Abstract
Expanded snacks based on Moringa (Moringa oleifera) leaf powder (MLP) and oat flour were produced using a lab-scale twin-screw extruder. Four levels of MLP (0, 15, 30, and 45%, dry blend basis) and three levels of in-barrel moisture content (19, 22, and 26%, wet basis) were studied. Expansion of extrudates decreased as MLP level increased. On the other hand, expansion was positively affected by in-barrel moisture for all treatments with MLP. The role of MLP and in-barrel moisture in modifying phase transition, water binding, and gas-holding capacity of the extrusion melt was evaluated and related to product properties.
INTRODUCTION
Moringa (Moringa oleifera), a tropical tree with multiple uses, is resistant to drought, particularly easy to reproduce, and grows very fast.[Citation1] The numerous economic uses of Moringa, together with its easy propagation, have resulted in growing international interest in this tree, which originated from India and is now found in most tropical countries of western Africa and south and south-eastern Asia. Parts of the Moringa tree, including the leaves, are part of the traditional human diet in the above regions. Perhaps more than any other tropical vegetable, Moringa leaves are an important source of several nutrients.[Citation2–5] One hundred grams of dried leaves contain 27.1 g protein, 16.3 mg vitamin A, 17.3 mg vitamin C, 2.0 g calcium, 1.3 g potassium, and 28.2 mg iron, in addition to 19.2 g dietary fiber and several other nutrients.[Citation5]
Snack foods is one of the fastest growing segments of the food industry[Citation6]; however, the delivery of nutrition via incorporation of fruits and vegetables in ready-to-eat expanded snacks is a relatively new concept and is motivated mainly by health concerns. The most effective means of achieving this is via extrusion processing, a continuous cooking and puffing technology that has gained widespread use in the last several decades for the production of a variety of expanded snacks and breakfast cereals.[Citation7, Citation8] Several recent studies have focused on using extrusion processing for incorporation of fruit- and vegetable-based ingredients in expanded snacks.[Citation9–14]
The extrusion process involves application of heat, moisture, and shear to a combination of biopolymers, such as starch and protein usually from cereal sources, leading to a viscoelastic melt in the extruder barrel.[Citation7, Citation12] This melt is pressurized in the barrel, heated to temperatures above 100°C, and forced to exit the extruder die. The sudden drop in pressure at the extruder die rapidly converts the compressed liquid water into steam. This flash-off of internal moisture causes the melt to explosively expand and attain a cellular structure. The extrudate structure is stabilized as the matrix transforms to a glassy state on cooling and drying. The combination of moisture, sheer, temperature, and pressure causes rapid cooking during the extrusion process and results in gelatinization of starch, denaturation of protein, inactivation of many native enzymes that causes food deterioration during storage, destruction of naturally occurring toxic substances, and diminishing microbial counts in the final product.[Citation7, Citation15, Citation16]
However, the presence of fruit and vegetable components, such as sugar, fiber, and, in some cases, protein, complicates the transformations during extrusion that are responsible for forming an expanded structure, can lead to processing difficulties, and may also be detrimental to key quality parameters in snacks.[Citation10, Citation11, Citation14, Citation17] This study attempts to understand this in a systematic fashion by using Moringa leaf powder as a model vegetable ingredient in oat-based extruded snacks. Physical properties of the raw materials were studied using both novel and traditional analytical methods, and these properties were related to product attributes with the goal of developing a conceptual model for the critical interactions and phenomena during extrusion when vegetable ingredients are a significant part of the formulation. Consumer acceptability of the Moringa leaf and oat-based extruded snacks was also evaluated.
MATERIALS AND METHODS
Materials
The ingredients used in the extrusion study were Moringa leaf powder (MLP), dehulled oat flour, and corn starch. In addition, potato flour, onion powder, garlic powder, black pepper powder, and vegetable oil were used for coating the products prior to the consumer acceptance study. MLP was obtained from Trees for Life (Wichita, KS), a non-profit organization engaged in spreading awareness about the beneficial aspects of the Moringa tree. All other ingredients were obtained from a local grocery store (Dillons, Manhattan, KS, USA).
