Abstract
Model systems of egg white protein and green coffee extract were dry heated at 110 or 180oC for up to 1 h to investigate the extent of interactions between coffee polyphenols and a protein of high nutritional significance. Saccharose, starch, sunflower oil, and ferrous ions were also applied to the model mixtures. In heated and control systems amino acid profiles, in vitro pepsin digestibility, color, and antioxidant properties were analyzed. Among heated samples, high protein nutritional value was obtained in the system containing 3% of green coffee extract, while the least favorable conditions for maintaining protein high nutritional value were observed in system of protein, saccharose, starch, and sunflower oil without addition of coffee polyphenols. The samples of high protein nutritional value were also characterized by high antioxidant activity.
INTRODUCTION
Currently implemented strategies of food research and development include, among others, innovative technologies for obtaining products with pro-health characteristics. New products are often enriched with proteins or protein hydrolysates, which have high nutritional value and potentially reduced allergenicity and, from the other side, with plant extracts containing polyphenols with high antioxidant activity. In this type of food, interactions between polyphenols and proteins would inevitably occur.[Citation1]
Green coffee contains up to 10% of polyphenols, mostly chlorogenic and caffeic acids, which make it an extremely rich source of polyphenols.[Citation2] In recent years, supplementation of food with coffee began to be perceived not only as an antioxidant of food lipids, but also as a part of chronic disease prevention.[Citation3,Citation4] But besides beneficial health effects, polyphenols affect the metabolism of proteins on a molecular level through the formation of adducts in reactions with proteins in food and in the gastrointestinal tract, furthermore, they can modify the expression and activity of enzymes and they can reduce availability of proteins from food.[Citation5] Interactions can occur during storage of raw materials, food processing, digestion, after absorption into plasma, and finally in human organs.[Citation6,Citation7] Factors affecting the interactions of proteins with polyphenols are: The structure of protein, amino acid sequence and spatial conformation, the type of polyphenol, and external conditions such as pH, temperature, and ionic strength.[Citation8]
Two main directions of interaction of polyphenols with proteins are the formation of covalent and non-covalent bonds.[Citation9] Covalent bonds are formed through the stage of oxidized products - quinones in the reactions of enzymatic and non-enzymatic browning. Quinone radicals are electrophilic molecules, and are subject to nucleophilic additions with the reactive side chains of proteins, mainly with lysine, cysteine, methionine, and tryptophan.
In addition to the covalent interactions, non-covalent interactions also occur. These include hydrogen, hydrophobic, and ionic bonds. Proline moieties hydrophobically interact with polyphenols. Hydrogen bonds are formed between the phenolic hydroxyl group and the peptide bond of protein, and they can be very strong. They are also formed between the hydroxyl groups of aromatic ring and alkaline protein groups, mainly arginine and lysine. It was found that at pH 9 mainly hydrogen and covalent bonds, at pH 5 hydrophobic bonds, and at pH 7 ionic bonds are formed. Carboxyl group of chlorogenic acid is ionized at neutral pH. Electrostatic interactions occur between it and still positively charged groups in protein side chains, such as lysine and ionic bonding may then be formed.[Citation5]
Depending on the structure of polyphenol and protein, interactions between them may decrease or increase the digestibility of protein. There is also a bidirectional influence on the antioxidant activity of polyphenols.[Citation10] The aim of this study was to exam the effect of interactions that occur during dry heating between egg white protein and green coffee extract (GCE) on amino acid profile, gastric digestibility, and antioxidant activity of heated systems.
MATERIAL AND METHODS
Materials and Reagents
Green Robusta coffee (Coffea canephora) beans originating from Brazil, dehulled by using the dry method, were used purchased from Bero Polska, (Gdynia, Poland). As a protein material, egg white protein was chosen because of its beneficial amino acids composition. Spray-dried chicken egg white protein was delivered by PPHU Japart (Opatów, Poland). Refined sunflower oil was provided by ZPT Kruszwica S.A. (Kruszwica, Poland), saccharose by Nordzücker S.A. (Opalenica, Poland), potato starch by Pepees S.A. (Łomża, Poland), FeCl2 by POCH S.A. (Gliwice, Poland). Standards of amino acids and 2,2-di(4-tert-octylphenyl)-1-picrylhydrazyl (DPPH) were supplied by Fluka (Mumbai, India), triethylamine by Fluka (Buchs, Switzerland), methanol by Chempur (Piekary Śląskie, Poland), phenyl isothiocyanate (PITC) by Sigma-Aldrich (Steinheim, Germany), fluoroacetic acid by Sigma-Aldrich (MO, USA), pepsin by Sigma-Aldrich (Dorset, UK), sodium acetate by Bioultra Sigma (Taufkirchen, Germany), acetic acid by Fisher Scientific (Loughborough, UK), and acetonitrile by JT Baker (NJ, USA).
Preparation of GCE
An aqueous extract of green coffee was prepared. Beans were ground and sieved to a particle size ranging from 480 to 680 μm. Then the extract was obtained using the ratio of ground coffee to the solvent 1:5.75, by boiling in a PS-5682 first pressure vessel (Vienne, Austria) at 110oC for 10 min, then rapid cooling and filtration under vacuum using a KNF Neuberger AT.18 035.3 N (NJ, USA) vacuum pump. The extraction conditions were consistent with earlier studies on obtaining a GCE of relatively high antioxidant activity. The extract was then freeze-dried in a DELTA 1-24LSC christ freeze drier (Osterode am Harz, Germany). The resulting lyophilisate had the following composition: Chlorogenic acids 37.6 g × 100 g−1, protein 14.5 g × 100 g−1, fat 0.7 g × 100 g−1, ash 11.9 g × 100 g−1, caffeine 6.3 g × 100 g−1, soluble fiber 6.8 g × 100 g−1, carbohydrates 19.9 g × 100 g−1.[Citation11] Ochratoxin A content was less than 0.4 μg × kg−1.
