Abstract
The mechanical characteristics (rupture force, maximal deformation, and rupture energy) of red and green lentils under compression loading were determined as a function of moisture content ranging from 9.5 to 21.1% (w.b.). Scanning electron microscopy, laser diffraction particle size analysis, and instrumental texture evaluation were successfully applied to relate the microstructure and texture of different lentil seed varieties. Results demonstrated that all of the mechanical parameters of the green lentils, which have smaller starch granules, were significantly (p < 0.05) higher than those of the red lentils. At a loading rate of 4 mm min−1, the force required for initiating seed rupture decreased with an increase in moisture content, for vertical and horizontal orientations (p < 0.05). The scanning electron microscopy observations also revealed that seeds were more flexible in a horizontal orientation.
INTRODUCTION
Legumes are consumed as a good source of protein, especially throughout the Middle East where animal proteins are expensive.[Citation1] Lentils are also an important traditional dietary item in developing countries like Iran. They play an essential role in human nutrition as rich sources of starch, calories, certain minerals, and vitamins. Despite being nutritious, legumes, especially lentils, are underused in many developed countries.[Citation2]
Lentils are an important cool-season legume crop grown extensively in Canada, India, Turkey, USA, and Australia. World lentil production was 4.15 million tons (Mt) in 2005–2006 consisting of 70% red, 25% green, and 5% brown or other colors. Canada is the largest lentil producer (1.3 Mt), followed by India (1.0 Mt). Also, after Turkey, Canada is the second-largest lentil-exporting country (70% of its total lentil production), sending its production to Europe, the Middle East, Africa, South America, North America, and Asia. Canada and the USA are the main producers of green lentils, whereas other countries mainly produce red lentils.[Citation3]
The physical and mechanical characteristics of lentil seeds can be used to predict their processability or to ensure satisfactory quality of the final product by the appropriate design of machines for different unit operations.[Citation4 − Citation8] The behavior of this seed under technological treatments is influenced by its differentiated mechanical resistance.[Citation9] A broad knowledge about both lentils’ mechanical properties and factors affecting seed hardness, such as microstructure and physicochemical characteristics, can be applied to the design of new equipment and processes. Therefore, the aim of this research was to evaluate the mechanical behavior of two varieties of lentil seeds under compression loading (rupture force, deformation at rupture point, and energy used for rupture) by examining the effect of moisture content, variety, and orientation of loading; the research emphasized the seeds’ microstructural and physicochemical attributes.
Table 1 Chemical characteristics of studied varieties of lentil seed
MATERIALS AND METHODS
Sample Preparation
Two varieties of lentil seeds (red and green) were used for all the experiments in this study. gives some details of the chemical compositions of the two lentil seed varieties. The seeds were obtained from a local market during June–July 2010, in a city located in the west of Tehran and kept in cooled bags during transportation to the laboratory. The seeds were cleaned in an air-screen cleaner to remove all foreign materials, such as dust, dirt, and chaff, as well as immature and damaged seeds. The initial moisture content of the seeds, as bought from the market, was determined by drying samples in a hot-air oven set at 105°C (±1°) for 24 h, and was found to be 9.5% w.b. The drying conditions were determined based on preliminary studies and in reference to ASAE standards S352.3.[Citation10] The lentil samples were brought to the desired moisture levels by mixing calculated amounts of distilled water with the lentil samples and sealing each sample in a separate plastic bag. The samples were kept at 5°C (±1°C) in a refrigerator for 7 days to permit the water to distribute uniformly throughout the sample. Before each test, the required quantities of the seed were allowed to reach room temperature.[Citation11] All of the samples’ mechanical properties were investigated at moisture levels of 9.5, 13.2, 16.8, 19.17, and 21.1% w.b. Every test was repeated ten times and the results averaged.