Extrusion Processing
Four oat flour-based formulations with 0, 15, 30, and 45% MLP (dry blend basis) were extruded at three in-barrel moisture levels (19, 22, and 26% w.b.). All formulations had 10% corn starch (dry blend basis) as an expansion aid. This constituted a 4 × 3 full factorial experimental design, resulting in a total of 12 treatments. Prior to extrusion, blends of MLP, oat flour, and corn starch were prepared and hydrated with water to reach the target moisture using a bench-top mixer (KSM5; Kitchen Aid, St Joseph, MI, USA). The hydrated blends were stored overnight at 4°C for equilibration before extrusion. A lab-scale co-rotating twin-screw extruder (Micro-18, American Leistritz Extruder Corp., Somerville, NJ, USA) with a six-head configuration, barrel diameter of 18 mm, and L/D ratio of 29 was used for production of the expanded MLP-oat flour snacks. The die diameter was 3 mm. Process parameters were set to a barrel temperature profile of 85‐90‐95‐100‐110‐120°C, feed screw speed of 220 rpm, and extruder screw speed of 230 rpm based on preliminary studies. The screw configuration is shown in . The extrudates were dried in an oven at 40°C for 24 h. Three replicates were conducted for each treatment.
Moisture Content
Moisture contents of raw ingredients and extruded products before drying were determined using AACC Approved Method 44‐15A.[Citation18] Results (n = 3) were expressed as a percentage (wet basis).
Expansion Ratio and Crispness Work
Sectional expansion index (SEI) of extrudates was measured according to the formula:
where Dextrudates and Ddie are the diameters of the extrudate and die, respectively. A texture analyzer (Model TA.XT2, Stable Micro Systems, Surrey, United Kingdom) was used to measure the fracture response of extrudates under compression. The instrument was configured to compression mode, with 10 mm/s test speed and 90% distance of compression. A 38-mm-diameter probe was used to apply compression. Thirty samples for each treatment were analyzed. Samples were cut to approximately the same length for analysis, and were compressed perpendicular to the direction of extrusion. A force-deformation curve was obtained from each compression test, and the number of peaks (n) and area below the curve from 0 to 90% strain (S) were used to compute the crispness work (Wc ) as follows[Citation19, Citation20]:
Phase Transition Properties
The phase transition analyzer (PTATM; Wenger Manufacturing, Sabetha, KS, USA) is a relatively new analytical tool for studying physical changes associated with the glass transition and melt transition in biopolymers, such as starch and protein, during thermal processing. The PTA is a modified capillary rheometer that uses a combination of time, temperature, and pressure to measure flow-related phase transition properties, such as the softening temperature (Ts ) and flow temperature (Tf ).[Citation20–24] These parameters are analogous to the glass and melt transition temperatures, respectively, obtained by other methods.[Citation20, Citation21, Citation25]
Ts and Tf of raw blends with 0–45% MLP were measured at four moisture levels (14, 17, 20, and 23% w.b.) following the procedure outlined below. A blank die (no opening) was inserted into the PTA, a 1.85-g sample was loaded, and the pressure was preset to 10 MPa and maintained for 5 sec for compaction. The pressure was then reduced to 8 MPa, and the sample was heated at a rate of 10°C per minute with a starting temperature of −5°C. Ts was obtained as the midpoint of the temperature range over which the sample exhibited softening. After conclusion of this test, the blank die was replaced with a 2-mm capillary die and the test was continued from approximately 10°C below the Ts , at the same heating rate and operating pressure as earlier. The flow temperature (Tf ) was obtained as the temperature at which the sample started to flow through the capillary.
Pasting Properties
Pasting properties of raw blends with 0–45% MLP and the extrudates were determined using a rapid viscoanalyzer or RVA (Model 3c, Newport Scientific, Sydney, Australia). The temperature profile used for the tests was holding at 50°C (0–1 min), followed by heating (50–95°C; 1–4:45 min), holding (95°C; 4.45–7:15 min), cooling (95–50°C; 7:15–11 min), and holding again at 50°C (11–13 min). Pasting parameters, such as the peak viscosity, were obtained from each pasting curve.
Nutritional Anlaysis
Protein content of extrudates was measured with the nitrogen combustion analysis (NCA) method using a LECO nitrogen anlayzer.[Citation26] Crude fiber of extrudates was measured with the Weende method using the Tecator Fibertec System (Foss, Denmark).[Citation27]
Color
The color of extrudates was measured in triplicate by using a Chroma Meter (Minolta CR-300, Minolta Corporation, Ramsey, NJ, USA) with a standard calibration plate CR-A44 having CIELAB values of L* = 93.2, a* = 0.3139, and b* = 0.3204. In the CIELAB system of color measurement, L* indicates lightness of the sample with values from 0 for black to 100 for white. A negative value of a* indicates greenness, whereas a positive value indicates redness. A negative value of b* indicates blueness and a positive value indicates yellowness.
