Abstract
The relative humidity at which crystalline materials begin adsorbing large quantities of water from the atmosphere, termed the deliquescence point (RHo), is an important temperature dependent stability parameter. The RHo of a number of food and pharmaceutical materials has been determined at 25°C, but it is difficult to find RHo values in the literature at other temperatures, even for common food materials, such as sucrose. Thus, the primary objective of this research was to assess the effect of temperature on RHo for crystalline sucrose using a new automatic isotherm generator. A secondary objective was to determine and compare the RHo value using three other methods (RH after the MC step change method, and two smoothing and second derivative methods [Savitzky-Golay and Yuan and others]), in addition to the commonly used linear extrapolation method. The AquaSorp DDI method provides an accurate, fast, and convenient means of measuring the initial real-time aw values of the adsorption of water by the crystalline solid, making the DDI method an outstanding RHo measuring method. A decrease in RHo with increasing temperature was observed and attributed to increasing sucrose solubility with increasing temperature. No statistical difference (p = 0.05) was found between the four RHo determination methods at each temperature, with the linear extrapolation method yielding RHo values of 85.9, 85.7, and 84.0% at 15, 25, and 35°C, respectively.
INTRODUCTION
Sucrose is widely used in the food industry because of the sweet taste and/or functional properties it contributes to food systems. Sucrose is one of the most common ingredients in foods and is important to the structure of many foods. For example, sucrose can either recrystallize or form an amorphous glass after baking, which affects the texture of baked products, such as cookies and biscuits.
Moisture sorption isotherms are useful for designing various food processes, such as dehydration,[Citation1] and can be used to predict changes in food storage stability. Isotherms map the relationship between moisture content and water activity (aw ). This moisture content-aw relationship is complex and unique for each material due to the various interactions that can occur between the water and solid components at different moisture contents. In the case of crystalline sucrose, the isotherm exhibits a Type III or J-shaped isotherm. At a critical aw or %RH value, the sucrose crystals begin to adsorb water from the atmosphere and dissolve. This critical %RH value is called the deliquescence point and is commonly termed the critical relative humidity (RHo ) or the deliquescence relative humidity (DRH). RHo is unique to each crystalline solid and is a function of temperature, where the temperature dependence follows the Clausius-Claperyron equation.[Citation2]
Deliquescence is a first-order phase transition from solid to solution, induced by water uptake from the atmosphere and is depicted schematically in . , as well as the following description of the deliquescence process, is based on: Van Campen and others,[Citation3] Zografi and Hancock,[Citation4] Kontny and Zografi,[Citation5] Hancock and Shambin,[Citation6] Airaksinen,[Citation7] Salameh and others,[Citation8] Hiatt and others,[Citation9] Newman and others,[Citation10] Espinosa and others,[Citation11] and Spackman and Schmidt.[Citation12] At environmental relative humidity values (RHE ) below the deliquescence point, water from the atmosphere is adsorbed at the crystalline solid surface, first forming a water monolayer, then at higher RHE values forming multilayers (). Upon increasing the RHE to the critical relative humidity (RHo ), the deliquescence process is initiated (), that is, the solid starts to dissolve in the condensate film on the surface of the solid, forming, over time, a saturated solution (), where RHo is equal to the saturated solution aw × 100. The %RH at which deliquescence occurs (RHo ) is a unique property of the crystalline solid and its storage temperature. If RHE is increased further (), the adsorption process continues until, over time, the vapor pressure of the solution becomes equal to the vapor pressure of the atmosphere (RHE ), yielding a dilute (or undersaturated) solution, where the aw × 100 is equal to RHE .
Figure 1 Schematic diagram of the interaction of a crystalline solid, such as sucrose, with water vapor in the atmosphere at various relative humility values, where RHE = environmental relative humidity and RHo = critical relative humidity. Stages A through D are explained in the text (color figure available online).
