Abstract
The purpose of this work was to investigate the effect of different spray drying conditions on the physicochemical properties of dried lactose. Results indicated that the feed concentration and inlet temperature, as well as storage condition, influenced the thermal properties of the dried material, which were evaluated by the degree of molecular relaxation, presumably associated to different levels of molecular mobility in the sample. It was evident that higher drying temperatures decreased molecular mobility of the dried material while increasing its glass transition and crystallization temperatures. This is most likely due to the existence of states of lower mobility within the material as those with higher mobility were relaxed. The change in heat capacity at the glass transition temperature, , and the reduced crystallization temperature,
, were used to compare the crystallization tendencies of the spray dried powders. It was observed that a decrease in
and an increase in
were associated with higher crystallization temperatures. On the other hand, the enthalpic relaxation
did not change significantly with storage conditions. The findings illustrated that the initial enthalpy relaxation, which occurred during spray drying, was related to the crystallization tendencies and storage conditions.
INTRODUCTION
Many foods and pharmaceuticals exist in a disordered amorphous state as a result of some deliberate or accidental variations in the processing conditions. Many processes used in production of these materials are usually associated with some kind of modification or alteration of their molecular structure. Processes, such as spray drying, lyophilization, milling, hot-melt extrusion, melt quench cooling, among others, are generally used to intentionally produce amorphous materials,[Citation1–Citation8] while other routine manufacturing operations, such as coating, granulation, drying, and compression, may accidentally introduce undesired amorphous regions in a given product.[Citation9] Thus, metastable amorphous solids, called glasses, are frequently encountered in foods, pharmaceuticals, and polymer ingredients.[Citation10] These materials exist in a non-equilibrium state that is characterized by an excess in their thermodynamic properties (volume, enthalpy, and entropy) as compared with their crystalline more stable form. The glassy state raises various concerns regarding the stability and quality of materials as these systems undergo structural changes that reduce the excess thermodynamic quantities tending to establish equilibrium states.[Citation11] Changes in the physicochemical properties may also occur at temperatures below Tg since molecular mobility is not totally eliminated but rather only minimized.
Several studies have revealed that glassy materials undergo slow transitions in an attempt to establish equilibrium even at temperatures well below their glass transition (Tg ) indicating that the molecules still have some degree of mobility,[Citation12,Citation13] contrarily to an assumed equilibrium condition even at temperatures below glass transition.[Citation12,Citation14] This gradual approach to equilibrium is described as physical aging accompanied by volume and enthalpy changes, also known as relaxations. These changes are a result of local molecular motions,[Citation15 − Citation17] which affect various physicochemical properties of the material.[Citation13,Citation18 − Citation20] It is also believed that physical aging may contribute to the development of residual stresses in the materials.[Citation21] Time, temperature, and environmental conditions, such as relative humidity, can contribute to this aging phenomenon, e.g., an increase in aging time can increase the enthalpy relaxation and decreases the glass transition temperature of the material.[Citation13,Citation14,Citation22 − Citation24]
Results obtained by differential scanning calorimetry (DSC) at or around Tg of aged materials mostly reveals any regained enthalpy that was lost during the aging process. demonstrates a schematic of a typical DSC result showing the glass transition phenomenon of an un-aged sample as it compares to the observed glass transition event accompanied by an endothermic peak, which corresponds to the enthalpy relaxation of an aged sample ().[Citation14,Citation21] According to Struik,[Citation13] physical aging can be explained by the free-volume concept since the mobility of the particles in a packed system is primarily determined by the degree of packing or by the available free volume. In other words, as the free volume decreases, the degree of packing increases and the mobility of the particles in a given system will decrease. As aging proceeds and the amorphous solid progresses towards a thermodynamic equilibrium, a decrease in the free-volume is observed, which is usually accompanied by changes in the material properties.[Citation13,Citation20,Citation25,Citation26] This thermodynamic equilibrium state is attained as the material relaxes to a more energetically favorable conformation and progresses over time towards its crystalline stable form.[Citation25,Citation27] Moreover, Hutchinson[Citation28] described physical aging as the process that involves reversible changes in the material properties. This entails that the changes accompanied by physical aging are not permanent and can be reversed at some point.
Figure 1 (a) Schematic showing a typical glass transition phenomena of an un-aged material and (b) schematic of an aged sample showing the onset, inflection, and end temperatures for the glass transition. ΔQ is the jump in heat flow during the glass transition, and the hatched area ‘A’ (in (b)) reflects the endothermic heat flow associated with the enthalpy relaxation of an aged sample (adapted from McKenna and Simon;[Citation21] Fukuoka et al.[Citation14]).