Coating of Extrudates
Prior to sensory evaluation, the dried extrudates were coated to about 40–50% of their weight with a slurry composed of 65.4% vegetable oil, 18.3% potato flour, and 16.3% water, and then transferred to a hot air oven and baked at 160°C for 5 min to reduce the moisture content of the coating to 2%.
Sensory Analysis
Sensory evaluation of coated extrudates was conducted in the form of a consumer acceptability test. Fifteen panelists were selected among the graduate and undergraduate students in the Department of Grain Science and Industry, Kansas State University, on the basis of their willingness to participate. Training sessions were conducted, during which panelists were presented with the products to familiarize them with the quality attributes. Panelists were asked to score the coated extrudates for color, oiliness, flavor, texture, and overall acceptability on a 9-point hedonic scale, where 9 = like extremely, 8 = like very much, 7 = like moderately, 6 = like slightly, 5 = neither like nor dislike, 4 = dislike slightly, 3 = dislike moderately, 2 = dislike very much, and 1 = dislike extremely. Means were subjected to pairwise comparisons using the Fisher's LSD procedure with Minitab Statistical Software (Minitab Inc., State College, PA, USA). The significance level was set at 95%.
RESULTS AND DISCUSSION
Residual Moisture
Moisture content (w.b.) of MLP-oat flour extrudates before the drying step, i.e., the residual moisture, is summarized in . As expected and irrespective of the MLP level, residual moisture of extrudates increased as the in-barrel or process moisture increased. MLP level had a more complex effect on residual moisture. When in-barrel moisture was a limiting factor (19% w.b.), an increase in MLP level led to higher residual moisture. This indicated reduced moisture flash-off at the die and was probably due to most of the internal moisture being bound by fiber and protein components in MLP. However, this trend was reversed at the higher in-barrel moistures of 22 and 26%, in which case residual moisture decreased as MLP level increased and indicated higher moisture flash-off. It is hypothesized that an increase in the MLP level led to greater resistance to flow and, consequently, higher mechanical energy input and melt temperature, thus causing greater moisture flash-off when in-barrel moisture was not limiting. This is difficult to support in the absence of specific mechanical energy and melt temperature data; however, the expansion and phase transition analysis data discussed below partially validate this argument.
Table 1 Residual moisture in MLP-oat flour extrudates
Expansion Characteristics
Expansion ratio data are shown in . The control formulation (0% MLP), extruded at 19% in-barrel moisture content, had the greatest expansion ratio (7.2) among all the 12 treatments. On the other hand, the 45% MLP blend extruded at the same in-barrel moisture and had the lowest expansion ratio (1.9). In general, expansion decreased with increasing Moringa leaf addition. For example, at a 45% MLP level, expansion was lower than the control by 74.0, 22.7, and 30.3% at in-barrel moisture levels of 19, 22, and 26%, respectively. This was an expected result and similar observations have been observed in other studies for extruded products with fruit and vegetable ingredients.[Citation10, Citation11, Citation14] A critical factor favoring expansion is the development of an extensible and continuous viscoelastic melt having gas holding properties. Presence of an adequate amount of gelatinized starch and absence of discrete phase fillers, such as fiber and most kinds of protein, promote formation of a gas holding matrix. Thus, it is obvious that a higher fraction of MLP in the extruded blend would lead to reduced expansion.
Figure 2 Sectional expansion index (SEI) of MLP-oat flour extrudates processed at various levels of in-barrel moisture (w.b.). Error bars represent standard deviation.
