Abstract
The influence of high intensity ultrasound on the rheological characteristics of guar, xanthan, and pectin dispersions was investigated. Guar 1%, xanthan 1% and pectin 2% (w/v) dispersions were sonicated at varying intensity levels of 3.7, 6.3, 8.1, and 10.1 W/cm2 for 5 min at 25oC. Significant differences were observed in the rheological characteristics of each sonicated hydrocolloid dispersion studied. Sonication affected the consistency index (k), flow behaviour index (n) and complex power law constants (a and b). No recovery was observed in the structure breakdown after a 24-h storage period. Changes in the rheological properties of guar and pectin dispersions were significantly higher than that of xanthan dispersions.
Keyword:
INTRODUCTION
Hydrocolloids such as guar gum, xanthan gum, and pectin are water-soluble, high molecular weight polysaccharides that find wide application in the food industry, optimising the rheological and textural characteristics of food systems. Guar gum, xanthan gum and pectin are typically used in food formulations for optimising viscosity, creating gel-structures and enhancing structural stability.[Citation1,Citation2,Citation3,Citation4] Power ultrasound has many potential applications in the food processing industry.[Citation5,Citation6] Recently high intensity ultrasound has been widely studied as a non-thermal food preservation method.[Citation7] Ultrasonic waves are mechanical waves at frequencies between 16 kHz and several hundred MHz.[Citation8,Citation9,Citation10] Propagation of high-intensity ultrasound is accompanied by generation of compression and shear waves at large intensities that in turn create shock waves.[Citation11] In food processing operations it has been used to induce structural changes in food components, to enhance filtration rates[Citation12], inactivation and disruption of microorganisms[Citation13,Citation14,Citation15] and enzymes.[Citation16,Citation17,Citation18,Citation19]
Application of high intensity ultrasound to dispersions of carbohydrates can lead to depolymerisation due to the intense mechanical and chemical effects associated with cavitation.[Citation9,Citation10,Citation20,Citation21] Cavitational thermolysis may produce hydroxyl radicals and hydrogen atoms that can be followed by formation of hydrogen peroxide and, in the absence of oxygen, hydroperoxyl radicals.[Citation8,Citation22,Citation23] These transient reactive species can subsequently react with carbohydrates.[Citation24] In addition hydrolysis and cleavage due to the strong mechanical forces has been reported for a variety of polysaccharides.[Citation25]
Rheological properties of polysaccharides are influenced by number of factors such as concentration and thermal or mechanical treatments.[Citation26] Irradiation has been reported to influence the flow behaviour of hydrocolloid dispersions.[Citation27,Citation28,Citation29] Price[Citation30] demonstrated that synthetic long-chain polymer solutions that were subjected to sonication underwent a controlled degradation, which reduced their molecular weight. Various authors have suggested that the mechanical effects of high-intensity ultrasound which contribute to the degradation of these polymers are due to cavitation.[Citation20,Citation21,Citation31,Citation32,Citation33] High intensity ultrasound has also been reported to influence structural and functional properties for a variety of polysaccharides.[Citation25] The objective of this study was to investigate the effect of high intensity sonication on the rheological properties of guar, xanthan and pectin dispersions.
MATERIALS AND METHODS
Hydrocolloids
Guar (Product No: G4129), xanthan from Xanthomonas campestris (Product No: 95465) and pectin from apples (Product No: 76282) were supplied by Sigma-Aldrich Ireland Ltd, Dublin, Ireland. Guar 1%, xanthan 1% and pectin 2% (w/w) dispersions were prepared in 500 ml distilled water and continuously mixed under high shear (2500 rpm, L4RT Laboratory Mixer, Silverson Machines Ltd, Chesham Bucks, England) at room temperature (20oC) for 30 min.