Model Systems Composition and Treatment
GCE was added to egg white protein at levels of 1.5 and 3%. The higher concentration was chosen based on the statement that 3 g of GCE, containing about 1 g of polyphenols and 100 g of protein, both represent recommended daily intake, so this ratio (3:100) is nutritionally beneficial. In model systems, in addition to egg white protein and GCE, saccharose, potato starch, and sunflower oil were also used. Ferrous ions were used in the experiment at a concentration typical for the fortification of food, and water in an amount of 3%. Larger addition of water caused a change in consistency from powder to a sticky paste and caused difficulties during blending, which was essential in the experiment, designed as a homogenous mixture of powder system in the whole process to perform dry heating. The resulting samples were heated at 110 or 180°C for up to 1 h in a horizontal retort equipped with a stirrer. Heating of systems containing saccharose was performed at temperature of 110oC because of its caramelization occurring at higher temperatures. Summary of the data for the preparation of particular systems is enclosed in .
Table 1 Composition of model systems and heating conditions
In Vitro Pepsin Digestibility of Egg White Protein
The protein model systems were analyzed for susceptibility to hydrolysis with pepsin.[Citation12] 10 mg of protein sample was dissolved in 800 μl of 0.1 M HCl solution, then 200 μl of pepsin (1 mg × ml−1, enzyme:substrate 1:50, v/v) was added and incubated at 35°C for 2 h in Reacti Therm I 18821 Thermo Scientific derivatizer (PA, USA). After 2 h the reaction was terminated by adding 100 μl of 20% trichloroacetic acid. Then 10 μl of the solution was injected to Dionex Ultimate 3000 UHPLC (CA, USA) using a C18 BioBasic, 5μm, 150 × 4.6 mm column. The used eluents were: A: 0.1% aqueous solution of trifluoroacetic acid, B: 0.1% solution of trifluoroacetic acid in acetonitile. Elution was performed using the following program: 0 - 10 min - 100% A, 10 - 43 min from 0 to 80% B with a linear gradient and a flow rate of 1 ml × min−1, the column was thermostated at 25°C in a TCC-3000 RS thermostat, detection was carried out with the use of UV DAD MWD-3000 RS detector at a wavelength of 215 nm. Typical chromatograms of egg white protein before and after pepsin digestion are shown on and . Peak areas from HPLC analysis allowed calculating the quantity of non-degraded by heating egg protein. It was calculated from the relation between peak areas of main protein fractions after and before heating (). Digestibility of protein was then established as a percent of hydrolyzed into peptides () non-degraded protein.
Amino Acid Profile
Amino acids quantitative analysis included pre-column derivatization after acid hydrolysis according to Elkin and Wasynczuk[Citation13] and Kwanyuen and Joseph.[Citation14] One mg of a sample was placed in a vial and 400 μl 6 M HCl containing 1% phenol (antioxidant agent) was added. Prepared samples were connected to a stream of nitrogen (TSSISG NG4-1 nitrogen generator AZ, USA) and a vacuum pump for 5 min. Hydrolysis was performed at 110°C for 24 h in Reacti Therm I 18821 Thermo Scientific derivatizer (PA, USA). After this vacuum drying under a nitrogen stream at room temperature until completely dry was done. After evaporation 150 μl of methanol:water:triethylamine 2:2:1 (v/v/v) buffer was introduced into vials. Vials were stirred vigorously through shaking, and next, added buffer was evaporated as above, this step was performed twice. Then the vials were supplemented with 150 μl methanol:water:triethylamine:PITC 7:1:1:1 (v/v/v/v) buffer, mixed and allowed to stand for 20 min. After the reaction, the buffer was evaporated as above. Next, 150 μl of methanol was added to the vials and samples were vacuum dried under nitrogen. Resulting residue was dissolved in 1 ml of 10 mM sodium acetate buffer in 6% acetic acid and centrifuged. The supernatant was filtered through a nylon syringe filter with a pore size of 0.20 μm in SPE JT Baker 12G (NJ, USA). Then the aliquot of 20 μl of the filtrate was injected to Dionex Ultimate 3000 UHPLC (CA, USA) using a BioBasic C18, 5 μm, 150 × 4.6 mm column. The used eluents were: A: 10 mM sodium acetate, pH 6.4, B: acetonitrile:water 6:4 (v/v). Elution was performed using the following linear solvent gradients: 95% A and 5% B at the start, to 80% A and 20% B at 5.5 min, 60% A and 40% B at 10 min, 100% B at 10.5 to 15 min, and 100% A at 18 min with flow rate of 1 ml × min−1. The column was thermostated at 30°C in a TCC-3000 RS thermostat, detection was performed with the use of UV-DAD MWD-3000 RS detector at a wavelength of 254 nm. Typical chromatograms of standard amino acids mixture and egg white protein amino acids sample are presented in and . Amino acid quantities were identified by comparing retention times of standard amino acids mixture with sample peaks and calculated on the basis of the equation of the calibration curve and also taking into account the loses of internal standard - norleucine and expressed as g × 100 g−1 of initial egg white protein content in model system.