![Figure 1 (a) Schematic showing a typical glass transition phenomena of an un-aged material and (b) schematic of an aged sample showing the onset, inflection, and end temperatures for the glass transition. ΔQ is the jump in heat flow during the glass transition, and the hatched area ‘A’ (in (b)) reflects the endothermic heat flow associated with the enthalpy relaxation of an aged sample (adapted from McKenna and Simon;[Citation21] Fukuoka et al.[Citation14]).](/cms/asset/67b1d58b-cc75-493c-9e35-4a9de03c6c55/ljfp_a_710287_o_f0001g.gif)
Physical aging of various food ingredients have been investigated, including gelatin,[Citation29,Citation30] lactose,[Citation31] sucrose,[Citation32] maltose,[Citation17,Citation24] the mixtures of glucose and fructose[Citation33,Citation34] and starch,[Citation3,Citation35–Citation42] and foods themselves.[Citation20] Other studies have been conducted to examine, explain, and model the enthalpic aging process in polymer glasses.[Citation13,Citation16,Citation25,Citation43–Citation52] Several researchers have reported on the relaxation and/or crystallization of an amorphous material below its glass transition temperature.[Citation53–Citation55] Considering synthetic polymers, it has been demonstrated that enthalpic events commonly occur near the glass transition,[Citation56] although for starches and some other synthetic polymers, sub-Tg endotherms have been illustrated.[Citation42,Citation57]
In regards to pharmaceuticals, Yoshioka et al.[Citation55] showed that dry amorphous indomethacin crystallizes completely within 3 weeks at temperatures as low as 17°C below the glass transition temperature, while Hancock et al.[Citation54] demonstrated that indomethacin, sucrose, and poly-vinylpyrrolidone (PVP) exhibited enthalpy relaxation or physical aging at temperatures 47°C below Tg . Haque et al.[Citation31] studied the glass transition and enthalpy relaxation of amorphous lactose prepared by melt quench cooling and aged at 25, 60, 75, and 90°C for periods ranging from 2 to 120 h.
The schematic curves shown in illustrate the change in enthalpy of a given material as a function of temperature for aged and un-aged samples, which can be observed during heating or cooling cycles in a DSC instrument. Thus, based on the principles of thermal analysis, enthalpy relaxation can be calculated as the difference in area between the thermograms around the glass transition temperature of the aged and un-aged samples. This can be seen as the hatched area designated as ‘A’ in .[Citation21,Citation24,Citation58] In addition, the choice of the integration limits can influence the value of ΔH, thus the selection of these limits should be done cautiously and reasonably so as to overlap the transition phenomenon.[Citation46]
Figure 2 Schematic diagram showing the change in enthalpy of a given material as a function of temperature for aged and un-aged samples. Path A-B-C-B-A represents that of the un-aged sample, whereas the aged path is given as A-B-C-D-E-B-A (adapted from Schmidt and Lammert[Citation24]).
![Figure 2 Schematic diagram showing the change in enthalpy of a given material as a function of temperature for aged and un-aged samples. Path A-B-C-B-A represents that of the un-aged sample, whereas the aged path is given as A-B-C-D-E-B-A (adapted from Schmidt and Lammert[Citation24]).](/cms/asset/918d05c1-a7ad-46ac-a7e3-6d639f686103/ljfp_a_710287_o_f0002g.gif)
Spray drying is considered to be an important process, which is commonly used in the pharmaceutical industry to produce amorphous solids.[Citation59] However, the nature of the process can create internal stresses within the dried product due to variations in moisture migration as a function of the different drying rates.[Citation60] The inlet air temperature plays an important role in the drying process and it is a critical factor in affecting the properties of the dried product. The high temperature of the surrounding air creates a temperature gradient in the formed droplet affecting its water content, and creating a moisture gradient in the droplet. Both temperature and moisture gradients inside the droplet influence the particle formation and properties as well as the residual water content and structure of the spray dried powder. As a result, during the drying process, the diffusion coefficient (i.e., water-vapor diffusion) decreases and overheating may occur. In fact, under certain extreme conditions, a hard crust may form on the surface of the dried material slowing the rate of water diffusion and, hence, increasing the particle temperature as the evaporation rate approaches zero.[Citation60,Citation61]
The feeding rate and the feed concentration are also key factors influencing the spray drying process. An increase in feeding rate produces coarser particles and may result in inefficient drying; likewise, increasing the solid content in the feed results in coarse sprayed droplets upon atomization. However, an increase in feed temperature, that reduces the viscosity of the feed, may decrease the droplet size produced. The influence of spray-dried feed concentration on the degree of crystallinity and the crystal form of lactose (β-lactose, anhydrous α-lactose, α-lactose monohydrate) has been previously studied.[Citation60] Variations in feed concentration during spray drying result in products having different amounts of amorphous and crystalline structures; the higher the feed concentration the more crystalline the material will be.[Citation60] In addition, the impact of the feed temperature as well as the conditions used to induce crystallization of spray dried lactose was formerly investigated.[Citation62] A study on the effect of varying the inlet air temperature over the range from 134 to 210°C showed an increase in crystallinity of spray-dried lactose powders.[Citation63–Citation65] Thus, understanding the effects of the various operating variables can result in a product that possesses the desired properties, stability, and quality.[Citation60]
Although extensive research has been done on spray drying of lactose and other materials, the effect of processing and subsequent storage conditions on the physicochemical properties and physical aging of the processed materials have not been assessed. This study explores the effect of feed concentration, inlet temperatures, and storage conditions on the physicochemical properties, including enthalpy relaxation, residual moisture, as well as the structural and physical forms of spray dried lactose.