Ultrasound Treatment
A 1,500 W ultrasonic processor (VC 1500, Sonics and Materials, Inc., Newtown, USA) with a 19 mm probe was used for all ultrasound treatments. All samples were processed at a constant frequency of 20 kHz. The energy input was controlled by varying the amplitude of the sonicator probe. The amplitude level was varied with pulse durations of 5 s on and 5 s off giving ultrasonic intensity levels of 3.7, 6.3, 8.1, & 10.1 W/cm2, respectively. 80 ml samples were maintained at a temperature of 25 ± 1.0oC using a water bath equipped with a pump to circulate water at 0.5 l/min through a 100 ml jacketed vessel. The ultrasound probe was submerged to a depth of 25 mm in the sample dispersion.
Ultrasonic Intensity
Ultrasonic intensity was determined using a calorimetric method[Citation34]. Relative ultrasonic intensity was calculated with reference to distilled water. Temperature (T) was recorded as a function of time under adiabatic conditions using a datalogger thermometer with T type thermocouple (RS-1315, Radionics, Dublin, Ireland). Temperature rise (dT/dt) was determined by polynomial curve fitting. The ultrasonic power (P) is given as:
Rheological Analysis
A controlled stress rheometer (Carrimed CSL2 – 100, TA Instruments, UK) was used for rheological analysis. 3.5 ml hydrocolloid dispersions were measured using a concentric cylinder geometry (length = 40 mm, diameter = 26.66 mm, gap width = 4000 μm) at 25 ± 0.1oC. Flow curves were obtained for a shear rate sweep between 0.1 and 99 s-1. Shear stress – shear rate data was fitted to a power law model (EquationEquation 3).
Control and sonicated samples were subsequently analysed after a 24 h storage period to investigate structural recovery. Complex modulus (G*) was calculated from G′ and G″ values using EquationEquation 4 and modelled using the power law EquationEquation (5):
where a (Pa.s) and b (dimensionless) are dynamic rheological power law constants and ω (Hz) is frequency.
Statistical Analysis
Analysis of variance (ANOVA) and separation of means was carried out using the Proc GLM procedure (SAS V.9.1, SAS Institute, NC, USA). Treatment means were separated using Tuckey's test and considered significantly different at p < 0.05. All trials were conducted in triplicate.
RESULTS AND DISCUSSION
Flow Curves
Apparent viscosities as a function of shear rate for guar, xanthan and pectin dispersions are shown in . Flow curves for all samples exhibit shear thinning behaviour at all ultrasonic intensity levels. Consistency (k) and flow behaviour (n) indices for all dispersions at each intensity level and control are presented in . These results indicate significant differences in k and n values for each of the hydrocolloid control samples studied.
Figure 1 Effect of ultrasonic intensity on apparent viscosity of a) guar gum 1% b) xanthan 1% & c) pectin 2% with respect to shear rate. Control (♦), 3.7 (▪), 6.3 (▴), 8.1 (×) and 10.1 W/cm2 (+).
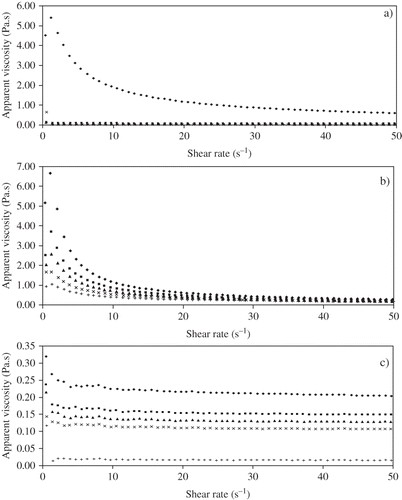
Table 1 Mean values of k and n for sonicated hydrocolloid dispersions
The effect of ultrasound intensity (UI) on the consistency index (k) values was modelled using regression analysis and shown in . The consistency index (k) of all three hydrocolloids decreased as a function of ultrasonic intensity. For xanthan, the consistency index decreased linearly with increasing intensity level. However, for pectin, the decrease in consistency index was exponential. A drastic reduction in k which followed a power-law decay was observed for guar. A 99.7% reduction in the guar consistency index was observed at an ultrasound intensity of 10.1 W/cm2 relative to the control. Equivalent reductions in k, for xanthan and pectin dispersions were 89.7% and 59.6% respectively. The magnitude of ultrasonic intensity was not found to be significant for guar (), with a 98.7% reduction in k value observed at the lowest intensity level of 3.7 W/cm2. Conversely, for xanthan and pectin dispersions the reduction in k was strongly dependent upon intensity level ().
