Abstract
Effects of high hydrostatic pressure combined with blanching on microorganisms and quality attributes of strawberry juices were investigated. High hydrostatic pressure at 600 MPa/4 min/ambient temperature inactivated total aerobic bacteria, coliform bacteria, yeasts, and molds in juices, ensuring their microbiological safety. Under this condition, the cloudiness of cloudy juices increased by 54.49% and its viscosity decreased by 12.40%. Ascorbic acid decreased by 7.82% in cloudy juices and 12.60% in clear juices. The content of total volatile flavor compounds increased by 13.21% in cloudy juices and decreased by 6.92% in clear juices. No significant changes in anthocyanins, total phenols, and antioxidant capacity were found with high hydrostatic pressure treatment.
INTRODUCTION
Strawberry is a soft juicy and palatable berry with bright-red color, and is an important bioresource with good processing potential. It is abundant in antioxidative compounds with antioxidant properties, mainly phenolic compounds, especially anthocyanins. Antioxidant capacity of strawberry can participate in the prevention of cancer, cardiovascular and other chronic diseases.[Citation1] At present, foods are assumed the status of being “functional,” that is they should be capable of providing additional physiological benefit, such as preventing or delaying onset of chronic diseases, as well as meeting basic nutritional requirements. Strawberry is one of the most popular fruits. Apart from being consumed freshly, strawberry is also processed into jams, juice, canned strawberry, candied fruits, and added into yoghurts. Strawberry juice is a natural antioxidant drink with bright-red color, attractive aroma, sweet-sour mouthfeel, and abundant antioxidant compounds. Thermal processing technologies used for inactivating microorganisms and enzymes in juice is generally at 70–121°C for 30–120 s,[Citation2−Citation3] and it seriously affects the quality of strawberry juice resulting from the loss of nutritional components and changes in color, flavor, and texture due to their heat sensitivity.[Citation4−Citation5]
As consumers are demanding minimally processed and fresh food products, the application of non-thermal techniques is gaining popularity. High hydrostatic pressure (HHP) is a non-thermal food preservation technique, which subjects foods to 100–1000 MPa using water as pressure transmitting medium at room or mild process temperatures (<60°C). Harmful pathogens, such as molds and vegetative spoilage microorganisms, were inactivated by HHP treatment, and the effects on taste, texture, appearance, or nutritional value of foods were minimal due to its limited effect on the covalent bonds of low molecular-mass compounds.[Citation4Citation6−Citation8] HHP has been applied to process many fruit and vegetable products, such as tomato puree,[Citation9−Citation11] apple juice,[Citation12] peach juice,[Citation13] mango pulps,[Citation14] guava juice and puree,[Citation15Citation16] orange juice,[Citation17] and strawberry puree.[Citation18] High-pressure processing provides a unique opportunity for food processors to develop a new generation of value-added food products having superior quality to those produced conventionally.[Citation6] Strawberry, apple, and kiwi jams represented the first wave of pressure-treated commercial products introduced into the Japanese market in 1990, and avocado-based products, especially guacamole, were subsequently commercialized in the United States since 1997.[Citation6]
Investigations about the effects of HHP on strawberry products have been carried out in previous literatures. Zabetakis et al.[Citation4] reported that there was no significant loss in anthocyanins in HHP-treated fresh strawberry whole fruit and the stability of anthocyanins was improved during storage. Patras et al.[Citation5] reported that the content of phenols in HHP-treated (400–600 MPa for 15 min) strawberry puree increased and the content of ascorbic acid decreased compared to that in untreated samples. Lambert et al.[Citation19] reported that no significant changes in all the aromatic volatile compounds were observed between untreated and HHP-treated (200 and 500 MPa/20°C/20 min) strawberry coulis. In these studies, strawberries were not blanched for inactivating indigenous enzymes prior to HHP processing. Studies have demonstrated that HHP could not effectively inactivate quality-related enzymes, such as polyphenol oxidase (PPO), peroxidase (POD), and pectin methylesterase (PME), in fruits and vegetables.[Citation2,Citation17,Citation20] Zhang[Citation21] found that the residual activity of PME in raw raspberry juice was still 85.3% after HHP treatment at 600 MPa for 20 min without combining with temperature. Garcia-Palazon et al.[Citation2] reported that residual activity of ß-glucosidase and POD in strawberry whole fruit were 39 and 65% after HHP treatment at 800 MPa for 15 min. Dalmadi et al.[Citation20] reported that PPO extracts of strawberry (pH = 7.0) were quite stable until 500 MPa, and there was a sharp decrease in the residual activity after 15 min between 500 and 700 MPa. The qualities of HHP-processed fruit and vegetable products would change during storage due to coexisting chemical reactions, such as oxidation, and biochemical reactions when endogenous enzymes are incompletely inactivated;[Citation8] thus, it is dispensable for HHP-processed fruits and vegetable products to be refrigerated. However, refrigeration increases the production cost and does not benefit the commercialization of HHP-processed fruits and vegetable products. Based on these studies, a combination of HHP and blanching for processing fruits and vegetable products should be carried out for extending their shelf life at room temperature, but there is no investigation about the combination of HHP and blanching in strawberry processing.
The purpose of this work was to evaluate the effects of HHP on microorganisms and quality attributes of strawberry cloudy and clear juices. This study will provide technical support for HHP commercialization in the processing of strawberry juices.
