Abstract
Wheat-legume composite flours were produced by blending Canada Western Extra Strong (CWES) and Canada Western Red Spring (CWRS) wheat with varying amounts of three legume proteins. Legume protein addition produced breads with lower specific loaf volume, coarser crumb and firmer texture, and cooked white-salted noodles with greater compression stress and less cutting stress than the wheat controls. The CWES wheat compensated for the negative baking effects of the legume proteins as much as the CWRS wheat. End-use quality did not change at 2% soybean protein addition. Yellow pea protein produced the greatest quality changes, followed by chickpea and soybean protein.
INTRODUCTION
Many studies have investigated the effect of supplementation of wheat flour with legume flour for making products such as bread.[Citation1–4] The benefits of these composite flours are largely centered on nutritional considerations. In general, legumes have higher amounts of the essential amino acid lysine and improved protein efficiency ratio (PER) compared to wheat. Soybean has a lysine content of 6.34–6.40 g/16 g N and a PER of 1.60.[Citation5–6] Field pea has a lysine content of 7.39 g/16 g N and a 1.56 PER.[Citation5–6] Although a low lysine content was found in chickpea (1.42 g/16 g N), chickpea protein has been reported to be more digestible and higher in quality (2.8 PER) than that of wheat (2.3 g lysine/16 g N, 0.95 PER).[Citation5,Citation7,Citation8] By complementing wheat flour with these legumes, the nutritional values of baked goods can be improved.
Reduced loaf volume and unacceptable physical and sensory properties including crumb structure and texture, crust and crumb color, loaf shape, flavor, and aroma of the yeast-leavened wheat-legume composite breads have often been reported.[Citation1–4] Two of the most important causes that have been suggested for reduced loaf volume are the disruption of the wheat protein-starch interface by non-wheat proteins in the supplemented breads[Citation9] and the dilution of wheat gluten, the protein matrix that is the primary determinant of baking quality, such that the integrity of the matrix is disturbed and non-functional.[Citation10–13]
To counteract the deleterious effects of legumes in composite breads, additions of gluten and/or dough conditioners such as glycolipids, sucrose monolaurate, polyoxyethylene-8-stearate, potassium bromate and sodium steaoryl-2-lactylate have been evaluated[Citation1,Citation23]. Studies of this nature have always used hard red spring and winter wheats, wheats with moderately strong dough mixing properties, rather than extra-strong mixing wheats. Canada Western Extra Strong (CWES) wheats have very strong gluten strength compared to other classes of Canadian wheat. Due to their long dough development time, they are mainly used in blending with other wheats to improve dough strength for the production of frozen doughs, hearth breads, and pan breads.[Citation14] Canada Western Red Spring (CWRS) wheats are known for their superior milling and baking quality and are used for making into high volume pan breads, specialty breads, noodles and common wheat pasta.[Citation14]
In this study, wheat-legume composite flours were produced from CWES and CWRS wheat flour and the legume proteins from soybean, yellow pea, and chickpea. The extra-strong dough properties of the CWES were postulated to ameliorate the negative effects of the legume proteins in baking. In addition, this study explored the potential of composite flours in white-salted noodles. The specific objectives of this study were 1) to evaluate the effect of soybean, yellow pea and chickpea protein on physical, chemical and end-product properties of wheat-legume composite flours; 2) to evaluate different wheat cultivar types for their performance in composite flours with legume proteins; and 3) to determine the tolerance level of legume protein addition in wheat flour for the production of bread and white-salted noodle.
MATERIALS AND METHODS
Wheat Cultivars and Legume Protein Samples
Three CWES (Burnside, Glenlea and ES 77) and one CWRS (AC Barrie) wheat cultivars were used in this study. ES 77 is a white seeded cultivar whereas the other cultivars are red seeded. All wheat samples were grown in 2002 at Indian Head, Saskatchewan. Yellow pea and chickpea protein concentrates were produced at the AAFC pilot plant in St. Hyacinthe, Quebec. The soybean protein was a commercial product, Supro 760 IP NON-GM, manufactured by the Solae Company (St. Louis, Missouri).