Figure 2 Changes in consistency index (k) value of guar gum 1% (♦), xanthan 1% (▪) and pectin 2% (▴) with respect to applied ultrasonic intensity.
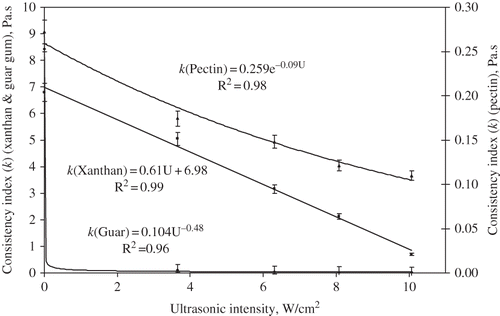
A trend towards increased Newtonian flow behaviour at increasing UI was observed, with pectin showing the highest n value followed by guar and xanthan (). This behaviour may be due to the branching structure of xanthan which displays greater structural stability over a wide range of temperature, pH and pK (ionic concentration) compared to natural gums such as guar[Citation35] and pectin. Xanthan shifts from an ordered to a disordered structure at temperatures above 40oC.[Citation36,Citation37,Citation38] Similarly sonication of xanthan is found to reduce viscosity and cause a shift in ordered structure.
The effects of applied intensity level on the flow behaviour (n) indices for all three hydrocolloid dispersions were modelled as shown in . Guar flow behaviour index showed a sharp increase at an intensity level of 3.7 W/cm2 with further small increases observed at higher intensity levels. For xanthan a small decrease in n value was initially observed at an intensity level of 3.7 W/cm2, subsequently followed by an increase in n value at higher intensity levels. In contrast to guar and xanthan, the n value of pectin showed a logarithmic increase with applied amplitude at all levels. With the exception of xanthan, both guar and pectin dispersions tended towards Newtonian behaviour at 10.1 W/cm2 intensity levels as n values approached 1 (). However, for xanthan the observed n value at intensity level of 10.1 W/cm2 was 0.59, which indicated a greater inherent resistance to structural breakdown.
Dynamic Rheological Properties
A typical frequency sweep of elastic (G′) and viscous (G″) moduli at 25±1 oC for control and lowest ultrasonic intensity level (3.7 W/cm2) sonicated samples is shown in for 1% guar, 1% xanthan, and 2% pectin dispersions. The elastic moduli (G′) of the guar and xanthan control dispersions were higher than their viscous moduli (G″), whereas for pectin the reverse relationship was observed. The dependence of G′ and G″ on ultrasonic intensity at the lowest level is demonstrated in . G′ values for guar and pectin were reduced towards zero for all sonicated samples, clearly indicating structural breakdown. While reductions in the G′ values of sonicated xanthan samples were observed at higher frequencies, a significant elastic component was retained. Guar was found to be the most sensitive to sonication. For example at a frequency of 5 Hz and an intensity level of 3.7 W/cm2, G` values decreased from 20.91 to 0.05 Pa.s, 0.23 to 0.00 Pa.s and 21.77 to 10.73 Pa.s for guar, pectin and xanthan dispersions respectively. Seshadri et al.[Citation39] reported a similar influence of sonication on gel strength properties of pectin.
Figure 4 Effect of ultrasonic intensity on a) elastic modulus (G′) and b) viscous modulus (G″) on guar gum 0 (♦), 3.7 W/cm2(⋄) xanthan 0(▪), 3.7 W/cm2(□) and pectin 0(▴), 3.7 W/cm2(Δ).