MATERIAL AND METHODS
Strawberries
Strawberries (Tongzi Ι) supplied by Tianyi Strawberry Bio-engineering Company (Beijing, China) were harvested at commercial maturation in June 2010. The fruit samples were stored at 4°C until being processed within 3 days. The basic physicochemical characteristics of the fruit samples were as follows: total soluble solid (TSS) 5.6 ± 0.1 °Brix using a digital Abbe Refraction meter (WAY-2S, Shanghai Precision and Scientific Instrument Co., Shanghai, China), titration acid (TA) 0.7 ± 0.02% determined by potentiometric titration (750, Metrohm, Swiss), pH 3.45 ± 0.1 by pH meter (Orion 868, Thermo Orion, USA).
Blanching
Fresh strawberries were rinsed with tap water and cut into 2–3-mm-thick slices. To inactivate endogenous enzymes, strawberry slices were steam blanched for 0.5, 1, 1.5, and 2 min in a steam pan (Zhenghan Stainless Steel Factory) heated on an electromagnetic furnace (Midea RT 2103, Guangdong Midea Electrical Co., LTD). PPO, POD, and PME were chosen as target enzymes.
Preparation of Cloudy and Clear Juices
Blanched strawberry slices were pulped by a juice extractor (JY-610, Joyoung Co., Ltd., Shandong, China) with a quarter of distilled water (strawberry:water = 4:1, m/v). Then the pulps were centrifuged at 2063 × g for 10 min, and the supernatant was collected as raw juices. One part of the raw juices was blended by adding sucrose and water to obtain cloudy juice (raw juice content 50%, 10 ± 0.1 °Brix, TA 0.38%). To prepare clear juices, pectinex ultra clear (Novozymes Biotechnology Co., Ltd., Beijing, China) was added to the raw juices with a final concentration of 50 ppm for maceration at 45°C, and the incubation time was 30, 45, 60, 75, and 90 min. After that, the pulps were centrifuged at 2063 × g for 10 min and the supernatant was collected. Then 50 mL of ethanol (95%, contained with 1% HCl) was added to 25 mL of supernatant juice for 15 min to detect pectin, and 60 min was finally selected as the maceration time since it could totally remove pectin. The collected supernatant after maceration was used to blend for clear juice (raw juice content 50%, 10 ± 0.1 °Brix, and TA 0.38%) by adding sucrose and distilled water. The cloudy and clear strawberry juices were filled into plastic bottles (EVOH/PVPP) and the lids were manually screwed. The juices were kept at 4°C until HHP treatment. The flow chart of juice preparation was shown in .
HHP Processing
Juice samples were placed in a high pressure vessel (15 cm internal diameter × 30 cm internal height, with a capacity of 7.0 L; Pressure Engineered System, HHP-750, Baotou Kefa Co., Inner Mongolia, China) and subjected to HHP at 600 MPa for 0, 2, 4, and 6 min at ambient temperature (≈ 25°C). The HHP conditions were selected based on preliminary experimental work by Zhang[Citation21] in which 400 or 500 MPa for 0–25 min could not totally inactivate the microorganisms, while 600 MPa/4–25 min could totally inactivate the microorganisms. The come-up time is 6 min to reach 600 MPa and the pressure release time is 10 s.
Enzyme Activity
For enzymes extraction, 100 g of strawberry pieces were pulped with 200 mL buffer (0.2 M, pH 6.5, phosphate buffer for PPO and POD, and 0.2 M, pH 8.0, Tris-HCl buffer containing 0.1 M NaCl for PME). After incubation at 4°C for 4 h, the mixture was centrifuged at 9000 × g for 10 min at 4°C, and the supernatant was collected and analyzed for enzyme activity. PPO and POD activity were determined by a spectrophotometric method.[Citation18] The reaction mixture for PPO was 1 mL of extract and 2 mL of catechol (0.07 M) in sodium phosphate buffer (0.5 M, pH 6.5) solution. The reaction mixture for POD was 2.2 mL of guaiacol (1.0%, m/v, dissolved in 0.2 M phosphate buffer, pH 6.5), 0.2 mL of H2O2 (1.5%, m/v), and 0.8 mL of extract. The increase in absorbance at 420 and 470 nm for PPO and POD, respectively, was monitored at intervals of 0.1 s−1 immediately after incubation with a spectrophotometer (Cary 50, Varian Co. Ltd., California, USA). The slope of the very first linear part of the reaction curve was taken as PPO and POD absolute activity (Abs/min). The initial PPO and POD activity were 0.1219 and 0.21608 Abs/min, respectively.
The activity of PME was measured at pH 7.5/30°C according to the method proposed by Cao et al.,[Citation18] which was based on carboxyl group titration. To begin, 5 mL of extract were mixed with 20 mL of apple pectin (1%, DE 70–75%, Andre Co., Shandong, China) containing 0.1 M NaCl and incubated at 30°C. The mixture was adjusted to pH 7.0 with NaOH (2.0 M), and then the pH of the solution was readjusted to 7.5 with NaOH (0.05 M). After that, 0.05 mL of NaOH (0.05 M) was added. The time required for the solution’s pH to return to 7.5 was measured. PME activity (A) expressed in pectin methylesterase units (PMEU) was calculated by the following equation:
Enumeration of Viable Cells
To detect the viable cells of natural microorganisms in cloudy and clear juices, a total plate count method was used (National Food Hygienic Standard of China 4789.2-2010). Untreated or HHP-treated juices of 25 mL were serially diluted with sterile NaCl (0.85%, m/v) solution to 250 mL, and 1.0 mL of diluted solution was plated into duplicated plates of appropriate agar. The plate count agar (PCA; composition: tryptone 10.0 g/L, yeast extract 2.5 g/L, glucose 1.0 g/L, agar 15.0 g/L, pH 7.0 ± 0.1; Beijing Luqiao Technology Co., Ltd., Beijing, China) was used for detecting the viable cells of total aerobic bacteria. The plates were incubated at 37°C for 24 ± 2 h. The rose bengal agar (RBA; composition: peptone 5.0 g/L, glucose 10.0 g/L, KH2PO4 1.0 g/L, (NH4)2SO4 0.5 g/L, rose Bengal 0.03 g/L, chloromycetin 0.1 g/L, agar 15.0 g/L, pH 6.0 ± 0.1; Beijing Luqiao Technology Co., Ltd.) was used for detecting the viable cells of yeasts and molds (National Food Hygienic Standard of China 4789.15-2010). The plates were incubated at 27°C for 72 ± 2 h.
