Abstract
The present study analyzed the flow and viscoelasticity behavior of dispersions of 15 to 45% (w/v) gum arabic, 1 to 8% (w/v) soy proteins, and of gum arabic/soy proteins mixtures. Flow of gum arabic dispersions showed Newtonian behavior, while soy protein dispersions and gum arabic/soy proteins mixtures presented non-Newtonian behavior (n < 1) of shear thinning type. Viscosity increased by 239% in the gum arabic/soy protein mixture (45/8%) compared with gum arabic/soy proteins dispersions (45/0%). The consistency coefficient (K) increased with rising gum arabic or soy proteins concentration in the dispersions. The viscoelastic behavior of mixtures with low gum arabic concentrations showed G′ > G″ values (1712% in gum arabic/soy protein [15/1%] dispersions), behaving as predominantly elastic systems (viscoelastic solids). However, at higher gum arabic concentrations (35 and 45%), the gum arabic/soy protein mixtures presented G′ < G″ values (389% for [45/8%] gum arabic/soy protein), behaving as viscose dispersions (viscoelastic liquids); this shows an antagonistic effect as gum arabic and soy protein concentrations increase, which is more evident in the rheological behavior of the studied mixtures. The interaction between polysaccharides and proteins leads to a better understanding of the rheological behavior of complex mixtures, at high concentrations, allowing these model systems to be useful in the future applications in the food industry.
INTRODUCTION
Food contains important macromolecules, polysaccharides, and proteins among other elements, with characteristic functional properties;[Citation1,Citation2] while polysaccharides act as stabilizers in the aqueous phase, proteins act as an active surface and also play an important role in the stabilization of emulsions.[Citation3,Citation4] Gum arabic (GA) is a polysaccharide commonly employed in emulsions because of its physical properties,[Citation5,Citation6] and soy proteins are mainly used incorporated into textured foods and shredded meat products.[Citation7,Citation8] The soy proteins promote the absorption and retention of fat and it has been reported[Citation9,Citation10] that isolated soy absorbs 50–90% of oil in weight; the use of soy proteins allows to reduce the fat content and increase the protein concentration in a large variety of meat products.[Citation11]
GA is soluble in water and forms dispersions with concentrations of up to almost 50% (w/v) that show Newtonian behavior.[Citation5,Citation12] It is a polysaccharide of natural origin that is extracted from trees of the Acacia genus, of which there are more than 700 species. GA as an exuded is principally obtained from the trees Acacia senegal, Acacia seyal, Acacia laeta, and Acacia tragacanthum;[Citation13] it is a food additive approved by the “Codex Alimentarius”[Citation14] and is used in the food industry because of its properties as a stabilizer and emulsifier.[Citation15] Currently, its contribution as a dietary fiber is being discussed.[Citation16] In 1990, the FAO[Citation17] introduced descriptive criteria for gum arabic stating that it should come from Acacia senegal or other species of Acacia, its optical rotation should be between –26 and –34° and its nitrogen content between 0.27 and 0.39%.
Structurally, GA is a polysaccharide of high molecular weight, with a primary chain composed of β-galactopyranose units bound with 1,3 links, galactopyranose ramifications bound with 1,6 links, and β-D-glucuronopyranose and 4-O methyl-β-D-glucuronopyranose terminations.[Citation18] α-L-Arabinofuranose and α-L-rhamnopyranoside molecules have also been identified, bound by 1,3 and 1,4 links, respectively.[Citation12]
On the other hand, soy proteins (SP) are hydroscopic biopolymers, also employed in the food industry in conjunction with other hydrocolloids, in order to modify the functional properties of processed foods.[Citation19] SP conforms up to 48–50% of the weight of this legume, which, besides its nutritional value, is important for health because the consumption of diverse products derived from this legume prevents a number of illnesses.[Citation20]
In terms of its chemical composition, the main components of SP are the globulins 11S (glycinin) and 7S (β-conglycinin), which form 37 and 31% of the total protein, respectively.[Citation21] The 11S fraction contains monomers composed of an acidic (40 kDa) and a basic polypeptide (20 KDa), linked by a disulfide bond. β-Conglycinin is a glucosyl and heterogeneous protein that contains three different subunits: α, α′, and β with 62, 65, and 47 KDa, respectively.[Citation22] SP participate in metabolism, and they are rich in glucoproteins and glycinin, and besides β- and γ-conglycinin are among the most important storage proteins. SP contain nine essential amino acids at a higher concentration than the daily requirements; besides, they are 95 to 99% digestible, confirming the quality of these proteins.[Citation23,Citation24]
Rheological studies have been performed on soy protein-derived gels[Citation25,Citation26] to determine their interaction with polysaccharides.[Citation19] Also, the coacervation process between proteins and hydrocolloids[Citation27] and the effect of certain hydrocolloids on the digestibility of soy proteins have been examined.[Citation28] Studies concerning the interaction of biopolymers are important to determine the macroscopic characteristics of food products, such as flow, stability, and texture; however, little is known about the contribution of these biopolymers to the rheological behavior of complex mixtures. Few studies have been carried out on the physical interaction between GA and SP;[5] thus, the main objectives of this work were to determine the influence of the concentration of GA and SP on the flow behavior, to obtain the rheological parameters of Ostwald de Waele model, to determine the linear viscoelasticity region (LVR), the behavior of dynamic moduli, and viscoelasticity of their mixtures.