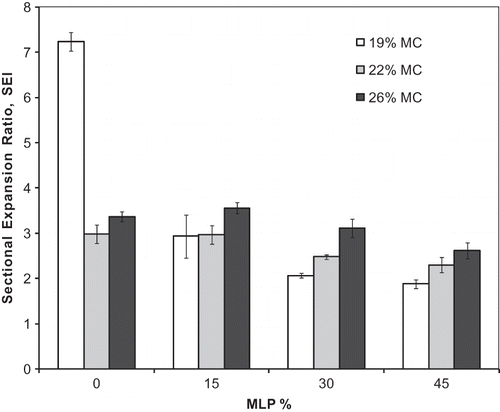
The effect of in-barrel moisture on the expansion of extrudates was more complex. For the control formulation, expansion decreased by as much as 58.9% with increasing in-barrel moisture. This was again an expected result and several studies in the past have reported similar results.[Citation7, Citation8, Citation19, Citation20, Citation28, Citation29] Water plays a critical role in the extrusion process due to its multiple roles as a plasticizer, gelatinization aid, and blowing agent. Higher in-barrel moisture results in reduced viscosity and, thus, less mechanical energy input into the melt and correspondingly lower temperatures. Water vapor pressure inside nucleated cells is a direct function of the melt temperature and water activity (or free water), and in turn drives expansion of the cells. Subsequent to expansion, it is very common for the extrudates to exhibit some degree of collapse due to elastic and surface tension forces; however, less viscous melts experience more collapse. Thus, typically the higher the in-barrel moisture, the lower is the internal water vapor pressure and the driving force for expansion. At the same time, more collapse is experienced. The end result is lower final expansion.
However, in the presence of 15–45% MLP, in-barrel moisture had a contrary effect, i.e., expansion was actually higher by as much as 22.2 and 50.7%, respectively, at 22 and 26% in-barrel moisture as compared to 19% moisture. It is hypothesized that MLP, with its fiber and protein components, had a greater water binding ability than the oat flour it replaced, leading to reduced water activity and, thus, lower internal water vapor pressure when in-barrel moisture was limiting (19%). It is also possible that preferential binding of water by MLP led to reduced availability of the former for starch gelatinization and, therefore, interfered with the formation of a gas-holding matrix. However, these drawbacks of MLP could at least partially be overcome as in-barrel moisture content was increased to 22 and 26%, leading to greater expansion. It is also noteworthy that the negative effect of MLP addition on expansion was less pronounced when in-barrel moisture was not limiting (22 and 26%). This corresponded with lower residual moistures and higher moisture flash off as discussed earlier, indicating higher water vapor pressure and driving force for expansion that balanced the negative effect of reduced starch content and higher amounts of discrete phase fillers.
Crispness Work
A typical force-deformation curve is shown in . Force levels rose with deformation, followed by a brittle crushing plateau. This roughly followed the crushing pattern of brittle foams as described by Gibson and Ashby.[Citation30] However, a densification region was not observed subsequent to the crushing plateau. Instead, test samples underwent several fractures as the compression proceeded and broke into pieces. After each fracture, compression of the remaining pieces resumed shortly, thus creating numerous sharp peaks.
Figure 3 A typical force-deformation curve for MLP-oat flour extrudates (color figure available online).
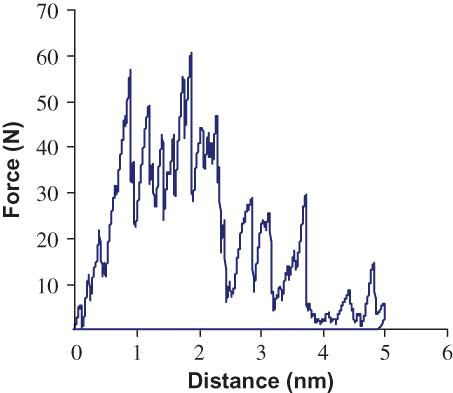
Crispness work (Wc ) data is shown in . Wc ranged from 1.5 to 4.2 N-mm, and was highest for the control formulation (0% MLP) processed at 26% in-barrel moisture content. Wc is a measure of the average energy per crushing peak in the force-deformation curve, and is directly impacted by the cellular structure and brittleness of the material making up the cell wall.[Citation19], [Citation20], [Citation29], [Citation30] In the absence of MLP, Wc was higher by 153.6 and 180.1% for products processed at 22 and 26% in-barrel moisture, respectively, as compared to the lowest moisture. Clearly, this was a result of decrease in expansion and, thus, shorter and thicker cell walls with greater resilience to fracture. Similar reasoning supported the 5.9–21.2% decrease in Wc for extrudates with 15–45% MLP processed at higher moistures, as compared to 19% in-barrel moisture. In this case, greater expansion led to longer and thinner cell walls with reduced resilience to fracture. Also, at any in-barrel moisture level, increase in MLP led to a weakening of the cell wall material because of disruption of the continuous starch matrix by the fiber and protein components. This is evident from the decrease in Wc for extrudates with 15–45% MLP by up to 37.8 and 50.3%, respectively, at 22 and 26% in-barrel moisture as compared to the control. However, an inverse trend with respect to MLP was observed for extrudates processed at 19% in-barrel moisture. This was probably due to predominance of the competing effect of lower expansion.