Geometric Characteristics and Density
To determine average seed size, 100 seeds were randomly picked and their three linear dimensions (length, width, and thickness) were measured using a digital Versnier caliper (SV-03 model, Taiwan) with an accuracy of 0.01 mm. The seeds’ arithmetic and geometric mean diameter, sphericity, and surface area were calculated according to Mohsenin.[Citation12] The thousand-seed mass was determined using an electronic balance (Mettler Toledo AT261) to an accuracy of 0.001 g. The true density was determined using the toluene-displacement method.[Citation12] The porosity of the bulk grain was computed from the values of the true density and the bulk density using the relationship given by Mohsenin.[Citation12]
Measurement of Mechanical Properties
The mechanical properties of red and green lentil seeds were measured by a texture-analyzer machine (Universal Testing Machine/SMT-5, Santam Company, Tehran, Iran) equipped with a 50 N load cell and integrator. The measurement accuracy was ±0.001 N in force and 0.001 mm in deformation. The individual grains were loaded between two parallel plates of the machine and compressed until rupture occurred as denoted by a bio-yield point in the force-deformation curve. The bio-yield point was detected by a break in the force-deformation curve. Once the bio-yield point was detected, the loading was stopped. To determine the effect of the loading orientation, the seeds were loaded in two orientations: horizontal and vertical. For the horizontal loading, the major axis and the crease of the grain were normal to the direction of loading, while for vertical loading, the major axis of the grain was parallel to the direction of loading. These tests were carried out at a loading rate of 4 mm min− Citation1 for two loading directions at the moisture contents of 9.5, 13.2, 16.8, 19.17, and 21.1% w.b. Energy absorbed by the sample at rupture was determined by calculating the area under the force-deformation curve by means of a digital planimeter (Model 1250-1, Numonics Corp., Lansdale, PA, USA).[Citation13]
Seed Morphology
Scanning electron microscopy (SEM)
The microstructural characteristics of lentil cotyledon cells were examined to investigate the effect of loading orientation on the deformation value and the force required for rupturing the seeds at a moisture level of 16.8% w.b. For SEM observation, the whole cotyledons were immersed in 20 ml of 4% (w/v) glutaraldehyde prepared in a 0.1 M phosphate buffer, pH 7.1, for 2 h, and washed three times in the buffer alone. After fixation, the cotyledons were fractured by cutting with a stainless-steel razor blade. The samples were post-fixed with 1% (w/v) osmium tetroxide prepared in buffer at 4°C. After the samples were washed with distilled water, they were dehydrated in an ethanol series: 15, 30, 50, and 70% for 10 min each; 85 and 95% for 15 min each, and 100% for 1 h. The dehydrated samples were critical-point-dried using liquid nitrogen, and the dried samples were mounted on the specimen holder. Aluminum cement was applied around the bottom of the cotyledons to provide better specimen conductivity. Finally, the mounted samples were sputtered with a thin layer of gold using a BAL-TEC SCD 005 sputter coater (BAL-TEC AG, Balzers, Liechtenstein). All the specimens were observed under a Philips XL 30 scanning electron microscope (Philips, Eindhoven, Netherlands) at an accelerating voltage of 17.0 Kv.
Starch isolation and purification
The starch granules of red and green lentil seeds were isolated according to the technique introduced by Bechtel et al.[Citation14] A drop of suspension of glycerol-rehydrated starch granules was mixed with iodine on a microscope slide, covered with a cover glass, and examined with a Biolar microscope (Biolar polarizing-interference, PZO, Poland). The starch was purified by the method applied by Li et al.:[Citation15] the crude starch was purified thrice, using 5 ml of 2M NaCl, then 0.2% NaOH and 2% SDS, and finally double-distilled water. The starch was then washed once with acetone to remove the water, air-dried at room temperature and stored at −20°C.
Particle-size analysis
The particle size characteristics of starch samples were evaluated with a laser diffraction particle size analyzer (Malvern Mastersizer 2600C, England) as explained by Li et al.[Citation15] Briefly, about 50 mg of starch was weighed into the 10-ml tubes and suspended with 5 ml of double distilled water. Then, the tubes were vortexed for 10 min and kept at 4°C for 1 h. The tubes were vortexed every 15 min during this time. The starch suspension was transferred into the analyzer's dispersion tank containing double-distilled water, and its size was determined.
Statistical Analysis
The results obtained from all mechanical experiments were subjected to analysis of variance (ANOVA), applying randomized complete block design, using SPSS 13 software (SPSS Inc., USA). The F-test was used to determine significant effects of mechanical treatments, and Duncan's multiple ranges test was used to separate means at a 5% level of significance.