Protein Extraction of Chickpeas and Yellow Peas
Chickpea and yellow pea seeds (Clic Premium, Canada) were purchased commercially. The seeds were first frozen in liquid nitrogen and then ground using a Brinkmann centrifugal grinding mill (Model No. ZM-1, Brinkmann Instruments Canada, Mississauga, ON, Canada) equipped with a 0.5 mm ring sieve. Samples of the meal were defatted three times using a mixture of hexane, petroleum ether, and ethyl ether with a meal-solvent ratio of 1:1 (w/v). For the preparation of the protein isolates, the defatted meal (10 Kg) was hydrated with 100 L of water (55°C) and the pH of the dispersion was adjusted and maintained at 8.5 with 2N NaOH for 45 min. The temperature was maintained by circulating hot water through the double-jacketed vessel. The mixture was cooled to room temperature and the slurry was pumped into a bucket centrifuge (Model STM-2000, Western States, Hamilton, Ohio, U.S.A.) equipped with a 1 μm filter and centrifuged at 3000 rpm. The supernatant was collected and the pH was adjusted to 4.5 with 2.5N HCl and kept at 4°C over night to allow the precipitating proteins to settle. The supernatant was decanted using a peristaltic pump and the precipitated protein was recovered and washed twice with distilled water to remove excess minerals using the same decantation process as described above. The washed precipitate was resuspended in a minimum amount of water (20 L), neutralized to pH 7.0 and freeze-dried using a SE-05 LYO-TECH freeze drier (Lyo-San Inc., Lachute, QC, Canada).
Preparation of Composite Flours
Wheat samples were tempered to 16.5% moisture overnight and were milled into straight-grade flours using the Buhler experimental mill (Buhler AG, Uzwil, Switzerland). Yellow pea and chickpea protein were reduced in size (≤250 μm) with a blender and a Ro-tap Sieve Shaker (Ro-tap, W.S Tyler, Mentor, Ohio, U.S.A.). The soybean protein was a fine powder, with 90% particles passing through US Standard 100 mesh (≤150 μm). Composite flour samples (500 g) were prepared by blending 0, 2, 5, and 8% of each of the legume proteins on an as-is flour basis with both the CWES and CWRS wheat flours.
Physicochemical Tests
All samples were measured for air-oven moisture[Citation15] and ash content.[Citation15] Flour color was measured in CIE color coordinates (L* a* b*) with a Minolta spectrophotometer (Model CM-525i, Minolta, Osaka, Japan) set at 2° observer and “C” illuminant. Total nitrogen (N) was determined by combustion method[Citation15] using the Leco FP-528 system (Leco Corp., St. Joseph, MI) and percent protein was calculated as N × 5.7 for wheat and N × 6.25 for legume proteins. Water absorption and dough strength were determined using a 50 g farinograph at 65 rpm.[Citation15]
Pan Bread
Composite flour samples were made into 25 g bread loaves using a modification of the optimized straight-dough (long fermentation) bread-making method.[Citation15] Mixing and sheeting were done with a 35 g mixograph (National MFG Co., Lincoln, Nebraska) and a Somerset dough sheeter (Somerset Industries Incorporated, Billerica, MA), respectively. Loaf volume was measured by rapeseed displacement. Crumb fineness and firmness were determined according to Ambalamaatil et al.[Citation16] with image analysis software (CrumbScan, American Institute of Baking, Manhattan, Kansas) and the TA.XT2i Texture Analyser (Texture Technologies Corp., Scarsdale, NY, and Stable Micro Systems, Godalming, Surrey, UK) with probe speed of 1.7 mm/sec and 40% strain, respectively. Crust and crumb color (CIE color coordinates, L* a* b*) were determined with a Minolta Baking Meter BC-10 (Konica Minolta, Tokyo, Japan). Specific loaf volume was calculated by dividing loaf volume by loaf weight.
