Abstract
The aim of the present study was to enhance thermal stability and functionality of high linoleic corn oil by blending with healthy oils. Oil blends (10% and 20%, w/w) of black cumin oil and coriander oil with corn oil were formulated. Thermal oxidative stability and radical scavenging activity of corn oil and blends stored under oxidative conditions (60°C) for 15 days were studied. By increasing the proportion of black cumin oil and coriander oil in corn oil, levels of polyunsaturated fatty acids decreased, while monounsaturated fatty acids content increased. Progression of oxidation was followed by measuring peroxide value, conjugated dienes, and conjugated trienes. Inverse relationships were noted between peroxide value and oxidative stability at termination of storage. Levels of conjugated dienes and conjugated trienes in corn oil and blends increased with an increase in time. Corn oil:black cumin oil and corn oil:coriander oil blends gave 12–22% and 26–36% inhibition of 1,1-diphenyl-2-picrylhydrazyl radicals, respectively. Oxidative stability of oil blends were better than corn oil, most likely as a consequence of changes in fatty acids and tocopherols’ profile, and minor bioactive lipids found in coriander and black cumin oils.
INTRODUCTION
Oxidation of lipids is an important problem for food industry. This is relevant when the lipidic substrates are composed of unsaturated or polyunsaturated fatty acids (PUFA) that are sensitive to oxidation. Antioxidants’ effectiveness depends on their chemical reactivity (as radical scavengers or metal chelators), interaction with food components, environmental conditions (e.g., pH and concentration), and physical location of the antioxidant in food systems.[Citation1] Oxygen-dependent deterioration of lipids has been recognized as a major problem in the storage of fats and oils. Oxidation imparts undesirable flavours and aromas, compromises the nutritional quality of oils, and leads to the induction of toxic compounds. Lipids involved in the oxidation process contain unsaturated fatty acids, such as oleic, linoleic, linolenic, and long chain PUFA; however, other unsaturated minor compounds, such as sterols, do become oxidized. The rate at which fatty acids are oxidized increases with the degree of unsaturation and decreases with the presence of lipid-soluble antioxidants. The oxidation of edible fats and oils can be controlled by the application of antioxidants, using processing techniques that minimize loss of tocopherols and other antioxidants, inactivation of prooxidant metals and enzymes, exposure to oxygen, heat and light, hydrogenation of PUFA, and the use of an inert gas or vacuum packaging to expel atmospheric oxygen.[Citation2]
During storage and thermal treatment, vegetable oils undergo hydrolysis, oxidation, and polymerization. The deep fried flavours are due to degradation products of linoleic acid and their intensity can be lowered if the food is fried in oil of low linoleic acid content.[Citation3] Nutritional advantages have been recognized for oils rich in oleic and other monounsaturated fatty acids (MUFA) with reduced linoleic acid and saturates contents.[Citation4] Oleic acid (C18:1n-9) is the most abundant MUFA in many edible oils. Compared with PUFA, oleic acid is more resistant toward oxidation at ambient storage and at high temperatures.[Citation5, Citation6] Moreover, diets with high contents of oleic acid are associated with low levels of low-density lipoprotein (LDL) cholesterol in blood plasma and they may reduce the incidence of coronary heart diseases.[Citation7, Citation8]
Corn, sunflower, and soybean oils with high levels of PUFA are the main oils used for cooking and frying. These oils, however, are not quite suitable for frying due to the higher magnitude of oxidation at elevated temperatures.[Citation9] Some high-oleic oils could be used for frying, e.g., canola or olive, as they are quite stable at high temperatures. However, their high cost restricts their usage on a major scale. Therefore, the use of more stable frying oils of comparatively low price would be desirable. To overcome the problem of poor oxidative stability (OS) of traditional oils, ways of reducing the unstable PUFA content and increasing natural antioxidants were sought. One way to improve the OS of these oils is by blending with oils of high-oleic acid contents and high antioxidants’ levels.