The most probable number method (National Food Hygienic Standard of China 4789.3-2010) was used to detect the coliform bacteria in strawberry juices. In the pre-fermentation test, samples were diluted into three appropriate series, and 1.0 mL of samples was inoculated with 10 mL of lauryl sulfate tryptose (LST) broth (Beijing Luqiao Biological Technology Co. Ltd., Beijing, China) in triplicate tubes at 37°C for 48 ± 2 h. And then, in the re-fermentation test, loopfuls of samples from inoculated LST broth, which were positive for turbidity and gas formation, were inoculated in tubes of 10 mL of brilliant green lactose bile (BGLB) broth(Beijing Luqiao Biological Technology Co. Ltd.). After incubating at 37°C for 48 ± 2 h, the positive tubes with color and turbidity changes and gas formation were counted.
Color Assessment
Color assessment was conducted at 20 ± 1°C using a Color Difference Meter (SC-80, Kangguang Co., Beijing, China) in the reflectance mode for cloudy juices and the transmission mode for clear juices. Color was expressed in L*, a*, and b* values. Three measurements were performed and results were averaged. In addition, total color difference (ΔE) was calculated using the following equation,[Citation22] where L*0, a*0, and b*0 are control values for untreated juices:
Cloud Assessment
Ten milliliters of juices were centrifuged at 2063 × g at 25°C for 10 min, and the supernatant was collected and measured at 660 nm using a spectrophotometer (UV-726, Shimadzu, Shanghai, China) at 20 ± 1°C, as described by Chandler and Robertson.[Citation23]
Determination of Viscosity
The dynamic viscosity of cloudy and clear juices was determined using an AR550 rheometer (TA Instruments,Waters Co., Ltd., Surrey, Great Britain) with a Conical End Concentric Cylinder (stator radius = 15.00 mm, rotor radius = 14.00 mm, immersed height = 42.00 mm, gap = 5920 um). An aliquot of 13.5 mL of juices were applied at each measurement at 25 ± 0.1°C controlled by circulating water in a thermostatic system. Viscosities were calculated as the average value of seven points of the flow curves obtained in the shear rate range between 4 and 63 s−1.
HPLC Analysis of Ascorbic Acid
For extracting and analyzing ascorbic acid in juices, the method was proposed by Patras et al.[Citation5] with some modifications. To begin, 50 mL of strawberry juices were mixed with 250 mL of metaphosphoric acid (2.5%) and incubated at 4°C for 2 h, then centrifuged using 9000 × g for 10 min at 4°C. The supernatant was filtered through two-layer cheese cloth, and the volume was measured. Before HPLC analysis, the ascorbic acid extract was passed through a 0.45-μm cellulose nitrate membrane (Beijing Bomex Co., Beijing, China).
Ascorbic acid was separated using a Shimadzu liquid chromatograph (Shimadzu Co., Japan) equipped with a prominence UV/Vis detector (SPD-20AV), an auto sampler (SIL-20A), and a column oven (CTO-20A). The separation was performed on an Alltech AlltimaTM C18 column (4.6 × 250 mm i.d., 5-μm particle size) from Waters. The mobile phase was an isocratic solvent system consisting of 95% monopotassium phosphate (50 mM, pH = 3.0) and 5% acetonitrile. The flow rate was 1 mL/min and aliquots of 20 μL were injected. The analyses were conducted at ambient temperature. The detection was carried out at 245 nm in absorbance mode. The content of ascorbic acid was calculated by the following equation and results were expressed as milligrams per 100 mL of juices:
HPLC Analysis of Monomeric Anthocyanins
For anthocyanins extraction, 10 mL of strawberry juices were mixed with 20 mL of HCl (0.1%) in methanol. After standing for 1 h at 4°C, the mixture was centrifuged at 9000 × g for 10 min at 4°C, the supernatant was collected, and the volume was measured. Before HPLC analysis, the anthocyanins extract was passed through a 0.45-μm cellulose nitrate membrane (Beijing Bomex Co., Beijing, China).