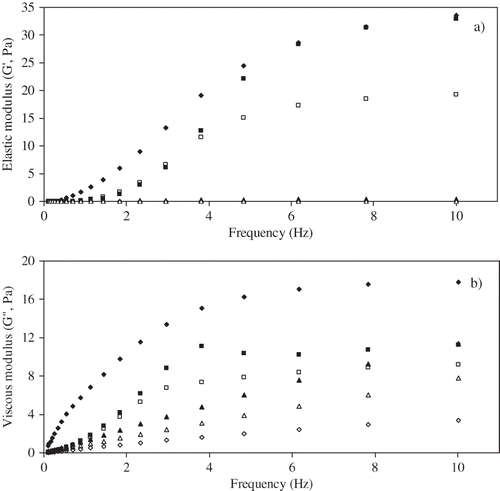
Previous studies in which starch and agar dispersions were sonicated, have shown that the reduction in dispersion viscosity was a result of chain cleavage.[Citation40] Sonication results in pressure fluctuations which propagate through the material resulting in the formation of microscopic bubbles that are highly unstable and collapse within a few milliseconds. Consequently, in the vicinity of these cavitational bubbles, high shear forces are applied to the hydrocolloid dispersion particles. In addition the temperature in the vicinity of the bubbles increases and may lead to cleavage of macromolecules. Cleavage of polysaccharides is mainly due to breakage of glycosidic linkages which may be induced by OH radicals formed during sonication. Czechowska-Biskup et al.[Citation41] reported ultrasound-induced degradation of chitosan solution was caused by either by OH radicals or mechanochemical effects.
Structural Recovery of Hydrocolloid Dispersions
shows the complex modulus power law constants of guar, xanthan, and pectin immediately after ultrasonic processing and after a recovery period of 24 h. From this figure it is clear that the complex modulus (G*) decreased with increasing intensity level. It was observed that after 24 h there were no significant changes in complex modulus power law constants for all hydrocolloid dispersions and intensity levels investigated. Therefore, these hydrocolloids dispersions when treated with high intensity ultrasound display time independent rheological behaviour.
CONCLUSIONS
Significant reductions in apparent viscosity and a trend towards Newtonian flow behaviour were observed for sonicated guar, xanthan, and pectin dispersions. Dynamic rheological studies indicated that sonication alters the rheological properties of hydrocolloid dispersions. Such changes which may be due to permanent pyrolysis of hydrocolloid dispersions were found to be irreversible. Changes in the rheological properties of guar and pectin dispersions were significantly higher than that of xanthan dispersions. These results show the effects of sonication on the rheology of hydrocolloid dispersions, should be considered when foods incorporating such hydrocolloids are subsequently treated with ultrasound. Ultrasound seems to be a promising alternative to presently used degradation techniques where degradation of hydrocolloid dispersions is desirable.
ACKNOWLEDGMENTS
Funding for this research was provided under the Irish National Development Plan, through the Food Institutional Research Measure (FIRM), administered by the Irish Department of Agriculture, Fisheries & Food (DAFF).
REFERENCES
- Sikora , M , Juszczak , L and Sady , M. 2003 . Hydrocolloids in forming properties of cocoa syrups . International Journal of Food Properties , 6 ( 2 ) : 215 – 228 .
- Garti , N. and Reichman , D. 1993 . Hydrocolloids as food emulsifiers and stabilizers . Food Structure , 12 : 411 – 426 .
- Fagan , C.C , O'Donnell , C. P , Cullen , P.J and Brennan , C.S. 2006 . The effect of dietary fibre inclusion on milk coagulation kinetics . Journal of Food Engineering. , 77 : 261 – 268 .
- Hinrichs , R. , Gotz , J. and Weisser , H. 2003 . Water-holding capacity and structure of hydrocolloid-gels, WPC-gels and yogurts characterised by means of NMR . Food Chemistry , 82 : 155 – 160 .
- Leadley , C. and Williams , A. 2002 . Power ultrasound – current and potential applications for food processing , Review No 32 Campden, , UK : Campden and Chorleywood Food Research Association .