MATERIALS AND METHODS
Materials
The present study determined the viscosity (η), the flow behavior index (n), and the consistency coefficient (K) of the Ostwald de Waele model, as well as the dynamic parameters of viscoelastic behavior; in other terms, the storage modulus (G′), loss modulus (G″), and phase angle (tan δ) for dispersions of GA, SP, and their GA/SP mixtures. Gum arabic of alimentary quality was employed (Farmacia París, México City, México). The gum contained 11.52% moisture, 83.94% carbohydrates, 1.39% proteins, 2.81% ash, and 0.34% fat. Soy protein (Protein Technologies International, St. Louis, MI, USA) consisted of 7.08% moisture, 89.76% protein, 3.04% ash, and 0.12% fat.
Preparation of the Dispersions
GA dispersions at 15, 25, 35, and 45% (w/v) concentrations, and SP dispersions at 1, 3, 5, and 8% (w/v) concentrations were prepared with distilled water on a hot plate stirrer model 524C model (Barnstead Int., IA, USA) with vigorous stirring for 15 min at 25°C. The samples were left to rest for 24 h at 4°C, prior to analysis. Likewise, dispersions of the GA/SP mixtures were prepared at concentrations from 15 to 45% of GA and up to 8% of SP, depending on the description of individual dispersions, firstly incorporating the SP and then the GA at a slow rate, in order to avoid lump formation and to obtain the most homogenous possible systems. Then the dispersions were left to stand at 4°C for 24 h prior to duplicate rheological determinations.
Rheological Study of Flow Behavior
Rheological determinations of simple shear flow were done at 25°C ± 0.1 in a concentric cylinder viscometer RV2 (Haake, Karlsruhe, Germany). The used geometries were MVI for intermediate viscosities (50 - 1.0 × 104 mPa.s) of 20.04 mm radius and 60 mm length; the SVI geometries for high viscosities (300 - 1.0 × 105 mPa.s), of 10.1 mm radius and 61.4 mm length. The determinations were carried out at shear rates between 0 and 2000 s--1 and the rheograms for shear stress (σ) vs. shear rate (γ) were obtained. The data were analyzed using standard software “Rotational C” PG 140 (Karlsruhe, Germany) with a viscometer for obtaining rheological parameters of the Ostwald de Waele model and apparent viscosity (η ap ).
Dynamic Rheological Test
Determinations were carried out at 25°C ± 0.1 in a stress-controlled rheometer LS100 Paar Physica (Stuttgart, Germany) connected to a power source and fitted to a water-recycling bath Julabo F10 (Stuttgart, Germany). The geometry was DG1 with a diameter of 48.0 mm, 36.0 mm in length, for shear rates between 1.22 × 10--4 and 2.45 × 103 s--1 and shear stress between 6.37 × 10--3 and 6.37 × 101 Pa. For calculation of the dynamic parameters, the Paar Physica software version 2.06–E was used. An amplitude scan was performed to determine the linear viscoelasticity region (LVR), which is obtained when the storage modulus (G′) and the loss modulus (G″) become independent of amplitude.[Citation5,Citation29,Citation30] Subsequently, a frequency scan was performed within the 6 to 60 rad s--1 interval to determine the behavior of elastic and viscose components related to oscillation frequency.