RESULTS AND DISCUSSION
Geometric Characteristics and Density
Table 2 gives the geometrical and gravimetrical properties of red and green lentil seeds at different moisture levels. All properties except bulk and true densities increased with an increase in moisture content from 9.5 to 21.1% w.b. (p < 0.05). The increase in the three axial dimensions is attributed to expansion or swelling as the result of moisture uptake in the intracellular spaces within the seeds. The observations in this study are in agreement with previous related studies. The diameter and thickness of lentil seeds increased linearly with an increase in moisture content.[Citation16] The sphericity of red and green lentil seeds increased from 81.85 to 82.16% and 69.88 to 70.41%, respectively. Similar trends have been reported by Gharibzahedi et al.[Citation17] for castor seeds and Gharibzahedi et al.[Citation18] for black cumin seeds. The thousand-seed mass increased from 51.23 to 51.82 g for red lentil seeds and 65.10 to 66.31 g for green as the moisture content increased from 9.5 to 21.1% w.b. The bulk density and true density determined by Carman[Citation19] and Amin et al.[Citation16] were found to be higher than those of the present study, although this may be due to varietal differences between the lentil samples used in their studies and the current study. Porosity increased from 37.7 to 41.8% for red lentils and 40.6 to 46.4% for green with the increase in moisture content (p < 0.05). The increase in porosity might be attributed to the expansion and swelling of seeds, which may have created more voids between the seeds and increased the bulk volume.
Table 2 Physical properties of two varieties of lentil seeds (G = green; R = red) at different moisture contents
Mechanical Properties
The variance analysis of the data concerning the mechanical properties of lentil seeds showed that the moisture content and loading orientation imposed a significant effect on the deformation values as well as the rupture force and energy (p < 0.01). According to statistical analyses, the effect of the lentil variety on the seeds’ mechanical properties was significant. Moreover, the interaction effects of variety × moisture content, variety × loading orientation and moisture content × variety × loading orientation were not significant on the deformation and required force and energy for rupturing the seeds (p > 0.05). The following section discusses the effects of all mechanical factors on the deformation and the rupture energy and force of lentil seeds.
Effect of Moisture Content
Table 3 compares the means for lentil seeds’ rupture force, deformation and rupture energy for various moisture contents, varieties and loading orientations. The rupture force of both red and green lentil seeds decreased from 138.62 to 95.07 N with an increase in seed moisture content. This may be due to the fact that at a higher moisture content, the seeds became softer and required less force.[Citation5,Citation7,Citation20] Saiedirad et al.[Citation21] reported that as the moisture content increased from 5.7 to 15%, the rupture force decreased from 36.977 to 20.358 N. Our findings were also consistent with the results of Konak et al.,[Citation22] who reported that the highest rupture force of chick pea seeds, 210 N was obtained at a moisture content of 5.2% d.b. It was also stated that the seeds became more sensitive and deformable to cracking at higher moisture contents; hence, they required less force to rupture. Tavakoli et al.[Citation23] studied the effect of moisture content on some mechanical properties of barley grains and reported that as the moisture content increased from 7.34 to 21.58%, the rupture-force values ranged from 161.97 to 93.94 N for vertical orientations, and 75.37 to 50.16 N for horizontal.
Table 3 Mean comparison of the seed mechanical properties for different moisture contents, varieties, and loading orientations
Deformation at seed rupture increased from 0.377 to 0.647 mm as the moisture content of the seed increased (). An increase of the deformation values with an increase in moisture content was also obtained for sunflower seeds[Citation24] and safflower seeds[Citation25] in both the vertical and horizontal positions. Moreover, the energy absorbed at the seed rupture increased from 26.57 to 31.47 mJ with the increase in moisture content from 9.5 to 21.1% w.b. (). Energy absorbed at seed rupture is a function of both force and deformation up to rupture point. At low moisture contents, the seed required high force to be ruptured and experienced low deformation, but at high moisture contents, the rupture force was low and the deformation was high. This suggests that seed rupture energy required during compressive loading increases as the moisture content of the seed increases.[Citation21] This study's findings also agree with those obtained by Khazaei,[Citation26] who reported that the energy absorbed in pea rupture under quasi-statistical loading increases significantly with an increase in seed moisture content. also shows the interaction effects of moisture content × seed orientation and moisture content × variety on the rupture force, rupture energy and deformation of the lentil seeds.
