates, with boundary conditions corresponding to heat transfer between 2 concentric cylindrical surfaces, kept at constant temperatures, as given by Gabas et al.[Citation13] EquationEq. (1) is given by Bellet et al.[Citation10] and can be used to calculate the sample thermal conductivity, λ, from experimental measurements of T1 and T2 under steady state conditions:
where is the heat flux in the thermal resistance (W); r the radius (m); R1 and R2 respectively the external and internal radius of the cylinder (m); S the surface area of a cylinder of radius r (m2); T the temperature (°C); T1 the steady state temperature at the internal cylinder (°C); T2 the steady state temperature in the thermostatic bath where cell was immersed (°C); and λ the thermal conductivity of the sample at the average temperature (T1+T2)/2 (W/m.°C).
Calibration procedure
The apparatus used for measuring freezing point depression was calibrated by using ethylene glycol, which has a well-known FPD. Fourteen replicated experiments were carried out and the average result is presented in , as well as a reference value reported by Perry and Chilton.[Citation14] A very good agreement was observed between the experimental and reference values. The reproducibility of results was also satisfactory, as shown in the histogram of data distribution () that presents a normal distribution of measurements around the average value. Values of the standard deviation (sd) and standard error (se) were also included in .
Table 1 Data obtained during calibration of the experimental apparatus for freezing point depression (FPD) and thermal conductivity
RESULTS AND DISCUSSION
Freezing Point Depression
Generally, it was expected that the larger the sample size, the slower the cooling process. This could interfere with the measurement of the initial freezing point, and in order to detect this source of experimental errors, samples of approximately 0.5, 0.7, and 1.0 ml of papaya and manga pulp were tested in separate runs. In these experiments, the thermocouple position was fixed at about 5 mm from the bottom of the cone. The freezing curves were recorded and the set of curves corresponding to mango pulp is shown in . Once the liquid sample became solid (very fast in the case of a small sample), the temperature decreased. It was observed that the sample size did not have a large effect on the plateau temperature of the freezing curves, and the initial freezing point was similar for all sample sizes. For mango pulp, values of the freezing point depression obtained with different sample volumes were approximately −1.69°C. The values of supercooling varied from −1.94 to −2.14°C. Similar behavior was observed for the papaya pulp.
The influence of the thermocouple position on the measurement of freezing point depression was also investigated. The freezing curves obtained with the thermocouple placed at distances varying from 5 to 9 mm from the sample holder bottom are shown in . The freezing curves were not affected by the different positions of the temperature measurement point. Similar results were obtained for papaya pulps. On the contrary, Chen and Chen[Citation9] observed some different plateau temperatures in the freezing curves measured at different positions in samples of NaCl solutions. They observed that the plateau temperatures at lower positions were all smaller than the freezing point temperature of the sample. This result was explained by considering that the solvent near the measurement point was frozen very quickly to reach the initial freezing point. The calculated value for the initial freezing point was −1.69 ± 0.02°C for mango pulp (84.4% of water in wet basis); and −1.24 ± 0.05°C for papaya pulp (88.2% of moisture in wet basis). The initial freezing point for mango was slightly lower than for papaya possibly due to its high contents on soluble solids. These results agree with those obtained with others fruits.[Citation15] Foods consist of solid and water fractions and, as sensible heat is removed, the temperature of the mixture containing solids and water decreases. Just below the initial freezing point, water begins to convert into ice. As more heat is removed, more of the water converts into ice, and the remaining solution becomes more concentrated in terms of solids content. Because of the higher solids concentration, the temperature at which freezing will occur is depressed.[Citation15]
Thermal Conductivity
The performance of the coaxial dual-cylinder apparatus was checked for accuracy according the procedure indicated by Pongsawatmanit et al.[Citation16] that used pure water containing 0.5% agar to prevent the convection effect during experiments in the range of 0° to −30°C. An analysis of the influence of temperature difference across the sample on the measured thermal conductivity was conducted by varying the heat flux applied to the heater and the temperature of the water bath. Current values of 0.33, 0.96, 1.52, 2.08, 2.56, 3.14, and 3.84 ampere were applied, whereas bath temperatures of −20 and −10°C were tested to determine the optimum temperature difference. shows the observed temperature difference across the water sample and the measured thermal conductivity of ice as a function of the heat flux. A temperature difference between 0.8 and 3.2°C did not have a significant effect on the observed thermal conductivity for all range of heat flux (0.33 to 3.84 ampere). As expected, the increase of heat flux produced an increasing of temperature difference, but this behavior was independent of the bath temperature. The measured thermal conductivity of water is presented in , as well as a reference value reported by Valentas et al.[Citation17] Values of the standard deviation and standard error are also included. The experimental error found in the water thermal conductivity was less than 2%.