Chromatographic separation of anthocyanins was performed on a vessuil C18 column (250 mm × 4.6 mm i.d., 5-μm particle size) equipped with a 5-μm C18 guard column also from Agela Technologies Co. (Tianjin, China). The mobile phases for anthocyanins separation were methanol (A) and formic acid/water (B, 5:95, v/v). The solvent flow rate was 1 mL/min and aliquots of 20 μL were injected. The gradient elution was as follows: 0–15 min, 15% A; 15–20 min, 15–30% A; 20–30 min, 30–50% A; 30–33 min, 50–80% A; 33–35 min, 80–15% A; 35–40 min, 15% A. Spectra at 520 nm were recorded by an ultraviolet-visible detector. Cy-3-glu, Pg-3-glu, and Pg-3-rut were quantified as monomeric anthocyanins by external standards. Standard anthocyanins were dissolved in HCl (0.1%). The content of anthocyanins was calculated by the following equation and results were expressed as milligrams per 100 mL of juices:
Determination of Total Phenols
Total phenols were determined using the Folin-Ciocalteau method described by Oszmiajski and Wojdyio[Citation1] with some modifications. Initially, 125 μl of 100-fold diluted phenolic compound extracts were mixed with 2 mL of Folin-Ciocalteau reagent (previously diluted 10-fold with distilled water) and reacted for 1 h in the dark at room temperature. Then, 1.8 mL of sodium carbonate (105.99 g/mol) was added to the mixture and reacted for 15 min, and the mixture was immediately measured at 765 nm by a spectrophotometer (UV-726, T6, PG General, Beijing, China). Results were expressed as milligrams of gallic acid per 100 mL of juices.
Determination of Antioxidant Activity
Antioxidant activity was studied through the evaluation of the free-radical scavenging-effect on 1,1-Diphenyl-2-picrylhydrazyl radical (DPPH) as described by Sánchez-Moreno et al.[Citation10] and ferric reducing/antioxidant power (FRAP) according to the method developed by Zhang.[Citation21]
DPPH assay
The reaction started by adding 100 μL of Vc solution or the diluted strawberry juice to the cuvette containing 4 mL of methanol solution of the free radical (0.14 mmol/L). The mixture was reacted in the dark for 15 min, and then the absorbance was measured at 765 nm with a spectrophotometer (UV-726, T6, PG General, Beijing, China). Results were expressed as equal to milligrams of Vc per 100 mL of juices.
FRAP assay
Freshly prepared FRAP solution contained 25 mL of 0.3 mol/L acetate buffer (pH 3.6) plus 2.5 mL of TPTZ (10 mmol/L, dissolved in 40 mmol/L HCl) and 2.5 mL of ferric chloride (FeCl3·6H2O, 20 mmol/L). To start, 4 mL of FRAP solution at 37°C was mixed with 100 μL of Vc solution or diluted strawberry juice. After reaction for 10 min, the ferric reducing ability of strawberry juice was measured by monitoring the increase of absorbance at 593 nm with a spectrophotometer (UV-726, T6, PG General, Beijing, China) and the FRAP solution was used as the blank. Results were expressed as equal to milligrams of Vc per 100 mL of juices.
GC-MS Analysis of Volatile Flavor Compounds
Volatile flavor compounds were analyzed by Solid-phase micro-extraction (SPME)/Gas Chromatograph (GC)/Mass Spectrometer (MS). Initially, 8 mL of juice and 2 g of NaCl were transferred to a SPME extraction bottle (15 mL), and preheated in a water bath at 40°C for 10 min before extraction. Then, the aging extraction fiber was extended and was exposed to the sorption surface above the liquid surface for sampling for 40 min at 40°C on a Corning stirrer/hot plate. The fiber was introduced into the GC injector where desorption was carried out at 250°C for 3 min, followed by a start-up and collection of data.
The identification and quantification of flavor components in juice samples were performed on a GC-MS (Agilent Technologies 7890A GC system and 5975C inert XL MSD with triple-axis detector). Separations were done with a DB-5 column, which was preconditioned at 250°C for a period of 40 min. Helium was used as the carrier gas (0.8 mL/min). The analysis was conducted following the program at 40°C for 3 min, 40–60°C (2°C/min), then 60–130°C (10°C/min), 130–280°C (20°C/min), and 280°C (4.5 min). The temperature of the injector was 250°C. A non-diffluent mode was used with a sample size of 1 L. The interface temperature between GC and MS was set at 250°C. Electron impact ionization was 70 eV, electronic ionization mode, and the filament current was 0.25 mA. The ion-source temperature was 200°C. The scan range was 33–450 amu. Mass spectra correlations were made using the National Institute of Standards and Technology library. Results were reported when the matching degree was higher than 85%.
Table 1 Inactivation of total aerobic bacteria, coliform bacteria, and yeasts and molds in cloudy and clear juices by HHP
Statistical Analysis
All experiments were performed in triplicate. The data were analyzed using the Statistical Program for Social Sciences (SPSS 12.0) software for analysis of variance and Duncan’s test. The significance was established at p ≤ 0.05.
RESULTS AND DISCUSSION
After steam blanching for 0.5 min, residual activity of PPO, POD, and PME were 35.11, 45.67, and 29.09%, respectively. With blanching time increased to 1, 1.5, and 2 min, PPO, POD, and PME were totally inactivated. Thus, the steam blanching time of 1 min was chosen.
