Abstract
Antioxidants are usually considered with regard to plant defense mechanisms due to the oxidative stress or their importance for human health or both. A comprehensive study in the present research was made to determine the variation in contents of antioxidants and their metabolites (such as ascorbate and glutathione) and enzymes activities of ‘Red Fuji’ apple pulp and peel tissue during cold storage (0 ± 1°C). Results showed that the majority of antioxidant metabolites were maintained in the reduced state, reduced ascorbate and glutathione, mainly localized in apple peel tissue. Strong or very strong correlations between hydrogen peroxide content and superoxide dismutase activity (r = 0.822 and 0.959), reduced/oxidized ascorbate ratio and monodehydroascorbate reductase activity (r = 0.966 and 0.984) as well as reduced/oxidized glutathione ratio and glutathione reductase activity (r = 0.969 and 0.924) were discovered. These results highlight that apple peel tissue could be selected to explore antioxidant mechanism and defense metabolism of apple fruit.
INTRODUCTION
Fruits are good sources of natural antioxidants, which are enzymatic and non-enzymatic components capable of quenching biologically toxic reactive oxygen species (ROS) without undergoing conversion to harmful free radicals.[Citation1– Citation3] The presence of natural antioxidants, such as flavonoids and other polyphenolic compounds, are gaining importance, due to their benefits for human health, decreasing the risk of degenerative diseases by reduction of oxidative stress and inhibition of macromolecular oxidation.[Citation4– Citation7] These antioxidants include the water-soluble metabolites such as ascorbate and glutathione, the lipid-soluble compounds such as the carotenoids and tocopherols, and the enzymes such as superoxide dismutase (SOD, EC 1.15.1.1), catalase (CAT, EC 1.11.1.6), ascorbate peroxidase (APX, EC 1.11.1.11), glutathione reductase (GR, EC 1.8.1.7), monodehydroascorbate reductase (MDAR, EC 1.6.5.4), and dehydroascorbate reductase (DHAR, EC 1.8.5.1). Previous studies have reported that there are significant and positive correlations (r > 0.9) between antioxidant capacity and certain antioxidant components.[Citation8, Citation9]
At the fruit tissue level, there are significant qualitative and quantitative differences among the antioxidant contents of seeds, peel, and flesh.[Citation10, Citation11] As previously reported, the peel was richer in phenols,[Citation12– Citation16] ascorbic acid, reduced glutathione (GSH)[Citation10, Citation11] contents, and antioxidant enzymes activities[Citation11] in apple cultivars. Regardless of different methods employed, the peel had a significantly higher antioxidant capacity than the flesh[Citation17– Citation19] or peel followed by flesh with skin and flesh.[Citation20] The peel tissue is commonly selected to explore antioxidant and defense mechanisms, because this part of the fruit is a primary target on unfavorable oxidative stress.
‘Red Fuji’ apple (Malus domestica Borkh.) stands out for its excellent quality and sensory characteristics, as well as for good storage potential at low temperatures. Increasing evidences suggest that apples are attracting the attention of consumers due to their high nutritional value and efficient antioxidant system. Although the antioxidant capacity of apple fruits is relatively known, as far as we know, little information is available about the differences of enzymatic and non-enzymatic antioxidant system between different types of fruit tissue in ‘Red Fuji’ apple fruit. Therefore, the main objective of this work was to determine the antioxidant system in different types of tissue in ‘Red Fuji’ apple, with investigations on the variation in contents of antioxidant metabolites [ascorbate (AsA), dehydroascorbate (DHAsA), reduced glutathione (GSH), and oxidized glutathione (GSSG)], which might be actively involved in oxygen detoxification. In addition, we also evaluated the activities of antioxidant enzymes (SOD, CAT, APX, GR, MDAR, and DHAR) involved in the AsA-GSH cycle in apple pulp and peel tissues during cold storage (0 ± 1°C).
MATERIALS AND METHODS
Chemicals
All chemicals and reagents, including reduced and oxidized ascorbate, reduced and oxidized glutathione, hydrogen peroxide, trichloroacetic acid (TCA), sulphosalicylic acid, dithiothreitol (DTT), N-ethylmaleimide (NEM), 2-vinylpyridine, EDTA, polyvinylpyrrolidone (PVP-10), Triton X-100, and β-mercaptoethanol were of analytical grade and were obtained from commercial sources (Sigma-Aldrich, St. Louis, MO, USA). The water was treated in a water purification system. The RC/DC protein assay kit and bovine serum albumin (BSA) standard was obtained from Bio-Rad Laboratories (Hercules, CA, USA).