- Seymour , I.J. , Burfoot , D. , Smith , R.L. and Cox , L.A. 2002 . Lockwood, A. Ultrasound decontamination of minimally processed fruits and vegetables . International Journal of Food Science and Technology , 37 : 547 – 557 .
- Tiwari , B.K , Muthukumarappan , K. , O'Donnell , C.P and Cullen , P.J. 2008 . Effects of sonication on the kinetics of orange juice quality parameters . Journal of Agricultural and Food Chemistry , 56 ( 7 ) : 2423 – 2428 .
- Cains , P.W. , Martin , P.D. and Price , C.J. 1998 . The use of ultrasound in industrial chemical synthesis and crystallization. 1 . Applications to synthetic chemistry. Organic Process Research and Development , 1 : 234 – 248 .
- Mason , T.J. and Cordmas , E.D. 1996 . Ultrasonic intensification of chemical processing and related operations—a review . Transactions of the Institute of Chemical Engineers , 74 ( A ) : 511 – 516 .
- Mason , T.J. 1997 . Ultrasound in synthetic organic chemistry . Chemical Society Reviews , 26 ( 6 ) : 443 – 451 .
- Price , G.J. , White , A.J. and Clifton , A.A. 1995 . The effect of high intensity ultrasound on solid polymers . Polymer. , 26 : 4919 – 4925 .
- Floros , J.D. and Liang , H. 1994 . Acoustically assisted diffusion through membranes and Biomaterials . Food Technology , 48 : 79 – 84 .
- Suslick , K.S. 1998 . Ultrasounds: Its Chemical, Physical and Biological Effects , New York : VHC Publishers .
- Earnshaw , R.G. 1998 . “ Ultrasound a new opportunity for food preservation ” . In Ultrasound in food processing , Edited by: Povey , M.J.W. and Mason , T. J. 183 – 192 . London : Blackie Academic & Professional .
- Lillard , H.S. 1994 . Decontamination of poultry skin by sonication . Food Technology , 48 : 72 – 73 .
- Sala , F.J. , Burgos , J. , Condon , S. , Lopez , P. and Raso , J. 1995 . “ Effect of heat and ultrasound on microorganisms and enzymes ” . In New Methods of Food Preservation , Edited by: Gould , G.W. 176 – 204 . London : Blackie Academic & Professional .
- Villamiel , M. and de Jong , P. 2000 . Influence of high-intensity ultrasound and heat treatment in continuous flow on fat, proteins and native enzymes of milk . Agriculture and Food Chemistry , 48 : 472 – 478 .
- Vercet , A. , Lopez , P. and Burgos , J. 1997 . Inactivation of heat-resistant lipase and protease from Pseudomonas fluorescens by manothermosonication . Journal of Dairy Science , 80 : 29 – 36 .
- De Gennaro , L. , Cavella , S. , Romano , R. and Masi , P. 1999 . The use of ultrasound in food technology I: inactivation of peroxidase by thermosonication . Journal of Food Engineering , 39 : 401 – 407 .
- Crum , L. A. 1995 . Comments on the evolving field of sonochemistry by a cavitation physicist . Ultrasonics Sonochemistry , 2 : S147 – S162 .
- Stephanis , C.G. , Hatiris , J.G. and Mourmouras , D.E. 1997 . The process mechanism of erosion of soluble brittle materials caused by cavitation . Ultrasonics Sonochemistry , 4 : 269 – 271 .
- Ashokkumar , M. and Grieser , F. 1999 . Ultrasound assisted chemical process . Reviews in Chemical Engineering , 15 ( 1 ) : 41 – 83 .
- Portenlaenger , G. and Heusinger , H. 1997 . The influence of frequency on the mechanical and radical effects for the ultrasonic degradation of dextranes . Ultrasonics Sonochemistry , 4 : 127 – 130 .