White-Salted Noodle
Sodium chloride (1%) and distilled water (32%), both based on flour weight, were mixed with a 20 g composite flour sample (14% moisture basis) in a 35 g mixograph (National MFG Co., Lincoln, Nebraska) for 5 min before being sheeted through a bench-top laboratory noodle machine.[Citation17] Dough was sequentially sheeted once at gap sizes: 5, 4, 4 (folded into half before sheeting), 3, 2, 1.5, 1.2, and 1 mm. A portion of sheet was incubated at 25°C for color measurement at 2 and 24 hours (Minolta Baking Meter BC-10). The remainder was cut into noodles strands (1.6 mm wide x 5 cm long) and rested at 25°C for 1 hour. Noodles were cooked for 6 min, cooled under water and drained each for 1 min. Color was determined from two sets of 10 strands each of cooked noodles (Minolta Baking Meter BC-10). Noodle compression and cut peak force were determined on three sets of five strands each of cooked noodles using the TA.XT2i Texture Analyzer with a 5 mm wide blade travelling at 0.4 mm/sec to 50% deformation and a 1-mm wide blade at a speed of 0.8 mm/sec to a maximum distance of 4.8 mm, respectively. Compression and cut stress were calculated by dividing peak force with the contact area (noodle width x blade width).[Citation18]
Statistical Analyses
All tests were done in duplicate. Ten samples including a control and nine wheat-legume composite flours for each cultivar were made into a product within one day. Composite flours were all randomized within one day and cultivars were randomly assigned to days. Data was analyzed using analysis of variance and means including all controls and composite flours were compared using the least significance difference test (α = 0.05). All analyses were done by SAS version 9.1.3.
RESULTS AND DISCUSSION
Chemical and Physical Properties of Legume Proteins, Wheat Flours, and Composite Flours
All legume proteins had significantly higher protein and ash contents and were less bright (lower L* value) and more yellow (higher b* value) in color compared to the wheat flours (). Among the legume proteins, soybean protein had the highest protein content and the lowest ash content. Chickpea protein had the lowest protein content and the highest ash content. The legume proteins differed in color; soybean protein was light yellow, yellow pea protein was slightly brown, and chickpea protein was yellow.
Table 1 Chemical and color properties (means and standard deviations) of legume proteins, wheat flours and composite flours
Among all the straight-grade flours, Burnside of the CWES wheat class had the highest protein content, followed by AC Barrie in the CWRS class, and Glenlea, and ES 77 in the CWES class (). All CWES cultivars had higher flour ash content than AC Barrie. All flours were similar in brightness (L*). Burnside flour was the least red (lowest a* value) and the most yellow (b*) in color, followed by AC Barrie, ES 77, and Glenlea.
Blending legume proteins with wheat flours generally increased protein and ash contents of the composite flours (). Legume proteins also decreased flour brightness (L*) and increased flour yellowness (b*). Flour redness (a*) was reduced with soybean protein addition but was increased with yellow pea and chickpea protein at each level. Addition of soybean protein had the least effect on the overall flour color changes. Composite flours were the most yellow (b*) with addition of chickpea protein and were the most dark (L*) and red (a*) with the addition of yellow pea protein at each level.
Farinograph
Increased additions of legume proteins to wheat flours increased farinograph water absorptions (). This was in agreement with some[Citation3,Citation19,Citation20] but not all[Citation1,Citation21] studies of composite flours. The increase in water absorption of the composite flours in this study is likely due to the increase in the total protein content of the composite flours and the high water absorption capacity of the legume proteins.[Citation3,Citation19,Citation20] Most composite flours had significantly higher water absorption than the controls except for composite flours made up of ES 77 and 2% soybean or yellow pea protein. Most differences in water absorption of the composite flours occurred at 5% and 8% legume protein additions. At these levels, yellow pea and chickpea protein effects were similar and were greater than that of soybean protein. Overall, AC Barrie flour and its composite flours had the highest water absorption, followed by ES 77, Glenlea, and Burnside.
Figure 1 Farinograph properties of composite flour blends. A) Water absorption, B) Stability; Soy = Soybean protein; YP = Yellow pea protein; CP = Chickpea protein.