Inactivation of Total Aerobic Bacteria, Coliform Bacteria, and Yeasts and Molds
shows the effects of HHP at 600 MPa as a function of treatment time on the inactivation of total aerobic bacteria, coliform bacteria, and yeasts and molds in cloudy and clear juices. The original count of total aerobic bacteria, coliform bacteria, and yeasts and molds in cloudy juice showed 1-log cycle higher than that in clear juices, which was possibly due to high insoluble solids in cloudy juice since microorganisms may be partly inhibited or killed during the enzyme hydrolysis step (45°C/60 min) and moved with the pulps in the centrifugation step. In our previous study using identical strawberries, the original counts of total aerobic bacteria, coliform bacteria, yeasts and molds in strawberry puree were 4.4 × 104 cfu/mL, 5.4 × 103 cfu/mL, and 2.8 × 103 cfu/100 mL, respectively, which were similar to those in cloudy juices in this study. Total aerobic bacteria, coliform bacteria, and yeasts and molds in cloudy and clear juices were totally inactivated after HHP treatment at 600 MPa regardless of treatment time when the original count was less than 4 log cycles (). However, it took 4 min to inactivate total aerobic bacteria to below the detectable level in cloudy juice at 600 MPa in this study, since the original count was 1.7 × 104 cfu/100 mL. Many studies related to HHP pasteurization in juices were reported. Pan et al.[Citation24] reported that the total aerobic bacteria in raw strawberry juice was reduced to 30 cfu/mL after HHP treatment at 500 MPa/29°C/15 min, while the coliform bacteria and yeasts and molds were totally inactivated at 350 MPa/29°C for 3 min and 10 min, respectively. Song et al.[Citation25] found that the total aerobic bacteria and coliform bacteria in mixed fresh carrot and strawberry juice treated at 400 MPa/20 min were less than 10 cfu/mL and 3 cfu/100 mL. Houská et al.[Citation26] reported that HHP treatment at 500 MPa/10 min was able to inactivate more than 5 logs of the viable microorganisms in raw apple-broccoli juice and coliform bacteria, yeasts and molds, and salmonella did not show any further growth during 30 days of storage at 5°C. However, the treatment time reported in previous literatures was longer than that used in this study. From an industrial viewpoint, shorter treatment time is top priority in HHP commercialization. To achieve a balance between microbiological safety and qualities of cloudy and clear juices in this study, HHP treatment at 600 MPa/4 min was selected for quality analysis.
Effects of HHP on Viscosity and Cloud of Cloudy and Clear Juices
shows changes in viscosity of cloudy and clear juices treated at 600 MPa/4 min as a function of shear rate. The viscosity of all the juices at different conditions exhibited no alteration with the shear rate range between 4 and 63 s−1 (P > 0.05), suggesting that the juices followed the Newtonian flow behavior. Zhang[Citation21] also found that the flow behavior of raw raspberry juice was well retained regardless of the pressure level or treatment time (100–600 MPa/5–20 min/room temperature). The viscosity of HHP-treated cloudy juices decreased by 12.40% (P < 0.05), which was different from previous studies. Dalmadi et al.[Citation20] found that the viscosity of strawberry puree was slightly increased after HPP treatment at 600 MPa/5 min, and showed a significant increase with extending the treatment time to 15 min. Yen and Lin[Citation16] reported that the viscosity of guava cloudy juice (30% raw juice, pH 3.8, 12.0 °Brix) did not change after HHP treatment at 100 or 300 MPa/10 min/25°C, and increased slightly at 500 and 600 MPa. Krebbers et al.[Citation9] found a stabilization of viscosity of tomato puree at low pressures and a linear increase in the viscosity with increasing pressures. In these studies, the stabilization or increase in viscosity of HHP-treated fruit products were attributed to the presence of PME activity, which could induce the demethoxylation reaction and make high methoxyl pectin change into low methoxyl pectin. The low methoxyl pectin cross-linked to calcium ion and other macromolecular compounds, such as protein, to form pectin gel,[Citation27] and thereby increases the viscosity. The decrease in viscosity of cloudy strawberry juice was attributed to disruption of no-covalent bonds, including hydrophobic bonding, salt bridges, metallic bonds, and intramolecular forces between pectin molecules and other components during HHP processing. Ahmed et al.[Citation14] reported that the viscosity of canned mango pulps purchased from a local market decreased while that of fresh mango pulps increased after HHP treatment at 100–400 MPa/15 or 30 min/20°C, since the fresh mango pulps without heat treatment had a higher PME activity while the canned pulps heated showed no PME activity. The viscosity of clear juices was much lower than that of cloudy juice since the pectin in the juices was degraded by the enzymatic hydrolysis.
Figure 2 The flow behavior of cloudy and clear juices: ▪, untreated cloudy juice; □, cloudy juice treated by HHP at 600 MPa/4 min/ambient temperature; ▲, untreated clear juice; △, clear juice treated by HHP at 600 MPa/4 min/ambient temperature.
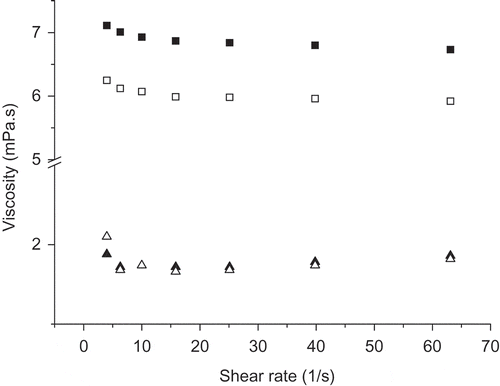
A common problem associated with cloudy juice is the loss of cloud, which is attributed to the suspension of particles composed of a complex mixture of protein, pectin, lipids, hemicellulose, cellulose, and other minor components. Up-to-date studies on the effect of HHP on the cloud of juices were rather limited. HHP treatment leads to a 54.49% increase (p < 0.05) of the cloud in cloudy juice (), indicating a remarkable improvement of the cloud stability of cloudy juice. Zhang[Citation21] found that the cloud of raspberry juice increased when subjected to HHP treatment at 100–600 MPa/5 or 10 min. The cloud stability was also related to the particles in the juice.[Citation3] Pressure would lead to homogenization effect causing smaller particles of the juice colloid[Citation3] and contributing to the improvement of cloud stability. However, Baron et al.[Citation12] reported that the particle size distribution of HHP-treated raw cloudy apple juice at 100–600 MPa/25°C did not change. Goodner et al.[Citation17] reported that only pressure combined with temperature (500 MPa/60°C) or pressure higher than 700 MPa could ensure the cloud stability of orange juice products.
