Abstract
The effect of hydrocolloid pre-treatment, i.e., pectin, carboxy methyl cellulose, xanthan gum and sodium alginate on textural properties, drip losses and sensory quality as well as on glass transition temperature (Tg′′′) of the frozen-thawed pre-cut carrots was studied. Untreated frozen samples showed detrimental effects in texture and also excessive drip losses while the carrot tissue integrity was well retained in the hydrocolloid pre-treated samples. As the concentration of the hydrocolloid increased, hardness and fracturability were also found to increase. However, adhesiveness was observed to follow a reverse trend. Xanthan gum (0.4%) resulted in higher texture retention than other hydrocolloids used as well as than the control samples. Hydrocolloids imparted lightness and higher red and yellowness to the carrot samples due to reduced solute mobility and moisture conditioning effects. Overall acceptability of the hydrocolloid pre-treated samples was found to be more than the experimental control samples. All the hydrocolloids were found to be effective in increasing the Tg′′′ to an extent, −2.73 to −0.28°C compared with Tg′′′ (−5.4°C) of untreated carrot samples. Tg′′′ increased to almost 74% in CMC (0.4%) pre-treated samples. The maximum enhancement was found in carboxy methyl cellulose followed by pectin, sodium alginate, and xanthan gum. The threshold concentrations in terms of sensory attributes were determined for optimal conditioning of the product prior to freezing.
INTRODUCTION
Frozen foods form an important aspect of processed foods due to several advantages inclusive of retention of better sensory attributes such as flavor with minimal alterations in the composition of heat sensitive vital nutrients. However, freezing and thawing of fruits and vegetables can have deleterious effects on certain physical attributes, i.e., texture and excessive soluble solid and water loss. When the water freezes it expands and the ice crystals cause the cell walls to rupture. When a frozen food is thawed for consumption, the moisture is readily separated from the matrix and it causes softening of the texture, drip loss, and often deterioration of the overall quality.[Citation1,Citation2] The quality of frozen foods depends on a number of factors inclusive of size of the ice crystals, rapidity of freezing, and the type of product as such.[Citation3–5] Physicochemical conditioning of the product could be helpful in retaining the overall quality in terms of texture and drip losses in addition to rapid freezing protocols.
Hydrocolloids are high molecular weight polysaccharides used in various applications in foods. They are known to render structural stability and also improve water holding capacity of foods.[Citation6,Citation7] Pectin is used in food industry to improve the dispersion, emulsification, and stability of foods.[Citation8] Carboxy methyl cellulose (CMC), a synthetic gum is a cellulose derivative and forms a three dimensional network with an ability to link water molecules within the system.[Citation9] Xanthan gum hydrates in cold water because of the large side chains around the cellulose backbone. It is stable against freezing and thawing and exhibits resistance to a wide range of pH conditions. Alginates are high molecular weight salts of alginic acid with block structure, which gives them the ability to cross-link and bind.[Citation10] The control of water through the use of hydro dynamically active ingredients is critical to maintaining texture and physical stability of the foods having relatively high moisture contents.[Citation11] Softening of texture as a result of cell wall rupture due to freezing could be reduced by hydrocolloid pre-treatments. Hydrocolloids (xanthan, guar, carrageenan and pectin) and dairy proteins (sodium casienate and whey protein concentrate) possess cryoprotectant properties.[Citation12,Citation13] Resistance to repeated-freezing and thawing in rice starch gel can be increased by using xanthan gum.[Citation14] The effect of individual hydrocolloids and mixture of hydrocolloids and dairy proteins (sodium casienate and whey protein concentrate) were studied in frozen and thawed pureed cooked potatoes.[Citation15,Citation16] Application of hydrocolloids is often followed to impart higher consistency in syrups[Citation6] and improve textural attributes of fruit leathers.[Citation7]
Frozen food quality during storage largely depends on the storage temperature which in turn is dependent on the glass transition temperatures (Tg ″′) of the product beyond which the chemical reactivity is enhanced due to increase in solute mobility. The temperatures associated with the transformations of the unfrozen phase such as Tg ″′ markedly affects the stability of the frozen product.[Citation17] Glass transition temperatures are the common markers used to check the stability of the frozen products. The information of maximally freeze-concentrated glass defines the limit state for the unfrozen matrix in frozen systems.[Citation18] Chemical and physical stability of frozen foods depends on the molecular mobility of the unfrozen phase, which could be quantified as a function of Tg ″′.[Citation19] The higher the storage temperature is above the Tg ″′, the less viscous the unfrozen matrix becomes, allowing faster diffusion to occur.[Citation20] As the storage temperature is increased above the Tg ″′, the amount of unfrozen water mobilizing solutes in the viscous matrix increases. The increased mobility of solutes allow enzymic and oxidation reactions to proceed and increases degradation in the product during frozen storage.[Citation21] Modifications in the Tg ″′ by use of hydrocolloids could be helpful in reducing chemical reactivity and quality changes inclusive of oxidative deterioration of vital nutrients such as carotenoids. Study of tri-stimulus color co-ordinates as a response of hydrocolloid pre-treatment would help in having an objective measurement of quality changes in terms of changes in lightness, redness and yellowness of the product such as pre-cut carrots and the same in comparison with the Tg ″′ profile would give a comprehensive understanding of solute mobility, chemical reactivity and consequent color changes.
