Abstract
Effects of various osmotic agents (i.e., glucose, fructose, sucrose, maltose, sorbitol, and honey) were evaluated in terms of moisture loss and solid gain besides objective measurements of colour, texture, glass transition temperature; subjective sensory profile; and scanning electron microscopic cellular structure of osmotically dehydrated apple slices. Significantly (p < 0.05) higher solid gains were observed in the samples dipped in glucose and fructose solutions, whereas maltose-treated samples showed higher water loss. The glass transition temperatures varied from −68.4 to −45.6°C, minimum in the case of glucose and maximum in maltose-treated ones. The sucrose- and maltose-treated samples had significantly (p < 0.05) higher L* showing restricted browning. The a* value was maximum and minimum in the case of sucrose- and fructose-treated samples, respectively. Hardness was found to be significantly (p < 0.05) higher (20.104 N) in sucrose-treated samples, while it was at a minimum (4.441 N) in sorbitol-treated ones. The scanning electron microscope studies revealed that cellular structure was retained in sucrose-treated samples, while the damage was observed to be more in the glucose- and fructose-treated ones. The sensory attributes of the osmo-dehydrated samples were found to be better in the case of sucrose-treated samples. The type of humectant, in terms of molecular size, significantly influences the mass transfer process that could be optimized to make the process versatile to meet the requirements of processors and consumers.
INTRODUCTION
Apple is commercially grown in the temperate regions of the world in the latitudes between 30 and 60 North and South. It grows well in climates where the average winter temperature is near freezing for at least two months. It is one of the leading fruits produced in the world and about 39% of total apple production is consumed as fresh, while the remaining 61% are processed. Out of the processed products, juice and cider comprised 46%, canned apples (mostly apple sauce) about 10%, frozen and dried 2% each, and other items (apple jam, apple butter, etc.) about 1%.[Citation1]
Dehydration of fruits is a well-known preservation method, which involves water removal to reduce the risk of microbial growth and proliferation. Moreover, dehydrated fruits can be stored and transported at relatively low cost. However, water removal using high temperatures and extended dehydration times may cause a severe reduction in the nutritive value and adversely affect sensory properties, including flavour, colour, texture, and other properties.[Citation2] One way of producing good quality dehydrated fruits is by using pre-drying treatments, such as osmotic dehydration, which can reduce energy consumption and improve food quality.[Citation3,Citation4] During the osmosis process, water from the tissues flows out and a simultaneous transfer of solute from the solution into the food occurs.[Citation3] Leaching of product solutes (sugars, acids, minerals, vitamins, etc.) into the medium also takes place during this process. The effect of this transfer process on the organoleptic and nutritional characteristic of the product is considered to be quantitatively negligible.[Citation5] Sugar uptake results in the development of a concentrated solid layer under the surface of the fruit, decreasing the osmotic pressure gradient across the fruit-medium interface and, thereby, decreasing the driving force for water flow.[Citation6] The rate of sugar penetration is also dependent on the molecular weight of the sugar and solution concentration. Osmotic drying at high sugar concentrations resulted in products with better physical properties than those treated at lower concentrations. The samples osmosed in glucose had the best characteristics and additionally exhibited higher drying and hardening rates than samples immersed in sucrose solutions. Osmotic drying condition, solute type, and concentration were found to affect the physical properties in terms of compressive fracture stress, porosity, and colour of apple slices.[Citation7] It has been suggested that the ability to predict moisture and sugar contents accurately is useful for producing good quality osmo-dried products.[Citation8] Since osmotic agents have a differential effect on physico-chemical properties of osmo-dried fruits or vegetables, the present study was conducted to see the effect of different osmotic agents on the physical, thermal, structural, and sensory properties of the osmotically dehydrated apple slices.