Phase Transition Properties
The phase transition properties, softening temperature (Ts ), and flow temperature (Tf ), of raw or unprocessed blends with 0–45% MLP are shown in . Ts of the blends ranged from 15.6–62.1°C, while Tf ranged from 62.1–136.6°C. Ts and Tf exhibited consistent decreasing trends with respect to moisture content, and were reduced by as much as 70.7 and 48.8%, respectively, as moisture increased from 14 to 23%. Similar results have been reported for various other formulations in previous studies.[Citation20–24] It has been established that Ts and Tf are correlated to the shear viscosity of the melt; thus, these results follow the well established inverse relationship between moisture and viscosity.[Citation20], [Citation21], [Citation28], [Citation31] Interestingly, a consistent increasing trend was observed for Ts and Tf with respect to MLP level, and these two parameters were higher by as much as 25.0 and 22.7%, respectively, as MLP increased from 0 to 45%. This could possibly be due to the viscosity enhancing effect of the fiber in MLP, but this was refuted by the pasting data discussed below. A more plausible reason is that MLP hindered the deformation and flow of the starch-based melt, because the constituent protein and fiber are not thermoplastic biopolymers. Addition of other high fiber ingredients to a starch-based melt has been known to negatively affect its ‘extrudability’ and these data are a direct confirmation of the same using analytical means.
Pasting Properties
Pasting curves for the unprocessed and extruded blends with 0–45% MLP are shown in . Pasting viscosity of materials depends on the viscosity building ability of starch and other components as a result of imbibing moisture and/or heat. The pasting curves of unprocessed blends exhibit a hot paste peak viscosity (75–300 RVUs) indicating swelling of starch granules due to gelatinization. This peak viscosity showed a decreasing trend with respect to MLP due to dilution of starch. The pasting curves of extruded blends exhibited higher cold paste viscosities as compared to raw blends, indicating the presence of gelatinized starch granules with enough of their structure intact to allow swelling. However, the hot paste peak viscosity (30–120 RVUs) was substantially lower as compared to the unextruded blends. This indicated degradation of starch granules and molecular breakdown during extrusion.[Citation32–35]
Nutritional Analysis
Crude fiber and protein content of the MLP-oat flour extrudates increased substantially with the addition of MLP (), indicating that Moringa leaf can enhance the nutritional properties of puffed products. The 45% MLP product had approximately 5 times more fiber content (4.2%) and 1.5 times more protein content (13.7%) than the control with no MLP (fiber = 0.8% and protein = 8.2%). Fiber and protein in a typical commercially available expanded snack are 1.0 g and 2.0 g, respectively, based on a serving size of 28 g. In contrast, the 45% MLP product had 1.2 g fiber and 3.8 g protein for the same serving size. It is important to note that the fiber levels in extrudates that are reported in are based on crude fiber analysis, which grossly underestimates the total dietary fiber. Apart from the obvious benefits of higher protein, increased fiber content has significant health benefits. Since the pioneering work of Burkitt,[Citation36] it has been widely believed that dietary fiber protects against the development of colorectal cancer and a range of other diseases, including obesity, cardiovascular ailments, and diabetes. As an oil-based coating was applied to the extruded snacks prior to sensory evaluation, the fat content of the coated product was calculated to be 6.8 g, which was 32% lower than the fat content of the commercial product mentioned above.
Table 2 Fiber and protein content of MLP-oat flour extrudates
Although the actual micronutrient profile of the extruded products was not measured, calculations based on the nutritional data available for MLP[Citation5] suggested that the uncoated extrudates contained up to 2.0 mg of Vitamin A, 3.6 mg of iron, and 250 mg of calcium per serving, which corresponds to 227, 19.7, and 25% of the recommended daily intake of these micronutrients, respectively. The commercial snack provided none to the negligible amount of these micronutrients.