Statistical Analysis
Variance analysis was performed on all data obtained from the storage modulus (G′) and loss modulus (G″) as a function of the concentration of gum arabic (GA), soy protein (SP), as well as mixtures thereof; with a statistical difference of α = 0.05 using Design Ease ver. 6.0 for windows (Stat-Ease, Inc., MN, USA)—software for design of experiments. The null hypothesis proposes, that at average level, G′ and G″ were equal regardless of the concentration. The calculated F was compared with the theoretical F.[Citation31]
RESULTS AND DISCUSSION
Behavior of Flow Dispersions
Figure 1a presents the behavior of GA at concentrations of 15 to 45% (w/v). The GA dispersions showed Newtonian behavior except at 45% where they showed non-Newtonian behavior of shear thinning type. Similarly, the SP dispersions at concentrations of 1 to 8% (w/v) () showed non-Newtonian behavior of shear thinning type. Mothe and Rao[Citation32] obtained values for the index of flow behavior between 0.150 and 0.750 with GA dispersions at concentrations of 4 to 50% using the Sisko model and these showed non-Newtonian behavior. Sanchez et al.[Citation12] found non-Newtonian behavior of shear thinning type in GA dispersions at concentrations of 3 to 32%, at a shear rate of 100 s--1. In this study, Newtonian behavior was observed for GA dispersions. This difference may be due to the fact that determinations were done at high shear rates, where Newtonian behavior was reported at concentrations higher than 10%.[Citation33] In addition, the source of GA, its purity, and the method of preparation of dispersions may also have influenced results.[Citation5]
Figure 1 Flow behavior of dispersions of (a) gum arabic (GA): (15%), (25%), (35%), (45%); and (b) soy protein (SP): (1%), (3%), (5%), (8%).
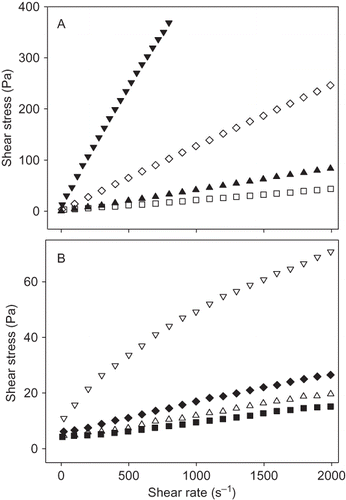
shows the rheological parameters according to the Ostwald de Waele model, with GA/SP mixtures at different concentrations. The viscosity of mixtures increased up to 239%, compared with the GA dispersions (374 mPas and 1269 mPas to 45% of GA and GA/SP [45/8%], respectively). Viscosity of these mixtures was higher than for GA dispersions at low concentrations of GA and SP (16 mPas (15/0%) and 10 mPas [0/1%] respectively) with increases in viscosity of 75%. As the concentration of GA in the mixtures rose (from 15 to 45%) the viscosity of the mixtures increased (4432%). This may have been due to a synergic effect with the SP, to an increase in concentration is increased and to possible GA-SP interactions.
Table 1 Rheological parameters according to the Ostwald de Waele model for mixtures of gum arabic/soy protein (GA/SP), at varying concentrations
Independently of GA concentration, the values of the consistency coefficient (K) increased as SP concentration rose, from 8 mPas n for GA/SP 15/1 up to 2132 mPas n for GA/SP 45/8; while the values for flow behavior index (n) for mixtures with 15% GA showed values near to 1, indicating that the dispersions behaved as Newtonian fluids, in contrast to the GA/SP (15/1%) mixture whose n value was more distant from 1. Concerning the GA mixtures at 25, 35, and 45%, n values were obtained of up to 0.775, indicating that these were systems with non-Newtonian behavior of the shear thinning type. Berli et al.,[Citation25] reported non-Newtonian behavior of the shear thinning type for SP dispersions with 9.1% (w/w), using the Cross model to obtain rheological parameters; Puppo et al.[Citation34,Citation35] found the same behavior as Berli et al.[Citation25] in SP dispersions structurally modified by thermal treatment and acidification, as well as in emulsions prepared from SP and maize oil. The results from these authors agree with the present results concerning SP dispersions.
