Abstract
The effect of five different emulsifying salts on the texture of processed cheese was studied using two different chemical compositions, one that corresponds to block-type products and another that gives spreadable-type samples. Texture profile analysis and stress relaxation tests were applied to the block-type processed cheeses, while the spreadable-type samples were subjected to oscillatory shear test. Measurements of the apparent viscosity of processed cheese during processing were carried out, using a capillary viscometer, in order to relate it with the texture of the final product. Trisodium citrate and disodium phosphate exhibited similar behavior and produced the softest, most adhesive, least elastic, and most liquid-like final products that showed reduced apparent viscosity during processing. Tetrasodium pyrophosphate gave harder, less adhesive, more elastic, and more solid-like samples, with higher apparent viscosity than pentasodium tripolyphosphate. Sodium hexametaphosphate produced cheeses that exhibited the lowest apparent viscosity during processing and the highest textural (except adhesiveness and stringiness) and viscoelastic properties (stress relaxation test).
INTRODUCTION
For the production of processed cheese the most commonly used emulsifying salts are citrates and phosphates. Although these compounds are not emulsifiers in the strict chemical sense, their essential role is to supplement the emulsifying capacity of the aggregated paracaseinate in natural cheese, with the aid of heat and shear. This is accomplished with the disruption of the casein micelles, by chelating the calcium which is required in maintaining micellar structure. Therefore, caseins are more soluble and capable to immobilize water and emulsify fat. Furthermore, emulsifying salts shift the pH of the cheese blend upward and stabilize it, resulting in a more open and reactive paracaseinate conformation with superior water-binding and emulsifying properties.[Citation1,Citation2,Citation3] Each emulsifying salt differs from the others in its sequestering ability, buffering capacity, ability to hydrate and disperse caseins, and effectiveness in promoting emulsification.[Citation3]
The use of different emulsifying salts can affect not only the flow properties[Citation1,Citation4,Citation5,Citation6] but also the texture[Citation7,Citation8,Citation9,Citation10,Citation11,Citation12,Citation13] of processed cheese. The behavior of each emulsifying salt can be interpreted by knowing its ability to hydrate and disperse proteins. The latter is evaluated by the determination of the peptization coefficient.[Citation6,Citation14] The effect of emulsifying salts on the textural properties of processed cheese has been studied using texture profile analysis (TPA)[Citation9,Citation12] and different penetration[Citation8,Citation10] and cutting tests.[Citation7] The viscoelastic properties of processed cheese, as affected by the use of two different emulsifying salts (trisodium citrate and disodium phosphate) have been studied by Sutheerawattananonda and Bastian,[Citation11] using dynamic rheology. The evaluation of the viscoelastic behavior of processed cheese as a function of emulsifying salts, using stress relaxation test, has not been reported in the literature yet.
Although many investigations have been carried out on the study of the texture of processed cheese, the evaluation of the relationship with its apparent viscosity during processing is yet limited.[Citation13] The objective of the present work was to study the effect of five different emulsifying salts on the texture of processed cheese. This was accomplished using two different chemical compositions. The first one gave block-type processed cheeses, while the second one resulted in spreadable-type samples. The textural and viscoelastic properties of the block-type processed cheeses were studied by the use of TPA and stress relaxation tests, respectively. Dynamic rheology was applied to the spreadable-type samples for the evaluation of their viscoelastic behavior. The determination of the apparent viscosity of processed cheese during processing was made in order to relate it to the textural and viscoelastic properties of the final product.
MATERIALS AND METHODS
Manufacture of Processed Cheese
A mixture of 75.5 g three-month-old Gouda cheese (41.9% moisture; 51.2% fat in dry matter; 21.8% proteins), 16.1 g water, 5.9 g skimmed milk powder (6.0% moisture; 0.5% fat; 34.5% proteins), and 2.5 g emulsifying salt (on an anhydrous base) were used for the manufacture of the block-type processed cheese samples. For the spreadable-type products the raw materials were 69.4 g Gouda cheese, 21.6 g water, 6.5 g butter (15.3% moisture; 82.5% fat), and 2.5 g emulsifying salt (on an anhydrous base). Trisodium citrate, disodium phosphate, tetrasodium pyrophosphate, pentasodium tripolyphosphate and sodium hexametaphosphate (BDH, England) were chosen, based on common usage, to be used as emulsifying salts (all were of the analytical grade). The use of salt mixtures was avoided so as to evaluate the action of each one separately. The final pH value of the products was affected only by the use of the different emulsifying salts and not by extraneous factors (addition of acidic or alkaline solutions).