METHODOLOGY
Materials
α-Lactose monohydrate purchased from Foremost (Rothschild, Wisconsin), lot #8501071210, was used as purchased without any further modifications to prepare the solutions for spray drying. Solutions of concentrations: 10 and 20% w/v in distilled water were prepared—given the solubility of lactose as 21 g/100 ml at 25°C.[Citation66] The lactose-water mixtures were stirred continuously over a hot plate until a clear solution was formed.
Methods
Spray drying
The α-lactose solutions at the given concentrations were spray dried using a pilot plant scale, Anhydro Spray Dryer Type PSD 52 (Anhydro, Soeborg, Denmark). Spray drying was conducted at three different inlet temperatures of 120, 160, and 190°C. The outlet temperature, which is dependent on feed concentration, was monitored throughout the drying process and varied from 73 to 94°C. The feed rate was kept constant at 20 ml/min and the atomizer speed was set at the maximum speed of 49,000 rpm for efficient liquid atomization. The drier is furnished with a 50-mm vaned wheel atomizer, a 1.4-m-diameter drying chamber with a cylindrical height of 2.4 m and a 60° conical base. The spray dried powders were tested within a few hours of preparation, while some samples were equilibrated under different environmental conditions prior to testing in order to investigate the effect of such conditions on their thermal properties. The storage conditions include: (a) Drierite® desiccators (0% RH) at 25°C for 24 h, (b) vacuum oven at 60°C for 24 h, and (c) vacuum oven at 90°C for 6 h.
Moisture analysis
Moisture analysis was done using AMB 110 moisture balance (Adam Equipment Co. Ltd., UK). The moisture was calculated as percentage of initial mass at a preselected temperature of 120°C and strobe time of 15 s as recommended by the manufacturer.
Differential scanning calorimetry
All samples were tested using both conventional and temperature modulated (DSC and MDSC). Approximately 4 ± 1 mg of the spray dried samples were placed in aluminum pans and scanned at 5°C/min in a differential scanning calorimeter model Q2000 (TA Instruments, New Castle, DE, USA) under a nitrogen gas flow of 50 cc/min. The temperature scan range was set from 0 to 220°C. As for the MDSC, the underlying heating rate was set to 5°C/min from 0 to 150°C with modulation amplitude of 1.0°C and a period of 100 s. The DSC cell was calibrated for baseline using empty pans of matched weights, whereas for temperature, heat capacity, and cell constant, sapphire discs and indium standards were used for calibration. MDSC was used to measure the change in heat capacities at the glass transition temperature as well as the enthalpy relaxation
, enthalpy of crystallization
, glass transition and crystallization temperatures,
and
, respectively. The change in heat capacity at the glass transition temperature
can be defined as the change in heat capacity between the liquid and the un-aged quenched glass at Tg
given as:
The enthalpy recovery (ΔH max) observed shows that the endothermic peak associated with glass transition increases with aging time towards the equilibrium limit of the super-cooled liquid at the specified aging temperature:[Citation67]
The value of ΔCp at Tg provides a relative measure of accessible molecular conformations at the material glass transition region and thus can be used to predict the crystallization tendency of a given material. Consequently, in order to facilitate the comparison between the different powders, a reduced crystallization temperature can be defined as:[Citation10]
RESULTS AND DISCUSSION
The average melting temperature of lactose was around 216°C and was associated with an enthalpy of fusion, ΔHm , of 165 J/g. The moisture content of all spray dried samples is summarized in . According to the MDSC scans, the heat capacity of crystalline lactose did not vary with temperature away from the glass transition region whereas the heat capacity of amorphous lactose shows a step-change at the glass transition region (). Based on DSC and MDSC results, the differences between the heat capacity of the material at the liquid and the glass states at the transition region were calculated for all samples and are presented in . In addition, the enthalpy relaxation ΔHrelax around the glass transition as well as the glass transition temperature Tg , the crystallization temperature TC , and the enthalpy of crystallization ΔHC were calculated using the Universal Analysis 2000 software (TA Instruments, New Castle, DE, USA) and are summarized in .