Figure 6 Effect of heat flux on thermal conductivity of ice and on temperature difference across the sample.
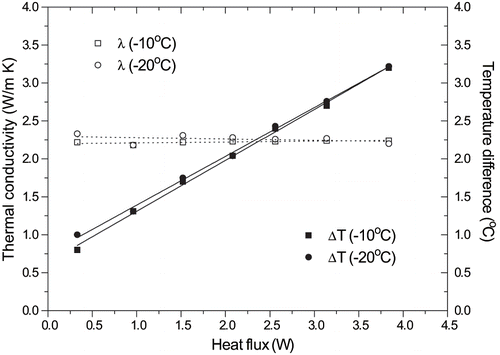
The results of the thermal conductivity determinations for mango and papaya pulps are presented in . Above the freezing point, thermal conductivity was almost independent of themperature for both pulps. In the frozen state, however, thermal conductivity was strongly affected by temperature, in such a way that thermal conductivity increased with decreasing temperature. This behavior is a consequence of the higher fraction of ice present in the samples exposed to temperatures well below their initial freezing point. Schwartzberg[Citation18] presented a mathematical study of the food freezing process and reported that thermal conductivity of ice is roughly 3.7 times as large as that of liquid water, which explains the marked increase in thermal conductivity of foods during freezing. Taking the whole range of temperatures considered the thermal conductivity at the lowest temperature was 2.7 and 2.5 times larger than that corresponding to the highest temperature for papaya and mango, respectively.
Table 2 Thermal conductivity of mango and papaya pulps at different temperatures
The thermal conductivity obtained by Shamsudin et al.[Citation19] for guava juice was not obviously affected by temperature varying from 30° to 80°C. According to Heldman and Lund,[Citation20] this thermal property of food product is mainly a function of water content and structure. The papaya pulp presented a little higher thermal conductivity in comparison to that obtained for mango pulp. This behavior could be attributed to the higher water content of papaya pulp and its smaller soluble solids content, which lead to a greater frozen water fraction at a given temperature and a consequent increase in thermal conductivity. It is well known from literature that thermal conductivity decreases with increasing food concentration.[Citation11,Citation19,Citation21,Citation22] According to Njie et al.,[Citation22] as the sugar-content increases, the quantity of free water in plant decreases, leading to a decrease in thermal conductivity. The values of frozen water fraction presented in were calculated by using EquationEq. 2:[Citation23]
where T and Tf are, respectively, the sample temperature and the initial freezing temperature (°C); Xice and XW are the mass fraction of ice (wet basis, dimensionless) and mass fraction of water (dimensionless), respectively.
The experimental results of thermal conductivity for mango and papaya pulps above the initial freezing point were well represented by EquationEqs. (3) and Equation(4), respectively, which were fitted to experimental data by non-linear regression (r2=0.996 for mango and 0.968 for papaya):
where λ is the thermal conductivity [W/mK]; and T is the sample temperature [°C]. On the other hand, the experimental results of thermal conductivity below freezing point could be described by the Fikiin equation,[Citation24] which was also fitted to experimental data by non-linear regression and resulted in a determination coefficient of 0.993 for mango (EquationEq. 5), and 0.884 for papaya (EquationEq. 6). The agreement between calculated and experimental data can be observed in and for mango and papaya pulp, respectively, at different temperatures.
Figure 7 Comparison between calculated and experimental data of thermal conductivity of mango pulps at different temperatures.