Color Measurement
Color measurement was performed using the CIE L*a*b* system with a Minolta (Osaka, Japan) automatic colorimeter. Color parameters, i.e., the lightness of the color L*, color red a*and yellow b*, and dE value, equal to square root of [(dL*)2 + (da*)2 + (db*)2], characterizing the total change of all parameters were established.[Citation15]
Antioxidant Activity
Redox potential: A solution of a protein model system in distilled water (2 g × 100 g−1) ultasonificated at 25°C for 15 min was prepared. Redox potential was measured using a Schott CG 843 pH meter with a BlueLine 31 RX redox electrode (Mainz, Geramany).[Citation16]
DPPH radical: The test with radical was carried out according to a standard procedure,[Citation17] using aqueous solutions of each of the systems at concentrations of 0.02, 0.05, 0.1, and 0.2 g × 100 g−1. Solutions were ultasonificated at 25oC for 15 min. Each concentration (0.1 ml) was reacted with a methanolic solution (3.9 ml) of the radical (DPPH: methanol 5:100 w/v). Then the absorbance of filtered mixtures after 30 min of reaction at a wavelength of 517 nm (UV/VIS spectrophotometer U-2800 A, Hitachi, Tokyo, Japan) was measured. Next a calibration curve of the dependence of concentration of the model system and antioxidant activity was obtained. On that basis the concentration of the system at which the quantity of radical form was reduced by 50% (IC50) was calculated.
Statistical Analysis
Preparation of model egg white systems was performed in triplicate. Analysis of each of the replicates of received systems was performed twice. The results represent the average of six measurements. Statistical analysis included an assessment of the statistical significance of differences between the results at a confidence level p ≤ 0.05.
RESULTS AND DISCUSSION
Protein Stability, Amino Acid Composition, and In Vitro Pepsin Digestibility of Egg White Protein
In , peak signals from basic fractions of egg protein are presented. Individual fractions of this protein were not identified, although from other literature data,[Citation18] it can be assumed that the largest peak 4 regards ovalbumin. Minor peaks 1, 2, 3, and 5 regard probably such proteins as lysozym, ovomucoid, ovotransferrin, and ovoglobulin. The circumstance that has to be taken into account when evaluating the nutritional value of protein is its degradation during heating in the presence of other food ingredients. Therefore, the ratio of protein after heating to protein before heating as a non-degraded protein was established, according to changes in the sum of peak areas of main egg protein fractions ( – protein after heating). In all heated systems the amount of main egg protein fractions was decreasing by about 50 g × 100 g−1. It can be assumed that losses of proteins resulted either from aggregating into bigger molecules, or from hydrolysis of peptide bonds caused by high temperature. Both of these possibilities could lead to the lack of detection of products in range of molecular masses used for analysis. These observations are in accordance with results obtained by Fu et al.,[Citation19] who concluded that 10 min of heating of egg white in dry conditions in temperature of 176°C, depending on implemented protein test, caused a degradation from 37 to 81 g per 100 g of egg white protein. Heating of proteins with addition of GCE caused higher losses of protein than heating protein alone.
Figure 3 Total amino acids, protein content after heating, and pepsin in vitro digestibility of egg white protein in model systems with green coffee extract. Error bars show the relative standard deviation. Total amino acids are expressed on amount of protein added to the systems. Egg protein after heating means the amount of main protein fractions remaining after treatment determined by HPLC analysis. Egg white protein digestibility is a decrease of the amount of main protein fractions caused by 2 h pepsin digestibility determined by HPLC analysis.
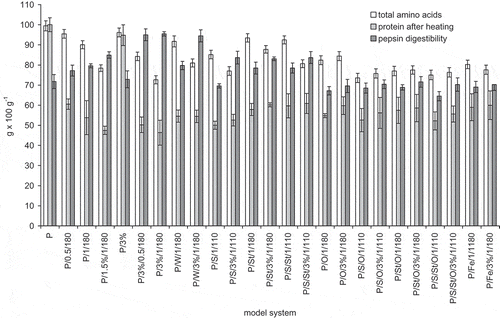
Protein content calculated from the sum of all assayed amino acids varied from 72.60 g × 100 g−1 in model system P/3%/1/180 to 99.42 g × 100 g−1 in fresh egg white protein ( – total amino acids). The smallest losses of total amino acid content occurred in systems in the following order: P/3%, P/0.5/180, P/St/1/180, P/S/St/1/110, P/W/1/180, and P/1/180. In these systems, the losses amounted to no more than 10% of the initial protein content. Amino acids losses of about 3 g × 100 g−1 were observed in protein system and 3% GCE, which was only stirred for the same amount of time, without heating. In this case, non-covalent interactions of proteins and coffee polyphenols are expected, and occurring losses regarded mostly cysteine. The biggest losses in amino acids content, amounting 25 – 27 g × 100 g−1, occurred after heating systems P/3%/1/180, P/S/O/1/110, and P/S/St/O/1/110, despite the fact, that for the last two systems, the applied heating temperature was lower.
Heating protein with GCE caused an overall larger degradation of amino acids compared to heating protein only. Protein heated in a mixture with starch showed lesser amino acid losses than heating just protein, when the same heating time is compared. This was probably due to the limited contact area of protein with heating medium, resulting from introducing another substance to the mixture, in this case starch, which is relatively stable in applied heating conditions. The addition of GCE to system of protein and starch also caused an increase in amino acid losses, but additional losses caused by GCE were smaller than in model systems containing protein and GCE only. Therefore, to some degree addition of starch could limit the interactions between proteins and GCE polyphenols. Heating system of proteins and saccharose, even in relatively low temperature of 110°C, caused significant losses in amino acid content, probably due to partial breakdown of saccharose to monosaccharides resulting in Maillard reactions with proteins. In model systems with saccharose, GCE addition also caused an increase in amino acid losses, but on the other hand saccharose decreased amino acid losses when compared with systems with protein and 3% addition of GCE. Similar results were obtained by Charurin et al.,[Citation20] who analyzed changes in concentrations of chlorogenic acid, saccharose, and arginine (N-acetylated) in heated (240°C, 4 min) in model systems. Chlorogenic acid caused an increase in arginine and saccharose degradation, comparing to systems without the polyphenol, this was especially noticeable for arginine. Like in this study, saccharose retarded the degradation of amino acid in system containing chlorogenic acid and arginine. Similarly, following an example of saliva protein and tannins, a decrease in bonding possibilities of protein and polyphenol was proven, when glucose was present in the system.[Citation21] Lysine is one of the main substrates in peptide chains Maillard reactions, and is also one of amino acids, which can easily react with chlorogenic acid.[Citation8] When comparing systems of egg white protein with saccharose, starch, and sunflower oil, the biggest losses in lysine content were noticed in a system with saccharose. It can back up the theory of an influence of both: Saccharose and GCE polyphenols on increased degradation of this amino acid during heating.