Table 1 Residual moisture determined after storage for each of the conditions
Figure 3 Heat capacity as a function of temperature for anhydrous lactose crystalline (continuous line) and amorphous (dotted line) produced by spray drying 10% w/v solution at 120°C inlet temperature.
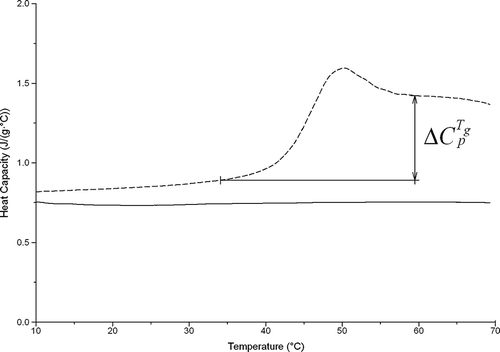
Table 2 Thermal properties of spray dried lactose samples as determined using differential scanning calirometry
and show MDSC scans indicating reversing and non-reversing heat flows of lactose spray dried at different temperatures (120, 160, and 190°C) for feed concentrations of 10 and 20% w/v. The glass transition can be clearly observed as it is associated with the reversing portion of the heat flow, while the enthalpy of relaxation is related to the non-reversing heat flow. An increase in Tg is clearly observed with increasing inlet temperatures. As evidenced from results shown in , the glass transition temperature tends to increase with increasing storage as well as inlet drying temperatures. It is worth mentioning that the increase in Tg values with higher inlet temperature may be attributed to the probable formation of rigid glasses during the spray drying process.
Figure 4 MDSC data for 10% w/v spray dried lactose solution dried at (a) 120, (b) 160, and (c) 190°C. The graph shows the reversing (grey line) and non-reversing (black line) heat flows.
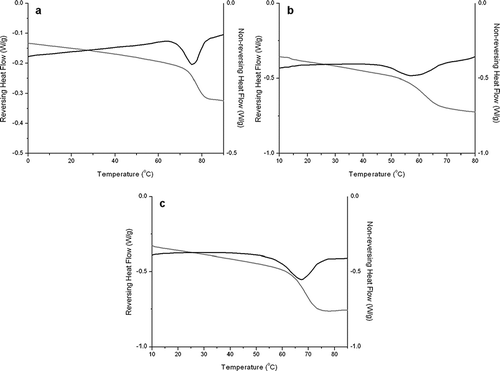
Figure 5 MDSC data for the 20% w/v spray dried lactose solution spray dried at 120°C, the graph shows the reversing (grey line) and non-reversing (black line) heat flows for: (a) no conditioning, (b) stored at 0% RH and 25°C for 24 h, (c) stored in vacuum at 60°C for 24 h, and (d) stored in vacuum at 90°C for 24 h.
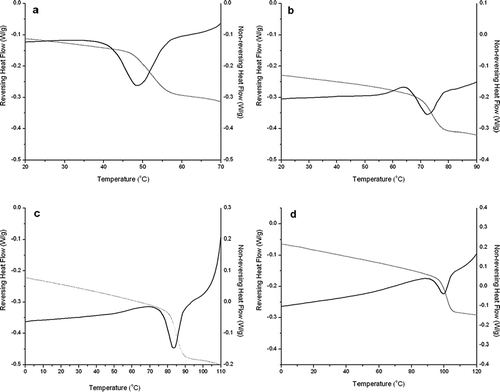
The storage conditions for 20% feed concentrations had an effect on the material properties in addition to those induced by the processing conditions; however, for this feed concentration there was a more apparent increase in both the glass transition and crystallization temperatures, which was not reflected in the measured values of enthalpy relaxation . For both concentrations of lactose, spray drying under the various conditions tested in this work, ΔHrelax
values presented in clearly show that the samples relaxed during the spray drying process. This is further illustrated by the non-significant change in enthalpy observed in the stored samples. This suggests that enthalpy recovery was a result of the processing and not caused by the storage conditions under the given tested conditions. Conversely, with the spray dried powder obtained from the 20% feed solution and processed at 190°C, the change in relaxation enthalpy increased with the varying storage conditions.
Table 3 Enthalpies of crystallization for amorphous lactose using isothermal microcalorimetry at 25°C as reported in the literature
In addition, an increase in crystallization temperature can be observed with varying storage conditions as well as an increase in Tg
accompanied by a slight increase in , but no significant change was observed in enthalpy of crystallization
among samples spray dried at the same temperature. For comparison, represents reported apparent enthalpies of crystallization for lactose determined using isothermal microcalorimetry at 25°C.[Citation60,Citation70–Citation72] In order to facilitate the comparison among the crystallization tendencies of the various samples, the change in enthalpy at Tg
as well as the reduced crystallization temperature
were calculated () as defined by Zhou et al.[Citation10] Examining both
and
, it is clear that there is a relationship between the reduced crystallization temperature, the change in enthalpy at Tg
, and the crystallization tendencies of the spray dried powder. This was reflected in the changes of glass transition and crystallization temperatures.
