Abstract
Inhibition of protein oxidation and DNA damage by reactive oxygen species (ROS) would confer benefit to living organisms exposed to oxidative stress, because oxidized proteins are associated with many diseases and can propagate ROS-induced damage. In this study, the ability of the ethanol extracts of Hypericum retusum Aucher (HR) and Hypericum scabrum L (HSm) to protect bovine serum albumin (BSA) from oxidation and DNA damage by hydroxyl radical derived from the Fenton system (Fe3+/H2O2/ascorbic acid) were measured. The ethanol extracts of HR and HSm at different concentrations (50–1000 μg/mL) efficiently prevented protein oxidation induced by hydroxyl radicals as assayed by protein oxidation markers, including protein carbonyl formation (PCO) and polyacrylamide gel electrophoresis. The effect of ethanol extracts of HR and HSm on DNA cleavage induced by UV-photholysis of H2O2 using pBluescript M13+ plasmid DNA were also investigated. These extracts significantly inhibited DNA damage induced by ROS. Therefore, Hypericum retusum, and Hypericum scabrum extracts may be useful in the food industry as effective synthetic antioxidants.
INTRODUCTION
Reactive oxygen species occur in tissues participating in potentially deleterious reactions controlled by a system of enzymatic and non-enzymatic antioxidants that eliminate pro-oxidants and scavenge free radicals.[Citation1] Once radicals form they can either react with another radical or another molecule by various interactions.[Citation2] The rate and selectivity of reactions of this type occurring depends on high radical concentration, delocalization of the single electron of the radical (thus increasing its lifetime), and the absence of weak bonds in any other molecule present with which the radical could interact.[Citation3,Citation4] Oxygen is essential for life but can also harm cells. This is because active forms of oxygen, such as hydroxyl (OH) and superoxide (O2) radicals, hydrogen peroxide (H2O2), and singlet oxygen (1O2), arise as by-products and intermediates of aerobic metabolism and during oxidative stress.[Citation5] Directly or indirectly, these chemical species of oxygen, as well as reactive species of nitrogen, can transiently or permanently damage nucleic acids, lipids, and proteins.[Citation6] Oxidative damage to these cellular macromolecules is implicated in the genesis of several diseases, including neurodegenerative disorders, cancer, liver cirrhosis, cardiovascular diseases, atherosclerosis, cataracts, diabetes, and inflammation.[Citation7] Compounds that can scavenge free radicals have great potential in ameliorating these diseases.[Citation8]
Phenolic compounds have been reported to play key antioxidant roles, especially using the mechanism of delocalization of the single electron of the radical.[Citation9,Citation10] They may produce their beneficial effects by scavenging free radicals.[Citation11] Recently, many researchers have taken a great interest in medicinal plants for their phenolic concentrations-related total antioxidant potential.[Citation12,Citation13] It is reported that some medicinal plants contain a wide variety of natural antioxidants, such as phenolic acids, flavonoids, and tannins, which possess more potent antioxidant activity than dietary plants.[Citation14] It is demonstrated that these compounds are of great value in preventing the onset and/or progression of many human diseases[Citation15,Citation16] and protective effect on oxidative-induced DNA damage in rat liver and intestine.[Citation17–19] Natural antioxidants are also in high demand for application as nutraceuticals, functional food, and bio-pharmaceuticals, because of consumers. Recently, the possibility that free radical damage to proteins might contribute to aging has also received attention. And several groups have focused their research in this area.[Citation20]
The genus Hypericum (Guttiferae) has received considerable interest from scientists, as it contains the variety of structurally diverse natural products, which possess a wide array of biological properties.[Citation21] Recent studies have demonstrated that different species of Hypericum contain compounds, such as flavonoids, xanthones, and phenolic, and can be used as antioxidants.[Citation22] Many pharmacological activities appear to be attributable to hypericin and the flavonoid constituents.[Citation23,Citation24] Flavonoids, the main components present in Hypericum plants, have attracted considerable interest as dietary constituents. Recently, results from clinical studies indicate their possible role in preventing cardiovascular diseases and several kinds of cancer.[Citation25]
The genus Hypericum, which contains more than 400 species, is involved in relatively dry temperate zones of the world. In Turkey, the genus represents 89 species of which 43 are endemic.[Citation26] These plants are used as sedatives and antiseptics in Turkish folk medicine under the names: kantaron, peygamber çiçeği, kanotu, kuzukıran, and binbirdelik otu.[Citation27] Various extracts of different Hypericum species have been examined for their antiinflammatory, antimicrobial, antioxidant, and hypolipidemic activity.[Citation28–31] In the previous study, the antioxidative potential of ethanol extracts of HR and HSm were investigated using different antioxidant tests and 1 mg of HR and HSm ethanol extract was found to be equivalent to 226 and 262 μg of gallic acid, respectively. The authors' previous experiment showed that ethanolic extracts of HR and HSm exhibit antioxidant activity.[Citation32]
Many studies have also focused on DNA damage as estimated by the levels of 8-hydroxy-deoxyguanosine (8-OHdG) after exposure to reactive oxygen species (ROS). It appears that the most prevalent product of DNA oxidation that is detected in genomic DNA in mammalian cells is 8-hydroxy-2-deoxyguanosine.[Citation33] Also, 8-OHdG is a promutagenic base modification that is rapidly removed from DNA by base excision repair mechanisms, although some evidence of removal by transcription-coupled and replication-associated repair also is reported.[Citation34] The half-life of 8-OHdG in mammalian DNA is estimated to be 11 min.[Citation35] If DNA is replicated prior to repair of this base modification, 8-OHdG can cause G to T transversion mutations.[Citation36] This type of mutation is reported in genes whose dysfunction is involved in the genesis of cancer. Such differences clearly obscure the ability to detect physiologically and/or pathophysiologically relevant levels of 8-OHdG and their modulation by antioxidants. Reactive species also damage proteins, several amino acids, especially arginine, histidine, methionine, and cysteine tend to undergo oxidation under conditions of antioxidant deficiency. The reaction products are a variety of protein carbonyls. The presence of carbonyl groups has, therefore, been used as a marker of ROS-mediated protein oxidation.[Citation37] The end products of lipid peroxidation, such as malondialdehyde (MDA) and 4-hydroxy-2-nonenal (HNE), as well as products from polyunsaturated fatty acids cause protein damage.[Citation38]
Currently, many kinds of synthetic antioxidants, such as butylated hydroxyanisole (BHA), butylated hydroxytoluene (BHT), tertiary butyl hyroquinone (TBHQ), and propyl gallate (PG), which are commonly used in processed foods, are known to have toxic and carcinogenic effects on human health. Natural antioxidants, such as α-tocopherol and L-ascorbic acid, are widely used, because they are seen as being safer but their antioxidant activities are lower than those of synthetic antioxidants.[Citation39] Based on accumulative evidence, in recent decades, tremendous interest has considerably increased in finding natural substances (i.e., antioxidants) present in foods or medicinal plants to replace synthetic antioxidants, which are being restricted due to their side effects. On the other hand, polyphenols, used as natural antioxidants, are gaining importance, due to their health benefits for humans, decreasing the risk of cardiovascular and degenerative diseases by reduction of oxidative stress and counteraction of macromolecular oxidation.[Citation40,Citation41]
There are some reports in the literature showing the protective effects of crude extracts of plants or their constituents against protein oxidation and DNA damage induced by ROS.[Citation42,Citation43] It has been shown that extracts from Indian medicinal plants have a significant effect on DNA cleavage induced by UV-photolysis of H2O2.[Citation44] Guglielmi also showed that 4-coumaric acid, a common constituent of some human diets, can reduce basal DNA oxidative damage and induce some GSH-related enzymes.[Citation45] So far, there are negligible reports related to the protective ability of HR and HSm extracts on protein and DNA damage. According to our previous experimental results, this study focuses on the protective effect of ethanol extract of HR and HSm against protein oxidation and DNA damage caused by reactive oxygen species (ROS).