Interesting results were obtained when egg white protein was heated with sunflower oil. In a mixture of protein and oil greater amino acid losses were observed than in heated mixtures of protein and starch, or protein and saccharose, which were probably caused by lipid oxidation. Peroxide radicals, created by unsaturated fatty acids oxidation, could cause greater amino acid oxidation. In this instance, the addition of GCE contributed to a decrease in amino acid losses. It can be assumed that this effect was caused by limiting lipid oxidation, but fatty acid composition in model systems will be established in further experiments. Preparatory analysis seems to confirm this statement. Polyphenols counteract lipid oxidation, in which oxidation reactions of unsaturated fatty acids could easily transfer from lipids to proteins. Due to that property, polyphenols can limit protein oxidation in complex products, which are susceptible to fatty acids oxidation, such as: mayonnaises, cheeses, diary, and bakery products.[Citation22,Citation23] Similar tendency was noticed in all studied systems containing oil.
A pro-oxidation factor to heated protein was an addition of Fe2+ ions. In this instance, amino acid losses were even greater than when heated with sunflower oil. They increased even more in the presence of GCE (p < 0.05). These results contradict some of previously performed analysis, for example, when the addition of polyphenol rich plant extracts to sausages prevented amino acids oxidation during boiling and storage.[Citation24] Although plant polyphenols can impair oxidative degradation of proteins by hindering pro-oxidative effect of transitional metal cations, as a result of chelation,[Citation25] it is possible that high temperature used in this study made it impossible to maintain protective effect.
Significant variability in the content of individual exo- and endogenous amino acids in prepared samples was observed ( and ). In the analyzed systems the highest degradation of amino acids was observed for histidine and cysteine. So far, there was relatively little investigation of polyphenol interactions with histidine. However Soares et al.[Citation26] proved that proteins can interact with polyphenols through histidine, arginine, and phenylalanine residues. Cysteine, on the other hand, was already clearly characterized as an amino acid easily reacting with chlorogenic acids.[Citation10] Average decrease in amount of histidine and cysteine was 52 and 45%, respectively. Another sensitive amino acid belonging to exogenous group was methionine. Degradation on the average was at a level of 30%. Average relative degradation of 20–25% was found for glutamine+glutamic acid, isoleucine, and alanine. The biggest losses in exogenous amino acids took place in systems P/3%/1/180, P/S/3%/1/110, and P/1.5%/1/180. Thus, prolonged heating with GCE, without presence of substances other than saccharose, resulted in creating the best circumstances for those amino acids degradation, probably due to covalent interactions with chlorogenic acids oxidized to quinones. Other amino acids after heat treatment remained in concentrations more similar to the output values in fresh protein. This applies mainly to tryptophan and leucine. Particularly surprising was the result for tryptophan, which can readily covalently bind with oxidized chlorogenic acid.[Citation27] The biggest losses of this amino acid (not exceeding the 20%) were noticed in systems containing saccharose or oil, but in both cases an addition of GCE limited this effect. Operation that seemed to effectively limit tryptophan oxidation was observed previously by Salminen and Heinonen[Citation27] with a presence of plant extracts and various reference substances including chlorogenic acid.
Figure 4 Amino acid profile of egg white protein in model systems with coffee extract (a) exogenous amino acids: leucine (LEU), lysine (LYS), isoleucine (ILE), valine (VAL), phenylalanine (PHE), threonine (THR), methionine (MET), and tryptophan (TRP), and (b) endogenous amino acids: glutamic acid + glutamine (GLU), aspartic acid + asparagine (ASP), serine (SER), arginine (ARG), alanine (ALA), proline (PRO), glycine (GLY), threonine (TYR), histidine (HIS), and cysteine + cystine (CYS). The relative standard deviation did not exceed 10%.
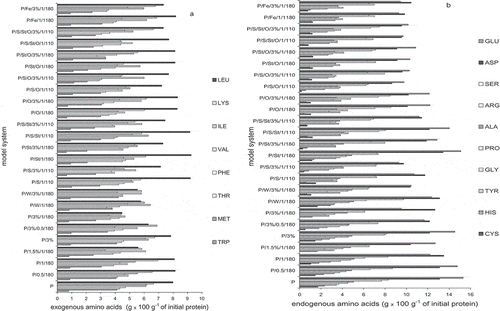
The above mentioned results are only partly consistent with works of Prigent et al.[Citation8] who analyzed the reactivity of several amino acids with chlorogenic acid. He did not observe the reaction with arginine, asparagine, and glutamine, while 75% of lysine, 30% of tyrosine, and 5% of tryptophan reacted. The differences may result from studying pure amino acids instead of large molecules of egg white proteins, containing all amino acids. However Kroll et al.[Citation28] observed changes in amounts of free indole, amino, and thiol groups in protein structure at room temperature when conducting a reaction of polyphenols with glicinin. Among the four analyzed by him, polyphenols chlorogenic acid caused the smallest losses of amino groups (24%), relatively high (compared to other polyphenols) losses of tryptophan (81%) and similar to other polyphenols losses of amino acids containing thiol groups (34%). In this study weak influence on tryptophan was observed. There was a moderate influence on lysine (on average 14% of degradation) and a significant influence on cysteine. Those results confirm the thesis that amino acid bioavailability after a reaction with polyphenols depends on protein structure and reaction conditions. Some, in theory unstable amino acids, could be placed in the inner part of protein molecule. Egg white proteins contain significant amounts of amino acids capable of creating disulfide bonds in the outer layer.[Citation29] This type of structure could be the cause of relatively high cysteine degradation.