CONCLUSION
The results of the present study clearly show that varying the spray drying and storage conditions can produce different structures in the material, which can be determined by measuring the degree of relaxation in the dried product. This is evident in the change in glass transition and crystallization temperatures as well as the change of heat capacities at the glass transition region. It appears that the initial relaxation, which occurred during spray drying, affected the crystallization tendency of the produced dried powders. One may also look at these results with a different perspective; that is, the lower the molecular mobility, the higher the Tg and crystallization temperatures of the material. The lesser is the difference in heat capacities between the liquid and glass forms, the more stable the material will be. In addition, mechanical or thermal stress along with process-induced variations may impact the overall crystallization process making the interpretation of results more difficult. Results obtained in this work are showing that physicochemical properties changes of a material during aging is not only a result of storage conditions but also a product of the processing environment. Consequently, variations in spray drying conditions must be monitored and controlled as they impact nucleation and crystal growth.
ACKNOWLEDGMENT
This work was funded by NSF IUCRC 000364-EEC, the Dane O. Kildsig Center for Pharmaceutical Processing Research and the University Research Board at the American University of Beirut.
REFERENCES
- Bhandari , B.R. and Howes , T. 1999 . Implication of glass transition for the drying and stability of dried foods . Journal of Food Engineering , 40 ( 1–2 ) : 71 – 79 .
- Broadhead , J. , Edmond Rouan , S.K. and Rhodes , C.T. 1992 . The spray drying of pharmaceuticals . Drug Development and Industrial Pharmacy , 18 ( 11 ) : 1169 – 1206 .
- Kim , Y.J. , Suzuki , T. , Hagiwara , T. , Yamaji , I. and Takai , R. 2001 . Enthalpy relaxation and glass to rubber transition of amorphous potato starch formed by ball-milling . Carbohydrate Polymers , 46 ( 1 ) : 1 – 6 .
- Le Meste , M. , Champion , D. , Roudaut , G. , Blond , G. and Simatos , D. 2002 . Glass transition and food technology: A critical appraisal . Journal of Food Science , 67 ( 7 ) : 2444 – 2458 .
- Kim , J.-S. , Kim , M.-S. , Park , H.J. , Jin , S.-J. , Lee , S. and Hwang , S.-J. 2008 . Physicochemical properties and oral bioavailability of amorphous atorvastatin hemi-calcium using spray-drying and SAS process . International Journal of Pharmaceutics , 359 ( 1–2 ) : 211 – 219 .
- Yang , W. , Johnston , K.P. and Williams , III . 2010 . R.O. Comparison of bioavailability of amorphous versus crystalline itraconazole nanoparticles via pulmonary administration in rats . European Journal of Pharmaceutics and Biopharmaceutics , 75 ( 1 ) : 33 – 41 .
- Fakes , M.G. , Vakkalagadda , B.J. , Qian , F. , Desikan , S. , Gandhi , R.B. , Lai , C. , Hsieh , A. , Franchini , M.K. , Toale , H. and Brown , J. 2009 . Enhancement of oral bioavailability of an HIV-attachment inhibitor by nanosizing and amorphous formulation approaches . International Journal of Pharmaceutics , 370 ( 1–2 ) : 167 – 174 .
- Onoue , S. , Sato , H. , Ogawa , K. , Kawabata , Y. , Mizumoto , T. , Yuminoki , K. , Hashimoto , N. and Yamada , S. 2010 . Improved dissolution and pharmacokinetic behavior of cyclosporine A using high-energy amorphous solid dispersion approach . International Journal of Pharmaceutics , 399 ( 1–2 ) : 94 – 101 .
- Hancock , B.C. and Zografi , G. 1997 . Characteristics and significance of the amorphous state in pharmaceutical systems . Journal of Pharmaceutical Sciences , 86 ( 1 ) : 1 – 12 .
- Zhou , D. , Zhang , G.G.Z. , Law , D. , Grant , D.J.W. and Schmitt , E.A. 2002 . Physical stability of amorphous pharmaceuticals: Importance of configurational thermodynamic quantities and molecular mobility . Journal of Pharmaceutical Sciences , 91 ( 8 ) : 1863 – 1872 .
- Abiad , M.G. , Carvajal , M.T. and Campanella , O.H. 2009 . A review on methods and theories to describe the glass transition phenomenon: Applications in food and pharmaceutical products . Food Engineering Reviews , 1 ( 2 ) : 105 – 132 .