MATERIALS AND METHODS
Apples (var. Fuji) of approximately the same size and maturity were obtained from a local market and stored at 4°C until processing. All of the chemicals (potassium metabisulphite, calcium chloride, citric acid) and osmotic agents required for osmo-drying of apple slices were procured from SD Fine Chem Limited, Mumbai, India. The apples were washed with chlorinated water (0.1 g kg−1 Cl2), manually peeled and sliced (2 mm thickness, circular discs) in water containing potassium metabisulphite (1 g kg−1) and calcium chloride (1 g kg−1), and dipped in the same solution for 10 min (1:2, w/v). Each experimental block consisted of 10-kg apple slices. The slices were blanched in 60° Brix solutions (1:2, w/v) made up with different sugars (glucose, fructose, sucrose, maltose, sorbitol, and honey) for 2 min at 95°C and subsequently dipped in the same for 2 h at 40°C for osmotic dehydration. Solid gain and water loss were recorded during the osmosis process. The dehydrated apple slices were analysed for solid gain and water loss, CIE colour values, textural, structural, thermal, and sensory properties. The experiment was repeated thrice and the mean values of six replicates were taken for reporting the data.
Water Loss and Solid Gain
During osmotic dehydration water loss and solute gain, both take place simultaneously. The weight of the sample undergoes a reduction due to water loss and at same time weight increases due to a solute gain. The water loss and solute gain were measured using the following equations:
where W 0 is initial weight of sample (g), Wt is weight of slices after osmotic dehydration for any time t (g), S 0 is initial weight of solids (dry matter) in slices (g), and St is the weight of solids (dry matter) in slices after osmotic dehydration for time t (g).
Water Activity
Water activity of the osmo-dried apple slices were measured using a water activity meter (Aqua Lab, Model 3TE; Decagon Devices Inc., Pullman, WA, USA). All the measurements were conducted at 25°C.
CIE Colour Values
Surface colour of the samples were recorded using a colour meter (Mini Scan XE Plus, Model 45/0-S; Hunter Associates Laboratory, Inc., Reston, VA, USA) as reflected in CIELAB (L*a*b*) colour space.[Citation9] All the measurements were referenced to the CIE (Commission Internationale de 1’Eclairage) using the standard illuminant D65 and 10° observer, and the equipment was calibrated using a white and black standard ceramic tile. The lightness coefficient, L*, ranges from black to white on a scale of 0–100. The chromatic attribute chroma [C* = (a*2 + b*2)1/2] and hue angle (h = tan−1(b*/a*) were also determined. Chroma represents the colour saturation and varies from dull (low value) to a vivid colour (high value). The hue angle may be defined as a colour wheel, with red-purple traditionally placed at an angle of 0°, and yellow, bluish-green, and blue following counterclockwise at 90, 180, and 270°, respectively.[Citation10]
Instrumental Texture Profile Analysis (TPA)
Instrumental TPA test was carried out by a compression test that generates plot of force (N) versus time (s) using a texture analyser (TAHDi, Stable Microsystems, Surrey, UK) equipped with a 5-kg load cell. The analyser was linked to a computer that recorded data via a software programme (Texture Expert, version 1.22; Stable Microsystems, Surrey, UK). A 75-mm diameter cylindrical plate was used to measure textural properties. The samples (10 × 5 × 2 mm) were taken and compressed up to 75% of their original height at a speed of 0.5 mm/s with a pre-test and post-test speed of 1 mm/s and 5 mm/s, respectively. The data obtained from the TPA curve were used for the calculation of textural parameters (hardness, adhesiveness, cohesiveness, springiness, and chewiness).[Citation11]
Structural Analysis
A scanning electron microscope was used to observe the surface and internal features of the apple slices. Apple slices with a size of 3 × 3 mm and a thickness of 1 mm were fixed in fixing solution (50 ethyl alcohol; 10 formalin; 5 acetic acid; 35 ml water) and were dehydrated in a graded ethanol-acetone series. The dehydration was initiated in 50% ethanol, passed through 70 and 90% ethanol; then two solvent changes each through 100% ethanol, 3:1, 1:1, and 1:3 ethanol-acetone combinations were made, and thereafter the process was carried out in pure acetone, keeping the tissues for 15 min at each stage. The specimens were then critically dehydrated in a critical point drier (EMS-850, Electron Microscopy Science, Hatfield, PA, USA) using CO2 as transition fluid. The dried samples were mounted over copper stubs using double-stick tape and coated with about 20-nm gold particles in a sputter coater (EMS-550, Electron Microscopy Science, Hatfield, PA, USA). Specimens were observed with a JEOL 100 CX-II electron microscope fitted with a scanning attachment (ASID-4D, JEOL Ltd., Tokyo, Japan) at 20 kV.[Citation12]
