Abstract
Low molecular weight carbohydrates including sugar alcohols and mono-, di- and oligosaccharides, in particular fructooligosaccharides and raffinose-family oligosaccharides, were determined in 32 fruits and 41 vegetables. Vegetables generally contained less monosaccharides than fruits. Sorbitol was detected in 18 fruits, xylitol in 15, while mannitol was found only in watermelon. On the other hand, sorbitol was found in 12, xylitol in 16, and mannitol in 14 vegetables. Cluster analysis was applied to categorized fruit and vegetables according to their content. Blueberry, pear, watermelon, and nectarine from the fruits; and garlic, spring garlic, leek, white onion, and scallion from the vegetables formed statistically significant clusters reach in oligosaccharides. Most fruits contained low amounts of fructooligosaccharides. The highest content of fructooligosaccharides was found in nectarine (0.89 ± 0.031 g/100 g fresh weight). The vegetable with the highest quantity of fructooligosaccharides was scallion (3.32 ± 0.108 g/100 g fresh weight). White onion (2.24 ± 0.092 g/100 g fresh weight) and scallion (4.10 ± 0.147 g/100 g fresh weight) were found to have relatively high fractions of fructooligosaccharides and raffinose-family oligosaccharides, while Jerusalem artichoke, chicory root, and garlic distinguished themselves by the highest fructan levels, 8.99 ± 0.238 g/100 g fresh weight, 8.40 ± 0.186 g/100 g fresh weight, and 7.51 ± 0.209 g/100 g fresh weight, respectively. These foods could be exploited as potential sources for commercial extractions of prebiotics which can be used as ingredients for functional food products.
INTRODUCTION
Foods are no longer judged only in terms of taste and immediate nutritional needs, but also in terms of their ability to improve the health and well-being of consumers. Since the late 1990s, scientists have shown great interest in the health properties of non-digestible oligosaccharides (NDO) as prebiotic compounds.[Citation1] Many scientific papers have been published describing the effect of prebiotics on human health. Prebiotics have been claimed to improve some physiological functions in humans such as relieving constipation,[Citation2] decreasing the risk of osteoporosis by increasing mineral absorption, especially of calcium,[Citation3] and atherosclerosis by lowering the synthesis of triglycerides and reducing plasma cholesterol concentrations.[Citation4] The effective intake level of prebiotic for reducing the risks of osteoporosis and atherosclerosis was found to be between 8–10 g per day[Citation5] and for relieving constipation was found to be 15–20 g per day.[Citation6]
Low molecular weight carbohydrates (LMWC) are regularly applied in human nutrition as part of the daily diet since they are widely present in fruits and vegetables. Of major importance are sugar polyols and sugars including mono-, di- and NDO, especially fructooligosaccharides (FOS), including 1-kestose (GF2), nystose (GF3) and 1F-β fructofuranosylnystose (GF4), as well as raffinose-family oligosaccharides (RFO), raffinose, and stachyose. Because of several scientific assertions of benefits of these carbohydrates with a “bioactive” or “functional” action in intestinal health, the food industry has increased the inclusion of NDO in their products.[Citation7–9] However, the consumption of natural sources of NDO, such as fruits and vegetables, has advantages for its lower price in comparison to industrialized products. Moreover, fruits and vegetables offer intake of different quantities of NDO combined with other nutrients such as bioactive compounds, vitamins, and minerals.[Citation10]
Nutrient composition of fruits and vegetables is very complex and difficult to assess. Levels of plant metabolites are strongly affected by genetic and environmental factors, as well as transportation and storage conditions.[Citation11] Growth factors such as light, temperature, humidity, type of soil, application of fertilizers, damage caused by microorganisms and insects, stress induced by UV radiation, heavy metals, and pesticides all alter metabolite composition of plants.[Citation12] Before fruits and vegetables appear on a supermarket shelf they have been handled by plant growers, transporters, packagers, storehouse operators, distributors, and/or processors. The chemical and physical changes that occur in fruits and vegetables during these stages can lead to loss of potentially beneficial components.[Citation13,Citation14]
Carbohydrate content (including mono-, di- and oligosaccharides) in food composition tables is usually calculated by difference. There are scarcely published data on oligosaccharide contents in foods, and even less on oligosaccharide consumption of any population in the world.[Citation15,Citation16] For the fruits and vegetables grown in Macedonia, there is scarce information on the different carbohydrate fractions. A need for such information is of particular importance because of the role of the fruits and vegetables in the diet and the fact that these products are often present in the European markets. Quantifying each individual carbohydrate and evaluating foods on case-by-case basis is the current tendency of worldwide databases.[Citation17,Citation18] Therefore, the objective of the present study was to provide detailed information on carbohydrate levels with special emphasis on prebiotic oligosaccharides in a wide range of fruits and vegetables. NDO (FOS and RFO) as well as sugars and sugar alcohols were quantified. Such information may facilitate the selection of foods as sources for commercial extractions of prebiotic oligosaccharides and creation of a food composition database that will be valuable in designing dietary strategies.