Viscoelastic Behavior
shows the linear viscoelasticity region (LVR) for dispersion GA and SP ( and ) as well as for mixtures of GA/SP at concentrations of 15, 25, 35, and 45% of GA and 1, 3, 5, and 8% of SP, respectively (–). As the greater the concentration of biopolymer, in each individual dispersion of GA or SP, the highest stress value ( and ), obtaining values close to 600 Pa for the elastic component (G′) in the SP dispersion at 8%, about three times the amount of stress for dispersions with 45% GA. In relation to mixtures GA/SP, depending on the concentration of GA and SP, and lower stress values, about 90% of the mixtures had the highest concentrations of GA and SP (). The linear viscoelasticity region is obtained when the storage modulus (G′) and the loss modulus (G″) are independent of amplitude.[Citation29,Citation30] Previous studies of gum arabic/maize starch (GA/MS) mixtures[Citation5] have shown a similar tendency when the concentration of GA is increased. However, torque amplitude becomes approximately three to four times greater as maize starch concentration increases (MS); thus, it is probable that the presence of starch influences the behavior of the GA/MS mixture.
Figure 2 Amplitude sweep on the storage moduli (G′) and loss moduli (G″) for dispersions (a) GA at 15, 25, 35, and 45%; (b) SP at 1, 3, 5, and 8%; (c) GA/SP mixtures, 15% GA and up to 8% of SP; (d) GA/SP mixtures, 25% GA and up to 8% of SP; (e) GA/SP mixtures, 35% GA and up to 8% of SP; (f) GA/SP mixtures, 45% GA and up to 8% of SP.
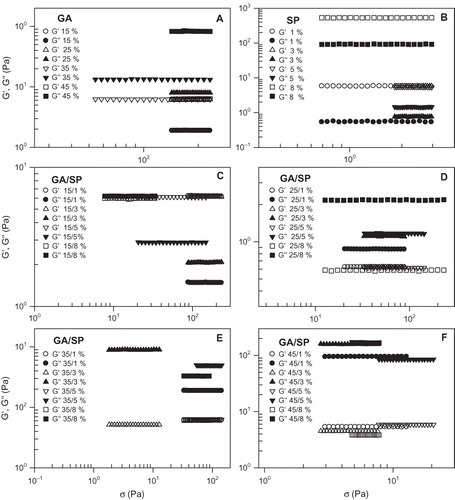
presents the behavior of the dynamic moduli as they relate to frequency (ω). In the mixtures containing GA/SP at 15 and 25% of GA ( and ; and , respectively) added with 0, 1, 3, 5, and 8% of SP, the storage modulus (G′) represents the elastic component (energy temporarily stored in the material) and the loss modulus (G″), represents the viscose component (energy used to activate the flow and which is dissipated and transformed into heat). The figure shows that G′ ( and ) increased by approximately two logarithm cycles, approximately 83.33 to 100.00 times, as the frequency in the studied mixtures increased. The exception was the GA/SP 15/8% mixture, where in a frequency of 11 rad s--1, less dependence of the elastic component (G′) on the frequency was observed. Whenever frequency exceeded (20 rad s--1) in mixtures with 15% of GA ( and ), the dispersion behavior was more elastic than viscose (G′ > G″), showing a viscoelastic behavior with a predominant elastic component, once more with the exception of the GA/SP (15/8%) mixture, where the gum protein system was more viscose than elastic (G″ > G′), probably due to an antagonistic effect caused by the high soy protein concentration. A similar behavior has been observed in aqueous systems containing starch/galactomannans[Citation36] as well as in mixtures of gum arabic/maize starch.[Citation5]
Figure 3 Behavior of dynamic moduli (G′, G″) in function of the frequency in gum arabic/soy protein mixtures (GA/SP) with 15 and 25% of GA, and 0% SP (, ); 1% SP (, ); 3% SP (, ); 5% SP (, ); and 8% SP (, ).