Some amino acids degraded simply after mixing protein and coffee extract, without heating the system. This was mainly cysteine and tyrosine, and their degradation did not exceed 20%. Created in these conditions non-covalent bonds, as previously established, can be first formed with lysine, proline, and arginine.[Citation2] Tyrosine, in theory, can also hydrophobically interact with polyphenols thanks to a presence of aromatic group in its structure. Cysteine, due to lower susceptibility to form disulfide bonds in a presence of polyphenols, is probably more open for oxidation, forming, for example, cysteic acid.
Another analyzed issue was the susceptibility of egg white protein to proteolytic digestion caused by pepsin. Pepsin digestibility of egg white preparation was about 72 g × 100 g−1 (). Heating increased protein susceptibility to pepsin digestion due to partial denaturation. These observations are in accordance with results obtained by Diaa El-Din and Farag,[Citation30] who dry-heated sunflower meal in 121°C for 30 min and noticed an increase in proteolytic digestibility by 5%, while in the same time availability of lysine decreased by 11%. The biggest protein digestibility, amounting 95 g × 100 g−1, was observed in systems containing 3% of GCE – heated in time of both 0.5 and 1 h (this was also true for system containing an addition of 3% of water). Heating protein with an addition of 1.5% of GCE also increased digestibility to 85 g × 100 g−1. These results confirm the statement that polyphenols can interact with proteins and therefore retard the protein digestibility. They support earlier studies performed by Rawel et al.[Citation31] who observed that an addition of chlorogenic acid to whey protein increased its digestibility by about 3%. However the opinions about possible effect on protein digestibility under the influence of that kind of interactions are quite divided. For example Venkatachalam and Sathe[Citation32] didn’t notice any negative influence on phaseolin digestibility with pepsin in a presence of 17 selected food products containing phenolic compounds, including coffee. In other studies, a reduction in digestibility of whey protein highly substituted with chlorogenic acid was proven in vivo in rats, while low-substituted protein or protein only simply consumed with chlorogenic acid without conditions allowing to form covalent bonds didn’t affect digestibility with pepsin.[Citation6] It does not clarify if chlorogenic acid influenced more the degradation of whey protein or proteolytic enzymes.
Positive effect of GCE addition on egg white protein digestibility can be directly linked to prevention of amino acids oxidation. Although no correlation between the degradation of amino acids engaged in hydrolysis with pepsin and reduced susceptibility to pepsin was noticed. So, relatively high digestibility of egg white protein heated with GCE must be depending on some other mechanism than its protective influence on amino acids, which are crucial to efficiency of pepsin hydrolytic effect. Pepsin acts hydrolytically to peptide bonds, in particular at the proline, phenylalanine, tyrosine, and tryptophan chain side. The conditions in which food systems are treated could lead to changes in protein conformation, and thus expose the above mentioned residues, or on the contrary, lead to the creating of adducts with these amino acids and reduce the possibility of cutting the peptide chain by pepsin. Availability of peptide bonds to enzyme can also be retarded by forming of aggregates between protein molecules or by additional cross-linking due to sulfhydryl groups oxidation. An addition of reducing agents in a form of green coffee polyphenols can limit the forming of disulfide bonds between peptide chains, and therefore enhance pepsin activity.[Citation32] Disulfide bonds are present in native forms of protein where they play an important role in stabilizing tertiary structure of protein. That, in some degree, limits availability of enzymes into the inside of a molecule. Pepsin digests a molecule from the outer parts to the inside, so in case disulfide bond are located on the surface of a molecule an addition of reducing factors, which can disrupt that bond, can significantly influence susceptibility to digestion by pepsin.[Citation33] Egg ovalbumin includes such schemes in its outer part.[Citation29] Interactions between proteins and polyphenols can affect also the secondary structure of proteins, which can influence pepsin digestibility as well. For example, gallic and sinapic acids caused a reduction in the amount of α-helix structure in protein model system.[Citation34] A-helix structures are also found in egg white proteins.[Citation29] Changes in secondary structure can occur only when polyphenols achieve proper concentration, when they are able to destroy hydrogen bonds.[Citation5] Indeed, pepsin digestibility of model system improved by over 10 g × 100 g−1 when GCE content was increased from 1.5 to 3% (p < 0.05).
For systems containing protein and sucrose, protein and starch or protein and both, sucrose and starch, heated with an addition of GCE obtained digestibility levels were greater than for analogous systems without GCE addition. Systems additionally containing sunflower oil demonstrated smaller susceptibility to enzymatic digestion. That is partly due to physically limiting enzyme availability to the structure, and partly from possible increased degradation of amino acids during heating caused by lipid radicals formed in high temperature. In this instance, a beneficial effect of green coffee antioxidants was observed, in addition to increasing the digestibility. Presence of Fe2+ ions caused a decrease in susceptibility to pepsin digestion. It can be caused partly by chelation of amino acids and metals, which limits the availability of peptide bonds to enzymes. Although not without importance, might be a pro-oxidant effect of iron on coffee bean polyphenols, which can lead to quinone formation, which bind covalently with amino acid residues of protein.