Both block-type and spreadable-type samples were manufactured inside the sample vessel of a tube viscometer (TR−1 Rheometer, A.T.E.I., Thessaloniki, Greece) that has already been used for the evaluation of the apparent viscosity of processed cheese during processing.[Citation6,Citation15] The processed cheese samples were produced at 80°C, as described by Dimitreli et al.[Citation6] and Dimitreli and Thomareis.[Citation13] The preparation of the samples for the textural evaluation and oscillatory shear test was made as described by Dimitreli and Thomareis[Citation13] and Dimitreli and Thomareis,[Citation16] respectively.
Determination of Processed Cheese Apparent Viscosity
Three capillary tubes of different dimensions (2.16, 1.27, and 1.02 mm in diameter; 52, 55, and 56 mm in height, respectively), applicable to the viscometer, were used for the determination of the apparent viscosity of processed cheese. Preliminary viscosity measurements were carried out, with the procedure that is described by Dimitreli and Thomareis,[Citation15] showing that slippage is no evident in the tube viscometer. The apparent viscosity of processed cheese samples was measured at 80°C, as described by Dimitreli and Thomareis,[Citation15] and their consistency index was determined. The measurements were made in triplicate.
Textural Evaluation of the Block-Type Processed Cheese
Textural evaluation of the block-type processed cheese samples was made using an Instron Universal Testing Machine, model 1140. The TPA test was used for the study of their textural properties, while the stress relaxation test was applied to the samples in order to evaluate their viscoelastic behavior. The experimental conditions, as well as the determined properties, were the same as described by Dimitreli and Thomareis.[Citation13] The values of the textural and viscoelastic properties were the means of at least six replications.
Oscillatory Shear Test on the Spreadable-Type Processed Cheese
The Bohlin C-VOR 150 rheometer was used for the dynamic rheological measurements of the spreadable-type processed cheese samples. Preliminary experiments were carried out to determine the linear viscoelastic region. The experimental conditions were as described by Dimitreli and Thomareis (2008). Elastic (G′) and viscous (G″) moduli, loss tangent (tan δ), complex modulus (G*) and complex viscosity (η*) were determined. Measurements were made in triplicate.
Chemical Analysis
Chemical analyses of the raw materials and processed cheese samples involved the determination of moisture, fat, proteins, pH,[Citation16] and peptization coefficient[Citation6]. Chemical measurements of the samples were made in triplicate.
Statistical Analysis
The General Linear Model (One-way ANOVA) was used for the statistical analysis of the experimental data, while the Student-Newman-Keuls (SNK) multiple comparison test[Citation17] determined whether statistically significant differences occurred among means. All differences considered as significant are at least P < 0.05. The statistical analysis of the experimental data was performed, using Minitab 13.0 statistical software.[Citation18]
RESULTS AND DISCUSSION
Textural and Viscoelastic Properties of the Block-Type Processed Cheese
The moisture, fat and protein content of the block-type processed cheese samples made with the five different emulsifying salts were 48.1 ± 0.1%, 22.5 ± 0.0%, and 18.2 ± 0.1%, respectively. The peptization coefficient and pH values of the samples are shown in .
Table 1 Peptization coefficient and pH values of the block- and spreadable-type processed cheese samples made with different emulsifying salts (the standard deviations are given in parenthesis)
Texture profile analysis
shows a typical Instron texture profile curve for block-type processed cheese. Fracturability, defined by an initial yield peak at the force-distance curve of the samples, does not characterize the rheological behavior of processed cheese, as have already been mentioned by Dimitreli and Thomareis.[Citation13] The textural properties of the block-type processed cheese samples that derived from the TPA curves are shown in . According to one-way ANOVA, all textural properties, except cohesiveness, showed statistically significant differences (P < 0.05) among the samples.
Figure 1 Typical Instron texture profile curve for block-type processed cheese. H1: hardness 1 (peak: force); H2: hardness 2 (peak: force); A1: work done on the sample during the first bite (area: work); A2: work done on the sample during the second bite (area: work); A3: adhesiveness (area: work); S1: springiness (distance); and S2: stringiness (distance).