- Liu , Y. , Bhandari , B. and Zhou , W. 2006 . Glass transition and enthalpy relaxation of amorphous food saccharides: A review . Journal of Agricultural and Food Chemistry , 54 ( 16 ) : 5701 – 5717 .
- Struik , L.C.E. 1978 . Physical Aging in Amorphous Polymers and Other Materials , Amsterdam, the Netherlands : Elsevier Scientific Pub. Co .
- Fukuoka , E. , Makita , M. and Yamamura , S. 1986 . Some physicochemical properties of glassy indomethacin . Chemical & Pharmaceutical Bulletin , 34 ( 10 ) : 4314 – 4321 .
- Bidault , O. , Assifaoui , A. , Champion , D. and Le Meste , M. 2005 . Dielectric spectroscopy measurements of the sub-Tg relaxations in amorphous ethyl cellulose: A relaxation magnitude study . Journal of Non-Crystalline Solids , 351 ( 14–15 ) : 1167 – 1178 .
- Cowie , J.M.G. and Ferguson , R. 1993 . Physical aging of poly(methyl methacrylate) from enthalpy relaxation measurements . Polymer , 34 ( 10 ) : 2135 – 2141 .
- Lammert , A. , Lammert , R. and Schmidt , S. 1999 . Physical aging of maltose glasses as measured by standard and modulated differential scanning calorimetry . Journal of Thermal Analysis and Calorimetry , 55 ( 3 ) : 949 – 975 .
- Montserrat , S. , Calventus , Y. and Hutchinson , J.M. 2005 . Effect of cooling rate and frequency on the calorimetric measurement of the glass transition . Polymer , 46 ( 26 ) : 12181 – 12189 .
- Struik , L.C.E. 1980 . The mechanical enhancement of physical aging . Polymer , 21 ( 8 ) : 962 – 967 .
- Gonzalez , D.C. , Khalef , N. , Wright , K. , Okos , M.R. , Hamaker , B.R. and Campanella , O.H. 2010 . Physical aging of processed fragmented biopolymers . Journal of Food Engineering , 100 ( 2 ) : 187 – 193 .
- McKenna , G.B. and Simon , S.L. 2002 . “ The glass transition: Its measurement and underlying physics ” . In Handbook of Thermal Analysis and Calorimetry , Edited by: Cheng , S.Z.D. 49 – 109 . Amsterdam, the Netherlands : Elsevier .
- Struik , L.C.E. 1987 . Effect of thermal history on secondary relaxation processes in amorphous polymers . Polymer , 28 ( 1 ) : 57 – 68 .
- Struik , L.C.E. 1987 . Volume relaxation and secondary transitions in amorphous polymers . Polymer , 28 ( 11 ) : 1869 – 1875 .
- Schmidt , S.J. and Lammert , A.M. 1996 . Physical aging of maltose glasses . Journal of Food Science , 61 ( 5 ) : 870 – 875 .
- Agrawal , A. 1989 . Effect of temperature and molecular weight on enthalpy relaxation in polystyrene . Journal of Polymer Science, Part B: Polymer Physics Edition , 27 : 1449 – 1461 .
- Perera , D.Y. 2003 . Physical ageing of organic coatings . Progress in Organic Coatings , 47 ( 1 ) : 61 – 76 .
- Hilden , L.R. and Morris , K.R. 2003 . Prediction of the relaxation behavior of amorphous pharmaceutical compounds . I. Master curves concept and practice. Journal of Pharmaceutical Sciences , 92 ( 7 ) : 1464 – 1472 .
- Hutchinson , J.M. 1995 . Physical aging of polymers . Progress in Polymer Science , 20 ( 4 ) : 703 – 760 .
- Badii , F. , MacNaughtan , W. and Farhat , I.A. 2005 . Enthalpy relaxation of gelatin in the glassy state . International Journal of Biological Macromolecules , 36 ( 4 ) : 263 – 269 .
- Rahman , M.S. and Al-Saidi , G.S. 2010 . Thermal relaxation of gelatin and date flesh measured by isothermal condition in differential scanning calorimetry (DSC) and its relation to the structural and mechanical glass transition . International Journal of Food Properties , 13 ( 4 ) : 931 – 944 .
- Haque , M.K. , Kawai , K. and Suzuki , T. 2006 . Glass transition and enthalpy relaxation of amorphous lactose glass . Carbohydrate Research , 341 ( 11 ) : 1884 – 1889 .
- Sahagian , M.E. and Goff , H.D. 1995 . Thermal, mechanical and molecular relaxation properties of stabilized sucrose solutions at sub-zero temperatures . Food Research International , 28 ( 1 ) : 1 – 8 .