MATERIALS AND METHODS
Samples and Sample Preparation
The selected foods for analysis were 32 fruits and 41 vegetables grown and commonly consumed in Macedonia. Fresh food samples (fruits and vegetables) were collected from local green markets (apple Golden Delicious, cherry, nectarine, pomegranate, quince, watermelon, cabbage common and red, cauliflower, eggplant, fennel bulb and leaves, kohlrabi, lettuce green, parsnip) and grocery stores (plum Ciruela, artichoke Jerusalem, brussel sprouts, broccoli, chard, daikon, kale, lettuce red, mushroom button, okra, rocket, tomato cherry) or directly from producers (apple Idared and Petrovka, apricot, blackberry, blueberry, currant black and red, fig common and wild green, grape red Vranec and Smederevka, medlar, mulberry black and white, melon Polidor, peach yellow-green, pear, plum red, plum cherry, pumpkin, raspberry, sour cherry, strawberry woodland, beans yellow, beetroot, carrot, celery bulb and leaves, chicory, dandelion bulb, garlic, spring garlic, leek, mushroom Lisicarka and oyster, onion white, peas, pepper red, radish, scallion, tomato common and strawberry, zucchini common and green) in the period from April 2010 to March 2011. The food samples were transferred to the laboratory and analyzed immediately. Approximately 500 g of each sample was chosen at random. The edible part was cut into small pieces (5–15 mm) and dried at 50°C in a vacuum oven (Heraeus Instruments vacutherm VT 6025, Germany) over period of 12 to 24 h, until constant dry mass was reached. Dried samples were crushed with a laboratory grinder to a particle size less than 1 mm before extraction. Classification of fruits and vegetables was made according to the consumer's perception, not always following the botanical classification; e.g., watermelon, although botanically belongs to the group of vegetables, was classified as fruit.
Dry Matter Determination
Approximately 3–5 g of each fresh tissue, chopped into small pieces (around five mm) were weighed in triplicate from each sample and dried at 105°C until constant mass was reached. Moisture content was calculated by difference between fresh and dried mass of the samples.
Extraction of LMWC
LMWC were extracted according to Espinosa-Martos et al.[Citation19] The samples (400 mg dried powder) were extracted with ethanol (85% v/v; 40 mL). Extractions were performed in screw-capped tubes, at 50°C in a water bath with constant shaking for 1 hour. After cooling at room temperature, the samples were centrifuged at 3000x g for 15 min. 10 mL of supernatants were evaporated in a vacuum rotary evaporator at 50°C until the samples were completely dried. The extracts were re-dissolved in deionised water (1.5 mL) and passed through 0.45-μm filters (Econofilter, Agilent Technologies, USA) just before high-performance liquid chromatography (HPLC) analysis. The experiments were performed three times. The analysis of LMWC, from the extraction till HPLC determination was carried out continuously.