- Bober , U. 1998 . Oxidativer Abbau aromatischer Verbindungen durch Ultraschall in wabriger Losung am Beispiel von Phenol, 3 Nitrophenol, 1,3-Dinitrobenzol und 2,4 Dinitrobenzol (in German) . Wissenschaftlich Berichte Forschungszentrum Karlsruhe—Technik und Umwelt , : 133
- Kardos , N. and Luche , J.L. 2001 . Sonochemistry of carbohydrate compounds. Carbohydrate Research . 332 : 115 – 131 .
- Marcotte , M. , Taherian , A.R. and Ramaswamy , H.S. 2001 . Evaluation of rheological properties of selected salt enriched food hydrocolloids . Journal of Food Engineering , 48 ( 2 ) : 157 – 167 .
- Aliste , A. J. , Vieira , F.F. and Del Mastro , N.L. 2000 . Radiation effects on agar, alginates and carrageenan to be used as food additives . Radiation Physics and Chemistry. , 57 ( 2 ) : 305 – 308 .
- Delincee , H. 2002 . Analytical methods to identify irradiated food — a review . Radiation Physics and Chemistry , 63 ( 3–6 ) : 455 – 458 .
- Kayacier , A. and Dogan , M. 2006 . Rheological properties of some gums-salep mixed solutions . Journal of Food Engineering , 72 ( 3 ) : 261 – 265 .
- Price , G. 1990 . “ The use of ultrasound for the controlled degradation of polymer solutions ” . In Advances in sonochemistry; Mason, T. J , 231 – 287 . London : JAI Press .
- Mason , T.J. , Paniwnyk , L. and Lorimer , J.P. 1993 . The uses of ultrasound in food technology. Ultrasonics Sonochemistry . 3 : 253 – 260 .
- Mason , T.J. , Lorimer , J.P. and Bates , D.M. 1990 . Quantifying sonochemistry: casting some light on a black art . Ultrasonics , 30 ( 1 ) : 40 – 48 .
- Mason , T.J. and Lorimer , J.P. 1988 . Sonochemistry: theory, applications and uses of ultrasound in chemistry , 99 – 138 . Chichester, , UK : Ellis Horwood .
- Manas , P. , Pagan , R. and Raso , J. 2000 . Predicting lethal effect of ultrasonic waves under pressure treatments on Listeria monocytogenes ATCC15313 by power measurements . Journal of Food Science. , 65 ( 4 ) : 663 – 667 .
- Kang , K.S. and Pettit , D.J. 1993 . “ Xanthan, gellan, welan, rhamsam, in industrial gums: polysaccharides and their derivatives ” . In Academic Press , 3rd Edited by: Whistley , R.L. and BeMiller , J.N. 342 – 371 . New York
- Morris , E.R. 1977 . “ Molecular origin of xanthan solution properties ” . In Extracellular Microbial Polysaccharides , ACS Symposium Series 45 Edited by: Sandford , P.A. and Laskin , A. 81 – 90 . Washington, DC : ACS .
- Milas , M. and Rinaudo , M. 1986 . Properties of xanthan gum in aqueous solutions: role of the conformational transition . Carbohydrate Research , 158 : 191 – 204 .
- Casas , J.A. , Mohedano , A.F. and Garcia-Ochoa , F. 2000 . Viscosity of guar gum and xanthan/guar gum mixture solutions . Journal of the Science of Food and Agriculture , 80 : 1722 – 1727 .
- Seshadri , R. , Weiss , J. , Hulbert , G.J. and Mount , J. 2003 . Ultrasonic processing influences rheological and optical properties of high-methoxyl pectin dispersions . Food Hydrocolloids , 17 : 191 – 197 .
- Price , G. 1993 . Applications of high intensity ultrasound in polymer chemistry . Chemistry and Industry , 3 : 75 – 78 .
- Czechowska-Biskup , R. , Rokita , B. , Lotfy , S. , Ulanski , P. and Rosiak , J.M. 2003 . Degradation of chitosan and starch by 360-kHz ultrasound . Carbohydrate Polymer. , 60 : 175 – 184 .