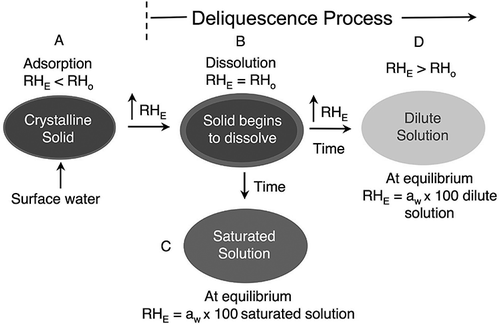
From a thermodynamic point of view, at each relative humidity, the crystalline sucrose can exist either as a solid or as an aqueous solution.[Citation2] As depicted in , for %RH values lower than RHo , the Gibbs free energy of the solid state is lower than the corresponding solution state. Therefore, the crystalline sucrose remains in the solid state. As the atmospheric %RH increases, the Gibbs free energy of the corresponding solution state decreases until it is equal to the Gibbs free energy of the solid state, which corresponds to the deliquescence point.
Figure 2 Gibbs free energy of a solid and its aqueous solution as a function of %RH. The %RH at which the solid and the solution Gibbs free energies become equal is the deliquescence point (DRH or RHo ) (excerpted from reference 2).
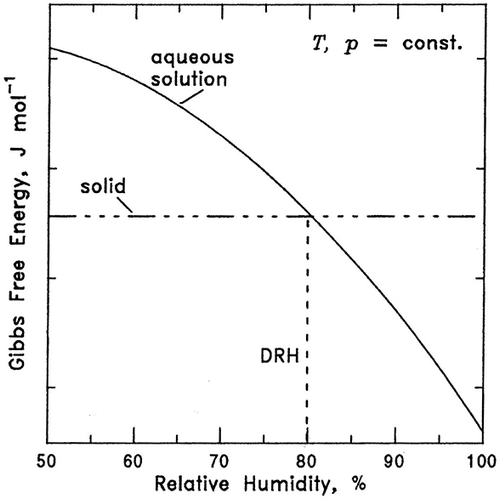
Many common food and pharmaceutical materials are deliquescent. They are usually crystalline solids that are highly water soluble and can interact with water vapor from the atmosphere. Crystalline sugars, low-molecular-weight organic acids, inorganic salts, and vitamins, for example, thiamine HCl and sodium ascorbate, are deliquescent materials.[Citation8] Salameh and Taylor[Citation13] and Salameh and others[Citation8] reported that the RHo of a material is usually equal to the aw of the corresponding saturated solution. Salameh and others[Citation8] also reported that mixtures of deliquescent materials exhibited deliquescence lowering; that is, where the RHo of two deliquescent materials is lower than the individual values of either deliquescent material. For example, a 50:50 mixture of crystalline sucrose, which has a RHo of 85% at 25°C, and α-glucose monohydrate, which has a RHo of 91% at 25°C, exhibited a lowered RHo of 79% at 25°C.[Citation8] Hiatt and others[Citation9] indicated that the deliquescence phenomenon could increase the reactant mobility and chemical instability. Therefore, deliquescence may affect the shelf life, quality, functionality and packaging material prediction of food products that contain two or more deliquescent ingredients.
Knowledge of the RHo of crystalline sucrose, a very a common deliquescent food material, as a function of temperature is very important for food product stability.[Citation14,Citation15] RHo is often determined from a water sorption isotherm using the linear extrapolation method described by Salameh and Taylor,[Citation13] where the %RHo is determined by extrapolating the linear portions of the isotherm before and after the deliquescence event. Three additional methods of determining RHo are explored in this article, a simple step change in moisture content method and two more calculation-intensive smoothing and second derivative methods.
The water sorption isotherm is traditionally generated by equilibrating the material of interest to select %RH (or aw ) values over several saturated salt solutions.[Citation16] Recently, automated water sorption instruments have been developed to overcome some of the limitations of the traditional saturated salt solution method.[Citation17] Commercially available automatic water sorption instruments include, for example, the Dynamic Vapor Sorption instrument from Surface Measurement Systems Ltd., the IGAsorp from Hiden Analytical, the Cisorp Water Sorption Analyzer from C. I. Electronics Ltd., the Q5000SA and VTI Sorption Analyzer from TA Instruments, the AquaSorp from Decagon Devices Inc., the SPS–Moisture Sorption Analysis instrument from Projekt Messtechnik, and the Hydrosorb 1000 from Quantachrome.