Blending of vegetable oils and fats has emerged as an economical way of modifying the physicochemical characteristics of vegetable oils besides enhancement in OS.[Citation9, Citation10] Mariod et al.[Citation11] investigated improvement in the OS of sunflower oil (SFO) by blending with non-conventional Sudanese oils. Fatty acid profile of oils can be improved by blending; hence, the need to hydrogenate unsaturated oils is appreciably decreased, thereby eliminating the chances of formation of harmful trans-fatty acids.
The need for widely usable and easily available bioactive lipids and natural antioxidants continues to grow. Black cumin (Nigella sativa) and coriander (Coriandrum sativum) seed oils have been part of a supplemental diet in many parts of the world and their consumption is also becoming increasingly popular in the non-producer countries. Among newer sources of edible oils, these seed oils are of interest and may play a major role in human nutrition and health because of their special fatty acid composition as well as the presence of valuable amounts of fat-soluble bioactives.[Citation12] Nigella sativa is an annual herbaceous plant belonging to the Ranuculacea family. In addition, Nigella sativa seed components have also been used to prepare functional cosmetic and dietary supplemental products. Studies were conducted on pharmacological properties of the essential oil of black cumin.[Citation13− Citation15] Black cumin seed oil (BCSO) is rich in essential fatty acids as well as bioactive phytosterols (ST) and tocopherols.[Citation16, Citation17] Coriander (Coriandrum sativum L.) is a culinary and medicinal plant from the Umbelliferae family, which contains high levels of petroselinic acid (Δ6-cis-octadecenoic acid, 18:1 n-12) as part of triacylglycerols. Coriander seed oil (CSO) had recently been intensively investigated and the results indicated that crude CSO is a highly promising edible oil with high levels of bioactive lipids.[Citation8]
Mixing different types of vegetable oils can increase the levels of bioactive lipids and natural antioxidants in the blends and give better quality oils, which include tailor-made physicochemical properties as well as improved nutritional value at affordable prices. Oil blends has been a common permitted practice in many countries. Lately, it has been permitted to manufacture and market blended oils containing commonly edible oil mixed with unconventional oil.[Citation8] In the present study, efforts have been made to investigate the effects of blending BCSO and CSO with corn oil (CO) on the OS and radical scavenging activity of high-linoleic CO. No previous studies have yet been conducted on the blending of BCSO and CSO with CO. This report might serve as a milestone toward development of newer blended oils with improved OS and nutritional value.
MATERIAL AND METHODS
Materials
Black cumin and coriander seeds were obtained from a local market (Zagazig, Egypt) and the oil was extracted using n-hexane for 6 h in a Soxhlet extractor. Refined, bleached, and deodorized CO was purchased from a local market (Zagazig, Egypt). Four oil blends were formulated by blending CO with BCSO as well as CSO in proportions of 9:1 and 8:2 (w/w). The oils were thoroughly mixed to form uniform blends at room temperature. Standards used for vitamin E (α-, β-, γ-, and δ-tocopherol) were purchased from Merck (Darmstadt, Germany). 1,1-Diphenyl-2-picrylhydrazyl (DPPH, approximately 90%) was purchased from Sigma (St. Louis, Mo, USA). Galvinoxyl was from Aldrich (Milwaukee, WI, USA). Toluene of HPLC grade was used throughout the antiradical test. All solvents and reagents from various suppliers were of the highest purity needed for each application and used without further purification.
Methods
Analysis of CO and oil blends
Fatty acids and tocopherols of CO and oil blends were analyzed using GLC and HPLC chromatographic techniques according to Ramadan et al.[Citation18]
Accelerated thermal oxidation experiment of CO and oil blends (Shaal oven test)
CO and oil blends were placed in a series of transparent glass bottles having a volume 20 mL each. The bottles were completely filled with CO and oil blends and sealed. No headspace was left in the bottles. The oxidation reaction was accelerated in a forced-draft air oven T6 (Heraeus Instruments GmbH, Hanau, Germany) set at 60 ± 2°C for up to 0, 5, 10, and 15 days. Immediately after the storage period, oil samples were withdrawn for triplicate analyses.