Several reports are available on the textural aspects and tissue integrity of frozen carrots illustrating the susceptibility of carrot tissue towards freezing and also heat treatments in the form of blanching. Prestamo et al.[Citation22] described the histological changes in terms of tissue disruption and also structural collapses in pectin, which is the cementing layer between adjacent cells in frozen carrots. Fuchigami et al.[Citation23] emphasized freezing as a critical factor affecting the cell structure of carrots. Programmed freezing of carrots was found to minimize the size of ice-crystals thereby facilitating higher retention of quality. Therefore, there is a need to introduce a physicochemical conditioning in the form of pre-treatment such as hydrocolloids to minimize tissue damages during the freezing process. Commercial freezing often resorts to quick freezing by blast freezing methods by moderate blast velocities and pre-conditioning could help in minimizing the tissue damage and also drip losses which are otherwise major problems associated with frozen carrots. In the present study, various food hydrocolloids (pectin, CMC, xanthan gum, and sodium alginate) were compared for their effects in reducing the drip losses, stabilizing the texture in terms of hardness, adhesiveness, and fracturability with high sensory acceptability in the frozen carrots. Glass Transition studies were also carried out.
MATERIALS AND METHODS
Materials
Raw carrots devoid of blemishes such as visible signs of microbial infections and physical injuries were procured from the local market. Carrots after washing were peeled manually and cut into longitudinal (30 × 10 × 10 mm), as well as transverse sections in a dicer (Urschel Laboratories, Inc., USA). Calcium chloride, pectin and carboxy methyl cellulose (S. D. Fine Chemicals), xanthan gum (ICN Biomedical) and sodium alginate (Across) were procured as analytical grade chemicals.
Pre-Treatment
Diced carrots were blanched for 5 minutes at 70°C in water. After blanching carrots were dipped in 1% calcium chloride solution for two hours at room temperature (28 ± 2°C). The calcium chloride treated samples were subjected to hydrocolloid dip treatment. Diced carrots (one part) were dipped in different hydrocolloid solutions (three parts) (pectin, CMC, xanthan gum and sodium alginate) at 0.2, 0.3, and 0.4% levels for 16 hours at 5 ± 2°C. After the dip period (16 h) hydrocolloid solution was drained and the surface hydrocolloid was removed by washing in water (1:1, w/v) followed by draining. Treated carrot slices were packed and sealed in polyethylene bags (500 g each). The blanched and calcium chloride treated samples without any hydrocolloid treatment served as the experimental control.
Freezing and Thawing
Polyethylene bags containing carrot samples were frozen at −40°C for 3 h at a blast velocity of 8 m s−1 in a blast freezer (Cryoscientific, Bangalore) equipped with freezing rate controller. The frozen samples were stored in deep freezer at −20°C for the instrumental and sensory analysis. Thawing of the samples was carried at a temperature of 28 ± 1°C for 30 min for the analytical work.