Particularly satisfactory results regarding minimal protein degradation, observed through high total amino acid, and high pepsin digestibility, were obtained for the samples P/3%/0.5/180 and P/W/3%/1/180. Attendance of water during protein heating can significantly enhance its degradation with pepsin. This is related to hydrolytic destruction of tertiary and quaternary protein structure, which makes albumin more susceptible to hydrolysis with pepsin.[Citation33] Taking into account the concentration of amino acids and pepsin digestibility in vitro, it can be observed that an addition of GCE didn’t severely decrease the nutritional value of protein. It caused a degradation of a share of egg white amino acids, but at the same time it enhanced protein susceptibility to pepsin activity. Negative influence on nutritional value of protein was caused more by the presence of saccharose and oil during heating, especially when both were added.
Color Changes in Model Systems
The study of color in CIE L*a*b* system showed the greatest color changes in model systems such as P/S/O/3%/1/110, P/O/3%/1/180, P/O/1/180, when it was about the value of 30 (). GCE addition didn’t have an undoubted effect on the darkening of oil (L*), although, it caused a decrease in yellow pigment content (p < 0.05). Heated stand-alone protein had relatively high value of parameter a*, which was caused by amino acid degradation with simultaneous creation of red colored oxidation products. An addition of GCE counteracted formation of red color compounds, although these observations were not reflected by decreased intensity of amino acid degradation. This dependence was noticed only in systems containing oil, in which GCE addition could limit oxidative changes of fatty acids. Presented results didn’t sufficiently confirm the results obtained by Charurin et al.,[Citation20] who analyzed the concentration of brown pigment in model systems containing chlorogenic acid, arginine, and saccharose. In those studies the intensity of browning was influenced mainly by the presence of saccharose. Differences in results probably derive from different conditions used in experiments (4 min and 204°C versus 1 h and 110°C, leading to a temperature under saccharose caramelization temperature). Generally all systems containing sunflower oil showed high total color change, measured by dE parameter. It might be partly caused by darker color of oil even before heating, comparing to other components. Addition of oil alone decreased the L* value of non-heated systems to about 85, while after heating these values were about 60. So heat treatment was definitely the deciding phase with the color of systems containing sunflower oil. This oil is relatively vulnerable to heat treatment, its smoking point temperature is about 170°C, so prolonged heating in these conditions lead to its partial decomposition, which is characterized, among others, by the darkening of both lightness of color parameter and yellow color.
Antioxidant Activity of Model Systems
Antioxidant activity of model systems was analyzed by two methods, by measuring the redox potential and in the test with DPPH radical. The redox potential of pure water was 310.7 mV and for fresh white egg protein solution was 146.4 mV (). The addition of GCE to egg white protein without heating caused a decrease of redox potential, so a rise in antioxidant properties. After 1 h heating without an addition of GCE, protein showed a redox potential of 299.3 mV, and with an addition of only GCE a redox potential was in the range of 44.2 – 155.1 mV, where longer heating was followed by deterioration of antioxidant properties. Model systems, where starch, saccharose, sunflower oil, and ferrous ions were also present, showed higher redox potential in the range between 97.4–315.2 mV. High values of redox potential in some of model systems resulted from creating components with pro-oxidative capabilities during heat treatment with sunflower oil or Fe2+ ions.
Figure 6 Antioxidant activity of egg white protein in model systems with coffee extract measured as redox potential (RP) and DPPH IC50 scavenging activity (DPPH). Bars with the same letters (caps – DPPH) indicate no significant difference (p > 0.05).
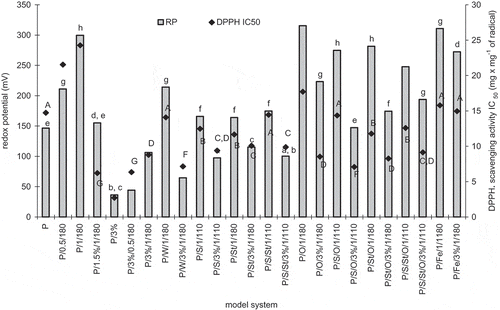
In the test with DPPH radical obtained results for IC50 of model systems ranged from about 3 to 24 mg × mg−1 of DPPH (). For example, Trolox has IC50 capacity of 0.28 mg × mg−1 DPPH and pure chlorogenic acid, which is the main ingredient with antioxidant properties in coffee extract, 1.63 mg × mg−1 DPPH and, therefore, pure antioxidants have activity of about 1 mg × mg−1 DPPH.[Citation35] Antioxidant properties calculated on the basis of radical scavenge ability were positively correlated with redox potential (Pearson’s correlation coefficient was 0.72). It confirmed limiting of antioxidant activity of stand-alone protein resulting from heating, and limiting of this adverse effect by adding GCE. Both tests of antioxidant activity demonstrated, that the worst antioxidant properties were shown by heating of stand-alone protein. Unheated protein had significantly better properties in this regard. All heated systems containing GCE and optionally other substances had better properties than the heated stand-alone protein. It is therefore worthwhile for enhancing antioxidant activity to add green coffee bean extract, rich in polyphenols, to food containing proteins, particularly to heated food. In this area, the highest antioxidant activity was the characteristics of protein with added 3% of GCE, in which a significant degradation of amino acids, but high pepsin protein digestibility were observed. Better antioxidant activity of complex model systems in comparison with heated stand-alone protein indirectly confirm findings of other studies, that antioxidant properties of food systems, particularly heated, significantly rise as a result of occurrence of Maillard reaction products.[Citation36] In some studies suggestions may be found, that caffeic and chlorogenic acids can have pro-oxidative effect, but it seems to be valid only in relation to early stages of their decomposition reactions. In latter stages of those reactions, caused for example by heat, polymerization of created compounds can occur, and high molecular weight products of this reaction show antioxidant properties.[Citation37] Many studies indicate a positive correlation between browning of food products due to Maillard reaction and antioxidant activity, but in products with initially high amounts of antioxidants this dependence is quite complex.[Citation38]