Sample Preparation
Apple (Malus domestica Borkh cv. ‘Red Fuji’) fruits were hand-harvested from 5-year-old uniform trees according to a completely randomized design and at the optimal commercial date. Fruits were selected for uniform size and external color, eliminated damaged material, and immediately stored at 0 ± 1°C, 95−97% RH, in 10-kg boxes, and for each tested time fruits were randomly selected for chemical analyses.
Apple pulp and peel tissues were tested just after harvest and after 60 and 90 days of storage for chemical analyses. Five replicates for chemical analyses for each type of tissue were prepared and each of them included tissue samples from three fruits. Each apple fruit was peeled with a potato knife, thus a thin layer of apple pulp remained adhered to the peel, and the pulp was cut into thin pieces. Both types of tissues were immediately frozen in liquid nitrogen and stored at −80°C until analyses. Directly before analyses, apple tissues were ground to a fine powder in liquid nitrogen using a Waring commercial blender (Black & Decker, MD, USA) followed by a mortar and pestle.
H2O2 Assay
Hydrogen peroxide (H2O2) content was determined according to the method proposed by Wang and Zheng with slight modifications.[Citation21] Ground pulp and peel tissues (5 g) were ground with a homogenizer (Sentry Microprocessor with Cyclone I.Q.2, Virtis, SP Industries, Gardiner, NY, USA) for 1 min in 10 ml of 0.1% (w/v) trichloroacetic acid (TCA) and the homogenate was centrifuged at 12,000× g for 15 min at 4°C. H2O2 concentration was determined at 410 nm by a standard graph from 5 μM to 1 mM H2O2 constructed by direct addition of H2O2 to the titanium solution. The H2O2 value was expressed as μmol g−1 fresh weight (FW).
Extraction and Measurement of Antioxidant Metabolites
Ground tissues were homogenized in 25 mM of ice cold sulphosalicylic acid. The final homogenate was centrifuged at 12,000× g for 20 min at 4°C, and the supernatant was collected for analyses of ascorbate and glutathione. Total ascorbate, reduced ascorbate (AsA), and dehydroascorbate (DHAsA) contents were determined according to the method reported by Law et al., with minor modifications.[Citation22] Total ascorbate was determined by initial incubation in 200 mM phosphate buffer solution (pH 7.4) and 1.5 mM dithiothreitol (DTT) for 50 min to reduce all DHAsA to AsA. After incubation, 200 μl of 0.5% (w/v) N-ethylmaleimide (NEM) was added to remove the excess DTT. AsA was analyzed in a similar manner except that 400 μl of deionized H2O was substituted for DTT and NEM. Absorbance was monitored at 525 nm. For each sample, DHAsA was calculated as the difference between total ascorbate and AsA. Total glutathione, reduced glutathione (GSH), and oxidized glutathione (GSSG) were measured according to the method of Griffith.[Citation23] GSSG was measured after the removal of GSH by 2-vinylpyridine derivatization. Absorbance was monitored at 412 nm for 15 min. GSH was determined by subtraction of GSSG from the total glutathione content.
Extraction and Assay of Antioxidant Enzymes
For enzymes extraction, ground tissues (100 mg) were homogenized in 500 μl of the following optimized media: (1) a buffer mixture containing 50 mM potassium phosphate buffer (pH 7.8), 0.1 mM EDTA, 1% (w/v) soluble polyvinylpyrrolidone (PVP-10), and 0.1% (v/v) Triton X-100 for SOD assay; (2) a buffer mixture containing 50 mM potassium phosphate buffer (pH 7.0) and 0.5% PVP-10 for APX and CAT assay (for the APX assay, this buffer was supplemented with 5 mM ascorbate to avoid the inactivation of the plastidic isoform); (3) a buffer mixture containing 50 mM potassium phosphate buffer (pH 7.8), 1% PVP-10, 0.2 mM EDTA, and 10 mM β-mercaptoethanol for MDAR, DHAR, and GR assay. Then the extracts were centrifuged at 12,000× g for 20 min at 4°C and the enzyme activities were assayed in the supernatants. For enzymes assay, the SOD activity was assayed by a method based on the inhibition of ferricytochrome c reduction by superoxide at 550 nm[Citation24] and CAT activity by following the decomposition of H2O2 at 240 nm.[Citation25] APX and DHAR activities were determined by monitoring ascorbate oxidation at 290 nm[Citation26] and ascorbate formation at 265 nm,[Citation27] respectively. MDAR and GR activities were assayed by following the oxidation of NADH[Citation28] and NADPH[Citation29] at 340 nm, respectively. Protein content was determined using the RC/DC protein assay kit (Bio-Rad Laboratories, Hercules, CA, USA) to compensate for interfering compounds according to the manufacturer's protocol. Bovine serum albumin (BSA) was employed as a standard.