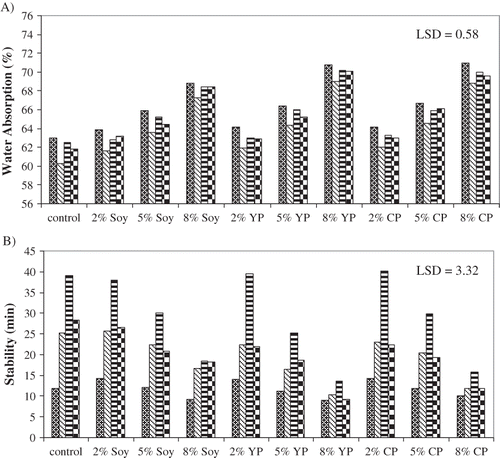
Our results generally agreed with other studies in that farinograph stability or dough strength decreased as the percent of legume protein addition increased for certain cultivars[Citation10,Citation11,Citation19,Citation22,Citation23] (). In this study, none of the legume proteins at any addition level significantly reduced AC Barrie stability. Burnside tolerated the addition of 5% soybean protein and 2% yellow pea or chickpea protein before dough stability decreased significantly. The tolerance levels for ES 77 were 2% soybean, yellow pea, or chickpea protein, whereas Glenlea only tolerated 2% soybean protein addition without reductions in dough stability. With the addition of 8% soybean protein, most CWES wheats had significantly greater dough stability than with other legume proteins. Overall, of all the cultivars, the stability of AC Barrie was the lowest and ES 77 was the greatest even after legume protein additions, in agreement with the general dough strength properties of CWRS and CWES wheats.
Pan Bread
Specific loaf volume decreased significantly compared to the controls and was dependent on the cultivar and the type and amount of legume protein supplementation (). Decreased specific loaf volume was detected at 5% soybean protein addition for all cultivars. The addition of chickpea protein at the 2% level or higher significantly affected the specific loaf volume of ES 77, whereas 5% or higher protein addition reduced specific loaf volume of the other cultivars. All yellow pea protein additions to AC Barrie, Burnside and Glenlea, and yellow pea protein additions at 5% and 8% levels to ES 77 decreased specific loaf volume significantly from the controls. Soybean protein and chickpea protein had a similar effect on the specific loaf volumes of most cultivars. At the 8% level, the specific loaf volumes of all cultivars with yellow pea protein addition were significantly lower than with the other protein types. Chemically leavened bread made with wheat-pea composite flour has been reported to be poorer in loaf volume than bread made with wheat and soybean flour.[Citation13] Generally, composite flour breads of AC Barrie and Glenlea had higher specific loaf volumes than Burnside and ES 77. The very strong mixing properties of Glenlea () were sufficiently diluted with legume proteins to produce bread of comparable specific volume to AC Barrie. However, the extreme dough strength as shown by ES 77 (), could not be ameliorated by legume proteins into a viscoelastic dough suitable for bread making. ES 77 loaf volumes were generally the lowest of all samples.
Figure 2 Bread loaf characteristics of composite flour blends. A) Specific loaf volume, B) Crumb fineness, C) Crumb firmness; Soy = Soybean protein, YP = Yellow pea protein, CP = Chickpea protein.
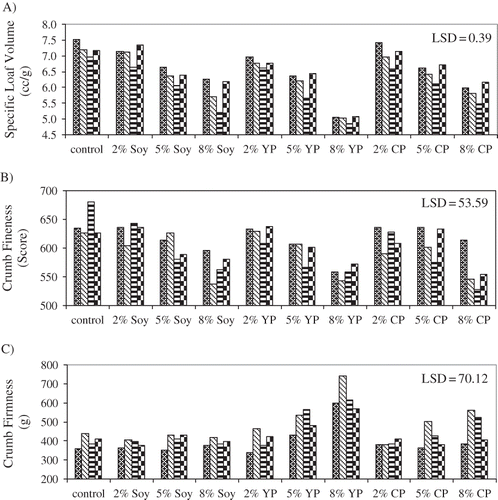
Compared to the controls, the addition of yellow pea protein decreased crumb fineness (i.e. coarser crumb), starting at the 2% level for ES 77 and at the 8% level for the other cultivars (). Poor crumb grain with additions of 5% yellow pea protein to wheat flours was also reported by Hsu et al.[Citation2] Bread crumb was also significantly coarser than the controls when chickpea protein was added to either Burnside or Glenlea at the 8% level and to ES 77 at 5 and 8% levels. The effects of chickpea protein on loaf crumb characteristics of AC Barrie and ES 77 are shown in . AC Barrie tolerated the addition of chickpea protein to a greater degree than ES 77. The addition of chickpea protein to AC Barrie did not significantly affect bread crumb fineness but had a significant negative effect on ES 77. Soybean protein effects on crumb fineness of each cultivar were similar to that of chickpea protein with one exception. In addition to AC Barrie, crumb fineness of Glenlea was not affected by soybean protein addition at any level. Similar degrees of crumb fineness occurred across most legume protein types for each cultivar at comparable levels of addition with the exception of 8% chickpea addition to AC Barrie, which produced a finer bread crumb structure than 8% yellow pea addition.