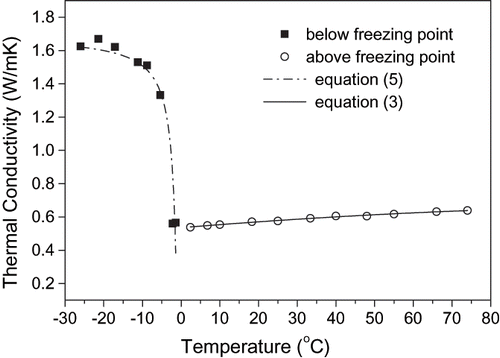
Figure 8 Comparison between calculated and experimental data of thermal conductivity of papaya pulps at different temperatures.
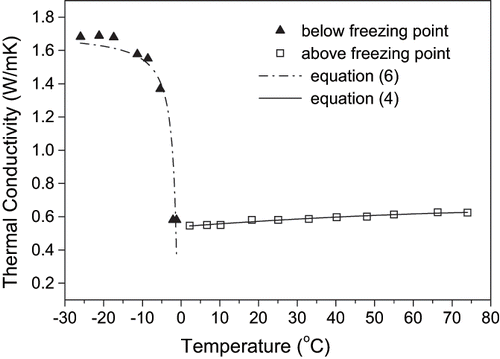
Thermal conductivity of food materials was selected from the literature and organized into a database by Krokida et al.[Citation25] They presented useful information about characteristics and statistics of this thermal property.
CONCLUSIONS
The measured values of initial freezing point were −1.69° ± 0.02°C and −1.24° ± 0.05°C for mango and papaya pulps, respectively. In the frozen state, the thermal conductivity decreased with increasing temperature. In general, papaya pulp presented a slightly higher thermal conductivity in comparison to values obtained for mango pulp, which could be attributed to differences in the composition of the fruit pulps. The mass fraction of ice in both pulps increased during freezing, causing an increase in thermal conductivity of the material due to the higher thermal conductivity of ice compared to that of liquid water.
ACKNOWLEDGMENTS
The authors wish to thank CNPq (Procs. 474626/2004-0 and 491504/2004-7) for financial support.
REFERENCES
- Manina , I. 1981 . Fruticultura Tropical 2: Manga , Italy : Campinas .
- Morton , J.F. 1987 . “ Papaya ” . In Fruits of Warm Climates , 336 – 346 . FL : Florida Flair Books, Boynton Beach .
- Delgado , A.E. and Sun , D-W. 2001 . Heat and Mass Transfer Models for Predicting Freezing Processes — A Review . Journal of Food Engineering , 47 : 157 – 174 .
- Di Matteo , P. , Donsí , G. and Ferrari , G. 2003 . The Role of Heat and Mass Transfer Phenomena in Atmospheric Freeze-Drying of Foods in a Fluidized Bed . Journal of Food Engineering , 59 : 267 – 275 .
- Lerici , C.R. , Piva , M. and Dallarosa , M. 1983 . Water Activity and Freezing Point Depression of Aqueous Solutions and Liquid Foods . Journal of Food Science , 48 : 1667 – 69 .
- Chen , P. , Chen , X.D. and Free , K.W. 1996 . Measurement and Data Interpretation of the Freezing Point Depression of Milks . Journal of Food Engineering , 30 ( 1–2 ) : 239 – 253 .
- 1990 . “ Association of Official Analytical Chemists ” . In Official Methods of Analysis , 1058 – 1059 . Washington, D.C. : AOAC .
- Pelegrine , D.H. , Silva , F.C. and Gasparetto , C.A. 2002 . Rheological Behaviour of Pineapple and Mango Pulps . Lebensmittel-Wissenschaft und-Technologie-Food Science and Technology , 35 ( 8 ) : 645 – 648 .
- Chen , X.D. and Chen , P. 1996 . Freezing of Aqueous Solution in a Simple Apparatus Designed for Measuring Freezing Point . Food Research International , 29 ( 8 ) : 723 – 729 .