Table 4 Mean comparison of the seed mechanical properties considering interaction effects of moisture content with variety and seed orientation
Effect of Variety
illustrates a significant difference between red and green lentil seeds (p < 0.05). The values of all the mechanical properties for green lentil seeds under loading were always higher than those of red lentil seeds. This fact is probably due to the green lentils’ higher starch and protein amounts and consequent higher levels of protein-carbohydrate complexity (). Scrutinizing the interaction effect of loading orientation and seed variety on mechanical characteristics indicated that the lentil seeds in the horizontal loading direction required more rupture force than those in the vertical loading direction (). shows the rupture force, maximal deformation and rupture energy required for initiating the seed rupture at different moisture contents and seed varieties. As the moisture content increased from 9.5 to 21.1% w.b., the rupture force values ranged from144.6 to 104.35 N for green lentil seeds and 132.65 to 87.6 N for red (). Singh and Goswami[Citation27] similarly reported that the required force to initiate rupture of cumin seed decreased from 50 to 40 N and 31 to 20.3 N with an increase in moisture content from 7 to 13% d.b., for the horizontal and vertical orientations, respectively. shows the energy required for initiating seed rupture for different moisture contents and varieties. The rupture energy values increased from 28.83 to 34.54 mJ for green lentils and 24.3 to 28.4 mJ for red with the increase in moisture content. Saiedirad et al.[Citation21] reported similar results in the case of cumin seeds grown in Iran. The maximum deformations at rupture varied respectively from 0.395 to 0.650 mm for green lentil seeds and 0.360 to 0.645 mm for red as the moisture content increased (). Our research findings agreed with those of Gupta and Das[Citation24] for sunflower seed kernels. In addition, they stated that partial separation of cotyledons under compressive force at higher moisture contents might explain the sharp increase in deformation that occurred before rupture was initiated in the kernels loaded in the vertical direction.
Seed Orientation
Based on the values given in and and , the lentil seeds were more flexible in the horizontal loading orientation, and seeds loaded in a vertical orientation required less energy to rupture than those loaded in the horizontal orientation. This is probably due to the fact that under vertical loading, a smaller contact area between the seed and the compressing plates results in buckling and expansion at high levels of stress in the lentil seed.[Citation21] These results are in good agreement with the findings of other researchers.[Citation20 − Citation21,Citation23,Citation25] However, Gupta and Das[Citation24] found that sunflower seeds are more flexible in the vertical loading direction. It could be related to the differences in the geometry, moisture content and chemical composition among types of seeds. As shown in , the values obtained for the maximum deformation and rupture force and energy in the horizontal orientation were statistically more significant than those for the vertical orientation (p < 0.01). Also, the interaction effect of orientation and moisture content resulted in the highest differences in seed rupture force and deformation under horizontal and vertical orientations at 21.1% moisture (). Baumler et al.[Citation25] studied the effect of moisture content and orientation of loading on the rupture force and the rupture deformation of the safflower hull. However, they found no significant difference in rupture force between the two seed directions. They found that the rupture force of the safflower hull decreased as the moisture content increased, attaining a minimum value at around 11% d.b., followed by an increasing trend with further increases in moisture content.
Figure 2 Interaction effect of moisture content, variety, and seed orientation on (a) rupture force, (b) rupture energy, and (c) deformation of lentil seeds at loading rate of 4 mm min− Citation1 (Red [horizontal: ■; vertical: □]; Green [horizontal: • vertical: ○]).
![Figure 2 Interaction effect of moisture content, variety, and seed orientation on (a) rupture force, (b) rupture energy, and (c) deformation of lentil seeds at loading rate of 4 mm min− Citation1 (Red [horizontal: ■; vertical: □]; Green [horizontal: • vertical: ○]).](/cms/asset/34b47c6c-a8b1-41f8-895c-897dc6f5832e/ljfp_a_642448_o_f0002g.gif)
shows relationships between the studied mechanical properties of two varieties of lentil seed as a function of moisture content along with their coefficient of determination (R Citation2) value are given in . shows the interaction effects of moisture content, variety and loading direction on the maximum deformation and the rupture force and energy of lentil seeds. The highest energy absorbed at seed rupture was as much as 44.55 mJ (for green lentil seeds at 21.1% moisture under horizontal loading) and the lowest was 22.66 mJ (for red lentil seeds at 9.5% moisture under vertical loading). The highest value for rupture force (166.7 N) was obtained for green lentils at the lowest moisture content (9.5% w.b.) under horizontal loading. These data are potentially useful in optimizing harvest, transportation, classification and packaging, and also for providing useful knowledge for industrial processing.