In food systems containing polyphenols and proteins, changes in polyphenol antioxidant properties are caused not only by oxidation of polyphenols, which in a small degree lowers antioxidant activity (resulting brown pigments have antioxidant activity), but also by non-covalent interactions with proteins, mainly through hydroxyl groups. Because of the fact that the highest observed antioxidant activity was in non-heated system of egg white protein and GCE, heating could lead to forming of adducts of chlorogenic acids with protein amino acids and limiting their antioxidant activity. Confirmation of this theory can be found in studies of Charurin et al.[Citation20] who performed analysis of model systems containing arginine, chlorogenic acid and saccharose. Their studies have shown that heating of stand-alone chlorogenic acid with filler in the form of cellulose didn’t cause any changes in antioxidant activity. However, heat treatment of chlorogenic acid with arginine caused a decrease of this activity, but a less significant one when additionally saccharose was put in the system. Stand-alone arginine or in a system with saccharose showed much less antioxidant activity than system containing chlorogenic acid. Some share in antioxidant activity of coffee extracts, beside hydroxycinnamic acids, also has caffeine, which is relatively stable in high temperatures.[Citation39]
CONCLUSIONS
This study confirmed that heating protein in the presence of polyphenols, and also in more complex systems leads to partial degradation of amino acids, including exogenous ones. Since the reaction between amino acids and polyphenols occurs faster with the products of oxidation of polyphenols (quinones), the presence of pro-oxidative factors may lead to increased degradation of amino acids. On the other hand, greater availability of water reduces this type of reactions. Protein heated together with coffee bean polyphenols shows high susceptibility to pepsin digestion. Heated model systems with the addition of GCE were characterized by high antioxidant activity, greater as more extract was added to the system, regardless of the degree of protein degradation.
ACKNOWLEDGMENT
The authors are grateful for the financial support provided by Polish Ministry of Science and High Education (project no. NN 312 300137).
REFERENCES
- Rawel, H.M.; Rohn, S. Nature of hydroxycinnamate-protein interactions. Phytochemical Review 2010, 9, 93–109.
- Zujko, M.E.; Witkowska, M. Antioxidant potential and polyphenol content of selected food. International Journal of Food Properties 2011, 14, 300–308.
- Nissen, L.R.; Byrne, D.V.; Bertelsen, G.; Skibsted, R.H. The antioxidative activity of plant extracts in cooked pork patties as evaluated by descriptive sensory profiling and chemical analysis. Meat Science 2004, 68, 485–495.
- Glei, M.; Kirmse, A.; Habermann, N.; Persin, C.; Pool-Zobel, B.L. Bread enriched with green coffee extract has chemoprotective and antigenotoxic activities in human cells. Nutrition and Cancer 2006, 56, 182–192.
- Petzke, K.J.; Schuppe, S.; Rohn, S.; Rawel, H.M.; Kroll, J. Chlorogenic acid moderately decreases the quality of whey proteins in rats. Journal of Agricultural Food Chemistry 2005, 53, 3714–3720.
- Kang, J.; Liu, Y.; Xie, M.X.; Li, S.; Jiang, M.; Wang, Y.D. Interactions of human serum albumin with chlorogenic acid and ferulic acid. Biochimica et Biophisica Acta 2004, 1674, 205–214.
- Zduńczyk, Z.; Frejnagel, S.; Wróblewska, M.; Juskiewicz, J.; Oszmianski, J.; Estrella, I. Biological activity of polyphenol extracts from different plant sources. Food Research International 2002, 35, 183–186.
- Prigent, S.V.E.; Voragen, A.G.J.; Feng, L.; Visse, A.J.G.; Koningsveld, G.A.; Gruppen, H. Covalent interactions between amino acid side chains and oxidation products of caffeoylquinic acid (chlorogenic acid). Journal of Agricultural Food Chemistry 2008, 88, 1748–1754.
- Richard, T.; Lefeuvre, D.; Descendit, A.; Quideau, S.; Monti, J.P. Recognition characters in peptide-polyphenol complex formation. Biochimica et Biophysica Acta 2006, 1760, 951–958.
- Bassil, D.; Makris, D.P.; Kefala, P. Oxidation of caffeic acid in the presence of L-cysteine: Isolation of 2-S-cysteinylcaffeic acid and evaluation of its antioxidant properties. Food Research International 2005, 38, 395–402.
- Budryn, G.; Nebesny, E.; Podsędek, A.; Żyżelewicz, D.; Materska, M.; Jankowski, S.; Janda, B. Effect of different extraction methods on the recovery of chlorogenic acids, caffeine, and Maillard reaction products in coffee beans. European Food Research and Technology 2009, 228, 913–922.
- Zhang, W.; Liu, S.; Wang, Y.; Zheng, L.; Liu, F.; Han, X.; Ren, Y.; Long, Q.; Zhao, Z.; Jiang, L.; Wan, J. A study of the in vitro protein digestibility of indica and japonica cultivars. Food Chemistry 2010, 122, 1199–1204.
- Elkin, R.G.; Wasynczuk, A.M. Amino acid analysis of feedstuff hydrolysates by precolumn derivatization with phenylisothiocyanate and reversed-phase-performance liquid chromatography. Cereal Chemistry 1987, 64, 226–229.
- Kwanyuen, P.; Joseph, J.W. A modified amino acid analysis using PITC derivatization for soybeans with accurate deetermination of cysteine and half-cystine. Journal of American Oil Chemists’ Society 2010, 87, 127–132.
- Andueza, S.; Manzocco, L.; de Peña, M.P.; Cid, C.; Nicoli, C. Caffeic acid decomposition products: Antioxidants or pro-oxidants? Food Research International 2009, 42, 51–55.