Differential Scanning Calorimetry (DSC)
DSC studies were carried out in a DSC-2010 calorimeter (TA Instruments, Inc., New Castle, DE, USA) equipped with a thermal analysis station. The instrument was calibrated by standard procedures. The samples were hermetically sealed in aluminium pans and loaded into the equipment at room temperature, keeping an empty pan as reference. The initial low temperature was achieved using liquid nitrogen as cooling media and 20 ml/min of dry gaseous nitrogen were injected to purge air and avoid water condensation on the DSC stage. Samples were heated from −100 to 110°C at a rate of 10°C/min.[Citation13] The cell was calibrated as per the DSC manufacturer's recommendations. It consists of three procedures (i.e., thermogram line, cell constant, and temperature calibration). The thermogram line calibration involved heating the cell through the entire temperature range, whereas the cell constant was determined by heating the standard material (indium) through its melting temperature. Temperature calibration was performed by using a multiple-point calibration with indium (mp 156.60°C, ΔHm 28.71 J/g), distilled water (mp 0°C, ΔHm 335 J/g), and heptane (mp −91.0°C, ΔHm 140 J/g). Results obtained were analysed using universal analysis software provided with the DSC equipment. The glass transition temperature (onset Tg values) reported in the study correspond to values at which a shift in the baseline of the thermogram was detected during sample scanning. This baseline shift is associated with a step change in the heat capacity of the sample.
Sensory Evaluation
The sensory attributes of osmo-dried apple slices were evaluated in terms of colour, appearance, aroma, taste, texture, and overall acceptability by a trained panel consisting of 10 members from the scientific staff of the laboratory with knowledge of consumer preferences using a 9-point hedonic scale having a score of 9 for extreme liking and 1 for extreme disliking.[Citation14] The samples were served separately to each panel member at 11 a.m. after coding with three digits randomly selected numbers in a sensory lab illuminated with white light and maintained at 20°C. The members of the sensory panel were initially given commercial osmo-dried apple samples and were asked to assess the quality of the experimental samples in terms of sensory colour, appearance, aroma, taste, texture, and overall acceptability.
Statistical Analysis
Three replications were carried out for each measurement and the data obtained were analysed statistically for analysis of variance using completely randomised design and least significant difference at P < 0.05 using Statistica 7 software (Stat Soft, Tulsa, OK, USA).
RESULTS AND DISCUSSION
Apples as such and apple products provide a wide variety of nutrients and have commercial potential due to nourishment and sensory attributes. The apple (var. Fuzi) used for the study had total soluble solids to an extent of 13.5° Brix. The texture of the fruit measured with a 2-mm diameter cylinder showed a firmness value of 34.25 N. The effect of different sugars (glucose, fructose, sucrose, maltose, sorbitol, and honey) during osmotic drying of apple slices was studied with respect to solid gain, water loss, and other physico-chemical and sensory attributes to optimize the process conditions involved.
Solid Gain, Water Loss, and Water Activity
The soaking of apple slices in different humectant solutions (60° Brix) for 2 h at 40°C temperatures had a significant (p < 0.05) effect on solid gain and water loss during osmotic treatment of apple slices (). Glucose, sorbitol, and fructose underwent high solute gain as compared to honey, sucrose, and maltose. The results revealed that the solute gain increased markedly with time, whereas the increase in solute molecular weight decreased the solid gain showing significantly (p < 0.05) lower values in the case of maltose. Similarly, significantly (p < 0.05) higher water loss was observed in the case of maltose and sucrose than that of other sugars. This could be attributed to low molecular mass sugars, such as glucose, sorbitol, and fructose, which favour the sugar uptake. Due to a higher rate of penetration of low molar mass molecules, the solid enrichment occurred instead of dehydration during the process.[Citation3] Low molecular weight solutions have higher corresponding osmotic pressures, which could favour plant cell plasmolysis. Consequently, the osmotic process facilitated uptake of low molecular weight solutes resulting in the spread of plasmolysis throughout the tissues and gradually dehydrating the different tissue layers.