Analytical procedures for monitoring oxidative stability
The progress of the oxidative deterioration of the oil blends during storage was followed by measuring at regular intervals changes in peroxide levels according to the official methods of the American Oil Chemists’ Society.[Citation19] The absorptivity values at 232 and 270 nm were recorded spectrophotometry (Shimadzu UV-260 visible recording spectrophotometer; Kyoto, Japan) following the analytical methods described by IUPAC[Citation20] method. The contents of conjugated diene (CD) and trienes (CT) were expressed as absorptivities of the 1% oil in 2,2,4-trimethylpentane.
Radical scavenging activity (RSA) toward DPPH radical
The RSA of CO and oil blends during the oven test was assayed with DPPH radicals dissolved in toluene according to Ramadan et al.[Citation12] RSA and the presence of hydrogen donors in CO and oil blends during an accelerated oxidation test were examined by reduction of DPPH in toluene. Toluenic solution of DPPH radicals was freshly prepared at a concentration of 10−4 M. The radical, in the absence of antioxidant compounds, was stable for more than 2 h of normal kinetic assay. For evaluation, 10 mg of CO or oil blends sampled during the shaal oven test (in 100 μL toluene at room temperature) were mixed with 390 μL toluenic solution of DPPH radicals and the mixture was vortexed for 20 s at ambient temperature. Against a blank of toluene without DPPH, the decrease in absorption at 515 nm was measured in 1-cm quartz cells after 60 min of mixing using UV-260 visible recording spectrophotometer (Shimadzu, Kyoto, Japan). RSA toward DPPH was estimated from the differences in the absorbance of toluenic DPPH solution with or without sample (control) and the inhibition percent was calculated from the following equation:
Radical scavenging activity toward galvinoxyl radicals
A Miniscope MS 100 Electron Spin Resonance (ESR) spectrometer (Magnettech GmbH; Berlin, Germany) was used throughout the analysis.[Citation21] Experimental conditions were as follows: measurement at room temperature; microwave power, 6 db; centerfield, 3397 G, sweep width 83 G, receiver gain 10, and modulation amplitude 2000 mG. Ten milligrams of oils (in 100 μL toluene) were allowed to react with 100 μL of toluenic solution of galvinoxyl (0.125 mM). The mixture was stirred on a vortex stirrer for 20 s then transferred into a 50-μL micro pipette (Hirschmann Laborgeräte GmbH, Ederstadt, Germany) and the amount of galvinoxyl radical inhibited was measured exactly 60 s after the addition of the galvinoxyl radical solution. The galvinoxyl signal intensities were evaluated by the peak height of signals against a control. A quantitative estimation of the radical concentration was obtained by evaluating the decrease of the ESR signals in arbitrary units after 1 min incubation using KinetikShow 1.06 Software program (Magnettech GmbH; Berlin, Germany). The reproducibility of the measurements was ±5, as usual for kinetic parameters. All work was carried out under subdued light conditions. All the experiments were repeated at least thrice when the variation on any one was routinely less than 5%. All experimental procedures were performed in triplicate and the mean values (± standard deviation) are given.