Table 5 The relationships between lentil seeds’ mechanical attributes and moisture content (Mc)
Scanning Electron Microscopy
A scanning electron micrograph showed that the cotyledon cells of green lentils create a regular structure containing ellipsoidal and smooth-surfaced starch granules, within a honeycomb-like structure embedded in the protein network ( and ). In these figures, the cell wall and middle lamella were clearly defined at areas of cell attachment. The exposed starch granules of the two varieties differed both in shape and size. The shape of the starch grain of red lentils appeared to be more elongated than those of green lentils ( and ). Moreover, the red lentils’ starch granules were larger than those of the green lentils (). Volume distribution of starch granules in green lentils was typically bimodal with peak values in the ranges of 0.9–19.5 μm and 20.3–35.1 μm, whereas the starch granules of red lentils had a normal distribution in the range of 4.1–33.9 μm (). Lindeboom et al.[Citation28] stated that starch-granule size may affect the lentil's physicochemical and mechanical properties, such as gelatinization and pasting, enzyme susceptibility, crystallinity, solubility, and crackability.
Figure 3 Scanning electron micrographs of cotyledon cells of green lentil seeds at a moisture content of 16.8% (w.b.): (a, d) without compression; (b, e) compression loading under horizontal orientation; (c, f) compression loading under vertical orientation. Bar = 200 μm in (a–c) and 100 μm in (d–f).
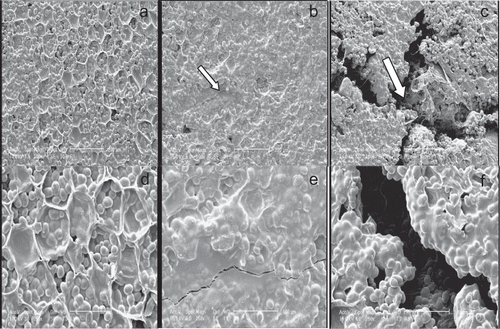
Figure 4 Scanning electron micrographs of cotyledon cells of red lentil seeds at a moisture content of 16.8% (w.b.): (a, d) without compression; (b, e) compression loading under horizontal orientation; (c, f) compression loading under vertical orientation. Bar = 200 μm in (a–c) and 100 μm in (d–f).
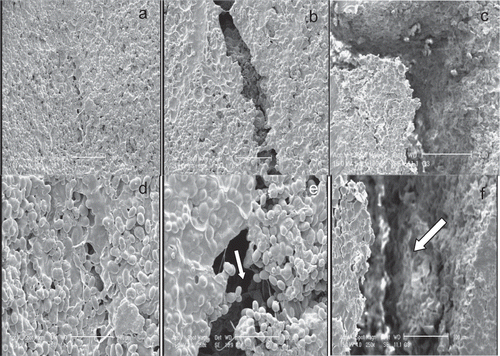
Different parameters could influence seed texture at microstructural level; these could include structure and chemistry of the polymers that make up the cell wall, cell-wall thickness, turgor pressure within cells and strength and nature of cell-to-cell adhesion. At higher structural levels, parameters could include cellular orientation, quantity of intercellular spaces, porosity, etc.[Citation29] Seed susceptibility to damage relative to variety and compression direction was studied in a model experiment: under the function of compressive stress, cell walls were broken and vanished, and starch granules were damaged. However, only endosperm tissues of both sides of cracks were damaged; tissues in other areas stayed intact ( and ).
In the current study, the amount of damage for both directions of compression was strongly related to the variety.[Citation8] SEM micrographs of the loaded samples clearly demonstrated lower fracture magnitude in green lentils than in red lentils for both vertical and horizontal orientations ( and ). As shown in , the higher fracture resistance of green lentil seeds is probably due to their higher levels of starch and protein and higher protein-carbohydrate complexity. Moreover, it can be concluded that average starch size influences mechanical resistance. Glenn et al.[Citation30] had reported that soft wheat had a lower number of small starch granules and a greater mean starch granule area compared with hard-wheat cultivars. Carvalho et al.[Citation31] also found that the mechanical resistance of the extrudates from the smallest particle size was significantly higher than that of the largest particle size.