MATERIALS AND METHODS
Collection of Plant Material
Plants Hypericum scabrum L. (HSm) and Hypericum retusum Aucher (HR) were collected in Mardin and Diyarbakır in the area of South East of Turkey in June 2004, by Professor A. Selçuk Ertekin. Voucher specimens have been deposited at the Herbarium of Dicle University (voucher no. DUF-2513-a, DUF-2513-c, respectively). They were identified by Professor Dr. A. Selçuk Ertekin from the University of Dicle, Faculty of Science and Arts, Biology Department.
Preparation of Crude Extract
Crude extracts were prepared as described before.[Citation46] Briefly, aerial parts (stems, leaves, and flowers) of HSm and HR were dried for 10 days at room temperature. A total of 20 g of dried material was ground in an electric blender and then incubated into a glass flask with 2000 mL ethanol (70%) for 3 days under a magnetic stirrer. Solvent was evaporated under vacuum and the crude ethanol extracts of HSm (5 g) as a dark yellow colour and HR (5 g) as a green colour were obtained and kept in dark glass bottles at 4°C until use.
Protein Oxidation
The effects of ethanol extract of both HSm and HR on protein oxidation were carried out according to the slightly modified method of Wang.[Citation47] Bovine serum albumin (BSA) was oxidized by a Fenton-type reaction. The reaction mixture (1.2 mL), containing sample extract (50–1000 μg/mL), potassium phosphate buffer (20 mM, pH 7.4), BSA (4 mg/mL), FeCl3 (50 μM), H2O2 (1 mM), and ascorbic acid (100 μM) were incubated for 30 min at 37°C. For determination of protein carbonyl content in the samples, 1 mL of 10 mM 2,4-dinitrophenylhydrazine (DNPH) in 2 M HCl was added to the reaction mixture. Samples were incubated for 30 min at room temperature. Then, 1 mL of cold TCA (10%, w/v) was added to the mixture and centrifuged at 3000g for 10 min. The protein pellet was washed three times with 2 mL of ethanol/ethyl acetate (1:1, v/v) and dissolved in 1 mL of guanidine hydrocholoride (6 M, pH 2.3). The absorbance of the sample was read at 370 nm. The data were expressed in terms of percentage inhibition, calculated from a control measurement of the reaction mixture without the test sample. BHT was used as a standard and the inhibition percentage of protein oxidation of the sample was calculated by the following equation:
SDS-Polyacrylamide Gel Electropheresis (SDS-PAGE)
To investigate electrophoretic patterns of protective ability of ethanol extracts of HSm and HR on proteins against H2O2/Fe3+/ascorbic acid attack, bovine serum albumin (BSA) was used as a model protein. BSA (1 mg/mL) was dissolved in 20 mM of potassium phosphate buffer (pH 7.4) and then 50 μM FeCl3, 1 mM H2O2, and 100 μM ascorbic acid were added in the reaction mixture and incubated in the presence or absence of butylated hydroxytoluen (BHT) (50–1000 μg/mL), ethanol extracts of HSm (50–1000 μg/mL), and ethanol extract of HR (50–1000 μg/mL) in a final volume of 1.2 mL. After incubation for three hours at 37°C, the reaction mixture was analyzed by electrophoresis in 10% sodium dodecyl sulfate-polyacrylamide gels, using Laemmli's method.[Citation48] Samples were mixed with equal volumes of sample buffer (Tris HCl pH 6.8, 2% SDS, 5% 2-mercaptoethanol, 10% sucrose, and 0.002% Bromophenol blue). The mixture was then boiled for 5 min and 5 μL of each sample was loaded to the wells. The gel was run in a BioRad tank in running buffer (25 mM Tris pH 8.3, 190 mM glycine, and 0.1% SDS) at a maximum voltage and a constant current of 25 mAmp for a mini gel, using a BioRad 1000/500 power supply. Gels were stained with 0.15% Coomassie brillant blue R-250 for two hours and then destained and digitally photographed.
Measurement of Band Intensity and Graph Representation
To determine the amount of protein and DNA damage, band intensity was estimated using the gel documentation system (Gel-Doc-XR; BioRad, Hercules, CA, USA). Bands on the gels were quantified discovery series Quantity One programme (version 4.5.2, BioRad Co.). The density of each band was estimated and standardized with respect to the control group. Results show the average of three different measurements.