Instrumental Textural Measurements
Texture profile analysis (TPA) of frozen and thawed carrot samples was carried out in four replications and the mean values were reported for hardness, fracturability, and adhesiveness. The measurements were taken using a texture analyzer (TAHDi, Stable Microsystems, London, UK) equipped with Texture Expert Software (Version 1.22; Stable Microsystems, London, UK). Samples were compressed with a load cell of 5 kg with 0.01 precision using a spherical probe of 75 mm diameter at a cross head speed of 1 mm/s to a fixed distance of 25 mm, withdrawn to the original sample height allowing the sample to recover during a fixed rest period of 5 seconds and repeating the original compression to precisely the same distance as the original penetration. The software automatically calculated the textural parameters as follows: hardness (N) is given either as the first force peak when there are two peaks on the TPA curve or by the second peak if there are three peaks. Adhesiveness (Nm) is the negative area between the point at which the first curve reached a zero force value after the first compression and the start of the second curve.
Centrifugal Drip Losses
Centrifugal drip loss was measured by modifying the procedure given by Downey.[Citation16] Approximately 3 g of sample was weighed into a paper thimble before centrifugation (MPW-350R, Med. Instruments, Poland) at 25°C at the rpm of 5000 g for 10 min. The content after centrifugation was taken out of the paper thimble, weighed and average centrifugal drip loss was reported on percentage basis. Samples were analyzed in four replicates.
Color Analysis
Frozen samples were allowed to equilibrate at room temperature (∼28°C) for 30 min. The mean CIE L*, a*, and b* values of surface of carrot samples were recorded using a colorimeter (Miniscan XE plus, Model No. 45/0-S, Hunter Associates Laboratory, Inc., Reston, VA, USA) which was calibrated using white and black standard ceramic tiles. The readings were taken using D-65 illuminant and 10O observer. Samples were analyzed in four replicates.
Glass Transition Studies
Thermal properties of carrot samples in terms of Glass Transitions were analyzed using DSC-2010 (TA Instruments, USA) equipped with a thermal analysis data station (TA Universal Analysis, Version 3.0). Samples (not more than 20 mg, wb) were weighed into an aluminum pan (900796.901, Du Pont, Wilmington, DE). The experimental temperature range was from −60 to 20°C which rises at the rate of 10°C/min. Samples were hermetically sealed and loaded into the DSC and cooled to −60°C and held at that temperature for about 5 minutes before being scanned over the temperature range set above. The DSC analyzer was calibrated using indium and an empty aluminum pan was used as reference. Thermal properties such as onset temperature (To); peak temperature (Tp), and glass transition temperature (Tg ″′) were calculated from DSC thermograms.[Citation24] Samples were analyzed in three replicates.
Sensory Analysis
Frozen-thawed carrot samples were served to a 10 trained member panel for sensory evaluation in terms of overall acceptability using a nine point hedonic scale.[Citation25] Panelists were scientific staffs of the laboratory who were trained in the use of attributing rating scale for the characteristics examined. The scores were assigned from extremely liked (9) to disliked extremely (1). The samples were served to the panelists after coding with three digits randomly selected numbers in a sensory lab illuminated with white light and maintained at 20°C.
Statistical Analysis
Statistical analysis was performed by analysis of variance (ANOVA) and means were compared using the least significant difference (LSD, 95%) by Duncan's multiple range tests using Statistica 7 software (StatSoft, Tulsa, Oklahama, USA).