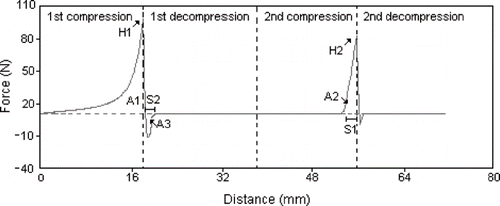
Table 2 Average values of the textural properties of the block-type processed cheese samples made with different emulsifying salts that derived from the TPA test (the standard deviations are given in parenthesis)
Sodium hexametaphosphate exhibited significantly the highest values for modulus of deformability, hardness 1 and 2, work for hardness 1 and 2, gumminess and chewiness, while trisodium citrate and disodium phosphate gave significantly the lowest (P < 0.05). No significant differences between trisodium citrate and disodium phosphate were obtained for all textural properties. Pentasodium tripolyphosphate exhibited significantly higher values than trisodium citrate and disodium phosphate, and significantly lower values than tetrasodium pyrophosphate for all the above-mentioned properties, except chewiness. For chewiness, tetrasodium pyrophosphate and pentasodium tripolyphosphate showed no significant difference. Sodium hexametaphosphate gave significantly the highest value for springiness: no significant differences between the other emulsifying salts were obtained.
The results are in agreement with those of Carić et al.[Citation19] and Cavalier-Salou and Cheftel,[Citation10] who reported that trisodium citrate and disodium phosphate exhibited similar effects and produced the softest processed cheese, whereas polyphosphates gave considerably harder cheeses. Thomas et al.[Citation8] and Rayan et al.[Citation7] also found that processed cheese samples made with sodium citrate and disodium phosphate were softer compared to samples made with tetrasodium pyrophosphate. Though, Thomas et al.[Citation8] reported that disodium phosphate lead to the manufacture of harder cheeses than trisodium citrate did, but similar with pentasodium tripolyphosphate. According to Shimp[Citation20], trisodium citrate and disodium phosphate gave soft and easily melted cheeses, while pentasodium tripolyphosphate and tetrasodium pyrophosphate produce hard and non-melting cheeses. The sequestering, dispersing and swelling ability of trisodium citrate and disodium phosphate was lower compared to that of the other emulsifying salts. The processed cheese samples made with these two emulsifying salts exhibited low values of peptization coefficient that showed no significant differences between them (). This means that trisodium citrate and disodium phosphate have the same effectiveness in promoting emulsification, which is lower compared to that of polyphosphates. Thus, this result in weak emulsification where the fat globules are large and suspended in the protein-containing phase. When pressure is applied, the fat deforms, the proteins readily follow and the cheese appears soft. On the other hand, polyphosphates are much more effective calcium binders. In fact, the increase in phosphorous anions in the chain results in higher peptization coefficients,[Citation6,Citation14] as shown in , and thus very good ability in promoting emulsification. These salts (polyphosphates) gave strong emulsification where the fat globules were very tiny and were almost lost in the protein network of the aqueous phase. The proteins linked up and form a structure of their own; the structural role of fat was greatly reduced. Such cheese tends to be hard and non-melting.
Though pentasodium tripolyphosphate gave significantly the highest value of peptization coefficient, tetrasodium pyrophosphate showed significantly higher values for modulus of deformability, hardness 1 and 2, work for hardness 1 and 2 and gumminess than pentasodium tripolyphosphate (P < 0.05) did. Thomas et al.[Citation8] also reported that processed cheese samples made with tetrasodium pyrophosphate were firmer compared to those made with pentasodium tripolyphosphate. This may be attributed to the stronger moisture absorbing action of pyrophosphates; when used alone they lead to overcreaming and to a hardening of texture.[Citation1] Tetrasodium pyrophosphate can permeate more easily between protein molecules and peptize them to a greater degree. The creaming action is characterized by the peptizing effect of emulsifying salts on the casein molecules and by the hydration of these molecules. When casein dispersion and hydration are intensive, the creaming action takes place very quickly, resulting in products with increased hardness. In contrast, Cavalier-Salou and Cheftel[Citation10] found that pentasodium tripolyphosphate produced processed cheese analogues with higher values of firmness than tetrasodium pyrophosphate did.
Though sodium hexametaphosphate gave the lowest value for peptization coefficient, it led to processed cheese with the highest textural properties (P < 0.05). This is due to the low pH of the sample. Fox et al.[Citation3] reported that low pH caused by sodium hexametaphosphate produces short, dry and crumbly cheeses. According to Marchesseau et al.[Citation21] and Lee and Klostermeyer,[Citation22] when the pH is increased, hardness is reduced. The authors support that the observed change in rheological properties with pH may be due to charge interaction effects. The increasing pH resulted in increasing charge repulsion between proteins, causing greater hydration of caseins. This higher sorption of water by the proteins was thought to result in a softer processed cheese at high pH. This is consistent with the notion that water acts as a plasticizer.