Figure 3 Effect of HHP at 600 MPa/4 min/ambient temperature on the cloud and viscosity of cloudy and clear juices.
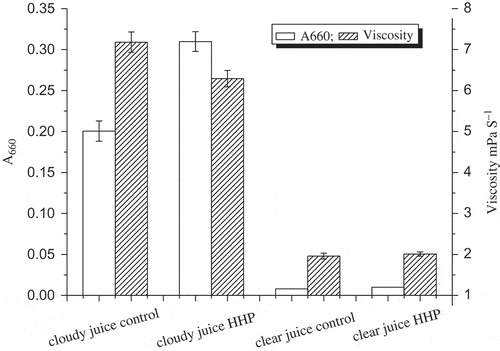
As shown in , lower viscosity corresponding to higher cloud in HHP-treated cloudy juice and higher viscosity corresponding to lower cloud in untreated cloudy juices was manifested. One explanation was that the gel formation of pectin catalyzed by PME in untreated cloudy juices caused higher viscosity, and the pectin gel was more easily removed by centrifugation and thus lower cloud was detected. Moreover, the disruption of no-covalent interactions between pectin molecules and other components in cloudy juice after HHP treatment made the juice more stable during centrifugation and led to higher cloud.
Effects of HHP on Antioxidative Compounds and Antioxidant Capacity of Cloudy and Clear Juices
Table 2 The effect of HHP at 600 MPa/4 min/ambient temperature on antioxidantive compounds, antioxidant capacity, and colour of cloudy and clear juices
Table 3 The effect of HHP at 600 MPa/4 min/ambient temperature on the volatile flavor compounds of cloudy and clear juices
The antioxidant capacity in cloudy and clear juices after HHP treatment was evaluated using·DPPH and FRAP methods. DPPH and FRAP values of cloudy juice was 74.31 and 38.28 mg Vc/100 mL, while those of clear juices were 50.34 and 26.21 mg Vc/100 mL, respectively (). Lower values of DPPH and FRAP in clear juices was due to lower content of antioxidantive compounds as mentioned above. Phenolic compounds, including flavonoids, phenolic acids, and anthocyanins, are known to be responsible for antioxidant capacities in fruits, and the fruits with higher phenolic contents generally show stronger antioxidant capacities. In this study, the content of anthocyanins and total phenols showed high correlation (P < 0.05) to the antioxidant capacities, with higher coefficients using DPPH assay (r = 0.847 and 0.931) and FRAP assay (r = 0.811 and 0.918), indicating that anthocyanins and total phenols made a great contribution to the antioxidant capacities of either cloudy or clear juices. Ascorbic acid content was negatively related to the antioxidant capacities with coefficients (r = −0.244 to −0.143). A similar result was reported that phenolics and anthocyanins were both strongly correlated to the antioxidant capacity of fruits,[Citation29] whereas the ascorbic acid content and antioxidant capacity were inversely correlated. No significant changes in antioxidant capacities in cloudy and clear juices were detected after HHP treatment, which may be due to insignificant changes in antioxidative compounds in HHP-treated cloudy and clear juices. Other authors also reported that HHP treatment did not affect antioxidant capacity of liquid foods.[Citation10,Citation21] Zhang[Citation21] found that the FRAP ability of raw raspberry juice did not change after HHP treatment at 400–600 MPa/5–20 min/room temperature. Sánchez-Moreno et al.[Citation10] indicated that total scavenging activity (DPPH) in aqueous and organic fractions of tomato puree was unaffected by HHP treatment at 400 MPa/15 min/25°C.
Effects of HHP on Volatile Flavor Compounds in Cloudy and Clear Juices
More than 360 volatiles have been identified in strawberries, and the group consisted of esters, aldehydes, ketones, alcohols, and acids.[Citation30] According to aroma characteristics, the strawberry volatiles were divided into three types of fruit aroma, green-grass, and sweet aroma.[Citation30] The volatile flavor compounds detected by GC-MS in untreated cloudy and clear juices are shown in . The main volatile flavor compounds in untreated cloudy juices were esters (84.39%), in which ethyl acetate made up 73.82%, followed by butanoic acid ethyl ester (7.17%), hexanoic acid methyl ester (1.26%), acetic acid hexyl ester (1.06%), acetic acid phenylmethyl ester (1.04%), and phthalic acid isobutyl octyl ester (0.04%). Aldehydes were composed of 2-hexenal (2.81%), 2-hexen-1-ol acetate (1.49%), decanal (0.10%), and triethylsilanol (1.47%). 2-Hexenal is a volatile compound associated with the smell of grass.[Citation8] Ketone in untreated cloudy juices was 3(2H)-furanone, 4-methoxy-2, 5-dimethyl (0.44%), which was an important aroma compound related to the typical sweet aroma of strawberry although its content was lower.[Citation30]
There were 49 volatile flavor compounds in untreated clear juices (); the level of total volatile compounds is 80.54% higher than that in untreated cloudy juices. Especially, aldehydes and alcohols increase most significantly. Esters were 52.88% mainly including ethyl acetate (30.87%), hexanoic acid ethyl ester (9.28%), benzoic acid ethyl ester (4.05%), acetic acid hexyl ester (2.99%), butanoic acid methyl ester (2.61%), and butanoic acid butyl ester (1.66%). The content of 2-hexenal was increased by 54.97% after enzyme hydrolysis. The compounds, including acetic acid octyl ester (0.12%), butanoic acid butyl ester (0.55%), butanoic acid ethyl ester (0.47%), hexanoic acid ethyl ester (9.36%), benzoic acid ethyl ester (4.05%), octanoic acid methyl ester (0.09%), 1-octanol (0.27%), 2,6-dimethyl-2,4,6-octatriene (0.05%), benzene,1,2,3,4-tetramethyl (0.86%), alpha-Farnesene (0.02%), 1,3,6-octatriene,3,7-dimethyl (0.06%), 1-methyl-naphthalene (2.43%), 2-methyl-naphthalene (1.65%), naphthalene,2,7-dimethyl (0.36%), and naphthalene,2,6-dimethyl (0.03%), were newly detected in untreated clear juices, which were produced by an enzyme hydrolysis treatment. It was reported that apart from free flavor components, a significant part of important flavor compounds was accumulated as non-volatile and flavorless glycol-conjugates, which are known as glycosidic aroma precursors in strawberry.