Determination of LMWC by HPLC
Twenty microliters of LMWC extracts were filtered through 0.45-μm filters and injected into Agilent 1200 HPLC (USA) fitted with Zorbax carbohydrate analysis column (4.6 × 150 mm, 5 μ m particle size), Zorbax NH2 guard column (4.6 × 12.5 mm; Agilent, USA) and refractive index (RI) detector. The mobile phase was 75:25 (v/v) acetonitrile/water and the flow rate was 1.4 mL/min. The column temperature was kept at 30°C. Appropriate dilutions of a solution containing each of the carbohydrates, glucose, fructose, sucrose, sorbitol, mannitol, xylitol, 1-kestose, nystose, 1F-β fructofuranosylnystose, raffinose, and stachyose, all purchased from Sigma-Aldrich, HPLC grade, were used as calibration standards. A chromatographic profile of an analyzed sample is shown in .
Determination of Total Water-Soluble Carbohydrates (WSC) and Free Reducing Sugars
For the determination of WSC present in fruits and vegetables, extraction was performed in screw-capped tubes mixing 1 g dried powder sample in 10 ml hot water. The tubes were placed in a water bath with constant shaking at 85°C for 90 min. The suspension was then centrifuged at 4000x g for 25 min. Total WSC were determined colourimetrically using phenol-sulphuric method according to procedure of Dubois. The assay was set up by preparing standard glucose solutions in the range 10–100 μg/mL. One mL of the sample, 1 mL of 5% phenol solution and 5 mL of 96% sulphuric acid were transferred to test tubes giving a final volume of 7 mL. A blank solution was prepared by substituting sample solution for 1 mL of distilled water. It was important to mix the solution thoroughly before incubating all test tubes in water bath at 30°C for 20 min. The absorbance of yellow-color thus developed was measured at 490 nm using a spectrophotometer (Varian, Cary 50 Scan). The amount of carbohydrates present was determined from the calibration curve.
The amount of free reducing sugars present in WSC extracts was determined by 3,5-dinitrosalycilic acid (DNS) reagent according to procedure of Miller. 1 mL of sample was mixed with 3 mL of DNS reagent. The mixture was shaken and then heated for 5 min at 100°C. The reaction was stopped by immersion in ice for 5 min, and then the free sugars were obtained comparing the absorbance of sample at 540 nm against a standard curve of glucose (0.1–0.5 mg/mL). The fructan content was calculated by the difference between the total WSC content and the free reducing sugars according to Arrizon et al.[Citation20]
Thin Layer Chromatographic Identification
For identifying the degree of polymerization (DP) of the extracted WSC thin layer chromatography (TLC) was carried out. 1 μL of a sample was applied on a silica gel 60 F254 plates (TLC aluminium sheets 20 × 20 cm, Merck, Germany). The carbohydrates were separated twice with a solvent system acetonitrile/water (85:15 by volume) at room temperature. Sugar spots were visualized by spraying the plates with sulphuric acid/phenol reagent followed by heating at 120°C for 5 min.
Data Analysis
All experiments were performed in triplicates. The results were expressed as g per 100 g fresh weight (FW), and represents mean values ± standard deviation (n = 3). Descriptive statistics and one-way analysis of variance (ANOVA) was performed on the parameters to evaluate significant differences among the samples at 95% confidence interval (p ≤ 0.05) according to Tukey's test using Minitab 15 statistical software. The data obtained from the analysis of the composition of the foods were analyzed by means of multivariate analysis employing hierarchical cluster analysis. The method used was Ward's method. The distances between samples were calculated using square Euclidean distances.[Citation21]
RESULTS AND DISCUSSION
Identification and quantification of the sugars, sugar alcohols, and oligosaccharides in fruits and vegetables is a challenging area as these foods contain a complex mixture of carbohydrates with a varying chain length (DP) from 2 to 60 units. This study provides comprehensive information on the content of oligosaccharides in 32 fruits and 41 vegetables.
The contents of sugars and sugar alcohols in 32 fruits are shown in . All fruits contained fructose and glucose. Fructose concentration was the highest in grapes, 8.13 ± 0.301 g/100 g FW for red grape Vranec and 7.58 ± 0.273 g/100 g FW for white grape Smederevka. Glucose was present in significantly high concentration in pomegranate, red grape Vranec, white grape Smederevka, blueberry, and sour cherry. Sucrose was found in all fruit samples in the range from 0.31 ± 0.024 to 6.48 ± 0.392 g/100 g FW, except in blackberry and medlar, where no sucrose was detected.