Table 1 Effect of osmotic agents on physico-chemical propertiesFootnote* of osmo-dried apple slices (n = 3)
The molecular weight of the solute in the soaking solution influenced the rate of water transfer in a more complex manner than the simple law described by Roult-Wack et al.[Citation15] for an agar gel model system, devoid of a tissue system, where water loss always increased as the solute molecular weight increased. Low molecular weight solutes quickly and markedly penetrated the fruits, whereas those of higher molecular weight only showed a slight and slow inward motion. This effect could be interpreted in terms of respective solute diffusivities. High molecular weight solutes were more easily blocked at the surface causing a greater osmotic concentration enhancing the dewatering effect during the osmotic treatment. Low molecular mass solutes caused a gradual decrease in the concentration gradient due to rapid penetration of the solute and restricted water driving force facilitating rapid uptake of low molecular weight solutes. This process results in plasmolysis throughout the tissues, whereas high molecular weight solutes resulted in enhanced dehydration due to a marked difference in solute concentration between the surface and the interior of the product. Water loss during osmotic dehydration of apple pieces was found to be more in the samples dipped in higher concentration of sugars and sugar gain tended to increase as the concentration of osmotic solution increased. The sugar gain was also reported to be more in the case of sucrose-treated samples followed by lactose- and maltose-treated ones.[Citation13] Results also showed that the water activity of the osmo-dehydrated apple slices ranged from 0.710–0.733 (). Gekas[Citation16] reviewed data on water activity of different osmotic solutions and reported that different osmotic solutions have different water activity values causing differential osmotic pressures, thereby, differential water and solute activities.
Glass Transition Temperature (Tg )
Fruit tissue, on mechanical standpoint, is regarded as a composite that consists of cell walls that provide elastic support and protoplasmic fluid acting as viscous filler that exerts hydrostatic pressure against cell walls. It also contains intercellular spaces filled initially with air and in the case of apple parenchymatous tissue, it accounts for approximately 25% of the total volume. An increase in the concentration of the plasticizing agent (water in the case of foods) causes a decrease in Tg with the end result of facilitated time and temperature-dependent physical and chemical changes that are responsible for quality losses during food processing and storage.[Citation17,Citation18] The methods for avoiding ‘glass-rubber’ transitions and associated structural collapse are by reducing processing or storage temperatures and adding a Tg depressing agent so as to increase the glass transition temperature of the food material.[Citation16] In the case of osmotically dehydrated apple slices, the Tg values were found to differ significantly (p < 0.05) from −45.6 to −68.4°C, the minimum being in the sample treated with glucose followed by samples treated with fructose, sucrose, sorbitol, honey, and maltose (). A typical DSC thermogram showing Tg is shown in . Water activity affects the Tg values of apple pieces.[Citation13] Slade and Levine[Citation19] reported the onset temperatures of glass-rubber transitions decreased from −15.8 to −86.3°C for freeze-dried grapes and from 20.1 to −90.8°C for freeze-dried strawberries, as aw increased from 0.12 to 0.85. The variation in the Tg values of variously processed apple slices might be due to the differential solid gain causing variation in water activity. The difference might also be due to the type of sugar present in the slices after osmosis. During osmotic dehydration, mobility of solutes occur from higher to lower concentration causing physical and mechanical changes in the tissues. It has also been claimed that mobility of sugars and proteins increase markedly with temperatures near Tg of the dry matrix, which is closely related although lower than Tc .[Citation20]
CIE Colour Coordinates
CIE L*, a*, and b* values of apple slices after osmotic dehydration using different osmotic solutions are presented in . The results showed that the L* value was significantly (p < 0.05) affected by the type of osmotic agent used during the osmotic process. L* values were observed to be the highest (87.75) in the case of maltose-treated samples and lowest (52.45) in the case of honey-treated ones. The CIE a* values, which indicate the redness/greenness component of the colour, increased in osmosed samples during dehydration, but it remained in the greenness (−a) region, except for honey-dipped samples (+5.849). The b* value indicates the yellowness/blueness component of the colour and was found to be significantly (p < 0.05) higher (31.04) in the case of the glucose-treated samples followed by samples treated with fructose, sorbitol, honey, maltose, and sucrose. Use of different osmotic agents during the osmosis process also affected significantly (p < 0.01) the c and h values of the finished product.