Figure 3 Pan breads of AC Barrie and ES 77 chickpea protein composite flours. CP = Chickpea protein.
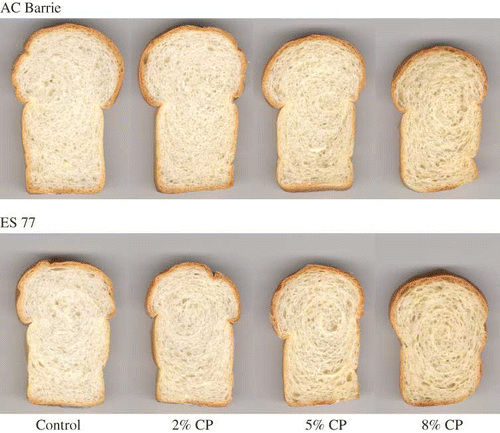
Soybean protein addition had no effect on the bread crumb firmness for any cultivar and level of addition (). Addition of 8% chickpea protein to Burnside and to ES 77 resulted in firmer bread texture than the controls and also firmer breads than 8% soybean protein additions to these same cultivars. Bread firmness also increased significantly from the controls with the addition of yellow pea protein at 5% and 8% levels in all cultivars. Burnside yellow pea composite flour produced the firmest bread, which may be related to the very high protein content of the wheat flour (). All breads containing 8% yellow pea protein were significantly firmer than the corresponding breads made with similar levels of soybean and chickpea protein. Compared to the study of Ribotta et al.[Citation19] that showed that firmer bread crumb texture at 5% soybean protein addition, this study showed greater tolerance of breads in maintaining their texture. The reason may be partly due to the strong dough strength of wheat flours used in this study and their ability to compensate for crumb firmness.
Crumb color (L* a* b*) comparisons of the composite breads are shown in , B and C. In general, color effects of legume protein addition were cumulative on the original color of the base flours. Addition of up to 8% soybean protein to all flours did not significantly affect bread crumb brightness (L*) (). Addition of 5% and 8% yellow pea protein, and 8% chickpea protein to AC Barrie, ES 77 and Glenlea reduced bread crumb brightness (L*) significantly from the controls. Crumb brightness (L*) of Burnside was only reduced from the control upon 8% yellow pea protein addition. Increasing additions of yellow pea or chickpea protein produced similar reductions in crumb brightness for most cultivars. Overall, there was no significant difference in crumb brightness among cultivars within each level and type of protein addition, with the exception of 8% chickpea protein addition in Burnside, which was significantly brighter than other cultivars.
Figure 4 Bread crumb color of composite flour blends. A) L* value; B) a* value; C) b* value; Soy = Soybean protein; YP = Yellow pea protein; CP = Chickpea protein.
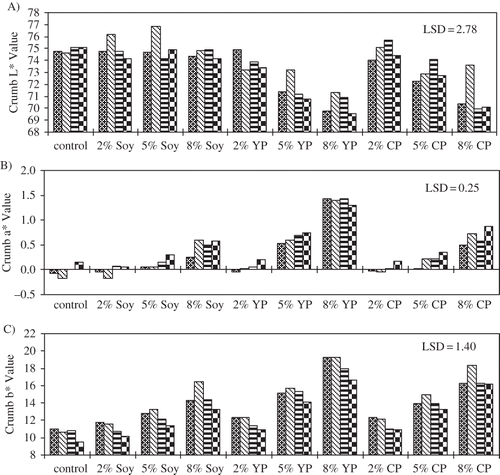
Bread crumb became more red (a*) () and more yellow (b*) () in color as the percent of legume protein addition increased. The addition of 8% soybean or chickpea protein, and 5% or 8% yellow pea protein to each flour significantly increased bread crumb redness (a*) compared to the controls. The addition of either 5% or 8% yellow pea protein to all flours had the greatest effect on crumb redness when compared to other protein types. Supplementation of all flours with 5% or 8% soybean, yellow pea or chickpea protein increased bread crumb yellowness (b*) significantly compared to the controls. Breads containing 5% or 8% yellow pea or chickpea protein were significantly more yellow (b*) than breads with similar levels of soybean protein addition. The most yellow bread crumb was produced with the addition of 8% yellow pea protein in AC Barrie, Burnside and ES 77, and with the addition of 8% chickpea protein in Burnside.