RESULTS
Fatty Acids and Tocopherols’ Composition of Corn Oil and Oil Blends
Fatty acid profile of BCSO was 13% C16:0, 3.16% C18:0, 24.1% C18:1n-9, 57.3% C18:2n-6, and 2.44% C20:2. Fatty acid composition of CSO was 5.45% C16:0, 1.36% C18:0, 68% C18:1n-12, 8.02% C18:1n-9, 15.9% C18:2n-6, and 0.27% C22:1. The fatty acid profile of BCSO and CSO is in agreement with Ramadan et al.[Citation12] The main fatty acids in the CO and BCSO blends were linoleic, oleic, and palmitic acids with 60.1, 26.0, and 9.46% (CO); 59.8, 25.8, and 9.81% (CO:BCSO, 9:1); and 59.5, 25.6, and 10.1% (CO:BCSO, 8:2), respectively (). Blending of BCSO non-significantly modified the concentration of minor fatty acids in CO:BCSO blends (). Due to blending with CSO, major changes were noted in the contents of C18:1 and C18:2 of blended oils (). Blending with CSO resulted in significant increases from 26.5% to 31.4 and 36.4% in the MUFA contents of CO:CSO blends 9:1 and 8:2, respectively. On the other side, the contents of PUFA were decreased from 60.6% to 56.2 and 51.8%, respectively. The changes in saturated fatty acids (SFA) as a result of blending CO with BCSO or CSO were very small (). It could be noted that the impact of blending CO with BCSO on fatty acid profile was not as marked as that of the blending with CSO.
Figure 1 Levels of SFA, MUFA, and PUFA in percentages (a) and tocopherols in mg/kg (b) in CO and oil blends. (Color figure available online.)
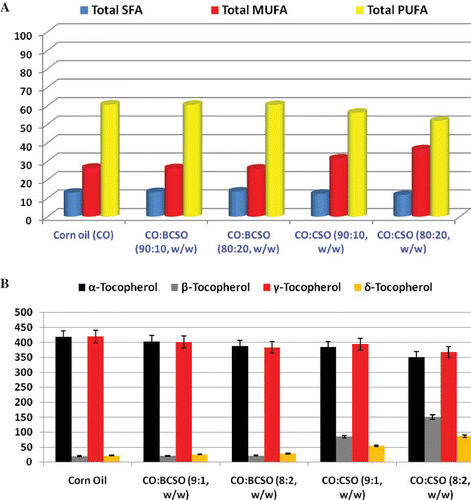
Results of qualitative and quantitative composition of tocopherols are summarized in . Corn oil contained a high amount of total tocopherols (879.1 mg/kg), wherein γ- and α-tocopherols were measured at levels of 419 and 417 mg/kg, respectively. Crude BCSO and CSO were also characterized by high levels of total tocopherol content, which accounted for 590 and 1267 mg/kg oil, respectively (data not shown). All tocopherol isomers were present in BCSO and CSO, wherein α-tocopherol constituted ca. 45% of the total tocopherols in BCSO followed by γ-tocopherol (40%), while β-tocopherol was the main component in CSO (ca. 56% of total tocopherol content). Gamma- and δ-tocopherols were measured at low levels. Oil blends of CSO contained higher levels () of total tocopherols (917–956 mg/kg) than blends containing BCSO (821–850 mg/kg). The OS of the oils might then be affected by their tocopherol profiles. Alfa and γ-tocopherols proved to be the major tocopherols in vegetable oils and fats. Gamma-tocopherol occurred in highest concentrations in camelina, linseed, cold-pressed rapeseed, and corn oil.[Citation22] Alfa-tocopherol is the most efficient antioxidant of the tocopherol isomers, while β-tocopherol has 25–50% of the antioxidative activity of α-tocopherol, and γ-isomer 10–35%. Another study stated that γ-tocopherol is known to serve as a better in vitro antioxidant than α-tocopherol.[Citation2, Citation23] It could be stated that levels of tocopherols detected in BCSO and CSO may contribute to the stability of the oil toward oxidation.