In contrast to the results of all the above authors, Gupta et al.[Citation9] reported that the firmness of processed cheese increased in the following order: tetrasodium pyrophosphate < trisodium citrate < disodium phosphate < sodium hexametaphosphate < pentasodium tripolyphosphate. In the present study, trisodium citrate and disodium phosphate exhibited significantly the highest values for adhesiveness and stringiness, while sodium hexametaphosphate gave the lowest (P < 0.05). No significant differences were obtained for tetrasodium pyrophosphate and pentasodium tripolyphosphate. According to Bryant et al.,[Citation23] who studied the texture of Cheddar cheese, the most adhesive cheeses were those containing an open and loose protein matrix. The weak emulsification produced by trisodium citrate and disodium phosphate may be the cause for the formation of such a matrix. As the protein matrix becomes increasingly more compact the cheese looses its adhesiveness,[Citation23] as in the case of the strong emulsification produced by tetrasodium pyrophosphate and pentasodium tripolyphosphate. The reduced solubility of the proteins due to low pH, caused by sodium hexametaphosphate also results in the formation of a very compact matrix with reduced adhesiveness.
The values of consistency index for the block-type processed cheese samples are shown in . Generally, as it can be seen emulsifying salts that give processed cheeses with increased values of consistency index and thus apparent viscosity, will exhibit high textural properties (except for adhesiveness and stringiness) in the final product and vice versa. Emulsifying salts that produce soft and adhesive processed cheeses, like trisodium citrate and disodium phosphate, disperse and hydrate proteins to a lesser degree compared to the others. The protein matrix did not have open structure and thus protein molecules showed reduced resistance to flow and low apparent viscosity. In contrast, tetrasodium pyrophosphate and pentasodium tripolyphosphate that produce hard and less adhesive cheeses are effective in dispersing and hydrating caseins, resulting in molecules with an open conformation, increased resistance to flow and thus high apparent viscosity.
Table 3 The means of the consistency index of the block- and spreadable-type processed cheese samples made with different emulsifying salts (the standard deviations are given in parenthesis)
Although sodium hexametaphosphate showed the lowest value of apparent viscosity during processing, it produced the hardest final product. This is due to the low pH of the sample. There is a different effect of pH on the apparent viscosity of the hot cheese melt during processing and on the texture of the final product. Low values of pH close to the isoelectric point of caseins result in reduced hydration of the protein molecules, which do not have an open conformation and their resistance to flow is greatly reduced. The apparent viscosity of such an emulsion will show low values. On the contrary, if the protein molecules are not well hydrated, the water does not disturb their network, being only trapped between molecules, and the final product appears to be hard.
Stress relaxation test
During stress relaxation, the applied force decayed to a residual value after a finite time (15 min), indicating that the processed cheese behaves as viscoelastic solid. Experimental relaxation data were fitted to the generalized Maxwell model. The number of necessary terms for a satisfactory fit was determined, obtaining four Maxwellian elements in parallel with a pure elastic element. The relaxation curves were expressed as relaxation functions:
where E(t) is the elastic modulus at time t, E∞ is the equilibrium modulus of the pure elastic element, E1, E2, E3 and E4 are the elastic moduli of the first, second, third and fourth element, respectively, and τ1, τ2, τ3 and τ4 are the relaxation times of each Maxwellian element. Coefficients of viscosity (ηi) of the samples in the dashpot of each element were calculated from the elastic moduli and relaxation times as follows[Citation24]:
Joshi et al.[Citation25] performed a stress relaxation test on processed cheeses made from concentrated milk and suggested a generalized Maxwell model of three exponential components with no residual term. In contrast, Dimitreli and Thomareis[Citation13] proposed a model of four Maxwellian elements in parallel with a pure elastic element, so as to characterize the stress relaxation behavior of processed cheese made with different chemical composition.