The AquaSorp Isotherm Generator, employed in this study, utilizes the Dynamic Dewpoint Isotherm (DDI) method, which is unique among automatic isotherm generators. The DDI method directly measures aw using a patented chilled-mirror dewpoint sensor, while it gravimetrically tracks weight change using a magnetic force balance. Thus, the sample is not equilibrated to a known (or selected) aw value, but rather the aw of the sample is measured throughout the real-time sorption process. Adsorption occurs as saturated wet air is passed over the sample, while desorption is accomplished as desiccated air is passed over the sample; both at a user selected flow rate. After roughly a 0.015 change in aw , airflow is stopped and a snapshot of the sorption process is taken by directly measuring aw and weight. The DDI method makes it possible to generate complete isotherms with 50 to 200 data points quickly (a few days) and accurately without long equilibration times. However, as pointed out by the manufacturer, in some cases (e.g., materials with slow diffusion rates) the DDI method may not yield an isotherm comparable to the traditional equilibrium-based isotherm.[Citation17,Citation18]
Moisture sorption isotherms, and thus the deliquescence point, vary with temperature.[Citation2,Citation19,Citation20] In general, the deliquescence point of sugars and salts decreases as temperature increases, due to increasing solubility of these materials with increasing temperature. This solubility temperature dependence can be observed in for sucrose, where the solubility of sucrose increases from 1.9443 g sucrose/g water at 15°C to 2.2435 g sucrose/g water at 35°C. Isotherms of crystalline sucrose are available in the literature, but most isotherms were obtained near room temperature, 20 or 25°C.[Citation21–23 Citation Citation23 Audu and others[Citation24] studied sucrose isotherms at 80°C using a dynamic method (details of the nature of the dynamic method, however, were not specified). Thus, the main objective of this study was to determine the deliquescence point of crystalline sucrose as a function of temperature, 15, 25, and 35°C, using the new isotherm AquaSorp isotherm generator. The secondary objective of this study was to determine and compare the RHo value using three other methods (RH after the MC step change method, and two smoothing and second derivative methods [Savitzky-Golay and Yuan and others]), in addition to the commonly used linear extrapolation method.
Table 1 Saturation solubility data for sucrose in water in both g sucrose per g water and %MC (dry basis) units as a function of temperature from Bubník and Kadlec.[Citation28]
MATERIALS AND METHODS
Materials
Crystalline sucrose, ACS reagent grade (Sigma-Aldrich Co., St. Louis, MO) was used “as is.” The particle size was measured using USA standard testing sieves (W.S. Tyler, Mentor, OH, USA). All sucrose particles were greater than 300 μm and 98.2% were greater than 425 μm. The moisture content of the “as is” crystalline sucrose, determined using Karl Fisher titration, was 0.004% on a wet basis. Deionized water was used to prepare the saturated sucrose solutions.
Methods
Dynamic dewpoint isotherms
Crystalline sucrose dynamic dewpoint isotherms (DDI) were generated using the AquaSorp isotherm generator (Decagon Devices, Inc., Pullman, WA, USA) at 15, 25, and 35°C. Approximately 600 mg of crystalline sucrose was loaded into a sample cup and placed on the precision balance. In general, a minimum amount of sample, just enough to cover the bottom of sample cup, was used to decrease the experimental time and, as will be discussed in the Results and Discussion section, to minimize the diffusion-associated increases in aw after the deliquescence point. However, for one run at 15°C, 2300 mg of crystalline sucrose was used, but exhibited no difference in the deliquescence point obtained. During the experiment, the sample was exposed to increasing %RH values via exposure to water saturated air at a user selected 300 mL/min flow rate. The ending %RH was approximately 90% (or 0.90 aw ). During the experiment, aw and weight data were collected. After the isotherm was complete, the weight data were converted to moisture content (dry basis) using the initial moisture content of the crystalline sucrose.
RHo determination
RHo is commonly determined by calculating the intersection of the extrapolated linear portions of the isotherm before and after the deliquescence event (termed the linear extrapolation method). The criteria used to distinguish the data to be used in the extrapolation method were as follows. The first large gap in the moisture content data, calculated as the difference between two sequential moisture content points, was used to separate the isotherm into two sections: before and after the deliquescence event. A change in aw greater than ±0.005 (the reported AquaSorp accuracy) was used to determine which isotherm data points to exclude in the extrapolation for the linear portion after the deliquescence event. A low R 2 is often associated with the linear portion after the deliquescence event due to the nearly vertical increase in moisture content with nearly no change in aw ; however, the low value of R 2 did not affect the use of the linear equation for determining RHo .