A lower total internal-damage number for the compression tests along the horizontal axis of ( and ) than along the vertical ( and ) was observed. and give a closer look at the lentils loaded horizontally, and and at lentils loaded vertically. This may be explained by the fact that under horizontal loading direction, the lentils can more successfully maintain their cell-wall structure ( and ), possibly due to more cell-wall thickness and a higher quantity of intercellular spaces, resulting in higher protein-carbohydrate complexity. SEM micrographs distinctly demonstrated the relation of force-deformation curves and textural changes in the seeds during compression loading.
CONCLUSION
The maximum deformation and rupture force and energy required for initiating rupture in green lentils were significantly higher than those for red lentils. Most probably this is due to the higher protein-carbohydrate complexity of green lentil seeds. In support of the hypothesis that wet seeds are capable of higher energy absorption than dry seeds. The mechanical strength of lentil seeds with greater moisture content compared to drier seeds significantly decreased. A reduced contact area between the lentil seed and the loading plate, and probably the occurrence of buckling in the horizontal loading direction, the force required to rupture the seeds is greater with horizontal compressive loading than with vertical. SEM analysis also demonstrated that the lentil seeds are more flexible in the horizontal loading direction, and that the rupture under vertical loading demands less energy than under horizontal loading.
REFERENCES
- Joshi , M. , Adhikari , B. , Panozzo , J. and Aldred , P. 2010 . Water uptake and its impact on the texture of lentils (Lens culinaris) . Journal of Food Engineering , 100 : 61 – 69 .
- Schneider , A.V.C. 2002 . Overview of the market and consumption of pulses in Europe . British Journal of Nutrition , 88 ( 3 ) : 243 – 250 .
- Wang , N. and Daun , K.J. 2006 . Effect of variety and crude protein content on nutrients and anti nutrients in lentils (Lens culinaris) . Food Chemistry , 65 : 35 – 39 .
- Fornal , J. , Sadowska , J. , Ornowski , A. , Jeliñski , T. and Velikanov , L. 2000 . Damage resistance and microstructure of barley kernels . International Agrophysics , 14 : 159 – 166 .
- Kalkan , F. , Kara , M. , Bastaban , S. and Turgut , N. 2011 . Strength and frictional properties of popcorn kernel as affected by moisture content . International Journal of Food Properties , 14 : 1197 – 1207 .
- Ogunsina , B.S. , Adegbenjo , A.O. and Opeyemi , O.O. Compositional . 2010 . mass-volume-area related and mechanical properties of sponge gourd (Luffa aegyptiaca) seeds . International Journal of Food Properties , 13 : 864 – 876 .
- Razavi , S.M.A. and Edalatian , M.R. 2011 . Effect of moisture contents and compression axes on physical and mechanical properties of pistachio kernel. . International Journal of Food Properties , doi: 10.1080/10942912.2010.492541.
- Khodabakhshian , R. , Emadi , B. and Abbaspour Fard, M.H.; Saiedirad, M.H. The effect of variety, size and moisture content of sunflower seed and its kernel on their terminal velocity, drag coefficient and Reynold's number . 2011 . International Journal of Food Properties doi: 10.1080/10942912.2010.483613.
- Nazari Galedar , M , Mohtasebi, , S.S. , Tabatabaeefar, , A. , Jafari, , A. and Fadaei, , H. 2009 . Mechanical behavior of pistachio nut and its kernel under compression loading . Journal of Food Engineering , 95 ( 3 ) : 499 – 504 .
- 1994 . ASAE. ASAE Standards S352.3: Moisture measurement-ungrounded grains and seeds
- Gharibzahedi , S.M.T. , Etemad , V. and Mirarab-Razi , J. 2010 . Fos'hat, M. Study on some engineering attributes of pine nut (Pinus pinea) to the design of processing equipment . Research in Agricultural Engineering , 56 ( 3 ) : 99 – 106 .
- Mohsenin , N.N. 1986 . Physical Properties of Plant and Animal Material , New York : Gordon and Breach Science Publications .
- Altuntas , E. and Erkol , M. 2009 . “ The effects of moisture content, compression speeds, and axes on mechanical properties of walnut cultivars ” . In Food and Bioprocess Technology doi: 10.1007/s11947-009-0283-y.
- Bechtel , D.B. , Zayas , I. , Kaleikau , L. and Pomeranz , Y. 1990 . Size distribution of wheat starch granules during endosperm development . Cereal Chemistry , 67 : 59 – 63 .