[Citation31] The enzyme hydrolysis treatment could not only release the volatile compounds from the bound fraction by hydrolyzing glycosides,[Citation32] but could also decrease the viscosity of the solution by degrading pectins, thus enabling the volatile components to escape from the matrix more easily. The use of pectinase enzyme preparations allowed the levels of monoterpenes, C13-norisoprenoids, and benzene derivatives to be increased. Li et al.[Citation32] found that all kinds of volatile compounds increased in litchi juice after pectin enzyme hydrolysis treatment, and the benzoic acid methyl ester and benzoic acid ethyl ester were newly detected.
As compared with untreated cloudy and clear juices, the content of total volatile compounds in HHP-treated cloudy juice increased by 13.21% while it decreased by 6.92% in HHP-treated clear juice. The homogenization effect of high pressure can improve the extraction of volatile compounds leading to the increase in volatile compounds in HHP-treated cloudy juice. The acid esters, including butanoic acid methyl ester, butanoic acid ethyl ester, and acetic acid hexyl ester, decreased in HHP-treated cloudy and clear juices. Lambert et al.[Citation19] reported that no changes in all the volatile flavor compounds were observed between untreated and HHP-treated strawberry coulis at 200 or 500 MPa/20 min/20°C, while a decrease in acid esters occurred at 800 MPa/20 min/20°C. The decrease in acid esters was attributed to the hydrolysis of acid esters under HHP treatment.[Citation19] The content of 2-hexenal in HHP-treated cloudy and clear juices increased by 5.88 and 4.29%, respectively. 3(2H)-Furanone, 4-methoxy-2, 5-dimethyl, one of the most important flavor compounds in strawberry, increased by 19.76 and 3.80% in HHP-treated cloudy and clear juices, respectively. However, Lambert et al.[Citation19] found that it did not change after HHP treatment. In HHP-treated cloudy juices, decanal, nonanal, acetic acid phenylmethyl ester, cyclodecane, and benzeneacetaldehyde were not detected, and nonanone, acetophenone, 1-butanol, 3-methyl acetate, and hexanoic acid were newly detected. Nonanone can be formed by the oxidation of nonanal and the hexanoic acid may form accompanied by the hydrolysis of hexanoic acid esters during HHP treatment. In HHP-treated clear juices, decanal and nonanal were lost, while 1-butanol, 3-methyl-,acetate, 1-hexanol, and 1-ethyl-1-hydroxy-1-silacyclopentane were detected as new compounds. Lambert et al.[Citation19] reported the concentration of many volatile compounds contributing to fresh strawberry flavor, such as nerolidol, linalool, and some ester compounds, was significantly lower in the strawberry puree processed at 800 MPa/20 min/20°C than that in untreated puree, and some new compounds, such as ɤ-latctone, were formed.
Effects of HHP on Color of Cloudy and Clear Juices
Effects of HHP on color of cloudy and clear juices are shown in . As compared to untreated juices, the lightness L* exhibited an increase in HHP-treated cloudy juices, while that of HHP-treated clear juices significantly increased (P < 0.05). The redness a* and yellowness b* of HHP-treated cloudy and clear juices showed no significant changes in this study, which was in agreement with previous studies. Zhang[Citation21] found that HHP treatment at 100–600 MPa/10–30 min had no significant effect on a* and b* of raw cloudy raspberry juices. Rodrigo et al.[Citation11] described a high retention of red color of strawberry juice after HHP treatment at 300–700 MPa/60 min/65°C. However, Patras et al.[Citation5] reported that a* value of strawberry and blackberry puree decreased after HHP treatment at 400–600 MPa/15 min. The strawberry and blackberry were not blanched before pulping, and there was residual PPO activity catalyzing the browning reaction during HHP treatment and contributed to significant color changes. Better color retention in this study was attributed to the total inactivation of PPO by blanching prior to juice extraction. Gimenez et al.[Citation33] reported that better color retention in jams treated by HHP (200–800 MPa/5 min) was due to better extraction of anthocyanins from the fruit matrix. ΔE values in HHP-treated juices were significantly different from those in untreated juices. ΔE values of cloudy juices is lower than 2 while that of clear juices was greater than 2, suggesting that there is a visible color difference in clear juices after HHP treatment. As compared to cloudy juices, the color difference susceptibility in clear juices was possibly ascribed to the lower content of antioxidant compounds (), which can protect the oxidation discolor.