Three additional methods were used to determine RHo : (1) the first RH value after the beginning of the deliquescence event, determined by the first large increase in the moisture content data, calculated as the difference between two sequential moisture content points (termed the RH after the MC step change method) was identified as the RHo value; (2) an Excel spreadsheet algorithm based on the Savitzky-Golay smoothing and differentiation method[Citation25] (assumes equally spaced aw data) developed by Decagon Devices Inc. (Pullman, WA, USA) (termed the Savitzky-Golay method); and (3) the relative humidity value where the moisture content changed most dramatically, determined by smoothing the isotherm data and taking the second derivative of the fitted isotherm curve using the MATLAB program (The MathWorks, Inc., Natick, MA, USA). The procedures used to obtain the second derivative were as follows. The water activity (aw ) and moisture content (% db) data were smoothed using loess (local polynomial regression fitting) smoothing (second degree polynomial) with a span of 11 points. Next, the smoothed data set was fit using a smoothing cubic spline, after which the first derivative of the fit was taken. Using the data from the first derivative as the new data set, the above smoothing and fitting procedures were repeated, after which the first derivative of the fit was again taken, evaluated at every 0.001 unit (to optimize peak location), resulting in the second derivative. The maximum of the second derivative curve, in the targeted region, is the critical relative humidity (RHo ) value. The procedure was developed by Yuan and others[Citation26] (termed herein the Yuan and others method). The major difference between the Savitzky and Golay and Yuan and others methods is that the Yuan and others method does not assume equally spaced aw data. A detailed discussion comparing the Savitzky-Golay and the Yuan and others methods is given in Yuan.[Citation27]
Saturated Sucrose Solution Preparation
Saturated sucrose solutions were prepared by adding sucrose to deionized water. The beaker was covered with parafilm. To ensure that the sucrose solutions would be saturated, 90 g of sucrose was added to 40 g of water at room temperature and stirred using a magnet stir bar on a stir plate for 24 h to ensure complete dissolution of all the sucrose that could dissolve. Excess sucrose crystals remained at the bottom of the beaker. The initial amount of sucrose used was based on the sucrose solubility data of Bubník and Kadlec[Citation28] at 35°C (). Aliquots of 10 mL were then taken and transferred into AquaLab sample cups and placed overnight (16 h) on a temperature control plate (Decagon Devices Inc., Pullman, WA, USA) set at the desired temperature, 15, 25, or 35°C. The sample cups were covered with lids. The sample was stirred with a glass stir rod several times during the temperature control period. The aw of the solutions was then measured three times using an Aqua Lab Series 4TE meter (Decagon Devices Inc., Pullman WA, USA). The entire sample preparation procedure was repeated three times at each temperature.
Water Activity Measurements
Saturated sucrose solution was prepared in triplicate and the aw value was measured in triplicate (for a total of nine aw measurements per temperature) at 15, 25, and 35°C using an Aqua Lab Series 4TE aw meter (Decagon Devices, Inc., Pullman, WA, USA). To ensure complete temperature and vapor equilibration, the aw values were taken after 1 h of continuous aw measurements.
Statistical Analysis
A one-way between-group analysis of variance was conducted to determine whether there was a significant difference among the RHo values obtained by the four methods and the saturated solution aw × 100 at each temperature. A least significant difference test was used to determine the location of the difference. The level of significance was set at p = 0.05.
RESULTS AND DISCUSSION
DDIs for crystalline sucrose at 15, 25, and 35°C, as well as the measured saturated sucrose aw values, are shown in . Since each DDI run is unique, average isotherm values cannot be calculated; rather, duplicate runs were plotted for each temperature so variation in the isotherms could be visually assessed. As expected, crystalline sucrose, at all three temperatures, exhibited typical type III isotherm behavior—little moisture adsorption at low aw values followed by a dramatic rise in moisture content after the deliquescence point, RHo . At RHo , the solid particle spontaneously adsorbs water from the atmosphere, producing a saturated solution.[Citation2] Further increase in the atmospheric %RH leads to additional water adsorption by the sucrose solution to maintain thermodynamic equilibrium. If the atmospheric %RH decreases, evaporation of water will occur. However, it is interesting to note that the solution typically does not recrystallize at the RHo , but remains supersaturated until a much lower %RH, at which recrystallization occurs.[Citation2]
Figure 3 Crystalline sucrose DDIs obtained using a 300 mL/min flow rate and saturated sucrose solution values (moisture content values from and aw values from this study []) at 15, 25, and 35°C.