Table 2 Effect of osmotic agents on CIE colour valuesFootnote* of osmo-dried apple slices (n = 3)
Sugar impregnation seemed to maintain lightness resulting in a final product close to that of fresh fruit. The colour parameters L* and a* values correlate well to colour changes in fruit tissues (darkening) due to enzymatic browning.[Citation21] As browning increases, L* values decrease and a* values increase, the increase in redness and yellowness was clear and seem to be a result of solids uptake during osmotic pre-treatment. The variations in the colour values of osmotically dehydrated apple slices may be due to the differential solute uptake, which resulted in lower transfer of O2 to the surface.
Instrumental Texture Profile Analysis
The results given in showed instrumental texture profiles of osmo-dried apple slices soaked in different osmotic solutions. It was found that hardness was significantly (p < 0.05) higher (20.104 N) in sucrose-dipped samples and at a minimum (4.441 N) in sorbitol-dipped samples. Fracturability and adhesiveness were significantly (p < 0.05) higher in sorbitol- and honey-osmosed samples, respectively. Springiness and cohesiveness were found to be at a maximum in fructose-treated samples, whereas chewiness was shown to be of a higher magnitude in sucrose-treated samples. Tregunno and Goff[Citation22] studied the effect of osmo-dehydro freezing on rheological testing (force deformation and small scale oscillatory deformation) of apple slices and reported that the presence of sugars increased firmness of thawed tissues compared with apples slices treated in the same manner without sugar; however, frozen controls were firmer than osmo-dehydro frozen samples after thawing, indicating that conditions used during osmotic dehydration (50°C for 40 min) were too severe causing tissue disintegration and textural damage. Lewicki and Lukaszuk[Citation23] dewatered apple cubes by osmosis in 61.5% sucrose solution at 30°C for 3 h and were subsequently dried by convection and found that dewatering caused substantial changes in mechanical properties of apple tissues and found that shrinkage due to osmotic pretreatment was small but stiffness was half the value obtained by convective drying alone. Also, in the present study, the pre-treatment with different osmotic solutions had a differential effect on the textural properties of the apple slices. The higher hardness values in the case of sucrose-treated samples could be due to formation of sugar coating on the surface of the slices.
Table 3 Effect of osmotic agents on texture profileFootnote* of osmo-dried apple slices (n = 3)
Structural Changes
The tissue of a fresh apple is composed of numerous cells and intercellular spaces. In fresh apples, cells are swollen and closely bonded to each other by means of a well-delimited middle lamella. Inside the cells, a large vacuole occupies most of the protoplast, and both the plasmalemma and the tonoplast are kept close to the cellular wall. In fresh apples, cellular walls are formed by cellulose fibrils closely packed together with no discontinuities and consequently, with a dense appearance. Scanning electron microscopic studies were carried out to visualize the structural changes during the osmo-drying process using different osmotic solutions. The results showed that cellular structure retained in sucrose-dipped samples while more damage was observed in the glucose- and fructose-dipped samples (). The apple slices osmosed in glucose, fructose, sorbitol, and honey showed more or less similar structures, whereas maltose- and sucrose-dipped samples were found to be similar in their cellular structure.
Figure 2 Scanning electron micrographs of osmotically dehydrated apple slices: (a) honey, (b) glucose, (c) sorbitol, (d) fructose, (e) sucrose, and (f) maltose (color figure available online).