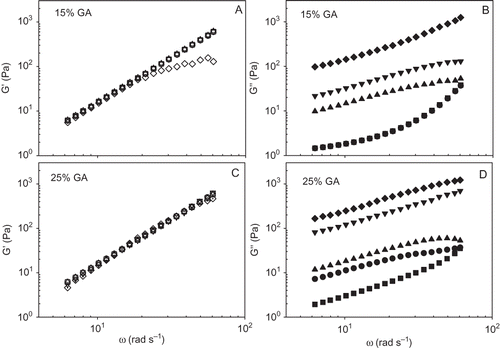
The loss modulus G″ ( and ) increased in relation to increasing SP concentration in mixtures with 15 and 25% of GA. In 15% of GA dispersions without SP, the G″ value was similar to that of the 15/1% GA/SP mixture, while the 25% GA dispersion without SP had a higher G″ value than that of the GA/SP (25/1%) mixtures, suggesting a possible antagonistic effect of SP. This situation was also observed in the flow behavior of GA/SP mixtures (data not shown). The 25% GA mixtures showed viscoelastic behavior of a predominantly elastic character (G′ > G″) only when SP concentrations were less than 5% (), and when frequencies were above 15 rad s--1. The dynamic moduli (G′ and G″) were strongly dependent on frequency, particularly G″, which increased along the study interval from 6 to 60 rad s--1 by approximately three logarithmic cycles, from 500 to 666 times its value.
shows the behavior of G′ (storage modulus) and G″ (loss modulus) as a function of frequency (ω) in GA/SP mixtures with 35 and 45% of GA containing 0, 1, 3, 5, and 8% of SP. The elastic component (G′) rose in the same magnitude ( and ), approximately two logarithmic cycles, about 83.33 to 100.00 times, as frequency increased from 6 to 60 rad s--1. The viscose component (G″) also increased with rising frequency and became higher with increasing SP concentration by approximately three logarithmic cycles, from about 166.66 to 350 times, in the above-mentioned frequency interval ( and ).
Figure 4 Behavior of dynamic moduli (G′, G″) in function of the frequency in gum arabic/soy protein mixtures (GA/SP) with 35 and 45% of GA, and 0% SP (, ); 1% SP (, ; 3% SP (, ); 5% SP (, ); and 8% SP (, ).
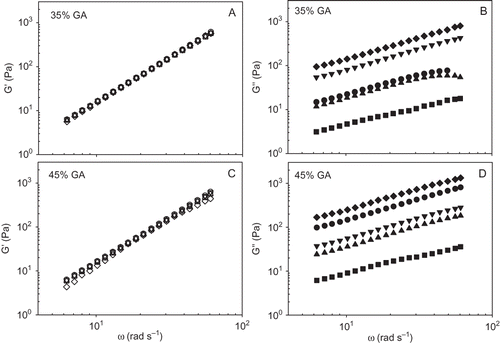
In dispersions containing only GA at 35% without SP, the viscose component was higher than in mixtures containing 1 and 3% SP, but lower than in mixtures containing 5 and 8% of SP, where the elastic component predominates (G′ > G″) (). However, in dispersions containing 45% GA, without SP (), the viscose component was higher than in the mixtures containing 1, 3, and 5% SP. Thus, as the GA concentration in the mixture increased, the antagonism became greater. As the frequency rises, starting at approximately 20 rad s--1, the mixtures containing 35 and 45% GA ( and ), showed a more elastic rather than viscose (G′ > G″) behavior, characteristic of macromolecular dissolutions.[Citation29,Citation37] The exception was the GA/SP (15/8%) mixture, where the system was more viscose than elastic (G″ > G′).
The observed behavior is probably the reflection of transition zones and may be explained in terms of the association of chains of biopolymer structures in dissolution, where a viscoelastic behavior in between the solid and the liquid type prevails, and where a transition from a macromolecular dissolution to a viscoelastic fluid occurs that is predominantly elastic and liquid in appearance, but shows greater elasticity.[Citation37–39] Independently of the GA concentration, 15 and 25% ( and ) or 35 and 45% ( and ), at low oscillation frequencies most mixtures display a rheological behavior similar to a fluid where G″ > G′. The “crossover” point in the curve representing G″ and G′ is located approximately between 15 and 20 rad s--1, and at greater frequencies the behavior of the mixture was in fact similar to that of a solid where G′ > G″.