According to the results in , sorbitol was detected in 18 fruits, ranging from 0.08 ± 0.007 g/100 g FW in pomegranate to 3.15 ± 0.169 g/100 g FW in blackberry. Mannitol was found only in watermelon, 0.12 ± 0.011 g/100 g FW. Xylitol was detected in 15 fruits, with the significantly highest concentrations found in strawberry, 0.32 ± 0.031 g/100 g FW followed by xylitol concentration in nectarine 0.28 ± 0.026 g/100 g FW.
The sugar content of 41 commonly grown and consumed vegetables are shown in . Vegetables generally contained less monosaccharides than fruits (). In the most of the vegetables, the total amount of sugars (fructose, glucose, and sucrose) was found to be less than 4 g/100 g FW. Eggplant, Jerusalem artichoke, common cabbage, red pepper, tomato, strawberry, and carrot contained total sugars above 5 g/100 g FW, with carrot containing the highest concentration of 8.12 ± 0.363 g/100 g FW.
Table 1 Sugar and sugar alcohol content in selected fruits.Footnote*
Table 2 Sugar and sugar alcohol content in selected vegetables.Footnote*
As can be seen from , sorbitol, mannitol, and xylitol were found in 23 vegetables. Green lettuce, yellow beans, fennel bulb, fennel leaves, carrot, and brussel sprouts are among those containing the lowest level of polyols. The significantly highest concentration of xylitol was detected in dandelion bulb (0.81 ± 0.042 g/100 g FW), of
mannitol in celery bulb (2.39 ± 0.116 g/100 g FW), and of sorbitol in rucola (0.28 ± 0.026 g/100 g FW) and mushroom Lisicarka (0.26 ± 0.022 g/100 g FW). Mannitol was found in a number of vegetables (13), while except in the watermelon, it was not present in fruits. Sugar alcohols may affect laxation and contribute to gastrointestinal effects, including abdominal discomfort, flatus, and diarrhea, especially at higher or excessive intakes.[Citation22] Therefore it is important to quantify the levels of these polyols in fruits and vegetables included in daily diet. The data in this study suggest that individuals with gastrointestinal disorder may limit the consumption of blackberry, pear, and nectarine (), as well as of celery bulb, cauliflower, and mushroom button (). On the other hand, others suffering from constipation or searching for presumed health benefits may increase their level.
Table 3 Oligosaccharides content separated via HPLC in selected fruits.Footnote*
The content of individual fractions of FOS, such as 1-kestose (GF2); nystose (GF3); and 1F-β-fructofuranosylnystose (GF4) and raffinose family oligosaccharides (RFO), raffinose and stachyose are presented in . Most fruits contained low amounts of total FOS. The content of total oligosaccharides in a descending order was nectarine > watermelon > pear > raspberry > blueberry (). Fruits with the highest amount of 1-kestose included raspberry 0.32 ± 0.009, watermelon 0.29 ± 0.009 and cherry 0.22 ± 0.011 g/100 g FW. Nystose was present in 18 fruit samples ranging from 0.08 ± 0.007 g/100 g FW in Ciruela plum to 0.65 ± 0.015 g/100 g FW in nectarine. 1F-β-fructofuranosylnystose was present only in five fruits with the highest amount of 0.08 ± 0.007 g/100 g FW in watermelon. Apricot and melons were the only fruits where raffinose and stachyose were detected in traces (between 0.01–0.05 g/100 g FW).
The level of FOS in the nectarine (0.89 ± 0.031 g/100 g FW) was found higher than that reported by Muir et al.[Citation23] (0.59 g/100 g FW). On the other hand, in the peach there has not been any FOS found, while Muir et al.[Citation23] reported their presence in traces, and Campbell et al.[Citation24] found 0.4 g/100 g. No published data on oligosaccharides content in Petrovka apple, black and red currant, common and wild fig, red grape Vranec, white grape Smederevka, medlar, black and white mulberry, cherry plum, pomegranate, quince, and strawberry woodland are available for comparison. These findings on the LMWC amounts can be used for development of a national fruit database.