- Fan , J. and Angell , C.A. 1995 . Relaxational transitions and ergodicity breaking within the fluid state: The sugars fructose and galactose . Thermochimica Acta , 266 : 9 – 30 .
- Wungtanagorn , R. and Schmidt , S.J. 2001 . Phenomenological study of enthalpy relaxation of amorphous glucose, fructose, and their mixture . Thermochimica Acta , 369 ( 1–2 ) : 95 – 116 .
- Chung , H.-J. , Woo , K.-S. and Lim , S.-T. 2004 . Glass transition and enthalpy relaxation of cross-linked corn starches . Carbohydrate Polymers , 55 ( 1 ) : 9 – 15 .
- Chung , H.-J. , Lee , E.-J. and Lim , S.-T. 2002 . Comparison in glass transition and enthalpy relaxation between native and gelatinized rice starches . Carbohydrate Polymers , 48 ( 3 ) : 287 – 298 .
- Chung , H.-J. and Lim , S.-T. 2003 . Physical aging of glassy normal and waxy rice starches: Effect of aging temperature on glass transition and enthalpy relaxation . Carbohydrate Polymers , 53 ( 2 ) : 205 – 211 .
- Chung , H.-J. , Chang , H.-I. and Lim , S.-T. 2004 . Physical aging of glassy normal and waxy rice starches: Effect of crystallinity on glass transition and enthalpy relaxation . Carbohydrate Polymers , 58 ( 2 ) : 101 – 107 .
- Lourdin , D. , Colonna , P. , Brownsey , G.J. , Noel , T.R. and Ring , S.G. 2002 . Structural relaxation and physical ageing of starchy materials . Carbohydrate Research , 337 ( 9 ) : 827 – 833 .
- Shogren , R.L. 1992 . Effect of moisture content on the melting and subsequent physical aging of cornstarch . Carbohydrate Polymers , 19 ( 2 ) : 83 – 90 .
- Thiewes , H.J. and Steeneken , P.A.M. 1997 . The glass transition and the sub-Tg endotherm of amorphous and native potato starch at low moisture content . Carbohydrate Polymers , 32 ( 2 ) : 123 – 130 .
- Yuan , R.C. and Thompson , D.B. 1994 . Sub-Tg thermal properties of amorphous waxy starch and its derivatives . Carbohydrate Polymers , 25 ( 1 ) : 1 – 6 .
- Bailey , N.A. , Hay , J.N. and Price , D.M. 2001 . “ A study of enthalpic relaxation of poly(ethylene terephthalate) by conventional and modulated temperature DSC ” . In Thermochimica Acta 425 – 431 . 367–368
- Brunacci , A. , Cowie , J.M.G. , Ferguson , R. and McEwen , I.J. 1997 . Enthalpy relaxation in glassy polystyrenes: 1 . Polymer , 38 ( 4 ) : 865 – 870 .
- Brunacci , A. , Cowie , J.M.G. , Ferguson , R. and McEwen , I.J. 1997 . Enthalpy relaxation in glassy polystyrenes: 2 . Polymer , 38 ( 13 ) : 3263 – 3268 .
- Cowie , J.M.G. and Ferguson , R. 1989 . Physical aging studies in poly(vinylmethyl ether) . I. Enthalpy relaxation as a function of aging temperature. Macromolecules , 22 ( 5 ) : 2307 – 2312 .
- Cowie , J.M.G. and Ferguson , R. 1989 . Physical aging studies in polymer blends. 2. Enthalpy relaxation as a function of aging temperature in a poly(vinyl methyl ether)/polystyrene blend . Macromolecules , 22 ( 5 ) : 2312 – 2317 .
- Hodge , I.M. and Berens , A.R. 1982 . Effects of annealing and prior history on enthalpy relaxation in glassy polymers. 2. Mathematical modeling. Macromolecules , 15 ( 3 ) : 762 – 770 .
- Hodge , I.M. and Huvard , G.S. 1983 . Effects of annealing and prior history on enthalpy relaxation in glassy polymers . 3. Experimental and modeling studies of polystyrene. Macromolecules , 16 : 371 – 375 .
- Pappin , A.J. , Hutchinson , J.M. and Ingram , M.D. 1992 . Enthalpy relaxation in polymer glasses: Evaluation and interpretation of the Tool-Narayanaswamy parameter x for poly(vinyl chloride) . Macromolecules , 25 ( 3 ) : 1084 – 1089 .
- Struik , L.C.E. 1987 . The mechanical and physical ageing of semicrystalline polymers: 1 . Polymer , 28 ( 9 ) : 1521 – 1533 .
- Struik , L.C.E. 1987 . The mechanical behaviour and physical ageing of semicrystalline polymers: 2 . Polymer , 28 ( 9 ) : 1534 – 1542 .