The dynamic parameters of the GA/SP mixtures at the concentrations under study are shown in ; the torque amplitude was between 0.086 and 3.465 mNm, and was higher with low SP content. In the majority of the studied mixtures, the elastic component (G′) was greater than the viscose component (G″), tan δ < 1, in mixtures of up to 5% of SP; i.e., they showed a rheological viscoelastic behavior predominantly elastic in character. In the control dispersions of GA without SP, the G′ values increased slightly (although statistically significant α = 0.05), from 145 to 150 Pa, as the concentration of GA increased and they were dependent on the frequency of G′ α ω2.00–2.06; whereas the G″ values increased up to 157%, with values of 7 to 18 Pa, so that G′ > G″ and they were dependent on the frequency of G′ α ω0.70–1.20. The values for the calculated exponents are those commonly presented by viscoelastic liquids.[12] In all the mixtures, values between 120 and 150 Pa were obtained for G′ and were dependent on the frequency of G′ α ω2.00–2.05, except for the GA/SP 15/8% mixture, whose G′ value was 63 and showed dependency on the frequency of G′ α ω1.47. The viscose component (G″) increased with rising SP concentration up to 5075% and frequency dependency was in the G″ α ω0.72–1.12 interval. In accord with the exponent values, the mixtures manifested the structural behavior of liquid samples.[12]
Table 2 Dynamic parameters in gum arabic/soy protein mixtures (GA/SP) at varying concentrations. Dependency of G′ and G″ on the frequency
GA dispersions are Newtonian fluids, the presence of SP influences the rheological behavior of dispersions transforming non-Newtonian fluids (), with increasing concentration of GA and SP evidently increases the viscosity; however, at higher concentrations of SP (5 and 8%, mainly) provides a change of G′ > G″ systems with G″ > G′ (), indicating a strong inhibition or antagonism at higher concentrations of biopolymers.
Puppo et al.[Citation34,Citation35] performed rheological studies of 10% (w/w) SP dispersions, applying different thermal and acidity treatments, as well as on SP and maize oil emulsions, and found that most studied samples showed an elastic behavior. Apichartsrangkoon[Citation40] determined the viscoelastic behavior of SP and gluten mixtures reporting that in accordance with the present study, G′ > G″, and that G′ showed dependency on any frequency exceeding that of G″. Similar results were obtained in the present study when GA was mixed with SP at low concentrations showing a viscoelastic behavior predominantly elastic in character. Knowledge of viscosity and viscoelasticity, rheological behavior, of a mixture or complex systems, can be used to design foods with high concentrations of polysaccharides and proteins, and these results could also be used to determine the interaction between components.
CONCLUSIONS
The studied GA dispersions (15, 25, and 35%) displayed Newtonian behavior, while 45% GA dispersions and SP dispersions of 1, 3, 5, and 8% showed non-Newtonian flow behavior of the shear thinning type. Most studied dispersions behaved predominantly as elastic systems (tan δ < 1), in virtue of the fact that G′ > G″. Concerning dynamic behavior, an antagonistic effect was observed in the GA/SP mixtures, possibly due to polysaccharide-protein interactions between GA and SP, which were segregated type. G′ did not vary in the mixtures when protein was incorporated, while G″ was greatly modified. Results of this study will contribute to a better understanding of the interaction between proteins and polysaccharides in mixtures, antagonisms and synergisms in food, with possible applications in the food industry, such as food model systems, source of soluble fiber, and fat substitutes.
ACKNOWLEDGMENT
The authors wish to thank to M. Márquez R. for the technical support.
REFERENCES
- Sikora , M. , Badrie , N. , Deisingh , A.K. and Kowalski , S. 2008 . Sauces and dressings: A review of properties and applications . Critical Reviews in Food Science and Nutrition , 48 ( 1 ) : 50 – 77 .
- Kasapis , S. 2008 . Phase separation in biopolymer gels: A low- to high-solid exploration of structural morphology and functionality . Critical Review in Food Science and Nutrition , 48 ( 4 ) : 341 – 359 .
- Flores , M. , Giner , E. , Fiszman , S.M. , Salvador , A. and Flores , J. 2007 . Effect of a new emulsifier containing sodium stearoyl-2-lactylate and carrageenan on the functionality of meat emulsion systems . Meat Science , 76 ( 1 ) : 9 – 18 .
- McClements , D.J. 2007 . Critical review of techniques and methodologies for characterization of emulsion stability . Critical Reviews in Food Science and Nutrition , 47 ( 7 ) : 611 – 649 .
- Jiménez-Avalos , H.A. , Ramos-Ramírez , E.G. and Salazar-Montoya , J.A. 2005 . Viscoelastic characterization of gum arabic and maize starch mixture using the Maxwell model . Carbohydrates Polymers , 62 : 11 – 18 .