DNA Oxidation
DNA damage protective activities of ethanol extract of HSm and HR were checked on pBluescript M13+ plasmid DNA. Plasmid DNA was isolated by Qiagene plasmid mini prep kit. Plasmid DNA was oxidized with H2O2 + UV treatment in the presence of different concentrations of HSm or HR extracts and checked on 1% agarose according to Attaguile et al.[Citation49] after modification. In brief, the experiments were performed in a volume of 10 in a microfuge tube containing 200 ng of plasmid DNA in phospate buffer (7.14 mmol phospate and 14.29 mmol NaCl), pH 7.4, H2O2 was added at a final concentration of 2.5 mmol/L with and without 1μL of (100, 200, 300, 400 μg/mL) ethanol extracts of HSm and HR. The reactions were initiated by UV irradiation and continued for 5 min on the surface of a UV transilluminator (8000 μW cm−1) at 300 nm under room temperature. After irradiation, the reaction mixture (10 μL) with gel loading dye was placed on 1% agarose gel for electrophoresis. Electrophoresis was performed at 40 V for 3 h in the presence of ethidium bromide (10 mg/mL). Untreated pBluescript M13+ plasmid DNA was used as a control in each run of gel electrophoresis along with partial treatment (i.e., only UV treatment and only H2O2). Percent inhibition of the DNA strand scission was calculated as follows[Citation50]:
Densitometric Analysis of Treated and Control pBluescript M13+ Plasmid DNA
Gel was scanned on a Gel documentation system (Gel-Doc-XR; BioRad, Hercules, CA, USA). Bands on the gels were quantified discovery series Quantity One programme (version 4.5.2, BioRad Co.).
Statistical Analysis
Data are presented as the means ± SD. Significant differences among the groups were determined by one-way ANOVA using SPSS 12.0 (SPSS, Chicago, IL, USA) software package program. The results were considered significant if the value of p was less than 0.05. Differences were considered significant at p < 0.05.
RESULTS AND DISCUSSION
Antioxidant activity has been directly linked to the presence of phenolic moieties present in the molecular structure of natural antioxidants. Many phytochemicals having phenolic moieties have been shown to exhibit antioxidant activity.[Citation51,Citation52] Due to the presence of the conjugated ring structures and hydroxyl groups, many phenolic compounds have the potential to function as antioxidants by scavenging a superoxide anion,[Citation53] singlet oxygen,[Citation54] lipid peroxy radicals,[Citation55] and stabilizing free radicals involved in oxidative processes through hydrogenation or complexing with oxidizing species.[Citation56] Some synthetic and natural phenolic compounds utilised in food preservation, protect lipids against oxidation but can accelerate oxidative damage to carbohydrate or DNA.[Citation57] The polyphenol plant extracts content seems to be responsible for the scavenging activity of the reactive oxygen species (ROS), resulting in protection against protein oxidation and DNA damage induced by oxidative stress.
ROS are involved in the normal physiology of living organisms. They act as a messenger for signal transduction and also affect gene expression.[Citation58] Besides, ROS are also involved in the pathogenesis of several chronic diseases, such as aging; neurodegenerative diseases; rheumatoid arthritis; and metabolic diseases like hypertension, diabetes, atherosclerosis, etc.[Citation59–61] The effect of ethanol extracts of HSm, HR, and BHT, on oxidative damage of bovine serum albumin (BSA) induced by • OH is shown in . ROS attack the polypeptide backbone of proteins and lead to the formation of alkyl, alkoxyl, and alkylperoxyl radical intermediates, which set the stage for cleavage of the peptide bond via several means. Tryptophan, histidine, and cysteine residues are especially prone to oxidative attack. The oxidation of those residues may also yield carbonyl derivates. Major molecular mechanisms, leading to structural changes in proteins are free-radical mediated protein oxidation characterized by carbonyl formation (PCO). Indeed, measurement of PCO has been used as a sensitive assay for oxidative damages of proteins.[Citation62] This then can be detected by various means, such as spectrophotometric assay, enzyme-linked immunosorbent assay (ELISA), and one-dimensional or two-dimensional electrophoresis followed by Western blot immunoassay.[Citation63]
Figure 1 Inhibitory effect of butylated hydroxytoluene (BHT), ethanol extract of Hypericum retusum (HR), and ethanol extract of Hypericum scabrum (HSm) on protein (BSA) oxidation expressed as protein carbonyl inhibition induced by Fenton system (Fe3+/H2O2/Ascorbic acid). Each value is expressed as mean ± S.D. of three different experiments. All the values are statistically significant than the control (p < 0.05). *BHT statistically more significant than ethanol extract of HS.
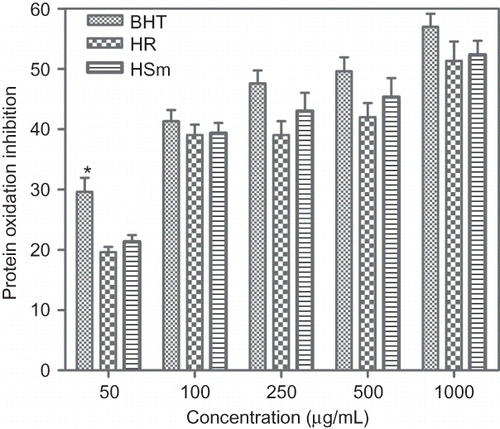
In the present investigation, BHT, HSm, and HR in the range of 50–1000 μg/mL, showed concentration-dependent reduction of albumin oxidation, induced by the H2O2/Fe3+/ascorbic acid system, which resulted in formation of a carbonyl group. The effect of BHT and ethanol extracts of HR and HSm at 1000 μg/mL, reached a plateau of 57 ± 3.7%, 51 ± 5.6%, and 52 ± 4.0% inhibition, respectively. BHT and ethanol extracts of HR and HSm showed a significant inhibitory effect compared with the control (p < 0.05). When BHT compared with ethanol extracts of HR and HSm, the former had a significant inhibitory effect than the latter, at all concentrations. However, at the range of 100–1000 μg/mL, no significant difference was found between inhibitory effect of BHT and ethanol extracts of HR and HSm. The inhibition of protein oxidation by HR and HSm was the result of its scavenging effect on Fe3+/H2O2/ascorbic acid generated reactive oxygen species.