![Figure 3 Crystalline sucrose DDIs obtained using a 300 mL/min flow rate and saturated sucrose solution values (moisture content values from Table 1 and aw values from this study [Table 2]) at 15, 25, and 35°C.](/cms/asset/61f5d77d-4e07-4f40-9f7a-6ec9f1ec2f84/ljfp_a_447814_o_f0003g.gif)
Theoretically, the aw of the saturated solutions should remain constant until all of the sucrose completely dissolves. However, as shown in , aw values after the deliquescence event do not remain constant until all of the sucrose completely dissolves, but rather begin to slowly increase. This increase in aw values before achieving the saturated solubility aw value is attributed to slow diffusion of the adsorbing water and dissolving sucrose, setting up an aw gradient from the atmosphere-solution interface (slightly higher aw ) to the dissolving crystalline sucrose interface (saturated solution aw ). It takes time for the water and dissolving sucrose to completely mix. However, before complete mixing can be achieved, the next set of weight change and aw measurements are being taken by the AquaSorp instrument. In order to minimize the effects of the diffusion process, a minimum amount of sample should be used and a reasonably slow AquaSorp flow rate selected. This slow diffusion problem explains why some of the aw data after the deliquescence point and before the saturated solubility point could not be included in the linear extrapolation calculation. It is important to point out that the increase in aw values before achieving the saturated solubility aw value observed in , does not interfere with obtaining accurate RHo values. Rather, being able to measure the initial real-time aw values (the real-time response to water adsorption by the crystalline solid), instead of equilibrium aw values, makes the DDI method an outstanding RHo measuring method.
RHo values obtained via four different methods at 15, 25, and 35°C are given in . In addition, aw and RHo values found in the literature are included in for comparison purposes. There was no statistical difference between the four methods of obtaining the RHo values at any of the three temperatures (). This result indicates that all four methods can be used to obtain the RHo values from the DDI method. The Linear Extrapolation and the RH after the MC Step Change are the simplest methods and, based on the results reported herein, generate the same RHo values as the more calculation intensive second derivative methods (Savitzky-Golay and Yuan and others).
Table 2 Saturated sucrose solution a w and deliquescence point (RH o ) values of crystalline sucrose at 15, 25, and 35°C
The statistical results in also show the comparison between the three RHo values and the saturated solution aw values at each temperature. At 15 and 25°C, there was no statistical difference between the RHo values and the saturated solution aw × 100 values; however, at 35°C a statistical difference was observed between the three RHo values and the saturated solution aw . Theoretically, at a given temperature, the saturated solution aw × 100 value should be equal to the RHo value.[Citation2,Citation3,Citation31] Thus, the statistical difference observed for 35°C may be attributed to the very small amount of variation obtained for the RHo values at that temperature. It should be pointed out that even though there is a statistical difference between the RHo values and the saturated solution aw at 35°C, there is no practical difference.
As predicted by theory and exhibited by the experimental values in , for sucrose both the RHo values and the saturated solution aw values decreased with increasing temperature.[Citation2,Citation32,Citation33] This result can be attributed to the increasing solubility of sucrose at higher temperatures (). The decrease in the deliquescence point with temperature for sucrose can be calculated using the following equation derived from the Clausius-Clapeyron equation as shown in Seinfeld and Pandis[Citation2]:
Table 3 Calculated RHo values at 15 and 35°C using EquationEq. (2) compared to extrapolated RHo values (). The solubility of sucrose in mol/mol (n) was calculated using the solubility data in
CONCLUSIONS
The deliquescence point (RHo ) of crystalline sucrose was determined using a new automatic isotherm generator at 15, 25, and 35°C. The DDI method provides an accurate, fast, and convenient means of measuring the initial real-time aw values of the adsorption of water by the crystalline solid, making the DDI method an outstanding RHo measuring method. Four different methods, Linear Extrapolation, RH after the MC Step Change, and two smoothing and second derivative methods [Savitzky-Golay and Yuan and others methods]), were used to obtain the RHo values from the isotherms. The RHo values from the four methods were found to be statically equivalent at p = 0.05. As predicted from theory, RHo decreased as the temperature increased.