White-Salted Noodle
The compression stress, a measure of noodle chewiness,[Citation24] of white salted noodles prepared from the composite flours was evaluated (). The effect of legume addition on noodle compression stress was positively related to the inherent dough strength (stability) and negatively related to the protein content of the flours; flours with long stability and lower protein content were less affected by legume protein addition (, , and ). The noodle compression stress of ES 77 composite flours, with the longest stability and one of the lowest protein contents, was not significantly affected by type or amount of legume protein. Glenlea, with intermediate CWES stability and lower protein content, had increased noodle compression with the addition of 5% and 8% yellow pea protein and 8% chickpea protein. Burnside, with the lowest stability of the CWES wheats and the highest overall protein content, showed significant effects of legume protein on noodle compression stress; all yellow pea and chickpea protein additions and 8% soybean protein addition to Burnside produced a significant increase in compression stress. Noodle compression stress was also significantly affected in the composite flours derived from AC Barrie, the cultivar with the lowest stability and intermediate protein content. Noodle compression stress increased in AC Barrie with the addition of 5% and 8% soybean, yellow pea or chickpea protein.
Figure 5 White-salted noodle texture of composite flour blends. (A) Compression stress; (B) Cut stress; Soy = Soybean protein; YP = Yellow pea protein; CP = Chickpea protein.
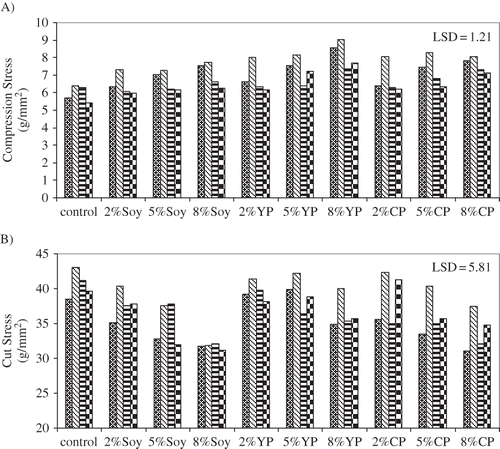
Oh et al.[Citation25] reported that the bonds between surface starch and proteins were stronger in hard wheat noodles than soft wheat noodles. The cooking process affected these noodles differently with the result that for soft wheat noodles, large amounts of protein/starch were removed during cooking resulting in a smooth and firm surface with the swollen and gelatinized starch. In this study, the legume proteins may have disrupted the wheat protein/starch matrix, resulting in greater surface loss of these components and consequently firmer noodles, analogous to the soft wheat noodle findings of Oh et al.[Citation25] Wheat cultivars with long farinograph stability and low protein content were able to withstand the effects of legume addition on noodle compression stress better than those with weaker dough properties and high protein content. Therefore, quality of the flour proteins accounted for noodle quality more than protein quantity.
Noodle cut stress, a measurement related to the bite characteristics[Citation24], was determined (). Yellow pea protein had no effect on noodle cut stress of any cultivars. Nielsen et al.[Citation26] reported no significant change in the cooked pasta texture with as much as 33% addition of pea protein to wheat flour. For soybean and chickpea proteins, the noodle cut stress generally decreased as the amount of legume protein increased across all cultivars. The noodle cut stress was more dependent on the legume protein type than on the stability and protein content of the wheat flours, in contrast to the compression stress. Soybean protein addition significantly reduced noodle cut stress compared to the controls at the 5% level for Glenlea and at the 8% level for all cultivars. Chickpea protein addition significantly reduced noodle cut stress compared to the controls at all levels in ES 77 and at the 8% level in AC Barrie. Oh et al.[Citation27] found that high protein noodles were firmer internally than low protein noodles. The addition of legume proteins to wheat flours in this study reduced the functionality of the gluten, thereby affecting the gluten matrix and reducing internal noodle cutting stress.
The color trends of the white-salted noodles produced from wheat-legume composite flours were similar to bread with a few exceptions (data not shown). Noodle yellowness (b*) and redness (a*) increased significantly from the controls at lower additions of legume protein than in bread. Noodle brightness (L*) generally decreased with 5% and 8% additions of legume proteins to the composite flours.