RESULTS AND DISCUSSION
Textural Parameters
The hardness, fracturability, and adhesiveness from texture profile analysis (TPA) of the hydrocolloid pre-treated frozen-thawed carrot samples cut transversally as well as longitudinally are presented in . Hydrocolloid pre-treatment significantly (P < 0.05) affected all the textural parameters. In case of longitudinally cut samples, a value of 0.838 ± 0.04 N for hardness, 0.075 ± 0.015 N for fracturability and 0.992 ± 0.005 Nm for adhesiveness was recorded in the experimental control samples through the TPA analysis. As the concentration of hydrocolloids increased, hardness values were also found to increase. The hardness of pectin, CMC, xanthan and sodium alginate treated longitudinally cut samples ranged from 1.26 to 1.80 N, 1.42 to 2.07 N, 1.59 to 2.61 N, and 1.33 to 1.85 N, respectively. Similarly the hardness of pectin, CMC, xanthan and sodium alginate treated transversally cut samples ranged from 1.41 to 1.96 N, 1.53 to 2.22 N, 1.73 to 2.74 N, and 1.49 to 2.25 N, respectively. Xanthan (0.4%) showed maximum increase in hardness from the control followed by CMC, sodium alginate and pectin, respectively in both the samples. The increased hardness of the hydrocolloid pre-treated samples might be due to the property of hydrocolloids to bind water and prevent excessive moisture loss during freezing and thawing. Increasing hydrocolloid concentration was accompanied by decrease in adhesiveness values. Xanthan gum showed maximum reduction in adhesiveness values followed by pectin, sodium alginate and carboxy methyl cellulose. An increase of fracturability in longitudinally and transversally cut carrot samples was found due to hydrocolloid pre-treatment. But the increase was statistically insignificant (P > 0.05) over the control as well as among the hydrocolloids. Though the increase in fracturability was not much significantly (P > 0.05) different from the control, xanthan gum showed higher values followed by pectin, sodium alginate and CMC. Overall, higher textural values were recorded in transversally cut carrot samples in terms of hardness and fracturability. Significant (P < 0.05) differences in hardness and adhesiveness were recorded for different hydrocolloids pretreated samples of transversally and longitudinally cut carrot samples. Higher concentration of hydrocolloids imparted significant differences on fracturability however; it was non-significantly affected by mode of cutting the samples.
Table 1 Effect of different hydrocolloid pre-treatments on instrumental texture parameters of frozen-thawed pre-cut carrot (n = 4)
Reduction in the deterioration of food products due to freezing by using hydrocolloids have been reported by several authors. Deteriorating effect from freezing in wheat flour and corn flour pastes can be reduced by xanthan.[Citation26] The retention of texture as observed by increased textural parameters over the experimental control could be due to interaction of the hydrocolloids with water. According to Lee et al.[Citation27] the hydrodynamicproperties of hydrocolloids is due to water binding and its distribution between particulates and matrix. In the present study xanthan gum was found to improve textural attributes in pre-cut carrot in frozen condition. However, the threshold values of xanthan gum at 0.3% concentration in terms of sensory scores discussed elsewhere need to be followed and the textural attributes showed significant improvement at 0.3% hydrocolloid concentration.
Drip Loss
Thawing of frozen products results in drip losses in which soluble solids leaches out along with water. Effect of different hydrocolloid pre-treatments on the retention of drip losses are predicted in . A maximum drip loss of 66.69% was observed in the control samples while; all the hydrocolloids were found to decrease the drip losses significantly (P < 0.05). Xanthan gum was more effective in decreasing the drip losses followed by pectin, CMC and sodium alginate, respectively. Pre-treatment of carrots in 0.4% xanthan gum showed maximum reduction in drip losses. It could reduce the drip losses up to 34.97%. The drip loss in xanthan pre-treated samples were found to be 48.69, 47.28, and 43.37% at 0.2, 0.3, and 0.4%, respectively. Drip losses in pectin pre-treated samples at 0.2, 0.3 and 0.4% were 52.94, 48.04, and 47.58%, respectively. Drip losses associated with 0.2, 0.3 and 0.4% CMC and alginate pre-treatments were also significant (P < 0.05) over the experimental control with values 53.55, 51.08, and 47.98% and 55.28, 52.49, and 49.64%, respectively. Reduction in drip losses of the hydrocolloid pre-treated samples could be attributed to the water-binding properties of the hydrocolloids.