Table 1 Composition of tocopherols (mg/kg) in CO and oil blends
Oxidative Stability of CO and Oil Blends
Peroxide value (PV)
Hydroperoxide is the primary product of lipid oxidation; therefore, determination of PV can be used as an oxidative index during the early stage of lipid oxidation.[Citation24, Citation25] The PV calculated for CO and blends are shown in . The initial PV of CO was 0.7 meq O2/kg oil. Addition of BCSO and CSO to the CO resulted in a marked decline in their PV during storage, thus showing enhancement of the OS of CO. On the basis of PV, the OS of CO and oil blends varied significantly, with the blends enriched with CSO (9:1 and 8:2, w/w) being the most stable. The PV clearly showed that as the storage time increased the OS of the CO and oil blends decreased (). Results show that PV of oil samples (CO and blends) increased with an increase in storage period and followed the order: CO:CSO (8:2, w/w) < CO:CSO (9:1, w/w) < CO:BCSO (8:2, w/w) < CO:BCSO (9:1, w/w) < CO. Both CSO-enriched blends had a much lower PV than that of blends enriched with BCSO over the entire storage period. PV in CO-enriched blends increased at a lower level over 15 days, whereas the peroxides accumulated at relatively higher amounts in BCSO-enriched blends than in CO. The PV after 15 days were 7.5 and 6.8 meq O2/kg for CO blends enriched with CSO in proportion of 9:1 and 8:2 (w/w), respectively. At the end of the storage experiment, PV recorded 9 and 11 meq O2/kg for CO blends enriched with BCSO in proportion of 9:1 and 8:2 (w/w), respectively. At the end of the storage period, CO control had the highest PV (18.5 meq/kg) and was oxidized rapidly.
Ultraviolet absorptivity
Formation of hydroperoxides is coincidental with conjugation of double bonds in PUFA, measured by absorptivity at the UV spectrum.[Citation24] Lipids containing methylene-intrupted dienes or polyenes show a shift in their double bond position during oxidation. The resulting CD exhibit intense absorption at 232 nm, similarly CT absorb at 270 nm. The increase in CD and CT contents is proportional to the uptake of oxygen. The greater the levels of CD and CT in oil, the lower will be the OS.[Citation25]
Absorptivity at 232 nm and 270 nm in CO and oil blends, due to the formation of primary and secondary products of oxidation, showed a pattern similar to that of the PV ( and ). Absorptivity at 232 nm increased gradually with the increase in time, due to the formation of CD (). Formation of aldehydes, ketones (rancid off-flavour compounds), and other oxidation products were followed by an increase in absorptivity at 270 nm (). The variation of absorptivity at 270 nm, due to the formation of CT as well as unsaturated ketones and aldehydes, presented a pattern similar to that of absorptivity at 232 nm. Again, the OS of CO:CSO blends were better during the oven test and UV absorptivity showed this phenomenon. The high content of conjugated oxidative products in CO can be attributed to its high linoleic acid content (60.1% of total fatty acids), which is readily decomposed to form conjugated hydroperoxides. Examination of the results shows that the rate of formation of oxidative products in oils examined, and the ranking order of OS derived using maximum PV levels, was identical to the order obtained using maximum CD and CT values (CO > CO:BCSO (9:1, w/w) > CO:BCSO (8:2, w/w) > CO:CSO (9:1, w/w) > CO:CSO (8:2, w/w)). CO:CSO blends contained the lowest level of conjugated oxidative products and these results are in agreement with the PV.
Radical scavenging activity toward DPPH and galvinoxyl radicals
Research on the application of free radicals to the study of the autoxidation processes included crude vegetable oils and deep-fried oils.[Citation23] The method is also valid for oil fractions with different polarity, such as neutral lipids, glycolipids, and phospholipids.[Citation12] In this study, the ability of CO and oil blends to prevent lipid peroxidation was screened using DPPH and galvinoxyl radicals. From the data presented in and , it can be seen that blending BCSO and CSO with CO increased antiradical action of CO. The impact of BCSO and CSO as additives on CO oxidation was strong and gave 12–22% and 26–36% inhibition of DPPH radicals, respectively (). ESR measurements sldo showed the same pattern and similar profile of deactivating galvinoxyl radicals was obtained during an oven test (). After 15 days, 37% of galvinoxyl radicals were quenched in CO:CSO (8:2, w/w), while the CO:BCSO (8:2, w/w) was able to quench 22%. The stronger RSA of CO:CSO blends compared to CO:BCSO might be due to the higher levels of polar lipids (glycolipids and phospholipids) found in CSO than in BCSO.[Citation12] In addition, the synergism between polar lipids found in crude oils (BCSO and CSO) and tocopherols found in CO as well as different kinetic behaviours of potential antioxidants might be anticipated factors.