CONCLUSION
The effects of soybean, yellow pea and chickpea proteins on the physical, chemical and end-product properties of wheat-legume composite flours were evaluated in this study. The significance of the legume protein additions on wheat was dependent on the flour properties and end-product qualities of the individual wheat cultivars and the type and level of legume protein incorporated. In general, soybean protein could be added at the 2% level to wheat flour without substantial changes to bread and noodle quality. On average, the addition of 2% soybean protein to wheat flour would increase lysine content by 28%, a substantial increase in nutritional value of composite flour based foods. Color and qualities of end-products were significantly affected when flours were substituted with greater than 2% soybean protein or other types of legume proteins. The CWES wheat cultivars (Burnside, Glenlea, ES 77) with extra-strong dough properties were not able to compensate for the negative baking effects of the legume proteins any more than the CWRS wheat cultivar, AC Barrie. Among the three CWES cultivars studied, Glenlea was most similar to AC Barrie in the tolerance of legume protein addition in overall bread quality. The addition of legume proteins affected the textures of white-salted noodles from all wheat cultivars. Legume proteins increased compression stress and/or reduced cut stress of white-salted noodles and may perhaps be an advantage to those wheat flours that inherently lack ideal noodle textures. Overall, legume protein addition significantly changed the color and the processing quality compared to the original wheat flour based end-products. Future studies should include sensory evaluation to determine the upper levels of legume protein addition with consequent change in quality that consumers will accept in order to reap the nutritional benefits of wheat-legume composite flour based foods.
ACKNOWLEDGMENTS
Authors thank Faurshou Farms and the AAFC Matching Investment Initiative for the financial support of this project. We also thank the Wheat Quality Research Team of the Cereal Research Centre of Winnipeg and the Food Research and Development Centre of Saint-Hyacinthe for technical assistance and Shelia Woods for statistical advice.
Notes
REFERENCES
- Fleming , S.E. and Sosulski , F.W. 1977 . Breadmaking properties of four concentrated plant proteins . Cereal Chem. , 54 ( 5 ) : 1124 – 1140 .
- Hsu , D.L. , Leung , H.K. , Morad , M.M. , Finney , P.L. and Leung , C.T. 1982 . Effect of germination on electrophoretic, functional, and bread-baking properties of yellow pea, lentil, and faba bean protein isolates . Cereal Chem. , 59 ( 5 ) : 344 – 350 .
- Ranhotra , G.S. and Loewe , R.J. 1974 . Breadmaking characteristics of wheat flour fortified with various commercial soy protein products. Cereal Chem . 51 : 629 – 634 .
- Repetsky , J.A. and Klein , B.P. 1981 . Partial replacement of wheat flour with yellow field pea flour in white pan bread . J. Food Sci. , 47 : 326 – 329 .
- Friedman , M. 1996 . Nutritional value of proteins from different food sources. A review . J. Agric. Food Chem. , 44 : 6 – 29 .
- Bell , J.M. 1975 . “ Utilization of protein supplements in animal feeds ” . In Oilseed and Pulse Crops in Western Canada—A Symposium , Edited by: Harapiak , J.T. 633 – 656 . Calgary, , Canada : Western Co-Operative Fertilizers Limited .
- Bushuk , W. and Wrigley , C.W. 1974 . “ Proteins: Composition, Structure and Function ” . In Wheat: Production and Utilization , Edited by: Inglett , G.E. 129 – 145 . Westport, CT : The AVI Publishing Company Inc .
- Nestares , T. , López-Frías , M. , Barrionuevo , M. and Urbano , G. 1996 . Nutritional assessment of raw and processed chickpea (Cicer arietinum L.) protein in growing rats . J. Agric. Food Chem. , 44 : 2760 – 2765 .
- Fleming , S.E. and Sosulski , F.W. 1978 . Microscopic evaluation of bread fortified with concentrated plant proteins . Cereal Chem. , 55 ( 3 ) : 373 – 382 .
- Deshpande , S.S. , Rangnekar , P.D. , Sathe , S.K. and Salunkhe , D.K. 1983 . Functional properties of wheat-bean composite flours . J. Food Sci. , 48 : 1659 – 1662 .