ACKNOWLEDGMENTS
The authors would like to acknowledge the support of Kraft Foods for their contribution to the joint Undergraduate and Masters Program between the University of Illinois, Urbana-Champaign, IL and China Agricultural University, Beijing, China. The use of the AquaSorp instrument from Decagon Devices Inc. (Pullman, WA) is also gratefully acknowledged.
Notes
REFERENCES
- Barbosa-Cánovas , G.V. , Juliano , P. , Barbosa-Cánovas , G.V. , Fontana , A.J. and Schmidt , S.J. 2007 . “ Desorption phenomena in food dehydration processes ” . In Water Activity in Foods: Fundamentals and Applications , Edited by: Labuza , T.P . 435 Ames, IA : Blackwell Publishing .
- Seinfeld , J.H. and Pandis , S.N. 1998 . Atmospheric Chemistry and Physics , 507 – 508 . New York : John Wiley & Sons, Inc .
- Van Campen , L. , Amidon , G.L. and Zografi , G. 1983a . Moisture sorption kinetics for water-soluble substances . I: Theoretical considerations of heat transport control. Journal of Pharmaceutical Sciences , 72 ( 12 ) : 1381 – 1388 .
- Zografi , G , Hancock , BC. and Crommelin , D.J.A. 1994 . “ Water-solid interactions in pharmaceutical system ” . In Pharmaceutical Sciences , Edited by: Midha , K.K. and Nagai , T. 405 – 419 . Stuttgart : Medpharm Scientific Publishers .
- Kontny , M.J. and Zografi , G. 1995 . “ Sorption of water by solids ” . In Physical Characterization of Pharmaceutical Solids , Edited by: Brittain , H.G . 387 – 418 . New York : Marcel Dekker, Inc .
- Hancock , B.C. and Shambin , S.L. 1998 . Water vapour sorption by pharmaceutical sugars . Pharmaceutical Science and Technology Today , 1 ( 8 ) : 345 – 351 .
- Airaksinen , S. 2005 . Role of water in the physical stability of solid pharmaceutical formulations. Ph.D , Helsinki, , Finland : Thesis, University of Helsinki .
- Salameh , A.K. , Mauer , L.J. and Taylor , L.S. 2006 . Deliquescence lowering in food ingredient mixtures . Journal of Food Science , 71 ( 2 ) : E10 – E16 .
- Hiatt , A.N. , Ferruzzi , M.G. , Taylor , L.S. and Mauer , L.J. 2008 . Impact of deliquescence on the chemical stability of vitamins B1, B6, and C in powder blends . Journal of Agricultural and Food Chemistry , 56 : 6471 – 6479 .
- Newman , A.W. , Reutzek-Edens , S.M. and Zografi , G. 2008 . Characterization of hydroscopic properties of active pharmaceutical ingredients . Journal of Pharmaceutical. Sciences , 97 ( 3 ) : 1047 – 1059 .
- Espinosa , R.M. , Franke , L. and Deckelmann , G. 2008 . Phase changes of salts in porous materials: Crystallization, hydration and deliquescence . Construction and Building Materials , 22 : 1758 – 1773 .
- Spackman , C.C.W. and Schmidt , S.J. 2010 . Characterization of sugar gum paste by water sorption isotherm behavior and texture analysis . Food Chemistry , 119 ( 2 ) : 490 – 499 .
- Salameh , A.K. and Taylor , L.S. 2005 . Deliquescence in binary mixtures . Pharmaceutical Research , 22 ( 2 ) : 318 – 324 .
- Mathlouthi , M. and Rogé , B. 2003 . Water vapor sorption isotherms and the caking of food powders . Food Chemistry , 82 : 61 – 71 .
- Rogé , B. and Mathlouthi , M. 2003 . Caking of white crystalline sugar . International Sugar Journal , 105 ( 1251 ) : 128 – 136 .
- Saravacos , G.D. , Tsiourvas , D.A. and Tsami , E. 1986 . Effect of temperature on the water adsorption isotherms of sultana raisins . Journal of Food Science , 51 ( 2 ) : 381 – 383 .