DISCUSSION
Oxidative reactions limit the shelf life of fresh and processed foodstuff and are a serious concern in food industry. Synthetic antioxidants, such as butylated hydroxyanisole, butylated hydroxytoluene, propyl gallate, and ter-butylhydroquinone, are very effective in the protection of unsaturated oils and are, therefore, used as potential inhibitors of oxidative reaction. However, growing concern over the safety of synthetic antioxidants has led to an increased interest in exploration of effective and economical natural antioxidants.[Citation26] Moreover, foods rich in natural antioxidants play an essential role in the prevention of cardiovascular diseases and cancer. Antioxidants are often added to food to prevent the radical chain reactions of oxidation and they act by inhibiting the initiation and propagation steps, consequently delaying the oxidation process. The effectiveness of an antioxidant mainly relies on its chemical reactivity but it is also important how it interacts with other food components, their concentration, and especially their physical location in different homogeneous or heterogeneous food systems.
Crude vegetable oils are usually oxidatively more stable than the corresponding refined and processed oils. The fatty acid composition of oil can be an indicator of its OS and nutritional quality. The CO and oil blends have different fatty acid profile. Petrocelinic acid (C18:1n-12) was the fatty acid marker of CSO, while linoleic (C18:2n-6) and oleic (C18:1n-9) were the major fatty acids in BCSO. A slower rate of increment in PV of CO:CSO blends compared with those of CO:BCSO might be attributed to the high amounts of oleic acid (less susceptible to oxidation) and linoleic acids (more prone to oxidation) present in the former and the latter, respectively. The reported rates of oxidation of C18:1 and C18:2 are of the order of 1:12.[Citation2] The results demonstrated that CO blends enriched with CSO and BCSO had a potent OS, and adding BCSO and CSO caused a decrease in peroxide levels during incubation for 15 days.
Mariod et al.[Citation11] investigated the effects of different blends of sunflower oil (SFO) and S. birrea oil on the development of PV during a storage test and reported that the blends of 10% Sclerocarya birrea oil with SFO showed marked improvement in the OS in comparison to SFO. Increase in the amount of S. birrea oil in the blends has been reported to cause a drastic increase in the OS. Similar effects of blending on the improvement of the OS of edible oils were shown by Allam,[Citation27] who studied the OS of SFO blended with nine oils distinguished by their high oleic acid content. Monika et al.[Citation28] reported that the OS of 1:1 (v/v) rapeseed/palm olein blend was improved up to 60% in comparison with rapeseed oil. Frankel and Huang[Citation29] reported that mixtures of canola oil and sunflower oil containing 1.0 and 2.0% linolenate had comparable or better OS than hydrogenated canola oil containing 1% linolenate.
The addition BCSO and CSO to CO increases the OS of different blends when heated in the dark at 60°C. Although different additions protected CO, this protection was more effective for CSO blends, while BCSO exhibited relatively lower protection. Generally, it is accepted that the high degree of unsaturation of oil makes it susceptible to oxidative deterioration. Aside from fatty acid profile, factors, such as oxygen concentration, metal contaminants, lipid hydroxy compounds, enzymes, and light, may also influence the OS of oil.