- EL-Adawy , T.A. 1995 . Effect of sesame seed proteins supplementation on the nutrition, physical, chemical and sensory properties of wheat flour bread . Plant Foods for Human Nutrition. , 48 : 311 – 326 .
- Knorr , D. and Betshart , A.A. 1978 . The relative effect of an inert substance and protein concentrates upon loaf volume of breads . Lebensm.-Wiss. u.-Technol. , 11 : 198 – 201 .
- Raidl , M.A. and Klein , B.P. 1983 . Effects of soy or field pea flour substitution on physical and sensory characteristics of chemically leavened quick breads . Cereal Chem. , 60 ( 5 ) : 367 – 370 .
- Lukow , O.M. 2006 . “ Wheat Flour Classification ” . In Bakery Products Science and Technology , Edited by: Hui , Y.H. , Corke , H. , DeLeyn , I. , Nip , W.K. and Cross , N. 69 – 86 . Ames, IA : Blackwell Publishing .
- American Association of Cereal Chemists (AACC) . 2002 . “ Approved Methods of the AACC ” . In Methods 44-15A, 08-01, 46-30, 54-21 and 10-10B , 10th , St. Paul, MN : The Association .
- Ambalamaatil , S. , Lukow , O.M. , Hatcher , D.W. , Dexter , J.E. , Malcolmson , L.J. and Watts , B.M. 2002 . Milling and quality evaluation of Canadian hard white spring wheats . Cereal Foods World , 47 ( 7 ) : 319 – 327 .
- Kovacs , M.I.P. , Fu , B.X. , Woods , S.M. , Dahlke , G. , Wang , C. , Sarkar , A.K and Khan , K. 2003 . A small scale laboratory noodle sheeting machine . J. of Texture Studies , 33 : 559 – 569 .
- Oh , N.H. , Seib , P.A. , Deyoe , C.W. and Ward , A.B. 1983 . Noodles I. Measuring the textural characteristics of cooked noodles . Cereal Chem. , 60 : 433 – 438 .
- Ribotta , P.D. , Arnulphi , S.A. , León , A.E. and Añón , M.C. 2005 . Effect of soybean addition on the rheological properties and breadmaking quality of wheat flour . J. Sci. Food Agric. , 85 : 1889 – 1896 .
- Suhendor , E.L. , Waniska , R.D. and Rooney , L.W. 1993 . Effects of added proteins in wheat tortillas . Cereal Chem. , 70 ( 4 ) : 412 – 416 .
- Gonzalez-Agramon , M. and Serna-Saldivar , S.O. 1988 . Effect of defatted soybean and soybean isolate fortification on the nutritional, physical, chemical and sensory properties of wheat flour tortillas . J. Food Sci. , 53 ( 3 ) : 793 – 797 .
- Morad , M.M. , EL-Magoli , S.B. and Afifi , S.A. 1980 . Macaroni supplemented with lupin and defatted soybean flours . J. Food Sci. , 45 : 404 – 405 .
- Silaula , S.M. , Lorimer , N.L. , Zabik , M.E. and Uebersax , M.A. 1989 . Rheological and sensory characteristics of bread flour and whole wheat flour doughs and breads containing dry-roasted air-classified pinto and navy bean high-protein fractions . Cereal Chem. , 66 ( 6 ) : 486 – 490 .
- Hatcher , D.W. , Lukow , O.M. and Dexter , J.E. 2006 . Influence of environment on Canadian hard white spring wheat noodle quality . Cereal Foods World , 51 ( 4 ) : 184 – 190 .
- Oh , N.H. , Seib , P.A. , Deyoe , C.W. and Ward , A.B. 1985 . Noodles II. The surface firmness of cooked noodles from soft and hard wheat flours . Cereal Chem. , 62 ( 6 ) : 431 – 436 .
- Nielsen , M.A. , Sumner , A.K. and Whalley , L.L. 1980 . Fortification of pasta with pea flour and air-classified pea protein concentrate . Cereal Chem. , 57 ( 3 ) : 203 – 205 .
- Oh , N.H. , Seib , P.A. , Ward , A.B. and Deyoe , C.W. 1985 . Noodles IV. Influence of flour protein extraction rate, particle size and starch damage on the quality characteristics of dry noodles . Cereal Chem. , 62 ( 6 ) : 414 – 446 .