To quantify protein damage, after treatment, protein samples were subjected to SDS-PAGE. Electrophoretic patterns of BSA after incubation with Fe3+ /H2O2/ascorbic acid system in the absence or presence of different concentrations of BHT and the corresponding densitometric analyses of the corresponding bands were presented in and . Three hours after incubation with Fe3+/H2O2/ascorbic acid, the density of BSA band decreased to 32 ± 4.5% of control. One thousand μg/mL of BHT almost completely prevented the oxidative degradation of BSA. At that concentration, BHT restored BSA band intensity to 95 ± 2.5% of control levels.
Figure 2 Protection of BSA oxidative damage by BHT. (a) The electrophoretic pattern of BSA and (b) densitometric analysis of the corresponding band intensity. BSA was oxidised by Fenton system (Fe3+/H2O2/ascorbic acid). The reaction mixture (1.2 mL), containing BHT (50–1000 μg/mL), potassium phosphate buffer (20 mM, pH 7.4), BSA (1 mg/mL), FeCl3 (50 μM), H2O2 (1 mM), and ascorbic acid (100 μM) were incubated for 3 h at 37°C. The oxidative damage of BSA was quantified by SDS-PAGE. SDS gels were digitally photographed and the integrated density of band measured using discovery series Quantity One Programme (version 4.5.2. BioRad Co.). Each bar represents the mean ± S.D. of three different experiments. Means with different letters at a time differ significantly, p < 0.05. The values sharing common letters are not significantly different at p > 0.05. *p < 0.05 compared with Fe3+/H2O2/ascorbic acid treated group.
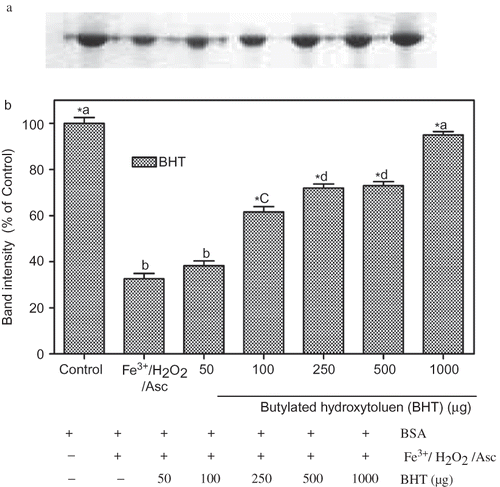
The different concentrations of ethanol extracts of HR and HSm also showed a protective effect on BSA degradation induced by Fe3+/H2O2/ascorbic acid system. The electrophoretic pattern of BSA and densitometric analysis of the corresponding bands are summarized in and b, and b. Densitometric analysis of each protein band showed a quantified gel image and confirmed that the protective effect of HR and HSm on protein against ROS attact more clearly. Ethanol extracts of HR ( and b), at the range of 250, 500, and 1000 μg/mL, protected BSA in a significant manner and also restored the BSA band intensity to 66 ± 6.0%, 75 ± 2.8%, and 96.87 ± 4% of control levels, respectively.
Figure 3 Protection of BSA oxidative damage by ethanol extract of Hypericum retusum (HR). (a) The electrophoretic pattern of BSA and (b) densitometric analysis of the corresponding band intensity. BSA was oxidised by Fenton system (Fe3+/H2O2/ascorbic acid). The reaction mixture (1.2 mL) containing HR (50–1000 μg/mL), potassium phosphate buffer (20 mM, pH 7.4), BSA (1 mg/mL), FeCl3 (50 μM), H2O2 (1 mM), and ascorbic acid (100 μM) were incubated for 3 h at 37°C. The oxidative damage of BSA was quantified by SDS-PAGE. SDS gels were digitally photographed and the integrated density of band measured using discovery series Quantity One Programme (version 4.5.2. BioRad Co.). Each bar represents the mean ± S.D. of three different experiments. Means with different letters at a time differ significantly, p < 0.05. The values sharing common letters are not significantly different at p > 0.05. *p < 0.05 compared with Fe3+/H2O2/ascorbic acid treated group.
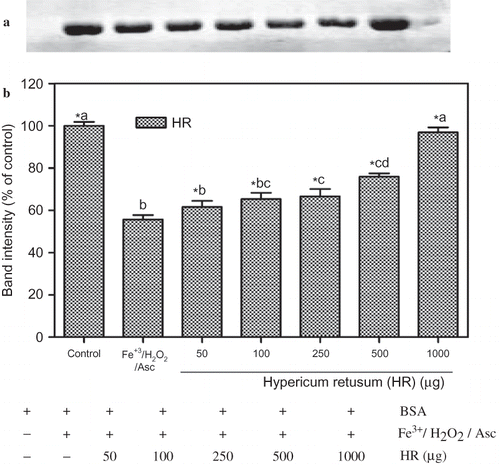
Figure 4 Protection of BSA oxidative damage by ethanol extract of Hypericum scabrum (HSm). (a) The electrophoretic pattern of BSA and (b) densitometric analysis of the corresponding band intensity. BSA was oxidised by Fenton system (Fe3+/H2O2/ascorbic acid). The reaction mixture (1.2 mL) containing HSm (50–1000 μg/mL), potassium phosphate buffer (20 mM, pH 7.4), BSA (1 mg/mL), FeCl3 (50 μM), H2O2 (1 mM), and ascorbic acid (100 μM) were incubated for 3 h at 37°C. The oxidative damage of BSA was quantified by SDS-PAGE. SDS gels were digitally photographed and the integrated density of band measured using discovery series Quantity One Programme (version 4.5.2. BioRad Co.). Each bar represents the mean ± S.D. of three different experiments. Means with different letters at a time differ significantly, p < 0.05. The values sharing common letters are not significantly different at p > 0.05. *p < 0.05 compared with Fe3+/H2O2/ascorbic acid treated group.
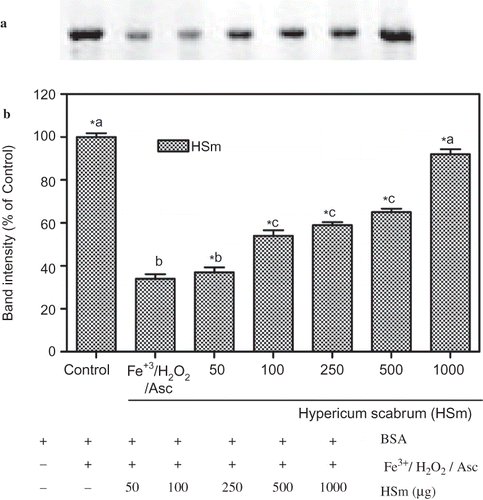
Ethanol extracts of HSm ( and b), in the range of 50–1000 μg/mL, showed a concentration-dependent inhibition of protein oxidation induced by Fe3+/H2O2/ascorbic acid system. At the range of 100, 250, 500, and 1000 μg/mL concentrations, HSm restored the BSA band intensity to 54 ± 4.5%, 59 ± 2.4%, 65 ± 2.8%, and 92 ± 4.0% of control levels, respectively. At these concentrations, ethanol extracts of HSm protected BSA significantly and 1000 μg/mL of HSm almost completely prevented the oxidative degradation of BSA.