The contents of individual fractions of FOS and RFO in vegetables are presented in . It is known that FOS are mostly produced in the roots, tubers, and fruits of plants belonging to Compositae[Citation25] and Amaryllidadeae families.[Citation26] Many samples in the present study were members of these plant families. Jerusalem artichoke and chicory are Compositae species, whereas onions, scallion, garlic, spring garlic, and leek are members of the Allium species of the Amaryllidadeae family. The content of FOS in a descending order was scallion > onion > garlic > leek > spring garlic (). A medium level of FOS (0.51 ± 0.019 to 0.84 ± 0.023 g/100 g FW), mainly nystose, was found in kale, fennel bulb, dandelion, common and red cabbage, brussel sprouts, broccoli, and Jerusalem artichoke. Raffinose was found in six vegetables in a range from 0.11 ± 0.009 g/100 g FW in kohlrabi to 0.78 ± 0.039 g/100 g FW in scallion, while stachyose was found in five vegetables in a range from 0.11 ± 0.006 g/100 g FW in broccoli to 0.78 ± 0.029 g/100 g FW in Jerusalem artichoke.
Table 4 Oligosaccharides content separated via HPLC in selected vegetables.Footnote*
Cluster analysis was used to identify the similar groups according to the oligosaccharides content within the fruit and vegetable samples. As can be seen from , the first division of the fruit samples is made into two clusters A and B. Blueberry, pear, watermelon, and nectarine which belong to cluster B are distinguished with the significantly highest concentration of total oligosaccharides as well as of GF3. Cluster A splits into
two subclusters C and D. Subcluster C contains fruits with low concentration of total oligosaccharides which stem only from GF2 and GF3. Subcluster D is composed of nine fruits characterized with medium concentration of total oligosaccharides with GF2 being a predominant component.
The cluster analysis of 41 vegetables renders a dendrogram that groups the samples in two main clusters A and B (). Cluster B comprises of five vegetables (garlic, garlic spring, leek, onion white, and scallion) with the highest total oligosaccharides. Scallion stands out as a separate subgroup due to its highest total oligosaccharides concentration of 4.10 g/100 g FW. Cluster A is divided in two subclusters C and D. Subcluster C contains vegetables with medium concentration of FOS. Subcluster D is further broken down into two groups G and H. Vegetables without oligosaccharides belong to the group G, while vegetables with low level of total oligosaccharides and varying levels of GF2 and GF3 are included in group H.
In a comparison has been made between data derived in a current study and those published elsewhere for a selection of five fruits and eight vegetables. Comparing the levels of FOS in garlic, it is evident that data reported by Campbell et al.[Citation24] and Muir et al.[Citation23] were lower than those found in this study; 0.39 and 0.92 g/100 g FW compared to 1.20 ± 0.059 g/100 g FW. In contrast to all these reports, Van Loo et al.[Citation27] found much higher level ranging from 3.6 to 6.4 g/100 g FW. Similar observation was noted for leek and white onion. There might be several reasons for this type of discrepancy as many factors affect oligosaccharide levels in foods including food variety, maturity, seasonal variation, climate, extraction procedure, storage time, and storage temperature as well as analytical method for oligosaccharides determination.[Citation28,Citation29] In the present study, we attempted to minimize the impact of many of these variables and to obtain a more “representative” food sample by collecting fruits and vegetables directly from producers.
Table 5 A survey of oligosaccharides content in selected fruits and vegetables
The selected fruits and vegetables presented in were analysed not only for FOS and RFO (oligofructans with low DP), but also for their fructan content (). The highest amounts of fructans were found in Jerusalem artichoke (8.99 ± 0.238 g/100 g FW), chicory root (8.40 ± 0.186 g/100 g FW), and garlic (7.51 ± 0.209 g/100 g FW). The white onion and scallion contained the lowest concentration of fructans (1.81 ± 0.073 and 2.05 ± 0.116 g/100 g FW), while the content of oligofructans was found to be the highest (2.24 ± 0.092 and 4.10 ± 0.147 g/100 g FW.
Figure 4 The content of fructans in selected fruits and vegetables. Values (mean ±SD) for fructans content followed by the different letters are significantly different (p < 0.05).