- Chryssikos , G.D. , Kamitsos , E.I. , Bitsis , M.S. and Patsis , A.P. 1991 . Chemical relaxations at the glass transition of a lithium conducting glass . Journal of Non-Crystalline Solids , 131–133 ( Part 2 ) : 1068 – 1071 .
- Hancock , B.C. , Shamblin , S.L. and Zografi , G. 1995 . Molecular mobility of amorphous pharmaceutical solids below their glass transition temperatures . Pharmaceutical Research , 12 ( 6 ) : 799 – 806 .
- Yoshioka , M. , Hancock , B.C. and Zografi , G. 1994 . Crystallization of indomethacin from the amorphous state below and above its glass transition temperature . Journal of Pharmaceutical Sciences , 83 ( 12 ) : 1700 – 1705 .
- Sperling , L.H. 2006 . Introduction to Physical Polymer Science , 4th , New Jersey : John Wiley & Sons, Inc. .
- Hodge , I.M. and Berens , A.R. 1981 . Calculation of the effects of annealing on Sub-Tg endotherms . Macromolecules , 14 ( 5 ) : 1598 – 1599 .
- Fukuoka , E. , Makita , M. and Yamamura , S. 1987 . Glassy state of pharmaceuticals . II. Bioinequivalence of glassy and crystalline indamethacin (pharmaceutical). Chemical & Pharmaceutical Bulletin , 35 ( 7 ) : 2943 – 2948 .
- Sebhatu , T. , Angberg , M. and Ahlneck , C. 1993 . Assessment of the degree of disorder in crystalline solids by isothermal microcalorimetry . International Journal of Pharmaceutics , 104 : 135 – 144 .
- Chidavaenzi , OC. , Buckton , G. , Koosha , F. and Pathak , R. 1997 . The use of thermal techniques to assess the impact of feed concentration on the amorphous content and polymorphic forms present in spray dried lactose . International Journal of Pharmaceutics , 159 ( 1 ) : 67 – 74 .
- 2009 . Niro-GEA. One-stage drying Soeborg, Denmark
- Buckton , G. , Chidavaenzi , O.C. and Koosha , F. 2002 . The effect of spray-drying feed temperature and subsequent crystallization conditions on the physical form of lactose . AAPS PharmSciTech , 3 ( 4 ) : 1 – 6 .
- Chiou , D. and Langrish , T.A.G. 2008 . A comparison of crystallisation approaches in spray drying . Journal of Food Engineering , 88 ( 2 ) : 177 – 185 .
- Chiou , D. , Langrish , T.A.G. and Braham , R. 2008 . The effect of temperature on the crystallinity of lactose powders produced by spray drying . Journal of Food Engineering , 86 ( 2 ) : 288 – 293 .
- Chiou , D. , Langrish , T.A.G. and Braham , R. 2008 . Partial crystallization behavior during spray drying: Simulations and experiments . Drying Technology , 26 ( 1 ) : 27 – 38 .
- Whittier , E.O. 1944 . Lactose and its utilization: A review . Journal of Dairy Science , 27 ( 7 ) : 505 – 537 .
- Biddlestone , F. , Goodwin , A.A. , Hay , J.N. and Mouledous , G.A.C. 1991 . The relaxation spectrum and physical ageing of polyetherimide . Polymer , 32 ( 17 ) : 3119 – 3125 .
- Montserrat , S. 1994 . Physical aging studies in epoxy resins . I. Kinetics of the enthalpy relaxation process in a fully cured epoxy resin. Journal of Polymer Science, Part B: Polymer Physics , 32 ( 3 ) : 509 – 522 .
- Graeser , K.A. , Patterson , J.E. , Zeitler , J.A. , Gordon , K.C. and Rades . 2009 . Correlating thermodynamic and kinetic parameters with amorphous stability . European Journal of Pharmaceutical Sciences , 37 ( 3–4 ) : 492 – 498 .
- Sebhatu , T. , Angberg , M. and Ahlneck , C. 1994 . Assessment of the degree of disorder in crystalline solids by isothermal microcalorimetry . International Journal of Pharmaceutics , 104 ( 2 ) : 135 – 144 .
- Briggner , L.E. , Buckton , G. , Bystrom , K. and Darcy , P. 1994 . The use of isothermal microcalorimetry in the study of changes in crystallinity induced during the processing of powders . International Journal of Pharmaceutics , 105 ( 2 ) : 125 – 135 .
- Darcy , P. and Buckton , G. 1998 . Quantitative assessments of powder crystallinity: Estimates of heat and mass transfer to interpret isothermal microcalorimetry data . Thermochimica Acta , 316 ( 1 ) : 29 – 36 .