Color Characteristics
Color is an important quality characteristic that directly impacts the consumer appeal of snack foods. Color parameters, L*, a*, and b*, for MLP-oat flour extrudates are shown in . L* values ranged from 47.1 to 91.4, a* values ranged from −5.0 to 0.0, and b* values ranged from -5.3 to 5.8. In the absence of MLP, an increase of in-barrel moisture content led to a decrease in lightness (L*) value of extrudates by up to 34.1%. This was due to lower expansion and porosity of extrudates processed at higher in-barrel moistures. In-barrel moisture had a less pronounced effect on lightness in extrudates having MLP. In general, an addition of 15–45% MLP led to a decrease in lightness and yellowness (low or negative b* values), and an increase in greenness (negative a* values). This was expected as MLP has a greenish-brown color.
Table 3 CIELAB color values of MLP-oat flour extrudates
Sensory Analysis
Results from the consumer acceptance tests are shown in . In general, high-fiber extruded products have low expansion, often accompanied by hard texture and rough and uneven “shark skin.”[Citation17] Such features make high fiber products unappealing to consumers. However, it was interesting to note that for the MLP-oat flour extrudates in this study, 10 out of the 12 treatments had an overall acceptability score of above 6 (‘like slightly’ or better). This was as least partly due to the potato flour/soybean oil coating applied to the products prior to their sensory evaluation. Due to the same reason, the color scores (6.4–7.1) were reasonably good and did not show any significant differences between treatments although instrumental color measurements of uncoated products indicated a substantial negative impact (decreased lightness and yellowness, and increased greenness) of MLP levels. The coating also seemed to have positively impacted flavor scores (5.6–7.4), which were reasonably good and did not show any significant differences between treatments although it was expected that MLP would lead to substantially poorer flavor scores. As expected, the coating had no influence on the texture scores that ranged from 3.4 to 7.6 and followed an inverse trend with respect to crispness work. For example, texture scores improved as the MLP level increased in the case of extrudates processed at 22 and 26% in-barrel moisture and this corresponded with lower values of crispness work. The control formulation (0% MLP) processed at 22 and 26% in-barrel moistures had the lowest overall acceptability scores of 4.1 and 4.4. These two treatments also had the lowest texture scores of 4.1 and 3.4, respectively. The three treatments with the best overall acceptability scores (6.9–7.5) were 30 and 45% MLP at 26% in-barrel moisture and the control at 19% in-barrel moisture. These three treatments also had the best texture scores (7.0–7.3). Thus, it was evident that texture had a big role in impacting the overall acceptability scores. The scores for oil (4.4–6.2) were the lowest among all attributes, and this indicated the need for a solution to the poor color and flavor of MLP-based snacks that involves less or no oil.
Table 4 Consumer acceptance scores of coated MLP-oat flour extrudates
CONCLUSION
This study demonstrated the utility of extrusion processing in incorporating high levels of leafy vegetable ingredients in expanded snacks. The extruded products, based on Moringa (Moringa oleifera) leaf powder and oat flour, had substantially improved macro and micronutrient profiles as compared to commercially available snacks. These MLP-oat flour snacks had at least 20 and 90% more fiber and protein, respectively, and also lower post-coating fat and higher vitamin A, iron, and calcium than a typical puffed commercial product. MLP-oat flour snacks, coated with a vegetable oil and potato flour blend, were also found to be appealing to consumers. Both formulation (MLP levels 0, 15, 30, and 45%) and processing (in-barrel moisture levels of 19, 22, and 26%) had an impact on expansion and mechanical properties of the product. A relatively new analytical tool, phase transition analyzer, was used to study the flow properties of raw material formulations. The PTA and pasting data, in combination with various extrudate measurements, were very useful in the development of strong hypotheses regarding the roles of process moisture and non-starch components in modifying viscosity, water binding, gas holding capacity, and cell wall strength, and their overall impact on the processing, expansion, and mechanical properties of vegetable-based expanded snacks produced by extrusion.
ACKNOWLEDGMENTS
The authors thank Eric Maichel, operations manager of the KSU extrusion lab, for conducting all processing runs, Tree for Life (Wichita, KS, USA) for providing Moringa leaf powder, and Wegner Manufacturing, Inc. (Sabetha, KS) for donation of the PTA equipment. This is contribution number 09–172-J from the Kansas Agricultural Experiment Station, Manhattan, Kansas. The paper is partly supported by Eastern China University of Science and Technology (grant number YF0142122).