Color Measurements
Changes in CIE color values in terms of L*, a*, and b* values are recorded in . Color value was found to increase as compared to control with the increase in the concentration of different hydrocolloids in terms of L, indices. The effect of increased hydrocolloid concentration on color was found to be most pronounced with respect to pectin followed by CMC, xanthan and sodium alginate, respectively. The increase in lightness could be attributed to the moisture conditioning effect of hydrocolloids. The redness of the samples decreased as the concentration of hydrocolloids increased. However, the recorded a* values for the hydrocolloid pre-treated samples were higher than the control samples. The changes in a* values were maximum in Pectin. The reduction in redness of the carrot samples might be due to the lightening effect of the hydrocolloids. The yellowness was found to follow similar trend like L values. Yellowness (b* values) of the carrot samples increased due to the hydrocolloid pre-treatment. Visual appearance of xanthan and alginate pre-treated samples were more appealing than the pectin and CMC pre-treated samples due to less changes in the color values. The color stabilization in terms of L*, a*, and b* values could be attributed to increase in the Tg ″′ values of the product causing restriction in solute mobility and chemical reactivity. These aspects could result in stabilization of carotenoids with restriction in oxidation. Lim et al.[Citation21] reported a profound influence of Tg ″′ on oxidation susceptible constituents such as chlorophyll and ascorbic acid. However, the effects of Tg ″′ on individual color entity specific and anthocyanins were reported to be less dependent on Tg ″′ values during freezing and subsequent storage.[Citation18] In the present study the changes seen in L*, a*, and b* values show a significant deviation from the control samples highlighting more lightness, redness and yellowness which could be attributed to moisture conditioning and carotenoids stabilization during the freezing and thawing processes.
Table 2 Instrumental color values and overall acceptability of the hydrocolloid pre-treated frozen-thawed pre-cut carrot (n = 4, nx = 10)
Sensory Acceptability
shows average values of sensory preference of hydrocolloid pre-treated frozen- thawed pre-cut carrot samples, as well as of the experimental control samples in terms of overall acceptability (OAA). The hydrocolloid pre-treatment significantly (P < 0.05) affected the OAA. Increase in pectin and CMC concentrations from 0.2 to 0.4% was accompanied by increase in the OAA as compared to the experimental control. Xanthan pre-treatments affected the OAA differently. Different concentrations exhibited different affects on OAA. Increasing the gum concentrations resulted in increased sensory scores. This could be due to the ability of the hydrocolloid to bind water within the carrot tissue, which in turn improved structural integrity and resulted in higher sensory scores than the control. But, when excess xanthan (0.4%) was used, however the tendency of gum to impart thickening could cause the adverse effect. As a result, 0.4% xanthan pre-treatment showed lower sensory scores. As the concentration of sodium alginate increased the sensory scores also decreased. The sensory acceptability of 0.2% alginate was more than the other two concentrations this might be due to the block structure of alginate, which gave the product inferior appearance, chewiness, and taste and resulted in lower OAA.
Thermal Properties
Tg ″′ of the carrot samples was analyzed after removing the surface moisture on the samples using partial air drying. The thermograms indicating the Tg ″′ of the carrot samples pre-treated with different hydrocolloids at 0.2, 0.3, and 0.4% levels are shown in . All the hydrocolloids were found to increase the Tg ″′ significantly (P < 0.05). Tg ″′ was also found to increase with increasing concentrations. Rizzolo et al.[Citation28] reported that addition of carbohydrates such as maltose could shift the Glass transition to higher temperatures in frozen blueberry juices. Lim et al.[Citation21] compared the Tg ″′ of tender and old peas and reported that higher Tg ″′ values in old peas was due to higher amount of starch content than the tender peas. CMC pre-treatment showed higher increase in the Tg ″′ values followed by pectin, sodium alginate and xanthan as compared to experimental control. The onset temperature, peak temperature and glass transition temperature are shown in . Maximum increase in Tg ″′ (74%) was observed in the samples pre-treated with 0.4% level of CMC. The glass transition temperature shifted from −5.4°C of control carrot samples to −1.50, −0.99, and −0.28 in carrot samples pre-treated with CMC at 0.2, 0.3 and 0.4% levels, respectively (). Increase in Tg ″′ associated with 0.2, 0.3, and 0.4% pectin, alginate and xanthan pre-treatments were also significant over the experimental control with values −1.41, −1.05, and −0.63; −2.73, −1.74, and −1.28, and −2.60, −2.17, and −1.39°C, respectively.
Figure 2 DSC thermograms showing glass transition temperatures (Tg ″′) of the carrot samples pre-treated with (a) CMC, (b) Pectin, (c) Sodium Alginate, and (d) Xanthan gum. (Figure provided in color online.)