CONCLUSIONS
As food habits worldwide are based on deep fried and baked foods, oxidative-resistant oils are needed. Conventionally available cooking oils cannot fulfil this requirement; rather, they may cause serious health disorders due to the generation of hazardous oxidation products. This requirement can be conveniently met through the blending process. BCSO and CSO have been part of a supplemental diet in many parts of the world and their consumption is also becoming increasingly popular in the non-producer countries. Yet, these oils rich in bioactive lipids (sterols and tocols) may bring nutraceutical and functional benefits to food systems. At different concentrations of BCSO and CSO, OS of high-linoleic CO was enhanced. Furthermore, blends enriched with BCSO and CSO had strong RSA. The optimal levels of BCSO and CSO enrichment will depend on the actual application. It is anticipated that commercial exploitation of CO:CSO and CO:BCSO blends will soon be realized.
REFERENCES
- Lucas , R. , Comelles , F. , Alcantara , D. , Maldonado , O.S. , Curcuroze , M. , Parra , J.L. and Morales , J.C. 2010 . Surface-active properties of lipophilic antioxidants tyrosol and hydroxytyrosol fatty acid esters: A potential explanation for the nonlinear hypothesis of the antioxidant activity in oil-in-water emulsions . Journal of Agricultural and Food Chemistry , 58 : 8021 – 8026 .
- Miraliakbaru , H. and Shahidi , F. 2008 . Oxidative stability of tree nut oils . Journal of Agricultural and Food Chemistry , 56 : 4751 – 4759 .
- Pokorny , J. 1989 . “ Flavor chemistry of deep fat frying in oil ” . In Flavor Chemistry of Lipid Foods , Edited by: Min , D.B. and Smouse , T.H. 113 – 155 . IL : AOCS: Champaign .
- Nestel , P. , Clifton , P. and Noakes , M. 1994 . Effects of increasing dietary palmoleic acid compared with palmitic and oleic acids on plasma lipids of hypercholesterolemic men . Journal of Lipid Research , 35 : 656 – 662 .
- Warner , K. and Knowlton , S. 1997 . Frying quality and oxidative stability of high-oleic corn oils . Journal of American Oil Chemists Society , 74 : 1317 – 1321 .
- Kolanowski . 2010 . W. Omega-3 LC PUFA contents and oxidative stability of encapsulated fish oil dietary supplements . International Journal of Food Properties , 13 : 498 – 511 .
- Noakes , M. , Nestel , P.J. and Clifton , P.M. 1996 . Commercial frying fats and plasma lipid-lowering potential . Australian Journal of Nutrition and Dietics , 53 : 25 – 30 .
- Ramadan , M.F. , Amer , M.M.A. and Coriander , Awad, A. 2008 . (Coriandrum sativum L.) seed oil improves plasma lipid profile in rats fed diet containing cholesterol . European Food Research and Technology , 227 : 1173 – 1182 .
- Anwar , F. , Hussain , A.I. , Iqbal , S. and Bhanger , M.I. 2007 . Enhancement of the oxidative stability of some vegetable oils by blending with Moringa oleifera oil . Food Chemistry , 103 : 1181 – 1191 .
- Premavalla , K.S. , Madhura , C.V. and Arya , S.S. 1998 . Storage and thermal stability of refined cottonseed oil: Mustered blend . Journal of Food Science and Technology , 35 : 530 – 532 .
- Mariod , A. , Matthaus , B. , Eichner , K. and Hussain , I.H. 2005 . Improving the oxidative stability of sunflower oil by blending with Sclerocarya birrea oil and Aspongopus viduatus oils . Journal of Food Lipids , 12 : 150 – 158 .
- Ramadan , M.F. , Kroh , L.W. and Moersel , J.-T. 2003 . Radical scavenging activity of black cumin (Nigella sativa L.), coriander (Coriandrum sativum L.) and niger (Guizotia abyssinica Cass.) crude seed oils and oil fractions . Journal of Agricultural and Food Chemistry , 51 : 6961 – 6969 .
- Ramadan , M.F. 2007 . Nutritional value, functional properties and nutraceutical applications of black cumin (Nigella sativa L): An overview . International Journal of Food Science and Technology , 42 : 1208 – 1218 .