Statistical Analyses
All experiments were replicated three times and all the values were expressed as means ± standard deviation. The data were performed using one-way analysis of variance (ANOVA) with SPSS version 17.0 (SPSS, Inc.) and Duncan's Multiple Range Test at the 0.05 significance level. Correlation coefficients between antioxidant metabolites contents and enzymes activities were performed. To provide a general overview of the results, a principal component analysis (PCA) was developed to outline the relationship between the variables studied.
RESULTS
H2O2 Production
As shown in , H2O2 content increased gradually both in apple pulp and peel tissues over the duration of our experiments. H2O2 content in apple peel tissue was 137.5–165.7% as high as in apple pulp tissue during the storage time.
Antioxidant Metabolites
The AsA content exhibited similar patterns both in apple pulp and peel tissues during cold storage, with a slight decreased value on 60 days (16.4 and 8.8%, respectively), then a significant increase on 90 days (35.9 and 14.9%, respectively) (). In contrast, DHAsA content decreased gradually with time of cold storage (). Total ascorbate and AsA contents in peel tissue were approximately 3.1 to 6.2 times higher than in pulp tissue, whereas DHAsA was only 1.9 to 2.2 times higher.
Figure 2 AsA (a), DHAsA (b), GSH (d), and GSSG (e) contents in apple pulp and peel tissues during cold storage. Calculated AsA/DHAsA ratio (c) and GSH/GSSG ratio (f) are issued from the observed values. Values are expressed as mean ± standard deviation (n = 3). Means followed by the same letters are not significantly different by Duncan's multiple range test (p < 0.05).
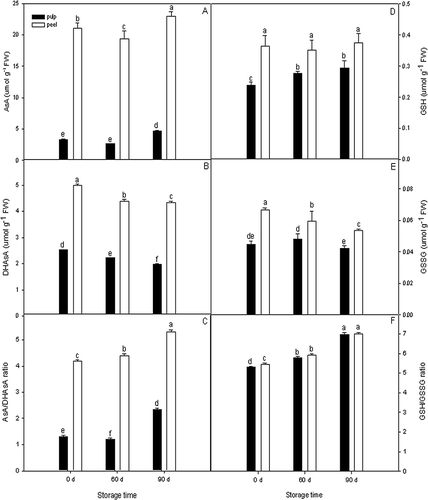
With regard to glutathione, the total glutathione, GSH, and GSSG contents in peel tissue were also significantly higher than in pulp tissue (p < 0.05). The majority of glutathione in a plant cell is maintained in the reduced state (GSH), with values of 0.24–0.30 and 0.35–0.38 μmol g−1 FW in apple pulp and peel tissues, respectively (), while GSSG was found to be present in low concentrations below 0.07 μmol g−1 FW in both tissues ().
The values of AsA/DHAsA and GSH/GSSG ratio were shown in and , respectively. In the present study, the AsA/DHAsA ratio was found to be significantly higher in peel tissue compared to pulp tissue during cold storage (p < 0.05). In contrast, the ratio of glutathione (GSH/GSSG) in peel tissue was also higher than in pulp tissue, but not significant (p > 0.05).