REFERENCES
- Lockett , C.T. , Calvert , C.C. and Grivett , L.E. 2000 . Energy and micronutrient composition of dietary and medical wild plants consumed during drought. Study of rural Fulani, Northeastern Nigeria . International Journal of Food Science and Nutrition , 51 : 195 – 208 .
- Makkar , H.P.S. and Becker , K. 1997a . Nutrient and antiquality factors I different morphological parts of Moringa oleifera tree . Journal of Agricultural Science (Cambridge) , 128 : 311 – 322 .
- Chawla , S. , Saxena , A. and Seshadri , S. 1988 . In vitro availability of iron in various green leafy vegetables . Journal of Science and Food Agriculture , 46 : 125 – 127 .
- Makkar , H.P.S. and Becker , K. 1997b . Nutrient value and antinutritional components of whole and ethanol extracted Moringa leaves . Animal Feed Science and Technology , 63 : 211 – 228 .
- Fuglie, L.J. 2005. The Moringa tree. A local solution to malnutrition http://www.moringanews.org/documents/Nutrition.pdf (http://www.moringanews.org/documents/Nutrition.pdf) (Accessed: 2 July 2009 ).
- Brower, A. The U.S. market for salted snacks. Packaged facts. Report ID: LA728892. 2002 http://www.packagedfacts.com/Salted-Snacks-728892/ (http://www.packagedfacts.com/Salted-Snacks-728892/) (Accessed: 24 July 2009 ).
- Colonna , P. , Tayeb , J. and Mercier , C. 1989 . “ Extrusion cooking of starch and starchy products ” . In Extrusion Cooking , Edited by: Mercier , C. , Linko , P. and Harper , J.M. 247 – 319 . St. Paul, MN : American Association of Cereal Chemists .
- Trater , A.M. , Alavi , S. and Rizvi , S.S.H. 2005 . Use of non-invasive X-ray microtomography for characterizing microstructure of extruded biopolymer foams . Food Research International , 38 : 709 – 719 .
- Altan , A. , McCarthy , K.L. and Maskan , M. 2009 . Effect of extrusion cooking on functional properties and in vitro starch digestibility of barley-based extrudates from fruit and vegetable by-products . Journal of Food Science , 74 ( 2 ) : E77 – E86 .
- Altan , A. , McCarthy , K.L. and Maskan , M. 2008a . Twin screw extrusion of barley-grape pomace blends: Extrudate characteristics and determination of optimum processing conditions . Journal of Food Engineering , 89 : 24 – 32 .
- Altan , A. , McCarthy , K.L. and Maskan , M. 2008b . Evaluation of snack foods from barley-tomato pomace blends by extrusion processing . Journal of Food Engineering , 84 : 231 – 242 .
- Camire , M.E. , Dougherty , M.P. and Briggs , J.L. 2007 . Functionality of fruit powders in extruded corn breakfast cereals . Food Chemistry , 101 : 765 – 770 .
- Yagci , S. and Gogus , F. 2008 . Response surface methodology for evaluation of physical and functional properties of extruded snack foods developed from food by-products . Journal of Food Engineering , 86 : 122 – 132 .
- Stojceska , V. , Ainsworth , P. , Plunkett , A. , Ibanoglu , E. and Ibanoglu , S. 2008 . Cauliflower by-products as a new source of dietary fiber, antioxidants and proteins in cereal based ready-to-eat expanded snacks . Journal of Food Engineering , 87 : 554 – 563 .
- Mitchell , J.R. and Areas , J.A.G. 1992 . “ Structural changes in biopolymers during extrusion ” . In Food Extrusion Science and Technology , Edited by: Kokini , J.C. , Ho , C.T. and Karwe , M.V. 631 – 652 . New York : Marcel Dekker .
- Cheftel , J.C. 1989 . “ Extrusion cooking and food safety ” . In Extrusion Cooking , Edited by: Mercier , C. , Linko , P. and Harper , J.M. 435 – 461 . St. Paul, MN : American Association of Cereal Chemists .
- Lue , S. , Hsieh , F. and Huff , H.E. 1991 . Extrusion cooking of corn meal and sugar beet fiber: Effects on expansion properties, starch gelatinization, and dietary fiber content . Cereal Chemistry , 68 : 227 – 234 .