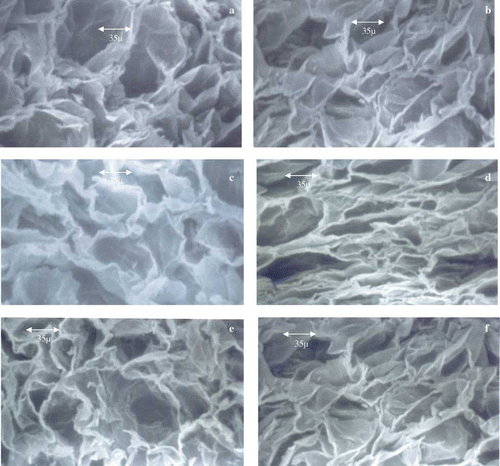
Osmotic dehydration produces plasmolysis in the cells resulting in deformation, contraction, and collapse; similarly, the intercellular spaces also become contracted and deformed. The spaces close to the interphase were filled by osmotic solution that formed a physical barrier against enzymatic browning. The close cell-to-cell contacts that characterize fresh apples were not present in dehydrated apples, where neighboring cell walls were found to be detached and middle lamellae were distorted. The protoplasts and cellular content were found to be retracted due to plasmolysis and were found at the center of the cell. Osmotic dehydration caused thickening and edge twisting of cell walls, as well as detachment of cellular fibrils, producing less density on some areas of the walls. The study showed that solute gain was more in the case of low molecular weight solute as in the case of glucose- and fructose-treated ones. Higher incidence of plasmolysis of cellular structure in glucose- and fructose-treated samples could be attributed to a higher solute gain as compared to sucrose-treated ones. Glenn and Poovaiah[Citation12] also described well preserved cell-to-cell contacts and middle lamella in a histological study on calcium- and sucrose-treated osmo-dried ‘Golden Delicious’ apples. The electron micrographs obtained for osmo-dehydrated apple slices treated with CaCl2 highlights the calcium mediated preserving effect on the microstructure. Calcium-treated osmo-dehydrated cells were found to have better tissue integrity and the plasmolysis was also found to be restricted. Calcium keeps neighboring cells bonded and reinforces the middle lamella, minimizing cellular detachment and cellulose fibrils separation. Therefore, in the present study, the samples treated with CaCl2 and osmosed in sucrose solution showed better textural and structural retention as compared to other samples showing the beneficial effects of CaCl2 and osmosis in sucrose solution.
Sensory Attributes
Sensory evaluation of osmo-dried apple slices revealed that sucrose-treated slices had significantly (p < 0.05) more overall acceptability than other samples (). Honey-treated samples showed significantly (p < 0.05) lower colour and texture values as compared to other samples as it possessed brown colour and leathery texture. The samples treated with sucrose showed significantly (p < 0.05) higher values for taste. The sorbitol osmosed samples had comparatively lower values for aroma, which was found to be the maximum in the case of fructose- and sucrose-dipped samples. The samples treated with maltose, sorbitol, or honey showed lower values for all the sensory parameters. The type of osmosis solution was found to affect the sensory attributes of ‘Golden Delicious’ apple slices, the highest being in the samples osmosed in 90:10 solution of sucrose and maltodextrin.[Citation24]
Table 4 Effect of osmotic agents on sensory attributesFootnote* of osmo-dried apple slices (n = 3)
CONCLUSION
The type of sugar used during the osmosis process in the development of osmo-dried apple slices had significant effects on the physico-chemical, colour, textural, structural, thermal, and sensory properties of the finished product. The slices osmosed in sucrose solution showed better colour in terms of CIE L*, a*, and b* values; texture in terms of hardness, adhesiveness, springiness, cohesiveness, and chewiness; structure in terms of cellular structure and cell wall integrity; and sensory attributes in terms of colour, appearance, aroma, taste, texture, and overall acceptability as compared to the samples osmosed in honey, glucose, sorbitol, fructose, or maltose. Solid gain and water loss during osmosis were higher in glucose- and maltose-treated samples, respectively. The glass transition values were found to be the minimum in the case of glucose-treated samples and maximum in maltose-treated ones. Sucrose-osmosed samples showed comparably lower solid gain and higher water loss, which is desirable in osmo-dried fruits. However, low molecular weight sugars showed restricted crystallization compared to sucrose-treated ones.
REFERENCES
- Forsline , P.L. , Aldwinckle , H.S. , Dickson , E.E. , Luby , J.J. and Hokanson , S.C. 2002 . Collection, maintenance, characterization, and utilization of wild apples of Central Asia . Horticultural Reviews , 29 : 1 – 62 .
- Lenart , A. and Dabrowska , R. 1999 . Kinetics of osmotic dehydration of apples with pectin coatings . Drying Technology , 17 : 1359 – 1373 .
- Torreggiani , D. 1993 . Osmotic dehydration in fruit and vegetable processing . Food Research International , 26 : 59 – 68 .