Activities of Antioxidant Enzymes
The apple peel tissue exhibited significantly higher antioxidant enzymes (SOD, APX, GR, MDAR, and DHAR) activities, with the exception of CAT (). With an increase in storage period, apple peel tissue exhibited a decrease in CAT activity by 8.6% on 60 days then a decrease by 18.5% on 90 days as compared with initial activity just after harvest. While apple pulp tissue showed a different trend with a sharp rise of 41.4% on 60 days and then a fast decline of 17.1% on 90 days (). The activities of SOD, GR, and MDAR enzymes in apple peel tissue increased much more rapidly than in pulp tissue with storage time (, , nd 3e). In the present study, we found that GR activity ranged from 56.9 to 70.1 and 71.2 to 99.5 nmol NADPH min−1 mg−1 protein in apple pulp and peel tissues, respectively (). In contrast, the DHAR activity decreased gradually during cold storage, with the values decreased by 36.3 and 22.0% for pulp and peel tissue after 90 days, respectively (). It is interesting to point out that the APX activity in pulp tissue decreased by 28.9% during the first 60 days, and then increased by 22.8% during the later 30 days of cold storage. Comparatively, the APX activity in peel tissue first increased by 5.5% and then decreased by 34.3% ().
Correlations
There was a strong positive correlation between H2O2 content and SOD activity both in apple pulp (r = 0.822) and peel (r = 0.959) tissue, while negative for APX activity (). But for CAT activity, only in pulp tissue was it positively correlated. The AsA content and AsA/DHAsA ratio strongly positive correlated with MDAR activity and negative correlated with DHAR activity. Obviously, a strong correlation between GR activity and GSH/GSSG ratio was found in pulp (r = 0.969) and peel (r = 0.924) tissue. High GR activity also strongly correlated to high GSH in pulp tissue, but weaker in peel tissue. The weak or negative correlations between AsA/DHAsA ratio and APX activity were also evident (r = 0.162 or –0.952), regardless of tissue types. Also, the correlations between DHAR activity and GSH content or GSH/GSSG ratio were negative in apple pulp and peel tissues.
Table 1 Correlation coefficient between antioxidant metabolites contents and enzymes activities in apple pulp and peel tissues
DISCUSSION
The present study showed that the antioxidant contents in ‘Red Fuji’ apple varied considerably depending on the tissue types. It was confirmed that apple is a good source of such antioxidant metabolites as ascorbate, glutathione, and a variety of phenolic compounds.[Citation30, Citation31] Tissue type was one of the most prominent factors that affected total ascorbate and glutathione content.[Citation32] Ascorbate plays a fundamental role in antioxidant system in relation to the enzymatic and non-enzymatic oxidations in the biological system. Total ascorbate content in the peel was from approximately 3 (90 days) to above 3.5 times higher (0 days) in comparison to the pulp tissue. Our results showed that both AsA and DHAsA contents in the peel were also above 4 times higher than in the pulp, in keeping with many other studies that fruit phytochemicals are predominantly localized in the peel.[Citation13, Citation33, Citation34] As shown in , DHAsA could easily be reduced to AsA by DHAR in the presence of the reduced form of glutathione (GSH). GSH is one of the most efficient scavengers and reacts directly with free radicals to prevent the inactivation of enzymes by oxidation of the essential thiol group.[Citation11, Citation35] As similar to ascorbate content, the contents of total glutathione, GSH, and GSSG in peel tissue were significantly higher than those in pulp tissue (). The results indicated that the developmental changes in GSH and GSSG contents were in agreement with results reported by Kosower and Kosower ().[Citation36]
Taking into account the AsA-GSH cycle, H2O2 was produced mainly by dismutation of O2 •− by the SOD enzyme, which has been implicated as essentially the first line of defense against the potent toxicity (). ROS, including H2O2 and O2 •−, which are toxic molecules capable of causing oxidative damage to proteins, DNA, and lipid.[Citation1] It is now widely recognized that H2O2 can act as a systemic signal in plant antioxidant defense responses and in adaptation of plants to abiotic stress.[Citation1, Citation37–39] Excess H2O2 was quenched as an effect of enzymes, such as CAT and APX (). During the first 60 days of cold storage, H2O2 accumulation induced an increase of CAT activity both in pulp and peel tissues, which played an important role in the catabolism of H2O2. Following, decreased CAT activity resulted in a dramatic increase of H2O2 content both in pulp and peel tissues (). However, the H2O2 content during the later cold storage was still much higher possibly also because of the higher SOD or lower APX activity or both (). These results suggested that different antioxidant enzymes showed different special and temporal patterns in the regulation of ROS during storage, among which CAT exerted its effect during the early storage, especially in pulp tissue, while SOD and/or APX played an essential role in peel tissue during the later storage period.