Deoxyribonucleic acid is a particularly important target for oxidation, as damage may lead to heritable alterations. Consequently, damage to DNA has been well studied, and several classes of product identified: base oxidation and fragmentation products (e.g., single- and double-strand breaks); inter/intra-strand cross-links; DNA–protein cross-links; and sugar fragmentation products. The hydroxyl radical is perhaps the most frequently considered of damaging species, with over 20 different products formed from OH radical attack on the bases in DNA. Data accumulated over many years clearly show that oxidative DNA damage plays an important role in a number of disease processes. Thus, oxidative DNA damage is implicated in carcinogenesis and neurodegenerative diseases, such as Alzheimer's disease. There is also strong evidence for the role of this type of DNA damage in the aging process.[Citation64]
DNA damage protective activity of ethanol extracts of HR and HSm were checked on pBluescript M13+ vector in E. coli XL-1 Blue strain. Plasmid DNA was isolated by Qiagene mini prep kit. and show the quantified band intensity for the circular supercoiled DNA (form I), circular relaxed DNA (form II), and linear DNA (form III). and show the electrophoretic pattern of DNA after UV-photolysis of H2O2 (2.5 mM) in the absence and presence of ethanol extracts of HR and HSm (100, 200, 300, 400 μg/mL), respectively. DNA derived from pBluescript M13+ DNA plasmid showed two bands on agarose gel electrophoresis (lane 1); the faster moving band corresponded to the native form of supercoiled circular DNA (scDNA) and the slower moving band was the circular relaxed form (ocDNA). The UV irradiation of DNA in the presence of H2O2 (lane 2) resulted in the cleavage of scDNA to linear form (linDNA), indicating that OH radical generated from UV photolysis of H2O2 produced DNA strand scission. The addition of extracts ( and , lanes 7–10) to the reaction mixture suppressed the formation of linear DNA and induced a partial recovery of scDNA. The inhibition activities of the ethanol extracts of HR and HSm on DNA damage were 84.00 ± 1.52%, 95.00 ± 1.0%, 98.00 ± 0.87%, 98.00 ± 1.6% and 87 ± 1.7%, 90.00 ± 1.1%, 95.00 ± 1.2%, 95.00 ± 1.6% at the concentrations of 100, 200, 300, and 400 μg/mL, respectively.
Figure 5 (a) The quantified band intensity for the sc-DNA (form I), oc-DNA (form II), and l-DNA (form III) with Quantity One 4.5.2. version software. (b) Electrophoretic pattern of pBluescript M13(+) DNA after UV-photolysis of H2O2 in the presence or absence of Hypericum retusum (HR). Reaction vials contained 200 ng of supercoiled DNA (31.53 nM) in distilled water (pH 7). Electrophoresis was performed using 1% agarose at 40 V for 3 h in the presence of ethidium bromide (10 mg/mL). Electrophoresis running buffer: TAE (40 mM Tris acetate, 1 mM EDTA, pH 8.2). Lane 1, control DNA; Lane 2, linearized pBluescript M13(+) DNA (EcoRI digest); Lane 3, DNA + H2O2 (2.5 mM) + UV; Lane 4, DNA + UV; Lane 5, DNA + H2O2 (2.5 mM); Lane 6, DNA + HR (200 μg/mL) + UV; Lane 7, DNA + HR (100 μg/mL) + H2O2 (2.5 mM) +UV; Lane 8, DNA + HR (200 μg/mL) + H2O2 (2.5 mM) + UV; Lane 9, DNA + HR (300 μg/mL) + H2O2 (2.5 mM) + UV; Lane 10, DNA + HR (400 μg/mL) + H2O2 (2.5 mM) + UV; Lane 11, DNA + Ethanol + UV; Lane 12, DNA + Ethanol + H2O2 (2.5 mM) + UV; Reactions were all performed at room temperature in phosphate buffer containing 100 mM sodium chloride (color figure available online).
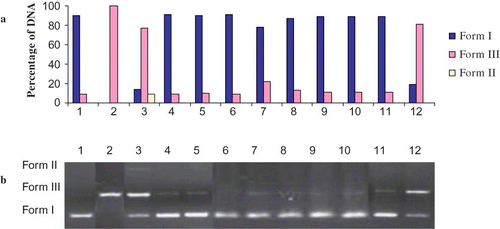
Figure 6 (a) The quantified band intensity for the sc-DNA (form I), oc-DNA (form II), and l-DNA (form III) with Quantity One 4.5.2. version software. (b) Electrophoretic pattern of pBluescript M13(+) DNA after UV-photolysis of H2O2 in the presence or absence of Hypericum scabrum (HSm). Reaction vials contained 200 ng of supercoiled DNA (31.53 nM) in distilled water (pH 7). Electrophoresis was performed using 1% agarose at 40 V for 3 h in the presence of ethidium bromide (10 mg/mL). Electrophoresis running buffer: TAE (40 mM Tris acetate, 1 mM EDTA, pH 8.2). Lane 1, control DNA; Lane 2, linearized pBluescript M13(+) DNA (EcoRI digest); Lane 3, DNA + H2O2 (2.5 mM) + UV; Lane 4, DNA + UV; Lane 5, DNA + H2O2 (2.5 mM); Lane 6, DNA + HSm (200 μg/mL) + UV; Lane 7, DNA + HSm (100 μg/mL) + H2O2 (2.5 mM) + UV; Lane 8, DNA + HSm (200 μg/mL) + H2O2 (2.5 mM) + UV; Lane 9, DNA + HSm (300 μg/mL) + H2O2 (2.5 mM) + UV; Lane 10, DNA + HSm (400 μg/mL) + H2O2 (2.5 mM) + UV; Lane 11, DNA + Ethanol + UV; Lane 12, DNA + Ethanol + H2O2 (2.5 mM) + UV; Reactions were all performed at room temperature in phosphate buffer containing 100 mM sodium chloride (color figure available online).