- Sereno , A.M. , Hubinger , M.D. , Comesana , J.F. and Correa , A. 2001 . Prediction of water activity of osmotic solutions . Journal of Food Engineering , 49 : 103 – 114 .
- Dixon , G.M. , Jen , J.J. and Paynter , V.A. 1976 . Tasty apple slices result from combined osmotic-dehydration and vacuum drying process . Food Process Development , 10 : 634 – 638 .
- Hawkes , J. and Flink , J.M. 1978 . Osmotic concentration of fruit slices prior to freeze dehydration . Journal of Food Processing Preservation , 2 : 265 – 284 .
- Mandala , I.G. , Anagnostaras , E.F. and Oikonomou , C.K. 2005 . Influence of osmotic dehydration conditions on apple air-drying kinetics and their quality characteristics . Journal of Food Engineering , 69 : 307 – 316 .
- Mendoza , R. and Schmalko , M.E. 2002 . Diffusion coefficients of water and sucrose in osmotic dehydration of papaya . International Journal of Food Properties , 5 : 537 – 546 .
- CIE . 1986 . Calorimetry , 2nd , Vienna, , Austria : Publication CIE 15.2; Central Bureau of the Commission Internationale de 1’Eclairage .
- McGuire , R.G. 1992 . Reporting of objective colour measurement . Horticultural Science , 27 : 1254 – 1255 .
- Bourne , M.C. 1978 . Texture profile analysis . Food Technology , 32 ( 7 ) : 62 – 66 .
- Glenn , G.M. and Poovaiah , B.W. 1990 . Calcium-mediated postharvest changes in texture and cell wall structure and composition in Golden Delicious apples . Journal of American Society of Horticultural Science , 115 : 962 – 968 .
- Valle , del J.M. , Cuadros , T.R.M. and Aguilers , J.M. 1998 . Glass transitions and shrinkage during drying and storage of osmosed apple pieces . Food Research International , 31 ( 3 ) : 191 – 204 .
- Larmond , E. 1977 . Laboratory Methods for Sensory Evaluation of Foods , 1637 Ottawa : Canada Dept. of Agric Publication .
- Raoult-Wack , A.L. , Botz , O. , Guilbert , S. and Rios , G. 1991 . Simultaneous water and solute transport in shrinking media. 3. A tentative analysis of the spatial distribution of the impregnating solute in the model gel . Drying Technology , 9 : 630 – 642 .
- Gekas , V. , Gonzalez , C. , Sereno , A. , Chiralt , A. and Fito , P. 1998 . Mass transfer properties of osmotic solutions . I. Water activity and osmotic pressure. International Journal of Food Properties , 1 : 95 – 112 .
- Roos , Y. 1987 . Effect of moisture on thermal behaviour of strawberries studied using differential scanning calorimetry . Journal of Food Science , 52 : 146 – 149 .
- Roos , Y. and Karel , M. 1991 . Plasticizing effect of water on thermal behaviour and crystallization of amorphous food models . Journal of Food Science , 56 : 38 – 43 .
- Slade , L. and Levine , H. 1991 . Beyond water activity: Recent advances based on an alternative approach to the assessment of food quality and safety . CRC Critical Reviews in Food Science and Nutrition , 30 : 115 – 360 .
- Levine , H. and Slade , L. 1986 . A polymer physico-chemical approach to the study of commercial starch hydrolysis products (SHPs) . Carbohydrate Polymer , 6 : 213 – 244 .
- Mastrocola , D. and Lerici , C.R. 1991 . Colorimetric measurements of enzymatic and non-enzymatic browning in apple purees . Italian Journal of Food Science , 3 : 219 – 229 .
- Tregunno , N.B. and Goff , H.D. 1996 . Osmodehydrofreezing of apples: Structural and textural effects . Food Research International , 29 : 471 – 479 .
- Lewicki , P.P. and Lukaszuk , A. 2000 . Effect of osmotic dewatering on rheological properties of apple subjected to convective drying . Journal of Food Engineering , 45 : 119 – 126 .
- Azuara , E. , Beristain , C.I. and Gutierrez , G.F. 2002 . Osmotic dehydration of apples by immersion in concentrated sucrose/maltodextrin solutions . Journal of Food Processing and Preservation , 26 : 295 – 306 .