In the present study, the mean SOD activity in peel tissue was up to 3.1 times higher than pulp tissue during storage period, similar to the results from Abbasi et al.,[Citation40] who observed that SOD activity in skin tissue was 4.2 times higher than pulp tissue during ‘Pink Lady’ maturation and ripening. Also, because high levels of H2O2 are toxic for plants, the activity of detoxifying enzymes, such as CAT, is needed for a fine modulation of H2O2 levels below toxic concentrations.[Citation41, Citation42] Change in CAT activity may be partly due to the inhibition by low temperature.[Citation43] Both SOD and CAT contributed to neutralize reactive oxygen species to maintain well-balanced metabolism in fruit, and increase in these enzymes activities in plant tissues subject to abiotic stress could prolong storage time.[Citation41]
On the basis of ROS elimination by AsA and GSH, AsA and GSH were oxidized and recycled by enzymes of AsA-GSH cycle (). It should be pointed out that the proportion of DHAsA and GSSG contents decreased during storage, which might mean that the capacity of AsA-GSH cycle did not decrease in the apple peel and pulp during 90 days of cold storage.[Citation11] As suggested by Kocsy et al., the change of AsA/DHAsA and GSH/GSSG ratio is more important as compared with AsA or GSH content in cell resistant to oxidative stress.[Citation44] The change in AsA/DHAsA ratio, an important indicator of the redox status of the plant cell, has been proved to be one of the first signs of oxidative stress.[Citation45] The significant increase in AsA/DHAsA ratio observed in the peel tissue could be due to an enhancement of reduced ascorbate regeneration. A previous study reported that a high GSH/GSSG ratio is also necessary for several physiological functions, and this includes activation and inactivation of redox-dependent systems.[Citation46]
According to the biochemical pathway of antioxidant reactions in plant (), APX catalyzes the first step of the AsA-GSH cycle and is highly specific for ascorbate as the electron donor.[Citation39] AsA is regenerated by utilizing GSH to form GSSG and GR catalyzes regeneration of GSH from GSSG.[Citation47] In that case, GR activity has been reported to be associated with alteration of the GSH/GSSG ratio, which is more decisive in determining plant resistance to abiotic and biotic stresses than the actual GSH content.[Citation48] The high activity of GR and a high level of GSH content may play a crucial role as antioxidants and could prevent the formation of S–S bonds, therefore providing high antioxidant activity in plants.[Citation35] Considering that peel tissue had higher GR activity than pulp tissue, it is possible that only a proportion of the total GR in a peel is engaged in converting GSSG to GSH in vivo.
Both MDAR and DHAR are enzymes responsible for ascorbate regeneration in plant tissues. The main function of MDAR is to limit the amount of MDHA radical undergoing non-enzymatic disproportionation for DHAsA generation.[Citation49] The higher activity of DHAR can result from GSH utilization as the electron donor for DHAsA reduction. In addition, the higher ratio of AsA/DHAsA in apple peel during cold storage indicated that the regeneration of AsA was increased compared with apple pulp (). In order to investigate the correlations, a PCA was performed using all the parameters studied (data of antioxidant metabolites and enzyme activities). Using all these values in the pulp and peel tissue, an evident differentiation among the parameters is possible, with the models explaining 99.6 and 99.3%, respectively, of all the variance detected in the experimental data ().
CONCLUSIONS
Collectively, the results presented in this study could confirm that ‘Red Fuji’ apple has high antioxidant capacities, antioxidant enzyme activities, and non-enzyme components, obviously with significant differences between tissue types. Apple peel exhibited higher content of antioxidant metabolites than apple pulp, associated with maintenance of higher activities of antioxidant enzymes involved in AsA-GSH cycle during cold storage (with the exception of CAT). These results indicated that the high ROS scavenging capacity provided by the apple tissue should be the potential to prevent unfavorable oxidative damage and improve health-promoting quality for consumers.