- Bellet , D. , Sangelin , M. and Thirriot , C. 1975 . Determination des Proprietés Themophysiques de Liquides Non-Newtoniens a L'áide D'une Cellule a Cylindres Coaxiaux . Int. J. Heat Mass Transfer , 18 ( 10 ) : 1177 – 1187 .
- Telis-Romero , J. , Telis , V.R.N. , Gabas , A.L. and Yamashita , F. 1998 . Thermophysical Properties of Brazilian Orange Juice as Affected by Temperature and Water Content . Journal of Food Engineering , 38 ( 1 ) : 27 – 40 .
- Telis-Romero , J. , Gabas , A.L. , Polizelli , M.A. and Telis , V.R.N. 2000 . Temperature and Water Content Influence on Thermophysical Properties of Coffee Extract . International Journal of Food Properties , 3 ( 3 ) : 375 – 384 .
- Gabas , A.L. , Marra-Júnior , W.D. , Telis-Romero , J. and Telis , V.R.N. 2005 . Changes of Density, Thermal Conductivity, Thermal Diffusivity and Specific Heat of Plums During Drying . International Journal of Food Properties , 8 ( 2 ) : 233 – 242 .
- Perry , R. and Chilton , C. 1986 . Manual de Engenharia Química , 5th Edited by: Guanabara , D. Rio de Janeiro
- Singh , R.P. 1995 . “ Thermal properties of Frozen Foods ” . In Engineering Properties of Foods , Edited by: Rao , M.A. and Rizvi , S.S.H. 139 – 167 . New York : Marcel Dekker .
- Pongsawatmanit , R. , Miyawaki , O. and Yano , T. 1993 . Measurement of the Thermal Conductivity of Unfrozen and Frozen Food Materials by a Steady State Method with Coaxial Dual-Cylinder Apparatus . Biosci. Biotech. Biochem. , 57 ( 7 ) : 1072 – 1076 .
- Valentas , K.J. , Rotstein , E. and Singh , R.P. 1999 . Handbook of Food Engineering Practice , 716 Boca Raton, FL : CRC Press .
- Schwartzberg , H.G. August 1981 . Mathematical Analysis of the Freezing and Thawing of Foods , August , 1 – 33 . Detroit, MI : AICHE Summer Meeting .
- Shamsudin , R. , Mohamed , I.O. and Yaman , N.K.M. 2005 . Thermophysical Properties of Thai Seedless Guava Juice as Affected by Temperature and Concentration . Journal of Food Engineering , 66 : 395 – 399 .
- Heldman , D.R. and Lund , D.B. 1992 . “ Food Freezing. ” . In Handbook of Food Engineering , Edited by: Heldman , D.R. and Lund , D.B. 756 New York : Marcel Dekker, Inc .
- Sweat , V.E. 1995 . “ Thermal Properties of Foods ” . In Engineering Properties of Foods , 2nd , Edited by: Rao , M.A. and Rizvi , S.S.H. 99 – 138 . New York : Marcel Dekker, Inc .
- Njie , D.N. , Rumsey , T.R. and Singh , R.P. 1998 . Thermal Properties of Cassava, Yam and Plantain . Journal of Food Engineering , 37 : 63 – 76 .
- Miles , C.A. , Van Beek , G. and Veerkamp , C.H. 1983 . “ Calculation of Thermophysical Properties of Foods ” . In Physical Properties of Foods , Edited by: Jowitt , R. , Escher , F. , Hallström , B. , Meffert , H.F. Th. , Spiess , W.E.L. and Vos , G. 269 – 312 . London and New York : Applied Science Publishers .
- Gabas , A.L. , Telis-Romero , J. and Telis , V.R.N. 2003 . Influence of Fluid Concentration on Freezing Point Depression and Thermal Conductivity of Orange Juice . International Journal of Food Properties , 6 ( 3 ) : 543 – 556 .
- Krokida , M.K. , Michailidis , P.A. , Maroulis , Z.B. and Saravacos , G.D. 2002 . Literature Data of Thermal Conductivity of Foodstuffs . International Journal of Food Properties , 5 ( 1 ) : 63 – 111 .