- Nicoli, M.C.; Toniolo, R.; Anese, M. Relationship between redox potential and chain-breaking activity of model systems and food. Food Chemistry 2004, 88, 79–83.
- Zhou, K.; Yin, J.J.; Yu, L.L. ESR determination of the reactions between selected phenolic acids and free radicals or transition metals. Food Chemistry 2006, 95, 446–457.
- Mine, Y. Recent advances in egg protein functionality in the food system. World’s Poultry Science Journal 2002, 58, 31–39.
- Fu, T.J.; Maks, N.; Banaszewski, K. Effect of heat treatment on the quantitative detection of egg protein residues by commercial enzyme-linked immunosorbent assay test kits. Journal of Agriculture and Food Chemistry 2010, 58, 4831–4838.
- Charurin, P.; Ames, J.M.; Castillo, M.D. Antioxidant activity of coffee model system, Journal of Agricultural Food Chemistry 2002, 50, 3751–3756.
- Sarni-Manchado, P.; Canals-Bosch, J.M.; Mazerolles, G.; Cheynier, V. Influence of the glycosylation on human salivary proline-rich proteins on their interactions with condensed tannins. Journal of Agricultural and Food Chemistry 2008, 56, 9563–9569.
- Viljanen, K.; Kivikari, R.; Heinonen, M. Protein-lipid interactions during liposome oxidation with added anthocyanin and other phenolic compounds. Journal of Agriculture and Food Chemistry 2004, 52, 1104–1111.
- Estévez, M.; Kylli, P.; Puolanne, E.; Kivikari, R.; Heinonen, M. Oxidation of skeletal muscle myofibrillar proteins in oil-in-water emulsion: Interaction with lipids and effect on selected phenolic compounds. Journal of Agricultural and Food Chemistry 2008, 56, 10933–10940.
- Ganhão, R.; Morcuende, D.; Estévez, M. Tryptophan depletion and formation of α-aminoadipic and γ-glutamic semialdehydes in porcine burger patties with added phenolic-rich fruit extracts. Journal of Agriculture and Food Chemistry 2010, 58, 3541–3548.
- Estévez, M.; Heinonen, M. Effect of phenolic compounds on the formation of α-aminoadipic and γ-glutamic semialdehydes from myofibrillar proteins oxidized by copper, iron, and myoglobin. Journal of Agriculture and Food Chemistry 2010, 58, 4448–4455.
- Soares, S.; Mateus, N.; Freitas, V. Interaction of different polyphenols with bovine serum albumin (BSA) and human salivary alpha-amylase (HSA) by fluorescence quenching. Journal of Agricultural Food Chemistry 2007, 55, 6726–6735.
- Salminen, H.; Heinonen, M. Plant phenolics affect oxidation of tryptophan. Journal of Agriculture and Food Chemistry 2008, 56, 7472–7481.
- Kroll, J.; Rawel, H.M.; Rohn, S.; Czajka, D. Interactions of glycinin with plant phenols - influence on chemical properties and proteolytic degradation of the proteins. Nahrung 2001, 45, 388–389.
- Iametti, S.; Donnizzelli, E.; Vecchio, G.; Rovere, P.P.; Gola, S.; Bonomi, F. Macroscopic and structural consequences of high pressure treatment of ovalbumin solutions. Journal of Agricultural and Food Chemistry 1998, 46, 3521–3527.
- Diaa El-Din, M.; Farag, H. Effect of radiation and other processing methods on protein quality of sunflower meal. Journal of the Science of Food and Agriculture 1999, 79, 1565–1570.
- Rawel, H.M.; Kroll, J.; Hohl, U.C. Model studies on reactions of plant phenols with whey proteins. Nahrung 2001, 45, 72–81.
- Venkatachalam, M.; Sathe, S.K. Phaseolin in vitro pepsin digestibility: Role of acids and phenolic compounds. Journal of Agriculture and Food Chemistry 2003, 51, 3466–3472.
- Guo, X.; Yao, H.; Chen, Z. Effect of heat, rutin, and disulphide bond reduction on in vitro pepsin digestibility of Chinese tartary buckwheat protein fractions. Food Chemistry 2007, 102, 118–122.
- Liu, Y.; Xie, M.X.; Jiang, M.; Wang, Y.D. Spectroscopic investigation of the interaction between human serum albumin and three organic acids. Spectrochimica Acta Part A 2005, 61, 2245–2251.
- Budryn, G.; Nebesny, E. Antioxidant properties of Arabica and Robusta coffee extracts prepared under different conditions. Deutsche Lebensmittel-Rundschau 2008, 104, 69–77.
- Bekedam, E.K.; Schols, H.A.; Cämmerer, B.; Kroh, L.W.; Van Boekel, M.A.J.S.; Smit, G. Electron spin resonance (ESR) studies on the formation of roasting-induced antioxidative structures in coffee brews at different degree of roast. Journal of Agricultural Food Chemistry 2008, 56, 4597–4604.
- Hotta, H.; Nagano, S.; Ueda, M.; Tsujino, Y.; Koyama, J.; Osakai, T. Higher radical scavenging activities of polyphenolic antioxidants can be ascribed to chemical reactions following their oxidation. Biochemica et Biophisica Acta 2002, 1572, 123–132.
- Popa, C.V.; Lungu, L.; Savoiu, M.; Bradu, C.; Dinoiu, V.; Danet, A.F. Total antioxidant activity and phenols and flavonoids content of several plant extracts. International Journal of Food Properties 2012, 3, 691–701.
- Davicino, R.; Alonso, R.; Anesini, C. Comparison between normal coffee and decaffeinated coffee effects on lymphocytes and macrophages: Role of the antioxidant activity of caffeine. Journal of Food Biochemistry 2011, 35, 877–897.