ABBREVIATIONS
APX | = |
Ascorbate peroxidase |
AsA | = |
Reduced ascorbate |
CAT | = |
Catalase |
DHAR | = |
Dehydroascorbate reductase |
DHAsA | = |
Dehydroascorbate |
GPX | = |
Glutathione peroxidase |
GR | = |
Glutathione reductase |
GSH | = |
Reduced glutathione |
GSSG | = |
Oxidized glutathione |
H2O2 | = |
Hydrogen peroxide |
MDAR | = |
Monodehydroascorbate reductase |
MDAsA | = |
Monodehydroascorbate |
NADPH | = |
Reduced nicotinamide adenine dinucleotide phosphate |
NEM | = |
N-Ethylmaleimide |
1O2 | = |
Singlet oxygen |
O2 •− | = |
Superoxide |
OH• | = |
Hydroxyl radical |
PCA | = |
Principal component analysis |
ROS | = |
Reactive oxygen species |
SOD | = |
Superoxide dismutase |
ACKNOWLEDGMENT
The authors are grateful for the financial support provided by the Cultivation Foundation for the Excellent Ph.D. Dissertation from Tianjin University of Science and Technology, granted for Li Li.
REFERENCES
- Apel , K. and Hirt , H. 2004 . Reactive oxygen species: Metabolism, oxidative stress, and signal transduction . Annual Review of Plant Biology , 55 : 373 – 399 .
- Møller , I.M. , Jensen , P.E. and Hansson , A. 2007 . Oxidative modifications to cellular components in plants . Annual Review of Plant Physiology , 58 : 459 – 481 .
- Nabavi , S.M. , Nabavi , S.F. , Eslami , S. and Moghaddam , A.H. 2012 . In vivo protective effects of quercetin against sodium fluoride-induced oxidative stress in the hepatic tissue . Food Chemistry , 132 ( 2 ) : 931 – 935 .
- Michalczyk , M. and MacUra , R. 2010 . Effect of processing and storage on the antioxidant activity of frozen and pasteurized shadblow serviceberry (Amelanchier canadensis) . International Journal of Food Properties , 13 ( 6 ) : 1225 – 1233 .
- Korus , A. 2011 . Level of vitamin C, polyphenols, and antioxidant and enzymatic activity in three varieties of kale (Brassica oleracea l. var. Acephala) at different stages of maturity . International Journal of Food Properties , 14 ( 5 ) : 1069 – 1080 .
- Nabavi , S.F. , Moghaddam , A.H. , Eslami , S. and Nabavi , S.M. 2012 . Protective effects of curcumin against sodium fluoride-induced toxicity in rat kidneys . Biological Trace Element Research , 145 ( 3 ) : 369 – 374 .
- Nabavi , S.F. , Nabavi , S.M. , Abolhasani , F. , Moghaddam , A.H. and Eslami , S. 2012 . Cytoprotective effects of curcumin on sodium fluoride-induced intoxication in rat erythrocytes . Bulletin of Environmental Contamination and Toxicology , 88 ( 3 ) : 486 – 490 .
- Tyug , T.S. , Johar , M.H. and Ismail , A. 2010 . Antioxidant properties of fresh, powder, and fiber products of mango (Mangifera foetida) fruit . International Journal of Food Properties , 13 ( 4 ) : 682 – 691 .
- Erge , H.S. and Karadeniz , F. 2011 . Bioactive compounds and antioxidant activity of tomato cultivars . International Journal of Food Properties , 14 ( 5 ) : 968 – 977 .
- Davey , M. , Franck , C. and Distribution , Keulemans, J. 2004 . developmental and stress responses of antioxidant metabolism in Malus . Plant Cell & Environment , 27 ( 10 ) : 1309 – 1320 .
- Łata , B. , Trąmpczyńska , A. and Oleś , M. 2005 . Antioxidant content in the fruit peel, flesh and seeds of selected apple cultivars during cold storage . Folia Horticulturae , 17 ( 1 ) : 47 – 60 .
- Escarpa , A. and Gonzalez , M. 1998 . High-performance liquid chromatography with diode-array detection for the determination of phenolic compounds in peel and pulp from different apple varieties . Journal of Chromatography A , 823 ( 1–2 ) : 331 – 337 .
- Van der Sluis , A.A. , Dekker , M. , De Jager , A. and Jongen , W.M.F. 2001 . Activity and concentration of polyphenolic antioxidants in apple: Effect of cultivar, harvest year, and storage conditions . Journal of Agricultural and Food Chemistry , 49 ( 8 ) : 3606 – 3613 .
- Tsao , R. , Yang , R. , Young , J.C. and Zhu , H. 2003 . Polyphenolic profiles in eight apple cultivars using high-performance liquid chromatography (HPLC) . Journal of Agricultural and Food Chemistry , 51 ( 21 ) : 6347 – 6353 .