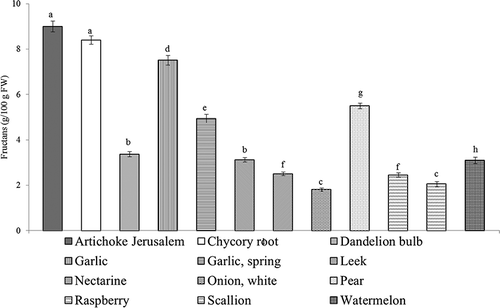
The individual components of WSC present in Jerusalem artichoke, garlic, spring garlic, and leek were separated by TLC (). The elution on the TLC plates corresponded to the amount of fructans quantified. Monosaccharides, fructose, and glucose were eluted first followed by sucrose, and then by components with higher DP. A great part of the spotted material of the Jerusalem artichoke stayed at the beginning, evidently showing that these fructans were with the highest DP. The extracted fructans of garlic, spring garlic, and leek moved from the origin indicating that these vegetables contained fructans with lower chain length than those of Jerusalem artichoke.
CONCLUSION
The present study provided detailed information on LMWC level in a wide range of fruits and vegetables and considerably expanded the understanding of food composition. The foods were evaluated on a case-by-case basis rather than developing generalizations on the relative proportions of NDO fractions according to food groups. These results are a good basis for a development of food composition database on LMWC content and may facilitate proper selection of fruits and vegetables in everyday diet. White onion and scallion were found to have fairly high proportions of FOS and RFO, while Jerusalem artichoke, chicory root, and garlic distinguished themselves by the highest fructan fractions. These foods could be exploited as potential sources for commercial extractions of prebiotics intended for development of new functional food products.
ACKNOWLEDGMENT
The authors acknowledge the financial support of the Macedonian Ministry of Education and Science.
REFERENCES
- Wang , Y. 2009 . Prebiotics: Present and future in food science and technology . Food Research International , 42 : 8 – 12 .
- Roberfroid , M.B. 1993 . Dietary fiber, inulin and oligofructose: A review comparing their physiological effects . Critical Reviews in Food Science , 33 : 103 – 148 .
- Van den Heuvel , E. , Muys , T. , Van Dokkum , W. and Schaafsma , G. 1999 . Oligofructose stimulates calcium absorption in adolescents . American Journal of Clinical Nutrition , 69 : 544 – 548 .
- Davidson , M.H. and Maki , K.C. 1999 . Effects of dietary inulin on serum lipids . Journal of Nutrition , 129 : 1474 – 1477 .
- Abrams , S.A. , Griffin , I.J. , Hawthorne , K.M. , Liang , L. , Gunn , S.K. and Darlington , G. 2005 . A combination of prebiotic short- and long-chain inulin-type fructans enhances calcium absorption and bone mineralization in young adolescents . American Journal of Clinical Nutrition , 82 : 471 – 476 .
- Gibson , G.R. , Beatty , E.R. , Wang , X. and Cummings , J.H. 1995 . Selective stimulation of bifidobacteria in the human colon by oligofructose and inulin . Gastroenterology , 108 : 975 – 982 .
- Mussatto , S.I. and Mancilha , I.M. 2007 . Non-digestible oligosaccharides: A review . Carbohydrate Polymers , 68 : 587 – 507 .
- Bengmark , S. 2010 . Pre-, pro-, synbiotics and human health . Food Technology and Biotechnology , 48 : 464 – 475 .
- Toress , D.P.M. , Goncalves , M.P.F. , Teixeira , J.A. and Rodriques , L.R. 2010 . Galacto-oligosaccharides: Production, properties, application and significance as prebiotics . Comprehensive Review in Food Science and Food Safety , 9 : 438 – 454 .
- Lattanzio , V. , Kroon , P.A. , Linsalata , V. and Cardinali , A. 2009 . Globe artichoke: A functional food and source of nutraceutical ingredients . Journal of Functional Food , 1 : 131 – 144 .
- Mangaraj , S. and Goswami , T.K. 2011 . Measurement and modeling of respiration rate of guava (CV. Baruipur) for modified atmosphere packaging . International Journal of Food Properties , 14 : 609 – 628 .