The viscoelastic properties of the processed cheese samples are shown in . Trisodium citrate and disodium phosphate showed significantly the lowest values for the equilibrium modulus, the four elastic moduli and the four viscosity coefficients (P < 0.05). No significant differences were noticed between trisodium citrate and disodium phosphate. Sodium hexametaphosphate showed significantly the highest values for the above-mentioned properties. Whereas, tetrasodium pyrophosphate gave significantly lower values than sodium hexametaphosphate and higher than pentasodium tripolyphosphate. Sodium hexametaphosphate exhibited significantly the highest values for the four relaxation times. Tetrasodium pyrophosphate showed significantly lower values than sodium hexametaphosphate and higher values than the other emulsifying salts also for the four relaxation times. Trisodium citrate, disodium phosphate, and pentasodium tripolyphosphate gave significantly the lowest values for the second, third and fourth relaxation times. For the first relaxation time, pentasodium tripolyphosphate exhibited significantly higher values than trisodium citrate and disodium phosphate. Increased values of elastic modulus and coefficient of viscosity indicate that processed cheese becomes harder. According to Rha,[Citation26] the higher the relaxation time, the more elastic the material. This means that the processed cheese sample made with sodium hexametaphosphate is harder (high values of elastic modulus and coefficient of viscosity) and more elastic (high values of relaxation time) than those made with the other emulsifying salts. Results from the stress relaxation test are in a good agreement with those of the TPA, in relation to the effect of each emulsifying salt on the texture of processed cheese.
Table 4 Average values of the viscoelastic properties of the block-type processed cheese samples made with different emulsifying salts resulted from the stress relaxation test (the standard deviations are given in parenthesis)
The relationship between the consistency index of the processed cheese samples and their viscoelastic properties was the same with that of the consistency index and their textural properties. Processed cheeses made with trisodium citrate and disodium phosphate showed decreased values of apparent viscosity and low values of viscoelastic properties. Tetrasodium pyrophosphate and pentasodium tripolyphosphate produced viscous samples during processing and hard and elastic final products. In contrast, sodium hexametaphosphate exhibited decreased apparent viscosity but increased viscoelastic properties.
Viscoelastic Properties of the Spreadable-Type Processed Cheese
The moisture, fat and protein content of the spreadable-type processed cheese samples made with the different emulsifying salts were 51.5 ± 0.1%, 25.5 ± 0.0% and 13.4 ± 0.0%, respectively. The peptization coefficient and pH values of the samples are shown in .
Under the conditions of the oscillatory shear test, the viscoelastic properties of the processed cheese manufactured with sodium hexametaphosphate could not be determined. This is due to the high firmness of this sample.
The changes of the elastic and viscous moduli, as a function of frequency, for the processed cheese samples made with the other four emulsifying salts, are shown in and are typical of a viscoelastic material. Similar behavior has been reported by Lee and Klostermeyer,[Citation22] Lee et al.,[Citation27] and Dimitreli and Thomareis,[Citation16] who studied the effect of different factors on the viscoelastic properties of processed cheese spreads. Both elastic and viscous moduli increase with increasing frequency. The viscous modulus is larger than the elastic modulus at lower frequencies but the opposite occurs at higher frequencies. The cross-over of G″ and G′ is a useful mean of evaluating the transition from liquid-like to solid-like behavior and it is characteristic of a concentrated solution.[Citation28] This means that processed cheese samples made with different emulsifying salts behave as concentrated macromolecular solutions. The cross-over point occurred at lower frequencies when tetrasodium pyrophosphate and pentasodium tripolyphosphate were used as emulsifying salts. The lowest cross-over frequency was shown with tetrasodium pyrophosphate, meaning that this sample exhibited the most solid-like behavior, and thus it is the most difficult to spread. The cross-over point of processed cheese samples made with trisodium citrate and disodium phosphate appeared at almost the same higher frequency. This means that these samples showed more liquid-like behavior and thus better spreadability, compared with the other two made with tetrasodium pyrophosphate and pentasodium tripolyphosphate.
Figure 2 Frequency sweep for the spreadable-type processed cheese samples made with trisodium citrate and disodium phosphate (shear stress 3 Pa, temperature 28°C). TSC: trisodium citrate; DSP: disodium phosphate; G′: elastic modulus; and G″: viscous modulus.
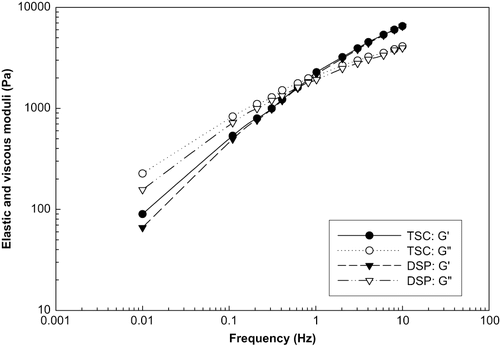
Figure 3 Frequency sweep for the spreadable-type processed cheese samples made with tetrasodium pyrophosphate and pentasodium tripolyphosphate (shear stress 3 Pa, temperature 28°C). TSPP: tetrasodium pyrophosphate; STPP: pentasodium tripolyphosphate; G′: elastic modulus; and G″: viscous modulus.