- Chinnici , F. , Gaiani , A. , Natali , N. , Riponi , C. and Improved , Galassi, S. 2004 . HPLC determination of phenolic compounds in cv . Golden Delicious apples using a monolithic column. Journal of Agricultural and Food Chemistry , 52 ( 1 ) : 3 – 7 .
- McGhie , T.K. , Hunt , M. and Barnett , L.E. 2005 . Cultivar and growing region determine the antioxidant polyphenolic concentration and composition of apples grown in New Zealand . Journal of Agricultural and Food Chemistry , 53 ( 8 ) : 3065 – 3070 .
- Schirrmacher , G. and Schempp , H. 2003 . Antioxidative potential of flavonoid-rich extracts as new quality marker for different apple varieties . Journal of Applied Botany , 77 ( 5–6 ) : 163 – 166 .
- Chinnici , F. , Bendini , A. , Gaiani , A. and Riponi , C. 2004 . Radical scavenging activities of peels and pulps from cv . Golden Delicious apples as related to their phenolic composition. Journal of Agricultural and Food Chemistry , 52 ( 15 ) : 4684 – 4689 .
- Tsao , R. , Yang , R. , Xie , S. , Sockovie , E. and Khanizadeh , S. 2005 . Which polyphenolic compounds contribute to the total antioxidant activities of apple? . Journal of Agricultural and Food Chemistry , 53 ( 12 ) : 4989 – 4995 .
- Wolfe , K. , Wu , X. and Liu , R.H. 2003 . Antioxidant activity of apple peels . Journal of Agricultural and Food Chemistry , 51 ( 3 ) : 609 – 614 .
- Wang , S.Y. and Zheng , W. 2001 . Effect of plant growth temperature on antioxidant capacity in strawberry . Journal of Agricultural and Food Chemistry , 49 : 4977 – 4982 .
- Law , M.Y. , Charles , S.A. and Halliwell , B. 1983 . Glutathione and ascorbic acid in spinach (Spinacia oleracea) chloroplasts . The effect of hydrogen peroxide and of Paraquat. Biochemical Journal , 210 ( 3 ) : 899 – 903 .
- Griffith , O.W. 1980 . Determination of glutathione and glutathione disulfide using glutathione reductase and 2-vinylpyridine . Analytical Biochemistry , 106 ( 1 ) : 207 – 212 .
- Fernández-Trujillo , J.P. , Nock , J.F. and Watkins , C.B. 2007 . Antioxidant enzyme activities in strawberry fruit exposed to high carbon dioxide atmospheres during cold storage . Food Chemistry , 104 ( 4 ) : 1425 – 1429 .
- Aebi , H. 1984 . Catalase in vitro . Methods in Enzymology , 105 : 121 – 126 .
- Chloroplasts , Asada, K. 1984 . Formation of active oxygen and its scavenging . Methods in Enzymology , 105 : 422 – 429 .
- Nakano , Y. and Asada , K. 1981 . Hydrogen peroxide is scavenged by ascorbate-specific peroxidase in spinach chloroplasts . Plant and Cell Physiology , 22 ( 5 ) : 867 – 880 .
- Dalton , D.A. , Baird , L.M. , Langeberg , L. , Taugher , C.Y. , Anyan , W.R. , Vance , C.P. and Sarath , G. 1993 . Subcellular localization of oxygen defense enzymes in soybean (Glycine max [L.] Merr.) root nodules . Plant Physiology , 102 ( 2 ) : 481 – 489 .
- Dalton , D.A. , Russell , S.A. , Hanus , F.J. , Pascoe , G.A. and Evans , H.J. 1986 . Enzymatic reactions of ascorbate and glutathione that prevent peroxide damage in soybean root nodules . Proceedings of the National Academy of Sciences , 83 ( 11 ) : 3811 – 3815 .
- Valencia , E. , Marin , A. and Hardy , G. 2001 . Glutathione—Nutritional and pharmacologic viewpoints: Part IV . Nutrition , 17 ( 9 ) : 783 – 784 .
- Awad , M.A. and De Jager , A. 2003 . Influences of air and controlled atmosphere storage on the concentration of potentially healthful phenolics in apples and other fruits . Postharvest Biology and Technology , 27 ( 1 ) : 53 – 58 .