- Schmidt , S.J. and Lee , J.W. 2009 . “ Comparison of water vapor sorption isotherms obtained using the new Dynamic Dewpoint isotherm method to the standard saturated salt slurry method ” . In Food Chemistry , submitted .
- Carter , B. and Fontana , A. 2008 . Dynamic dewpoint isotherm verses other moisture sorption isotherm methods , Pullman, WA : Application Note. Decagon Devices .
- Kapsalis , J.G. 1987 . “ Influence of hysteresis and temperature on moisture sorption isotherms ” . In Water Activity: Theory and Application to Foods , Edited by: Rockland , L.B. and Beuchat , L.R. 173 – 213 . New York : Marcel Dekker, Inc .
- Schmidt , S.J. 2004 . “ Water and solids mobility in foods ” . In Advances in Food and Nutrition Research , Edited by: Taylor , S. Vol. 48 , 1 – 101 . London : Academic Press .
- Lang , K.W. and Steinberg , M.P. 1981 . Linearization of the water sorption isotherm for homogeneous ingredients over aw 0 . 30–0.95. Journal of Food Science , 46 : 1450 – 1452 .
- Kou , Y. , Molitor , P. and Schmidt , S.J. 1999 . Mobility and stability of model food systems using NMR, DSC, and conidia germination techniques . Journal of Food Science , 64 ( 6 ) : 950 – 959 .
- Kappes , S.M. 2001 . Dynamic vapor sorption, differential scanning calorimetry and textural analysis of freeze-dried sucrose , Urbana, IL : Ph.D. Thesis, University of Illinois at Urbana-Champaign .
- Audu , T.O.K. , Loncin , M. and Weisser , H. 1978 . Sorption isotherm of sugars . Lebensmittel-Wissenschaft Und-Technologie , 11 : 31 – 34 .
- Savitzky , A. and Golay , MJE. 1964 . Smoothing and differentiation of data by simplified least squares procedures . Analytical Chemistry , 36 ( 8 ) : 1627 – 1639 .
- Yuan , X. , Schmidt , A.R. and Schmidt , S.J. June 2009 . Investigating the use of automatic water vapor sorption instruments for the determination of the critical relative humidity at which the glassy to rubbery transition occurs in amorphous food materials , Anaheim, CA : 70th Annual Institute of Food Technologists .
- Yuan , X. 2009 . “ Investigation of the relationship between the critical relative humidity and the glassy to rubbery transition in polydextrose ” . In Masters Thesis , Urbana, IL : University of Illinois at Urbana-Champaign .
- Bubník , Z. and Kadlec , P. 1995 . “ Sucrose solubility ” . In Sucrose Properties and Applications , Edited by: Mathlouthi , M. and Reiser , P. 107 Blackie Academic and Professional, an imprint of Chapman Hall, Glasgow .
- Van Campen , L. , Amidon , G.L. and Zografi , G. 1983b . Moisture sorption kinetics for water-soluble substances . II: Experimental verification of heat transport control. Journal of Pharmaceutical Sciences , 72 ( 12 ) : 1388 – 1393 .
- Rüegg , M. and Blanc , B. 1981 . The water activity of honey and related sugar solutions . Lebensmittel-Wissenschaft Und-Technologie , 14 : 1 – 6 .
- Kontny , M.J. and Zografi , G. 1985 . Moisture sorption kinetics for water soluble substances . Part IV. Studies with mixtures of solids. Journal of Pharmaceutical. Sciences , 74 ( 2 ) : 124 – 127 .
- Wexler , A.S. and Seinfeld , J.H. 1991 . Second-generation inorganic aerosol model. Atmospheric Environment . Part A. General Topics , 25A : 2731 – 2748 .
- Tang , I.N. and Munkelwitz , H.R. 1993 . Composition and temperature dependence of the deliquescence properties of hydroscopic aerosols . Atmospheric Environment. Part A. General Topics , 27A : 467 – 473 .
- Salvetti , G. , Tognoni , E. , Tombari , E. and Johari , G.P. 1996 . Excess energy of polymorphic states or glass over the crystal state by heat of solution measurement . Thermochimica Acta , 285 : 243 – 252 .