- Luther , M. , Parry , J. , Moore , J. , Meng , J. , Zhang , Y. , Cheng , Z. and Yu , L. 2007 . Inhibitory effect of chardonnay and black raspberry seed extracts on lipid oxidation in fish oil and their radical scavenging and antimicrobial properties . Food Chemistry , 104 : 1065 – 1073 .
- Lutterodt , H. , Luther , M. , Slavin , M. , Yin , J.-J. , Parry , J. , Gao , J.-M. and Yu , L. 2010 . Fatty acid profile, thymoquinone content, oxidative stability, and antioxidant properties of cold-pressed black cumin seed oils . LWT–Food Science and Technology , 43 : 1409 – 1413 .
- Ramadan , M.F. and Moersel , J.-T. 2002 . Characterization of phospholipid composition of black cumin (Nigella sative L.) . seed oil. Nahrung/Food , 46 : 240 – 244 .
- Gupta , M. 2010 . Pharmacological properties and traditional therapeutic uses of important Indian spices: A review . International Journal of Food Properties , 13 : 1092 – 1116 .
- Ramadan , M.F. , Zayed , R. and El-Shamy , H. 2007 . Screening of bioactive lipids and radical scavenging potential of some solanaceae plants . Food Chemistry , 103 : 885 – 890 .
- AOCS . 1995 . Official Methods and Recommended Practices of the American Oil Chemists’ Society , 4th , Champaign , IL : AOCS .
- IUPAC . 1979 . Standard Methods for the Analysis of Oils and Fats and Derivatives , Toronto : Pergamon Press .
- Ramadan , M.F. , Kinni , S.G. , Seshagiri , M. and Mörsel , J.-T. 2010 . Fat-soluble bioactives, fatty acid profile and radical scavenging activity of Semecarpus anacardium seed oil . Journal of American Oil Chemists Society , 87 : 885 – 894 .
- Schwartz , H. , Ollilainen , V. , Piironen , V. and Lampi , A.-M. 2008 . Tocopherol, tocotrienol and plant sterol contents of vegetable oils and industrial fats . Journal of Food Composition and Analysis , 21 : 152 – 161 .
- Ramadan , M.F. , Sharanabasappa , G. , Seetharam , Y.N. , Seshagiri , M. and Moersel , J.-T. 2006 . Profile and levels of fatty acids and bioactive constituents in mahua butter from fruit-seeds of Buttercup tree [Madhuca longifolia (Koenig)] . European Food Research and Technology , 222 : 710 – 718 .
- Ramadan , M.F. and Moersel , J.-T. 2004 . Oxidative stability of black cumin (Nigella sativa L.), coriander (Coriandrum sativum L.) and niger (Guizotia abyssinica Cass.) upon stripping . European Journal of Lipid Sciences and Technology , 106 : 35 – 43 .
- Mohdaly , A.A.A. , Sarhan , M.A. , Mahmoud , A. , Ramadan , M.F. and Smetanska , I. 2010 . Antioxidant efficacy of potato peels and sugar beet pulp extracts in vegetable oils protection . Food Chemistry , 123 : 1019 – 1026 .
- Iqbal , S. , Bhanger , M.I. and Anwar , F. 2005 . Antioxidant properties and components of some commercially available varieties of rice bran in Pakistan . Food Chemistry , 93 : 265 – 272 .
- Allam , S.H. 2001 . Utilization of some untraditional sources of high oleic acid oils for improving vegetable oils stability . Rivista Italiana Delle Sostanze Grasse , 78 : 337 – 341 .
- Monika , H. , Franciszek , S. , Stanislaw , Z. and Stanislaw , B. 2002 . Frying performance of rapeseed–palm oil blends . Polish Journal of Food Nutrition Science , 11 : 65 – 71 .
- Frankel , E.N. and Huang , S.W. 1994 . Improving the oxidative stability of polyunsaturated vegetable oils by blending with high-oleic sunflower oil . Journal of American Oil Chemists Society , 71 : 255 – 259 .