- Li , W.Y. , Yan , S.H. , Yin , Y.P. , Li , Y. , Liang , T.B. , Gu , F. , Dai , Z.M. and Wang , Z.L. 2008 . Comparison of starch granule size distribution between hard and soft wheat cultivars in eastern china . Agricultural Sciences in China , 7 ( 8 ) : 907 – 914 .
- Amin , M.N. , Hossain , M.A. and Roy , K.C. 2004 . Effect of moisture content on some physical properties of lentil seeds . Journal of Food Engineering , 65 : 83 – 87 .
- Gharibzahedi , S.M.T. , Mousavi , S.M. and Ghahderijani , M. 2011 . A survey on moisture-dependent physical properties of castor seed (Ricinus communis L.) . Australian Journal of Crop Science , 5 ( 1 ) : 1 – 7 .
- Gharibzahedi , S.M.T. , Mousavi , S.M. and Moayedi , A. 2010 . Taheri Garavand, A.; Alizadeh, S.M. Moisture-dependent engineering properties of black cumin (Nigella Sativa L.) seed . Agricultural Engineering International: The CIGR Ejournal , 12 ( 1 ) : 194 – 202 .
- Carman , K. 1996 . Some physical properties of lentil seeds . Journal of Agricultural Engineering Research , 63 : 87 – 92 .
- Gorji , A. , Rajabipour , A. and Tavakoli , H. 2010 . Fracture resistance of wheat grain as a function of moisture content, loading rate and grain orientation . Australian Journal of Crop Science , 4 ( 6 ) : 448 – 452 .
- Saiedirad , M.H. , Tabatabaeefar , A. , Borghei , A. , Mirsalehi , M. and Badii , F. 2008 . Ghasemi Varnamkhasti, M. Effects of moisture content, seed size, loading rate and seed orientation on force and energy required for fracturing cumin seed (Cuminum cyminum Linn.) under quasi-static loading . Journal of Food Engineering , 86 : 565 – 572 .
- Konak , M. , Carman , K. and Aydin , C. 2002 . Physical properties of chick pea seeds . Biosystems Engineering , 82 ( 1 ) : 73 – 78 .
- Tavakoli , H. , Mohtasebi , S.S. , Rajabipour , A. and Tavakoli , M. 2009 . Effects of moisture content, loading rate, and grain orientation on fracture resistance of barley grain . Research in Agricultural Engineering , 55 ( 3 ) : 85 – 93 .
- Gupta , R.K. and Das , S.K. 2000 . Fracture resistance of sunflower seed and kernel to compressive loading . Journal of Food Engineering , 46 ( 1 ) : 1 – 8 .
- Baumler , E. , Cuniberti , A. , Nolasco , S.M. and Riccobene , I.C. 2006 . Moisture dependent physical and compression properties of safflower seed . Journal of Food Engineering , 72 ( 2 ) : 134 – 140 .
- Khazaei , J. 2002 . “ Determination of force required to pea pod harvesting and mechanical resistance to impact. Ph.D. ” . In Thesis, Faculty of Biosystem Engineering, , Karaj , Iran : University of Tehran .
- Singh , K.K. and Goswami , T.K. 1998 . Mechanical properties of cumin seed under compressive loading . Journal of Food Engineering , 36 ( 3 ) : 311 – 321 .
- Lindeboom , N. , Chang , P.R. and Tyler , R.T. Analytical . 2004 . , biochemical and physicochemical aspects of starch granule size, with emphasis on small granule starches: A review. Starch/Starke , 56 : 89 – 99 .
- Sasikala , V.B. , Ravi , R. and Narasimha , H.V. 2011 . Textural changes of green gram (phaseolus aureus) and horse gram (Dolichos Biflorus) as affected by soaking and cooking . Journal of Texture Studies , 42 : 10 – 19 .
- Glenn , G.M. , Pitts , M.J. , Fleege , L.M. and Andrews , L.C. 1992 . Block-surface staining for differentiation of starch and cell walls in wheat endosperm . Biotechnic and Histochemistry , 67 : 88 – 97 .
- Carvalho , C.W.P. , Takeiti , C.Y. , Onwulata , C.I. and Pordesimo , L.O. 2010 . Relative effect of particle size on the physical properties of corn meal extrudates: Effect of particle size on the extrusion of corn meal . Journal of Food Engineering , 98 : 103 – 109 .