- Łata , B. 2007 . Relationship between apple peel and the whole fruit antioxidant content: Year and cultivar variation . Journal of Agricultural and Food Chemistry , 55 ( 3 ) : 663 – 671 .
- Eberhardt , M.V. , Lee , C.Y. and Liu , R.H. 2000 . Antioxidant activity of fresh apples . Nature , 405 ( 6789 ) : 903 – 904 .
- Kondo , S. , Tsuda , K. , Muto , N. and Ueda , J.E. 2002 . Antioxidative activity of apple skin or flesh extracts associated with fruit development on selected apple cultivars . Scientia Horticulturae , 96 ( 1–4 ) : 177 – 185 .
- Jiao , H. and Wang , S.Y. 2000 . Correlation of antioxidant capacities to oxygen radical scavenging enzyme activities in blackberry . Journal of Agricultural and Food Chemistry , 48 ( 11 ) : 5672 – 5676 .
- Kosower , N.S. and Kosower , E.M. 1978 . The glutathione status of cells . International Review of Cytology , 54 : 109 – 160 .
- Levine , A. , Tenhaken , R. , Dixon , R. and H2O2 , Lamb, C. 1994 . from the oxidative burst orchestrates the plant hypersensitive disease resistance response . Cell , 79 ( 4 ) : 583 – 593 .
- Vandenabeele , S. , Van Der Kelen , K. , Dat , J. , Gadjev , I. , Boonefaes , T. , Morsa , S. , Rottiers , P. , Slooten , L. , Van Montagu , M. , Zabeau , M. , Inzé , D. and Van Breusegem , F. 2003 . A comprehensive analysis of hydrogen peroxide-induced gene expression in tobacco . Proceedings of the National Academy of Sciences of the United States of America , 100 ( 26 ) : 16113 – 16118 .
- Foyer , C.H. and Noctor , G. 2005 . Redox homeostasis and antioxidant signaling: A metabolic interface between stress perception and physiological responses . Plant Cell , 17 ( 7 ) : 1866 – 1875 .
- Abbasi , N.A. , Singh , Z. and Khan , A.S. 2010 . Dynamics of anti-oxidant levels and activities of reactive oxygen-scavenging enzymes in ‘pink lady'apple fruit during maturation and ripening . Pakistan Journal of Botany , 42 ( 4 ) : 2605 – 2620 .
- Mittler , R. 2002 . Oxidative stress, antioxidants and stress tolerance . Trends in plant science , 7 ( 9 ) : 405 – 410 .
- Zhu , S. , Sun , L. , Liu , M. and Zhou , J. 2008 . Effect of nitric oxide on reactive oxygen species and antioxidant enzymes in kiwifruit during storage . Journal of the Science of Food and Agriculture , 88 ( 13 ) : 2324 – 2331 .
- Fadzillah , N.M. , Gill , V. , Finch , R.P. and Burdon , R.H. 1996 . Chilling, oxidative stress and antioxidant responses in shoot cultures of rice . Planta , 199 ( 4 ) : 552 – 556 .
- Kocsy , G. , Galiba , G. and Brunold , C. 2001 . Role of glutathione in adaptation and signalling during chilling and cold acclimation in plants . Physiologia Plantarum , 113 ( 2 ) : 158 – 164 .
- Meneguzzo , S. , Navari-Izzo , F. and Izzo , R. 1999 . Antioxidative responses of shoots and roots of wheat to increasing NaCl concentrations . Journal of Plant Physiology , 155 ( 2 ) : 274 – 280 .
- Ziegler , D. 1985 . Role of reversible oxidation-reduction of enzyme thiols-disulfides in metabolic regulation . Annual Review of Biochemistry , 54 ( 1 ) : 305 – 329 .
- Zhao , S. and Blumwald , E. 1998 . Changes in oxidation-reduction state and antioxidant enzymes in the roots of jack pine seedlings during cold acclimation . Physiologia Plantarum , 104 ( 1 ) : 134 – 142 .
- Kmives , T. , Gullner , G. and Király , Z. 1998 . Role of glutathione and glutathione-related enzymes in response of plants to environmental stress . Annals of the New York Academy of Sciences , 851 : 251 – 258 .
- Noctor , G. and Foyer , C.H. 1998 . Ascorbate and glutathione: Keeping active oxygen under control . Annual Review of Plant Biology , 49 ( 1 ) : 249 – 279 .