The loss tangent gave also an indication of whether elastic or viscous properties of processed cheese predominated (). High values of tan δ at low frequencies indicate a tendency toward liquid-like behavior; tan δ decreased with more solid-like behavior at high frequencies.[Citation28] The loss tangent values showed a predominantly viscous behavior (tan δ > 1 or G″ > G′) for all the samples at frequencies lower than their cross-over point. The lower values of tan δ over the whole range of frequency tested were shown by tetrasodium pyrophosphate and the higher by trisodium citrate and disodium phosphate.
Figure 4 Loss tangent as a function of frequency for the spreadable-type processed cheese samples (shear stress 3 Pa, temperature 28°C). TSC: trisodium citrate; DSP: disodium phosphate; TSPP: tetrasodium pyrophosphate; and STPP: pentasodium tripolyphosphate.
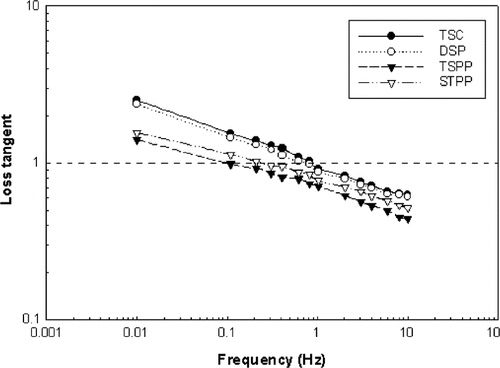
Complex modulus (Pa) describes the total resistance to deformation of a material that it is considered as an elastic solid. In contrast, complex viscosity (Pa s) describes the total resistance to flow of a material that it is considered as a viscous liquid. and show G* and η* of processed cheese samples, respectively, as a function of frequency. The processed cheese samples made with tetrasodium pyrophosphate and pentasodium tripolyphosphate showed increased values of complex modulus and complex viscosity over the entire frequency range (due to higher resistance), compared to those made with trisodium citrate and disodium phosphate (lower resistance). The complex modulus increases while the complex viscosity decreases across the frequency range. Trisodium citrate and disodium phosphate showed similar behavior and exhibited samples with more liquid-like behavior compared to those made with tetrasodium pyrophosphate and pentasodium tripolyphosphate that showed more solid-like behavior.
Figure 5 Complex modulus as a function of frequency for spreadable-type processed cheese samples (shear stress 3 Pa, temperature 28°C). TSC: trisodium citrate; DSP: disodium phosphate; TSPP: tetrasodium pyrophosphate; and STPP: pentasodium tripolyphosphate.
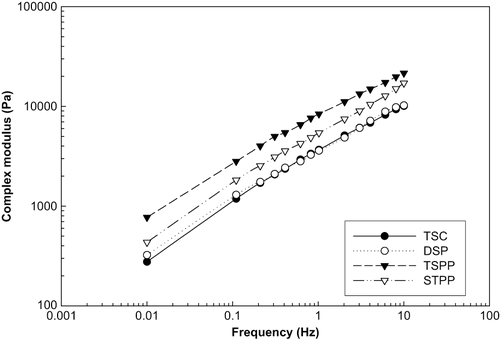
Figure 6 Complex viscosity as a function of frequency for spreadable-type processed cheese samples (shear stress 3 Pa, temperature 28°C). TSC: trisodium citrate; DSP: disodium phosphate; TSPP: tetrasodium pyrophosphate; and STPP: pentasodium tripolyphosphate.
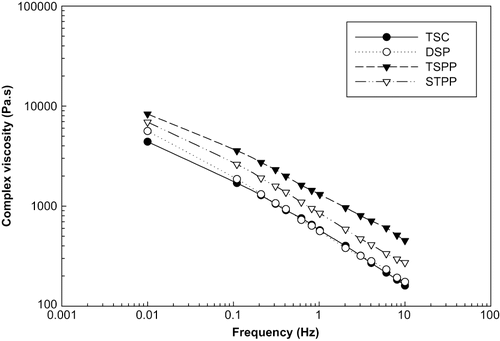
For the purpose of comparing values of G′, G″, tan δ, G*, and η* of the different samples, a reference oscillation frequency of 10 Hz was chosen. The values of the viscoelastic properties at 10 Hz frequency are shown in . The samples made with trisodium citrate and disodium phosphate exhibited similar values which were the lowest for G′, G″, G*, and η* and the highest for tan δ, confirming their liquid-like behavior. The highest values for G′, G″, G*, and η* and the lowest for tan δ were recorded for the sample made with tetrasodium pyrophosphate. In contrast to the results of the present study, Sutheerawattananonda and Bastian[Citation11] found that the elastic modulus of processed cheese made with disodium phosphate was usually 20 to 60% higher than that made with trisodium citrate.