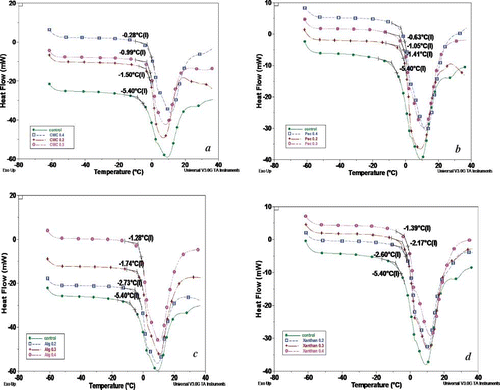
Table 3 Glass transition temperatures (Tg ″′), Onset temperature (To ) and Peak temperatures (Tp ) of the hydrocolloid pre-treated frozen-thawed pre-cut carrot (n = 3)
In the present study it is highlighted that pre-treatment with hydrocolloids is as such effective in terms of improvement in textural profile without softening and excessive drip losses. The enhancement in Tg ″′ values could not only result in color stabilization but also in enhancement of requisite storage temperature which needs to be equal to or lesser than the Tg ″′. However, the sensory scores need to be the markers in establishment of maximum levels for commercial usage. The pre-treatment with hydrocolloids is as such cost effective and could be used in combination with quick freezing techniques to maximize the quality attributes of frozen carrots in pre-cut form.
CONCLUSIONS
Textural parameters of hydrocolloid pre-treated samples were significantly higher than the respective non-treated samples when measured after thawing. This indicated a protective effect of hydrocolloids on cell integrity, which is altered during the freezing process. Xanthan gum (0.4%) concentration showed best results in terms of mechanical texture. Mode of cutting significantly affected the textural properties in terms of hardness and adhesiveness of pre-cut carrot treated with different levels of various hydrocolloids. Sensory acceptability of pre-cut frozen carrot also increased due to hydrocolloid treatment. Acceptability of xanthan gum pre-treated product at 0.3% concentration was observed maximum compared with other hydrocolloids. It can be concluded that all selected hydrocolloids (pectin, CMC, xanthan gum and alginate) were useful in reducing the drip losses, improving the texture as well as sensory acceptability to different extents. Tg ″′ was also found to increase with increasing concentrations of hydrocolloid with the effect being more pronounced with CMC followed by pectin, sodium alginate and xanthan gum as compared to control samples in untreated frozen and thawed condition.
ACKNOWLEDGMENT
The valuable suggestions by Dr. Alok Saxena during manuscript preparation are gratefully acknowledged.
REFERENCES
- Goodenough , P.W. and Atkin , R.K. 1981 . Quality in stored and processed vegetables and fruit , 373 London , , UK : Academic Press .
- Rahman , M.S. 1999 . “ Food preservation by freezing ” . In Handbook of Food Preservation , Edited by: Rahman , M.S. 259 – 284 . New York : Marcel Dekker .
- Fuchigami , M. , Hyakumoto , N. , Miyazaki , K. , Nomura , T. and Sasaki , J. 1994 . Texture and histological structure of carrots frozen at a programmed rate and thawed in an electrostatic field . Journal of Food Science , 59 : 1163 – 1168 .
- Fuchigami , M. , Kato , N. and Teramoto , A. 1997 . High pressure freezing effects bon textural quality of carrots . Journal of Food Science , 62 ( 4 ) : 804 – 808 .
- Li , B. and Sun , D.-W. 2002 . Novel methods for rapid freezing and thawing of foods-a review . Journal of Food Engineering , 54 : 175 – 182 .
- Sikora , M. , Juszczak , L. and Sady , M. 2003 . Hydrocolloids in forming properties of cocoa syrups . International Journal of Food Properties , 6 : 215 – 228 .
- Gujral , H.C. and Brar , S.S. 2003 . Effect of hydrocolloids on the dehydration kinetics, color, and texture of mango leather . International Journal of Food Properties , 6 : 269 – 279 .
- Sawayama , S. and Kawadata , A. 1991 . Effects of salts on thermal properties of pectin solution on freezing and thawing . Food Hydrocolloids , 5 ( 4 ) : 393 – 405 .