- American Association of Cereal Chemists (AACC) . 2000 . Approved Methods of the AACC , 10th , St. Paul, MN : American Association of Cereal Chemists .
- Agbisit , R. , Alavi , S. , Cheng , E. , Herald , T.J. and Trater , A.M. 2007 . Relationships between microstructure and mechanical properties of cellular corn starch extrudates . Journal of Texture Studies , 38 : 199 – 219 .
- Agbisit , R.N.M. 2007 . Relationships between material properties and microstructure-mechanical attributes of extruded biopolymeric foams , Manhattan, KS : Ph.D. Thesis. Kansas State University .
- Bengoechea , C. , Arrachid , A. , Guerrero , A. , Hill , S.E. and Mitchell , J.R. 2007 . Relationship between the glass transition temperature and the melt flow behavior for gluten, casein and soya . Journal of Cereal Science , 45 : 275 – 284 .
- Strahm , B. 1998 . Fundamentals of polymer science as an applied extrusion tool . Cereal Foods World , 43 : 621 – 625 .
- Strahm , B. , Plattner , B. , Huber , G. and Rokey , G. 2000 . Application of food polymer science and capillary rheometry in evaluating complex extruded products . Cereal Foods World , 45 ( 7 ) : 300 – 303 .
- Strahm , B. and Plattner , B. 2000 . Thermal profiling—Predicting processing characteristics of feed materials . Feed International , 21 ( 4 ) : 26 – 29 .
- Slade , L. and Levine , H. 1995 . Glass transitions and water-food structure interactions . Advances in Food and Nutrition Research , 38 : 103 – 164 .
- Anese , M. and Gormley , R. 1996 . Effects of dairy ingredients on some chemical, physico-chemical and functional properties of minced fish during freezing and frozen storage . Lebensmittel-Wissenschaft und-Technologie , 29 ( 1–2 ) : 151 – 157 .
- Amarteifio , J.O. , Munthali , D.C. , Karikari , S.K. and Morake , T.K. 2002 . The composition of pigeon peas (Cajanus cajan L. Millsp.) grown in Botswana . Plant Foods for Human Nutrition , 57 ( 2 ) : 173 – 177 .
- Kokini , J.L. , Chang , C.N. and Lai , L.S. 1992 . “ The role of rheological properties on extrudates expansion ” . In Food Extrusion Science and Technology , Edited by: Kokini , J.C. , Ho , C.T. and Karwe , M.V. 631 – 652 . New York : Marcel Dekker .
- Cheng , E. , Alavi , S. , Pearson , T. and Agbisit , R. 2007 . Mechanical-acoustic and sensory evaluations of corn starch-whey protein isolate extrudates . Journal of Texture Studies , 38 : 473 – 498 .
- Gibson , L.G. and Ashby , M.F. 1997 . Cellular Solids: Structure and Properties , Cambridge, , U.K : Cambridge University Press .
- Parker , R. , Ollett , A.-L. , Lai-Fook , R. and Smith , A.C. 1989 . “ The rheology of food melts and its applications in extrusion processing ” . In Rheology of Food, Biological and Pharmaceutical Materials; Carter, R.E , London : Elsevier Science .
- Whalen , P.J. 1998 . Detection of differences in corn quality for extrusion processes by rapid visco analyzer . Cereal Foods World , 43 : 69 – 74 .
- Whalen , P.J. , Bason , M.L. , Booth , R.I. , Walker , C.E. and Williams , P.J. 1997 . Measurement of extrusion effects by viscosity profile using the rapid viscoanalyser . Cereal Foods World , 42 ( 6 ) : 469 – 477 .
- Gajula , H , Liu , S. , Alavi , S. , Herald , T. , Madl , R. , Bean , S.R. and Tilley , M. 2009 . Pre-cooked fiber-enriched wheat flour obtained by extrusion: rheological and functional properties . International Journal of Food Properties , 12 ( 1 ) : 27 – 44 .
- Guha , M. and Ali , S.Z. 2002 . Molecular degradation of starch during extrusion cooking of rice . International Journal of Food Properties , 5 ( 3 ) : 509 – 521 .
- Burkitt , D. 1969 . Related disease—Releated cause? . Lancet II , ii : 1229 – 1231 .