Table 5 The means of elastic modulus (G′); viscous modulus (G″); loss tangent (tan δ); complex modulus (G*); and complex viscosity (η*) of the spreadable-type processed cheese samples at 10 Hz frequency (the standard deviations for viscoelastic properties are given in parenthesis)
The consistency index values of the samples made with different emulsifying salts are shown in . Excluding the sample made with sodium hexametaphosphate that gave the lowest apparent viscosity during processing but its viscoelastic properties could not be determined using oscillatory shear test, processed cheese samples produced with trisodium citrate and disodium phosphate exhibited the most liquid-like behavior and the lowest values for consistency index during processing. The sample made with tetrasodium pyrophosphate, which exhibited the most solid-like behavior, gave significantly the highest value for consistency index. Processed cheese sample produced with pentasodium tripolyphosphate had a lower value for consistency index and also showed less solid-like behavior than tetrasodium pyrophosphate did.
CONCLUSIONS
In the case of block-type processed cheeses, the sample made with sodium hexametaphosphate was the hardest, least adhesive and most elastic, although its apparent viscosity during processing showed the lowest value. Trisodium citrate and disodium phosphate had similar effect on the texture of processed cheese. The samples made with these emulsifying salts were the softest, most adhesive and least elastic, while their apparent viscosity during processing was reduced. Tetrasodium pyrophosphate gave harder, less adhesive, more elastic samples, with a higher apparent viscosity than pentasodium tripolyphosphate did. As it concerns spreadable-type processed cheeses, under the conditions of the oscillatory shear test, the viscoelastic properties of the sample manufactured with sodium hexametaphosphate could not be determined due to its high firmness. The other four emulsifying salts exhibited similar effect as in the case of block-type processed cheeses. Tetrasodium pyrophosphate produced cheeses with more solid-like behavior and higher apparent viscosity during processing than pentasodium tripolyphosphate did. In contrast, trisodium citrate and disodium phosphate showed similar behavior and gave processed cheese samples with the most liquid-like behavior and the lowest apparent viscosity (excluding the sample made with sodium hexametaphosphate).
ACKNOWLEDGMENTS
The authors would like to thank the Ministry of National Education & Religious Affairs of Greece and the European Union (EU) which co-funded this work by 25% and 75%, respectively (Archimedes research program). Special thanks are also expressed to Dr S. Raphaelides for his valuable assistance during experiments.
REFERENCES
- Meyer , A. 1973 . Processed cheese manufacture , 329 London : Food Trade Press .
- Carić , M. and Kaláb , M. 1987 . “ Processed cheese products ” . In Cheese: chemistry, physics and microbiology. Volume 2. Major cheese groups , Edited by: Fox , P.F. 339 – 383 . London , New York : Elsevier Applied Science .
- Fox , P.F. , Guinee , T.P. , Cogan , T.M. and McSweeney , P.L.H. 2000 . Fundamentals of cheese science , 587 Gaithersburg , , Maryland : Aspen Publishers, Inc .
- Schulz , M.E. and Hetzel , H.F. 1960 . Untersuchungen über Maßnahmen zur Standardisierung des Schmelzprozesses bei der Herstellung von Schmelzkäse, I. Mitteilung Schmelzdiagramme und Schmelzkreuz . Milchwissenschaft , 15 : 1 – 7 .
- Kirchmeier , O. , Weiss , G. and Kiermeier , F. 1978 . Fliessverhalten von Schmelzkäsen unter der Wirkung verschiedener Phosphate . Zeitschrift für Lebensmittel-Untersuchung und Forschung , 166 ( 4 ) : 212 – 220 .
- Dimitreli , G. , Thomareis , A.S. and Smith , P.G. 2005 . Effect of emulsifying salts on casein peptization and apparent viscosity of processed cheese . International Journal of Food Engineering , 1 ( 4 ) : 1 – 17 . article 2
- Rayan , A.A. , Kaláb , M. and Ernstrom , C.A. 1980 . Microstructure and rheology of process cheese . Scanning Electron Microscopy , 3 : 635 – 644 .