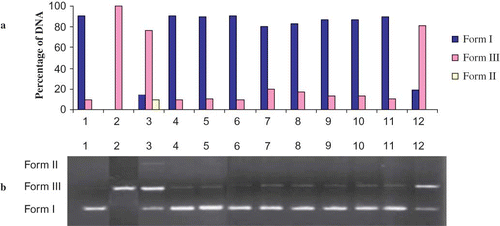
These inhibitory activities on DNA damage slightly increased with each increment of the concentrations. In and , lane 6 represents the effects of ethanol extracts and UV on DNA without H2O2. The ethanol extracts of HR (200 μg) and HSm (200 μg) have no significant effect on DNA. As shown in and (lanes 7–10), ethanol extract from aerial parts of HR and HSm showed a concentration-independent hydroxyl radical scavenging effect. In order to characterize the oxygen radicals leading to DNA cleavage, the effects of three free radical scavengers (DMSO, thiourea, and KI) were studied. It has been found that ethanol extracts of HSm and HT have more protecting activity than DMSO, thiourea, and KI on DNA cleavage (data not shown). Thus, the identification of natural products able to give protection against UV radiation-induced inflammatory responses and the generation of oxidative stress may have important human health implications. The DNA cleavage analysis demonstrated the strong antioxidant properties of HSm and HT. In fact, both these extracts suppressed the formation of linear DNA, generated by exposure of plasmid DNA to OH radical generated by UV-photolysis/H2O2, and induced a partial recovery of scDNA. DNA damage protecting activity of both extracts are corresponding to its antioxidant potential.
CONCLUSIONS
The present study suggested that the ethanol extracts of HR and HSm have antioxidative activity, which was capable of supressing DNA cleavage and protein oxidation in vitro. These Hypericum species may be used as a source of natural antioxidants to stabilize food against oxidative deterioration or as biologically active products to protect cells from damage and may be beneficial in the prevention of reactive oxygen species (ROS)-related diseases, such as cardiovascular, inflammatory, and cancer. The phenolics might be the major active component responsible for the strong antioxidant activity. However, a more detailed investigation between the individual phenolic compounds present in Hypericum species and the antioxidant activities needs to be carried out. The mechanism(s) of antioxidant action of Hypericum species remain(s) open for investigation.
ACKNOWLEDGMENTS
The authors are thankful to Dicle University Scientific Research Funds for the financial support (Grant numbers: 04-FF-55 and 03-FF-63).
REFERENCES
- Paolo , D.M. , Michael , E.M. and Helmut , S. 1991 . Antioxidation defence systems: The role of carotenoids, tocopherols and thiols . American Journal of Clinical Nutrition , 53 : 194 – 200 .
- Okezie , I.A. 1996 . Characterization of drugs as antioxidant prophylactics . Free Radical Biology and Medicine , 20 ( 5 ) : 675 – 705 .
- Bensasson , R.V. , Land , E.J. and Truscott , T.G. 1993 . Excited States and Free Radicals in Biology and Medicine. Contribution from Flash Photolysis and Pulse Radiolysis , 76 Oxford : Oxford University Press .
- Weiss , S.J. 1986 . Oxygen, ischemia and inflammation . Acta Physiologica Scandinavica , 548 : 9 – 37 .
- Halliwell , B. and Gutteridge , J.M.C. 1999 . Free Radical in Biology and Medicine , 614 New York : Oxford Science Publications .
- Panel on Dietary Antioxidants and Related Compounds et al., Panel on Dietary Antioxidants and Related Compounds, Subcommittees on Upper Reference Levels of Nutrients and Interpretation and Uses of Dietary Reference Intakes, the Standing Committee on the Scientific Evaluation of Dietary Reference Intakes, Food and Nutrition Board, Institute of Medicine . 2000 . Dietary Reference Intakes for Vitamin C, Vitamin E, Selenium and Carotenoids , Washington, D.C : National Academy Press .
- Aruoma , O.I. 1998 . Free radical, oxidative stress, and antioxidants in human health and disease . Journal of the American Oil Chemists' Society , 75 : 199 – 212 .
- Kirakosyan , A. , Seymour , E. , Kaufman , O.B. , Warber , S. , Bolling , S. and Chang , S.C. 2003 . Antioxidant capacity of polyphenolic extracts from leaves of Crataegus laevigata and Crataegus monogyna (Hawthorn) subjected to drought and cold stres . Journal of Agricultural Science , 51 : 3973 – 3976 .
- Swallow , A.J. 1953 . The radiation chemistry of ethanol and diphosphopyridine nucleotide and its bearing on dehydrogenase action . Biochemical Journal , 54 : 253 – 257 .
- Mendel , F.J. 1997 . Chemistry, biochemistry and dietary role of potato polyphenols . Journal of Agricultural and Food Chemistry , 45 : 1523 – 1540 .
- Gil , M.I. , Tomas-Barberan , F.A. , Hess-Pierce , B. , Holcroft , D.M. and Kader , A.A. 2000 . Antioxidant activity of pomegranate juice and its relationship with phenolic composition and processing . Journal Agricultural and Food Chemistry , 48 : 4581 – 4589 .
- Mandic , A.I. , Ðilas , S.M. , Letkovic , G.S. , Canadanovic-Brunet , J.M. and Tumbas , V.T. 2008 . Polyphenolic composition and antioxidant activities of grape seed extract . International Journal of Food Properties , 11 : 713 – 726 .
- Okmen , B. , Sigva , H.O. , Mutlu , S. , Doganlar , S. , Yemenicioglu , A. and Frary , A. 2009 . Total antioxidant activity and total phenolic contents in different Turkish eggplant (Solanum melongena L.) cultivars . International Journal of Food Properties , 12 : 616 – 624 .
- Wong , C. , Li , H. , Cheng , K. and Chen , F. 2006 . A systematic survey of antioxidant activity of 30 Chinese medicinal plants using the ferric reducing antioxidant power assay . Food Chemistry , 97 : 705 – 711 .