- Gómez-Díaz , D. , Navaza , J.M. and Quintáns-Riveiro , L.C. 2009 . Intrinsic viscosity and flow behavior of arabic gum aqueous solutions . International Journal of Food Properties , 11 ( 4 ) : 773 – 780 .
- Parmer Jr , E.L. , Wang , B. , Aglan , H.A. and Mortley , D. 2004 . Physicochemical properties of texturized meat analog made from peanut flour and soy protein isolate with a single-screw extruder . Journal of Texture Studies , 35 ( 4 ) : 371 – 382 .
- Das , A.K. , Anjaneyulu , A.S.R. , Gadekar , Y.P. , Singh , R.P. and Pragati , H. 2008 . Effect of full-fat soy paste and textured soy granules on quality and shelf-life of goat meat nuggets in frozen storage . Meat Science , 80 ( 3 ) : 607 – 614 .
- Chen , S.T. , Chen , J.R. , Yang , C.S. , Peng , S.J. and Ferng , S.H. 2006 . Effect of soya protein on serum lipid profile and lipoprotein concentrations in patients undergoing hypercholesterolaemic haemodialysis . British Journal of Nutrition , 95 ( 2 ) : 366 – 371 .
- Torres , N. , Torre-Villalvazo , I. and Tovar , A.R. 2006 . Regulation of lipid metabolism by soy protein and its implication in diseases mediated by lipid disorders . Journal of Nutritional Biochemistry , 17 ( 6 ) : 365 – 373 .
- Hsu , S.Y. and Sun , L.Y. 2006 . Comparisons on 10 non-meat protein fat substitutes for low-fat Kung-wans . Journal of Food Engineering , 74 ( 1 ) : 47 – 53 .
- Sanchez , C. , Renard , D. , Robert , P. , Schmitt , C. and Lefebvre , J. 2002 . Structure and rheological properties of acacia gum dispersions . Food Hydrocolloids , 16 : 257 – 267 .
- Al-Assaf , S. , Phillips , G.O. , Aoki , H. and Sasaki , Y. 2007 . Characterization and properties of Acacia senegal (L.) Willd. var. senegal with enhanced properties (Acacia (sen) SUPER GUMTM): Part 1—Controlled maturation of Acacia senegal var. Senegal to increase viscoelasticity, produce a hydrogel form and convert a poor into a good emulsifier . Food Hydrocolloids , 21 : 319 – 328 .
- Al-Assaf , S. , Phillips , G.O. and Williams , P.A. 2005 . Studies on acacia exudates gums. Part I: The molecular weight of Acacia Senegal gum exudate . Food Hydrocolloids , 19 : 647 – 660 .
- Verbeken , D. , Dierckx , S. and Dewettinck , K. 2003 . Exudate gums: Occurrence, production, and application . Applied Microbiology and Biotechnology , 63 : 10 – 21 .
- Phillips , G.O. , Ogasawara , T. and Ushida , K. 2008 . The regulatory and scientific approach to defining gum Arabic (Acacia senegal and Acacia seyal) as a dietary fiber . Food Hydrocolloids , 22 : 24 – 35 .
- FA , O. 1990 . “ Specifications for identity and purity of certain food additives ” . In Food and Nutrition; Paper No Vol. 49; FAO , 23 – 25 . Rome , , Italy
- Cui , S.W. , Phillips , G.O. , Blackwell , B. and Nikiforuk , J. 2007 . Characterization and properties of Acacia senegal (L.) Willd. var. senegal with enhanced properties (Acacia (sen) SUPERGUM™): Part 4. Spectroscopic characterization of Acacia senegal var. senegal and Acacia (sen) SUPERGUM™ arabic . Food Hydrocolloids , 21 ( 3 ) : 347 – 352 .
- Kruif , C.G. and Tuinier , R. 2001 . Polysaccharide protein interactions . Food Hydrocolloids , 15 : 555 – 563 .
- Sirtori , C.R. , Lovati , M.R. , Manzoni , C. , Monetti , M. , Pazzucconi , F. and Gatti , E. 1995 . Soy and cholesterol reduction: Clinical experience . Journal of Nutrition , 125 : 598S – 605S .
- Cao , N. , Fu , Y. and He , J. 2007 . Preparation and physical properties of soy protein isolate and gelatin composite films . Food Hydrocolloids , 21 : 1153 – 1162 .