- Andrew , C. H. 2004 . Hydrocolloids: practical guide for the food industry , 41 – 65 . St. Paul , MN : Eagan Press Handbook .
- Gurkin , S. 2002 . Hydrocolloids- Ingredients that add flexibility to tortilla processing . Cereal Foods World , 47 : 41 – 43 .
- Lee , C.M. 2002 . Role of hydrodynamically active biopolymeric ingredients in texture modification and physical stabilization of gel-based composite foods . Journal of Food Science , 67 : 902 – 908 .
- Tsuchiya , Y. , Nomura , Y. and Matsumoto , J. 1975 . J. Prevention of freeze denaturation of carp actomyosin by sodium glutamate . Journal of Biochemistry , 77 : 853 – 862 .
- Holt , C. 1991 . “ The effect of polymers on ice crystal growth ” . In Food Freezing: Today and Tomorrow , Edited by: Bald , W.B. 81 – 86 . London : Springer-Verlag .
- Lo , C.T. and Ramsden , L. 2000 . Effects of xanthan and galactomannan on the freeze/thaw properties of starch gels . Nahrung , 44 : 211 – 214 .
- Downey , G. 2002 . Quality changes in frozen and thawed, cooked pureed vegetables containing hydrocolloids, gums and dairy powders . International Journal of Food Science and Technology , 37 : 869 – 877 .
- Downey , G. 2003 . Effects of cryopotectant mixtures on physical properties of frozen and thawed pureed cooked potatoes: Some introductory studies . International Journal of Food Science and Technology , 38 : 857 – 868 .
- Roos , Y. 1995 . Characterization of food polymers using state diagrams . Journal of Food Engineering , 24 : 339 – 360 .
- Reid , D.S. , Kerr , W. and Hsu , J. 1994 . The glass transition in the freezing process . Journal of Food Engineering , 22 : 483 – 494 .
- Torreggiani , D. , Forni , E. , Guercilena , I. , Maestrelli , A. , Bertolo , G. , Archer , G.P. , Kennedy , C.J. , Bone , S. , Blond , G. , Lopez , E.C. and Champion , D. 1999 . Modification of glass transition temperature through carbohydrates additions: effect upon color and anthocyanin pigment stability in frozen strawberry juices . Food Research International , 32 : 441 – 446 .
- Slade , L. and Levine , H. 1995 . Glass transitions . Advances in Food and Nutrition Research , 38 : 103 – 269 .
- Lim , M. , Wu , H. , Breckell , M. and Birch , J. 2006 . Influence of the glass transition and storage temperature of frozen peas on the loss of quality attributes . International Journal of Food Science and Technology , 41 : 507 – 512 .
- Prestamo , G. , Fuster , C. and Risueno , M. 1998 . C. Effects of blanching and freezing on the structure of carrots cells and their implications for food processing . Journal of Science of Food and Agriculture , 77 : 223 – 229 .
- Fuchigami , M. , Hyakumoto , N. and Miyazaki , K. 1995 . Programmed freezing affects texture, pectic composition, and electron microscope structure of carrots . Journal of Food Science , 60 : 137 – 141 .
- Goff , H.D. 1994 . Measuring and interpreting the glass transition in frozen foods and model systems . Food Research International , 27 : 187 – 189 .
- Larmond , E. 1977 . Laboratory methods for sensory evaluation of foods , 1637 Ottawa , ON : Canada Department of Agric Publication .
- Ferrero , C. , Martino , M.N. and Zaritzky , N.E. 1993 . Stability of frozen starch pastes: effect of freezing, storage and xanthan gum addition . Journal of Food Processing and Preservation , 17 : 191 – 211 .
- Lee , M.H. , Baek , M.H. , Cha , D.S. , Park , H.J. and Lim , S.T. 2002 . Freeze-thaw stabilization of sweet potato gel by polysaccharide gums . Food Hydrocolloids , 16 : 345 – 352 .
- Rizzolo , A. , Nani , R.C. , Viscardi , D. , Bertolo , G. and Torreggiani , D. 2003 . Modification of glass transition temperature through carbohydrates addition and anthocyanins and soluble phenol stability of frozen blueberry juices . Journal of Food Engineering , 56 : 229 – 231 .