- Halliwell , B. and Gutteridge , J.M.C. 1989 . “ Free ” . In Radicals in Biology and Medicine , 614 Oxford and New York : Clarendon Press .
- Halliwell , B. , Gutteridge , J.M.C. and Cross , C.E. 1992 . Free radicals, antioxidants, and human disease: where are we now? . The Journal of Laboratory and Clinical Medicine , 119 : 598 – 619 .
- Casalini , C. , Lodovici , M. , Briani , C. , Paganelli , G. , Remy , S. , Cheynier , V. and Dolara , P. 1999 . Effect of complex polyphenols and tannins from red wine (WCPT) on chemically induced oxidative DNA damage in the rat . European Journal of Nutrition , 38 : 190 – 195 .
- Giovannelli , L. , Testa , G. , De Filippo , C. , Cheynier , V. , Clifford , M.N. and Dolara , P. 2000 . Effect of complex polyphenols and tannins from red wine on DNA oxidative damage of rat colon mucosa in vivo . European Journal of Nutrition , 39 : 207 – 212 .
- Lodovici , M. , Casalini , C. , De Filippo , C. , Copeland , E. , Xu , X. , Clifford , M. and Dolara , P. 2000 . Inhibition of 1,2-dimethylhydrazine induced oxidative DNA damage in rat colon mucosa by black tea complex polyphenols . Food and Chemical Toxicology , 38 : 1085 – 1088 .
- Berlett , B.S. and Stadtman , E.R. 1997 . Protein oxidation in aging, disease, and oxidative stress . Journal of Biological Chemistry , 272 ( 33 ) : 20313 – 20316 .
- Patocka , J. 2003 . The chemistry, pharmacology, and toxicology of the biologically active constituents of the herb Hypericum perforatum L . Journal of Applied Biomedicine , 1 : 61 – 73 .
- Cakir , A. , Mavi , A. , Yıldırım , A. , Duru , M.E. , Harmandar , M. and Kazaz , C. 2003 . Isolation and characterization of antioxidant phenolic compounds from the aerial parts of Hypericum hyssopifolium L . by activity-guided fractionation. Journal of Ethnopharmacology , 87 : 73 – 83 .
- Kim , D.O. , Lee , K.W. , Lee , H.J. and Lee , C.Y. 2002 . Vitamin C equivalent antioxidant capacity (VCEAC) of phenolic phytochemicals . Journal of Agricultural and Food Chemistry , 50 : 3713 – 3717 .
- Agostinis , P. , Vantieghem , A. , Merlevede , W. and De-Witte , D. 2002 . Hypericin in cancer treatment: More light on the way . International Journal of Biochemistry & Cell Biology , 34 : 221 – 241 .
- Chu , Y.H. , Chang , C.L. and Hsu , HF. 2000 . Flavonoid content of several vegetables and their antioxidant activity . Journal of The Science of Food and Agriculture , 80 : 561 – 566 .
- Davis , P.H. 1988 . Flora of Turkey and the East Aegean Islands , 399 Edinburgh : Edinburgh University Press .
- Baytop , T. 1999 . Therapy with Medicinal Plants in Turkey , 166 İstanbul : İstanbul University Press .
- Sokmen , A. , Jones , M.B. and Ertürk , M. 1999 . Antimicrobial activity of extracts from the cell cultures of some Turkish medicinal plants . Phytotherapy Research , 13 : 355 – 357 .
- Kizil , G. , Toker , Z. , Özen , H.Ç. and Aytekin , Ç. 2004 . The antimicrobial activity of essential oils of Hypericum scabrum, Hypericum scabroides and Hypericum triquetrifolium . Phytotherapy Research , 18 : 339 – 341 .
- Toker , Z. , Kizil , G. , Özen , H.Ç. , Kizil , M. and Ertekin , S. 2006 . Composition and antimicrobial activities of the essential oils of two Hypericum species from Turkey . Fitoterapia , 77 : 57 – 60 .
- Hakimoglu , F. , Kizil , G. , Kanay , Z. , Kizil , M. and Isi , H. 2007 . The effect of ethanol extract of Hypericum lysimachioides on lipid profile in hypercholesterolomic rabbits and its in vitro antioxidant activity . Atherosclerosis , 192 : 113 – 122 .
- Barış , D. , Kızıl , M. , Aytekin , Ç. , Kızıl , G. , Yavuz , M. , Çeken , B. and Ertekin , S. 2011 . The in vitro antimicrobial and antioxidant activity of ethanol extracts of three Hypericum and three Achillea species from Turkey . International Journal of Food Properties , 14 : 339 – 355 .
- Cadet , J. , Douki , T. , Frelon , S. , Sauvaigo , S. , Pouget , J.P. and Ravanat , J.L. 2002 . Assessment of oxidative base damage to isolated and cellular DNA by HPLC-MS/MS measurement . Free Radical Biology and Medicine , 33 : 441 – 449 .
- Hazra , T.K. , Izumi , T. , Kow , Y.W. and Mitra , S. 2003 . The discovery of a new family of mammalian enzymes for repair of oxidatively damaged DNA, and its physiological implications . Carcinogenesis , 24 : 155 – 157 .
- Hamilton , M.L. , Guo , Z. , Fuller , C.D. , Van Remmen , H. , Ward , W.F. , Austad , S.N. , Troyer , D.A. , Thompson , I. and Richardson , A. 2001 . A reliable assessment of 8-oxo-2-deoxyguanosine levels in nuclear and mitochondrial DNA using the sodium iodide method to isolate DNA . Nucleic Acids Research , 29 : 2117 – 2126 .
- Henderson , P.T. , Delaney , J.C. , Gu , F. , Tannenbaum , S.R. and Essigmann , J.M. 2002 . Oxidation of 7,8-dihydro-8-oxoguanine affords lesions that are potent sources of replication errors in vivo . Biochemistry , 41 : 914 – 921 .
- Stadtman , E.R. and Levin , R.L. 2000 . Protein oxidation . Annals of the New York Academy of Sciences , 899 : 191 – 208 .
- Esterbauer , H. , Schaur , R.J. and Zollner , H. 1991 . Chemistry and biochemistry of 4-hydroxynonenal, malonaldehyde and related aldehydes . Free Radical Biology and Medicine , 11 : 81 – 128 .