- Lam , M. , Shen , R. , Paulsen , P. and Corredig , M. 2007 . Pectin stabilization of soy protein isolates at low pH . Food Research International , 40 : 101 – 110 .
- Lusas , E.W. and Riaz , M.N. 1995 . Soy protein products: processing and use . Journal of Nutrition , 125 : 573S – 580S .
- Dias , K. , Myers , D.J. , Bian , Y. , Lihono , M.A. , Wu , S. and Murphy , P.A. 2003 . Functional properties of the acidic and basic subunits of the glycinin (11S) soy protein fraction . Journal of the American Oil Chemists Society , 80 : 551 – 555 .
- Berli , C.L.A. , Deiber , J.A. and Añón , M.C. 1999 . Connection between rheological parameters and colloidal interactions of a soy protein suspension . Food Hydrocolloids , 13 : 507 – 515 .
- Renkema , J.M.S. 2004 . Relations between rheological properties and network structure of soy proteins gels . Food Hydrocolloids , 18 : 39 – 47 .
- Weinbreck , F. , de Vries , R. , Schooyen , P. and de Kruif , C.G. 2003 . Complex coacervation of whey proteins and gum arabic . Biomacromolecules , 4 : 293 – 303 .
- Mouécoucou , J. , Villaume , C. , Sanchez , C. and Méjean , L. 2004 . Effects of gum arabic, low methoxy pectin and xyland on in vitro digestibility of peanut protein . Food Research International , 37 : 777 – 783 .
- Steffe , J.F. 1992 . Rheological Methods in Food Process Engineering , East Lansing , MI : Freeman Press .
- Yáñez-Fernández , J. , Ramos-Ramírez , E.G. and Salazar-Montoya , J.A. 2008 . Rheological characterization of dispersions and emulsions used in the preparation of microcapsules obtained by interfacial polymerization containing Lactobacillus sp . European Food Research and Technology , 226 ( 5 ) : 957 – 966 .
- Walpole , R.E. and Myers , R.H. 1997 . Probabilidad y Estadística , 4ta , 797 – 808 . México : McGraw-Hill .
- Mothé , C.G. and Rao , M.A. 1999 . Rheological behavior of aqueous dispersions of cashew gum and gum Arabic: Effect of concentration and blending . Food Hydrocolloids , 13 : 501 – 506 .
- Meer , W. 1980 . Gum Arabic in Handbook of Water-Soluble Gums and Resins , 8.1 – 8.24 . Monterey, CA , , USA : McGraw-Hill .
- Puppo , M.C. , Sorgentini , D.A. and Añón , M.C. 2000 . Rheological study of dispersions prepared with modified soybean protein isolates . Journal of the American Oil Chemists Society , 77 ( 1 ) : 63 – 71 .
- Puppo , M.C. , Sorgentini , D.A. and Añón , M.C. 2003 . Rheological properties of emulsions containing modified soy protein isolates . Journal of the American Oil Chemists Society , 80 ( 6 ) : 605 – 611 .
- Closs , C.B. , Conde-Petit , B. , Roberts , I.D. , Tolstoguzov , V.B. and Escher , F. 1999 . Phase separation and rheology of aqueous starch/galactomannan system . Carbohydrates Polymers , 39 : 67 – 77 .
- Clark , A.H. and Ross-Murphy , S.B. 1987 . “ Structural and mechanical properties of biopolymer gels ” . In Advances in Polymer Science , Edited by: Clark , A.H. , Kamide , K. , Ross-Murphy , S.B. and Saito , M. Vol. 83 , 59 – 192 . Berlin, Heidelberg, New York , London, Paris, Tokyo : Springer-Verlag .
- Doublier , J.L. 1994 . “ Rheological investigation of polysaccharide interactions in mixed system ” . In Gums and Stabilizers for the Food Industry , Edited by: Phillips , G.B. , Williams , P.A. and Wedlock , D.J. 257 – 270 . Oxford , , New York : IRL Press at Oxford University Press . Oxford, New York
- Meza , B.E. , Verdini , R.A. and Rubiolo , A.C. 2009 . Viscoelastic behavior of heat-treated whey protein concentrate suspensions . Food Hydrocolloids , 23 : 661 – 666 .
- Apichartsrangkoon , A. 2002 . Dynamic viscoelastic properties of heated gluten/soy protein gels . Journal of Food Science , 67 : 653 – 657 .