- Orcutt , D.M. and Nilsen , E.T. 2000 . The Physiology of the Plants Under Stress: Soil and Biotic Factor , New York , , USA : John Wiley & Sons .
- MacEvily , C. and Peltola , K. 2003 . “ The effect of agronomy storage processing and cooking on bioactive substances in food ” . In Plants: Diet and Health, The Report of a British Nutrition Foundation Task Force , 226 – 239 . Oxford , , UK : Blackwell Science Ltd .
- Obulesu , M. and Bhattacharya , S. 2011 . Color changes of tamarind (Tamarindus indica L.) pulp during fruit development, ripening, and storage . International Journal of Food Properties , 14 : 538 – 549 .
- Menezes , E.W. , Giuntini , E.B. , Dan , M.C.T. and Lajalo , F.M. 2009 . New information on carbohydrates in the Brazilian food composition database . Journal of Food Composition and Analyses , 22 : 446 – 452 .
- Trumbo , P. and Shimakawa , T. U.S. 2009 . Food and Drug Administration on modernization of the nutrition and supplements facts labels . Journal of Food Composition and Analyses , 22 : 13 – 18 .
- Greenfield , H. and Southgate , D.A.T. 2003 . Food Composition Data: Production, Management and Use , 2nd , Rome , , Italy : Food and Agriculture Organization of United Nations (FAO) .
- accessed May 23, 2010). www.ars.usda.gov (http://www.ars.usda.gov)
- Espinosa-Martos , I. , Rico , E. and Ruperez , P. 2006 . Note. Low molecular weight carbohydrates in foods usually consumed in Spain . Food Science Technology International , 12 : 171 – 175 .
- Arrizon , J. , Morel , S. , Gschaedler , A. and Monsan , P. 2010 . Comparison of water-soluble carbohydrate composition and fructan structures of Agave tequilana plants of different ages . Food Chemistry , 122 : 123 – 130 .
- Alvin , C.R. 2002 . Methods of Multivariate Analysis , USA : John Wiley & Sons. Inc .
- Livesey , G. 2001 . Tolerance of low-digestible carbohydrates: A general view . British Journal of Nutrition , 85 : 7 – 16 .
- Muir , J.G. , Rose , R. , Rosella , O. , Liels , K. , Barrett , J.S. , Shepherd , S.J. and Gibson , P.R. 2009 . Measurement of short-chain carbohydrates in common Australian vegetables and fruits by high-performance liquid chromatography (HPLC) . Journal of Agricultural and Food Chemistry , 57 : 554 – 565 .
- Campbell , J.M. , Bauer , L.L. , Fahey , G.C. , Hogarth , A.J.C.L. , Wolf , B.W. and Hunter , D.E. 1997 . Selected fructo-oligosaccharide (1-kestose, nystose, and 1F-β- fructofuranosylnystose) composition of foods and feeds . Journal of Agricultural and Food Chemistry , 45 : 3076 – 3082 .
- Praznik , W. and Beck , R.H.F. 1987 . Inulin composition during growth of tubers of Helianthus tuberosus L . Agricultural and Biological Chemistry , 51 : 1593 – 1599 .
- Darbyshire , B. and Henry , R.J. 1981 . Differences in fructan content and synthesis in some Allium species . New Phytologist , 87 : 249 – 256 .
- Van Loo , J. , Coussement , P. , Leenheer , L.D. , Hoebregs , H. and Smits , G. 1995 . On the presence of inulin and oligofructose as natural ingredients in the western diet . Critical Reviews in Food Science , 35 : 525 – 552 .
- Wilson , R.G. , Smith , J.A. and Yonts , C.D. 2004 . Chicory root yield and carbohydrate composition is influenced by cultivar, planting, and harvest date . Crop Science , 44 : 748 – 752 .
- Kocsis , L. , Liebhard , P. and Praznik , W. 2007 . Effect of seasonal changes on content and profile of soluble carbohydrates in tubers of different varieties of Jerusalem artichoke (Helianthus tuberosus L.) . Journal of Agricultural and Food Chemistry , 55 : 9401 – 9408 .