- Thomas , M.A. , Newell , G. , Abad , G.A. and Turner , A.D. 1980 . Effect of emulsifying salts on objective and subjective properties of processed cheese . Journal of Food Science , 45 : 458 – 459 .
- Gupta , S.K. , Karahadian , C. and Lindsay , R.C. 1984 . Effect of emulsifier salts on textural and flavor properties of processed cheeses . Journal of Dairy Science , 67 : 764 – 778 .
- Cavalier-Salou , C. and Cheftel , J.C. 1991 . Emulsifying salts influence on characteristics of cheese analogs from calcium caseinate . Journal of Food Science , 56 : 1542 – 1547 .
- Sutheerawattananonda , M. and Bastian , E.D. 1998 . Monitoring process cheese meltability using dynamic stress rheometry . Journal of Texture Studies , 29 : 169 – 183 .
- Awad , R.A. , Abdel-Hamid , L.B. , El-Shabrawy , S.A. and Singh , R.K. 2002 . Texture and microstructure of block type processed cheese with formulated emulsifying salt mixtures . Lebensmittel-Untersuchung und Forschung , 35 : 54 – 61 .
- Dimitreli , G. and Thomareis , A.S. 2007 . Texture evaluation of block-type processed cheese as a function of chemical composition and in relation to its apparent viscosity . Journal of Food Engineering , 79 : 1364 – 1373 .
- Lee , B.O. , Paquet , D. and Alais , C. 1986 . Etude biochimique de la fonte des fromages. IV . Effet du type de sels de fonte et de la nature de la matière protéique sur la peptization. Utilisation d'un système modèle. Lait , 66 : 257 – 267 .
- Dimitreli , G. and Thomareis , A.S. 2004 . Effect of temperature and chemical composition on processed cheese apparent viscosity . Journal of Food Engineering , 64 : 265 – 271 .
- Dimitreli , G. and Thomareis , A.S. 2008 . Effect of chemical composition on the linear viscoelastic properties of spreadable-type processed cheese . Journal of Food Engineering , 84 : 368 – 374 .
- Hocking , R.R. 1996 . Methods and applications of linear models. Regression and the analysis of variance , 731 New York , Chichester : John Wiley & Sons, Inc . Weinheim, Brisbane, Singapore, Toronto
- Minitab . 2000 . Minitab Reference Manual , Pennsylvania : Minitab, Inc .
- Carić , M. , Gantar , G. and Kaláb , M. 1985 . Effects of emulsifying agents on the microstructure and other characteristics of processed cheese – A review . Food Microstructure , 4 : 297 – 312 .
- Shimp , L. 1985 . Process cheese principles . Food Technology , 39 : 63 – 69 .
- Marchesseau , S. , Gastaldi , E. , Lagaude , A. and Cuq , J.-L. 1997 . Influence of pH on protein interactions and microstructure of process cheese . Journal of Dairy Science , 80 : 1483 – 1489 .
- Lee , S.K. and Klostermeyer , H. 2001 . The effect of pH on the rheological properties of reduced-fat model processed cheese spreads . Lebensmittel-Wissenschaft und Technologie , 34 : 288 – 292 .
- Bryant , A. , Ustunol , Z. and Steffe , J. 1995 . Texture of Cheddar cheese as influenced by fat reduction . Journal of Food Science , 60 : 1216 – 1219 .
- Rao , V.N.M. 1992 . “ Classification, description and measurement of viscoelastic properties of solid foods ” . In Viscoelastic properties of foods , Edited by: Rao , M.A. and Steffe , J. F. 3 – 47 . New York : Elsevier Applied Science: London .
- Joshi , N.S. , Jhala , R.P. , Muthukumarappan , K. , Acharya , M.R. and Mistry , V.V. 2004 . Textural and rheological properties of processed cheese . International Journal of Food Properties , 7 : 519 – 530 .
- Rha , C.K. 1979 . Viscoelastic properties of foods as related to micro- and molecular structures . Food Technology , 33 : 71 – 76 .
- Lee , S.K , Anema , K. and Klostermeyer , H. 2004 . The influence of moisture content on the rheological properties of processed cheese spreads . International Journal of Food Science and Technology , 39 : 763 – 771 .
- Steffe , J.F. 1996 . Rheological methods in food process engineering , 411 East Lansing : Freeman Press .