- Barlow , S.M. 1990 . “ Toxicology aspects of antioxidants used as food additives ” . In Food antioxidants , Edited by: Hudson , B.J.F. 253 New York : Elsevier . InEds.pp.
- Bingham , M. , Gibson , G. , Gottstein , N. , Pascual-Teresa , S.D. , Minihane , A.M. and Rimbach , G. 2003 . Gut metabolism and cardio protective effects of dietary isoflavones . Current Topics in Nutraceutical Research , 1 : 31 – 48 .
- Silva , B.M. , Andrade , P.B. , Valentãoo , P. , Ferreres , F. , Seabra , R.M. and Ferreira , M.A. 2004 . Quince (Cydonia oblonga Miller) fruit (pulp, peel, and seed) and jam: Antioxidant activity . Journal of Agricultural Food Chemistry , 52 : 4705 – 4712 .
- Ardestani , A. and Yazdanparast , R. 2007 . Inhibitory effects of ethyl acetate extract of Teucrium polium on in vitro protein glycoxidation . Food Chemical Toxicology , 45 : 2402 – 2411 .
- Kizil , G. , Kizil , M. and Çeken , B. 2009 . Protective ability of ethanol extracts of Hypericum scabroides Robson Poulter and Hypericum triquetrifolium Tura against protein oxidation and DNA damage . Food Science and Biotechnology , 18 ( 1 ) : 130 – 136 .
- Russo , A. , Izzo , A.A. , Cardile , V. , Borrelli , F. and Vanella , A. 2001 . Indian medicinal plants as antiradicals and DNA cleavage protectors . Phytomedicine , 8 ( 2 ) : 125 – 132 .
- Guglielmi , F. , Luceri , C. , Giovannelli , L. , Dolara , P. and Lodovici , M. 2003 . Effect of 4-coumaric and 3,4-dihydroxybenzoic acid on oxidative DNA damage in rat colonic mucosa . British Journal of Nutrition , 89 : 581 – 587 .
- Kizil , G. , Kizil , M. , Yavuz , M. , Emen , S. and Hakimoglu , F. 2008 . Antioxidant activities of ethanol extracts of Hypericum triquetrifolium and Hypericum scabroides . Pharmaceutical Biology , 46 ( 4 ) : 231 – 242 .
- Wang , B.S. , Lin , S.S. , Hsiao , W.C. , Fan , J.J. , Fuh , L.F. and Duh , P.D. 2006 . Protective effects of an aqueous extract of Welsh onion green leaves on oxidative damage of reactive oxygen and nitrogen species . Food Chemistry , 98 : 149 – 157 .
- Laemli , U.K. 1970 . Cleavage of structural proteins during the assembly of the head of bacteriophage T4 . Nature , 27 : 680 – 685 .
- Attaguile , G. , Russo , A. , Campisi , F. , Savoca , F. , Acquaviva , R. , Ragusa , N. and Vanella , A. 2000 . Antioxidant activity and protective effect on DNA cleavage of extracts from Cistus incanus L. and Cistus monspeliensis L . Cell Biology and Toxicology , 16 : 83 – 90 .
- Fukuhara , K. and Miyata , N. 1998 . Resveratrol as a new type of DNA-cleaving agent . Bioorganic & Medicinal Chemistry Letters , 8 : 3187 – 3192 .
- Kahkonen , M.P. , Hopia , A.I. , Vuorela , H.J. , Rauha , P. , Pihlaja , K. , Kujala , T.S. and Heinonen , M. 1999 . Antioxidant activity of plant extracts containing phenolic compounds . Journal of Agricultural and Food Chemistry , 47 : 3954 – 3962 .
- Frankel , E.N. 1995 . Natural and biological antioxidants in food and biological systems, their mechanism of action, applications and implications . Lipid Technology , 7 : 77 – 80 .
- Robak , J. and Dryglewski , R.J. 1988 . Flavonoids are scavengers of superoxide anion . Biochemical Pharmacology , 37 : 83 – 88 .
- Husain , S.R. , Cillard , J. and Cillard , P. 1987 . Hydroxyl radical scavenging activity of favonoids . Phytochemistry , 26 : 2489 – 2491 .
- Torel , J. , Cillard , J and Cillard , P. 1986 . Antioxidant activity of avonoids and reactivity with peroxy radical . Phytochemistry , 25 : 383 – 385 .
- Shahidi , F. and Wanasusdara , J.P.K.P.D. 1992 . Phenolic antioxidants . Critical Reviews in Food Science and Nutrition , 32 : 67 – 103 .
- Smith , C. , Halliwell , B. and Aruoma , O.I. 1992 . Protection by albumin against the pro-oxidant actions of phenolic dietary components . Food Chemistry and Toxicology , 30 : 483 – 489 .
- Armario , A. , Company , L. , Borras , M. and Hidalgo , J. 1990 . Vitamin E-supplemented diets reduce lipid peroxidation but do not alter either pituitary-adrenal, glucose, and lactate responses to ımmobilization stress or gastric ulceration . Free Radical Research , 9 ( 2 ) : 113 – 118 .
- Moskovitz , J. , Yim , M.B. and Chock , P.B. 2002 . Free radicals and disease . Archives of Biochemistry and Biophysics , 397 ( 2 ) : 354 – 359 .
- Halliwell , B. and Gutteridge , J.M.C. 1984 . Oxygen toxicity, oxygen radicals, transition metals and disease . Journal of Biochemistry , 219 : 1 – 14 .
- Prakash , D. , Upadhyay , G. , Singh , B.N. and Singh , H.B. 2007 . Antioxidant and free radical-scavenging activities of seeds and agri-wastes of some varieties of soybean (Glycine max) . Food Chemistry , 104 : 783 – 790 .
- Reznick , A.Z. and Packer , L. 1994 . Oxidative damage to proteins: Spectrophotometric method for carbonyl assay . Methods in Enzymology , 233 : 357 – 363 .
- Dalle-Donne , I. , Rossi , R. , Giustarini , D. , Milzani , A. and Colombo , R. 2003 . Protein carbonyl groups as biomarkers of oxidative stress . Clinica Chimica Acta , 329 : 23 – 38 .
- Dizdaroglu , M. 2002 . Introduction to serial reviews on oxidative DNA damage and repair . Free Radical Biology and Medicine , 32 : 677 – 677 .