CONCLUSION
This study indicated that no natural microorganisms and endogenous enzymes were detected in cloudy and clear strawberry juices after HHP treatments at 600 MPa/4 min/ambient temperature combined with steam blanching. Moreover, HHP treatment increased the cloud stability of cloudy juice since the PME was totally inactivated during the blanching process. The quality attributes, including anthocyanins, total phenols, antioxidant capacity, volatile flavors, and color, were well retained after HHP treatment. Blanching may be a good choice in combination with HHP in commercial practice to extend the shelf life and maintain the quality of strawberry juices. This study could be valuable to potential processors evaluating the effective commercialization of HHP-treated strawberry juices.
FUNDING
This work was supported by Project No. 101105046610000 of the Beijing Municipal Commission of Science and Technology of China and the “Novel Technologies and Equipments of Food Non-thermal Processing” (No. 2011AA100801) of 863 High-Tech Plan of China.
REFERENCES
- Oszmiajski, J.; Wojdyio, A. Phenolic acids, flavonoids, vitamin C and antioxidant capacity of strawberry juices processed by high-intensity pulsed electric welds or heat treatments. European Food Research and Technology 2008, 228, 623–631.
- Garcia-Palazon, A.; Suthanthangjai, W.; Kajda, P.; Zabetakis, I. The effects of high hydrostatic pressure on β-glucosidase, peroxidase and polyphenoloxidase in red raspberry (Rubus idaeus) and strawberry (Fragaria × ananassa). Food Chemistry 2004, 88, 7–10.
- Zhou, L.Y.; Wang, Y.Y.; Liao, X.J. Effect of high pressure carbon dioxide on the quality of carrot juice. Innovative Food Science & Emerging Technologies 2009, 10, 321–327.
- Zabetakis, I.; Koulentianos, A.; Orrunä, E.; Boyes, I. The effect of high hydrostatic pressure on strawberry flavor compounds. Food Chemistry 2000, 71, 51–55.
- Patras, A.; Brunton, N.P.; Pieve, S.D. Impact of high pressure processing on total antioxidant activity, phenolic, ascorbic acid, anthocyanin content and color of strawberry and blackberry purées. Innovative Food Science & Emerging Technologies 2009, 10, 308–313.
- Bala, B.; Farkas, D.; Turek, E.J. Preserving foods through high-pressure processing. Food Technology 2008, 11, 32–38.
- Zabetakis, I.; Leclerc, D.; Kajda, P. The effect of high hydrostatic pressure on the strawberry anthocyanins. Journal of Agriculture and Food Chemistry 2000, 48, 2749–2754.
- Oey, I.; Lille, M.; Loey, A.V.; Hendrickx, M. Effect of high-pressure processing on color, texture and flavour of fruit- and vegetable-based food products: A review. Trends in Food Science and Technology 2008, 19, 320–328.
- Krebbers, B.; Matser, A.M.; Hoogerwerf, S.W. Combined high pressure and thermal treatments for processing of tomato puree: Evaluation of microbial inactivation and quality parameters. Innovative Food Science & Emerging Technologies 2003, 4, 377–385.
- Sánchez-Moreno, C.; Plaza, L.; De Ancos, B.; Cano, M.P. Impact of high-pressure and traditional thermal processing of tomato purée on carotenoids, vitamin C and antioxidant activity. Journal of the Science of Food and Agriculture 2006, 86 (2), 171–179.
- Rodrigo, D.; Loey, A.N.; Hendrickx, M. Combined thermal and high pressure color degradation of tomato puree and strawberry juice. Journal of Food Engineering 2007, 79, 553–560.
- Baron, A.; Dénes, J.; Durier, C. High-pressure treatment of cloudy apple juice. LWT–Food Science and Technology 2006, 39, 1005–1013.
- Rao, L.; Guo, X.F.; Pang, X.L.; Tan, X.Y.; Liao, X.J.; Wu, J.H. Enzyme activity and nutritional quality of peach (Prunus persica) juice: Effect of high hydrostatic pressure. International Journal of Food Properties 2014, 17 (6), 1406–1417.
- Ahmed, J.; Ramaswamy, H.S.; Hiremath, N. The effect of high pressure treatment on rheological characteristics and color of mango pulp. International Journal of Food Science and Technology 2005, 40, 885–895.
- Yen, G.C.; Lin, H.T. Comparison of high pressure treatment and thermal pasteurisation on the quality and shelf life of guava purée. International Journal of Food Science and Technology 1996, 31, 205–213.
- Yen, G.C.; Lin, H.T. Effects of high pressure and heat treatment on pectic substances and related characteristics in guava juice. Journal of Food Science 1998, 63 (4), 684–687.
- Goodner, J.K.; Braddock, R.J.; Parish, M.E.; Sims, C.A. Cloud stabilization of orange juice by high pressure processing. Journal of Food Science 1999, 64 (4), 699–700.
- Cao, X.M.; Zhang, Y.; Zhang, F.S.; Wang, Y.T.; Yi, J.Y.; Liao, X.J. Effects of high hydrostatic pressure on enzymes, phenolic compounds, anthocyanins, polymeric color and color of strawberry pulps. Journal of the Science of Food and Agriculture 2011, 91, 877–885.
- Lambert, Y.; Demazeaua, G.; Largeteau, A. Changes in aromatic volatile composition of strawberry after high pressure treatment. Food Chemistry 1999, 67, 7–16.
- Dalmadi, I.; Rapeanu, G.; Loey, V.A.; Hendrickx, M. Characterization and inactivation by thermal and pressure processing of strawberry (Fragaria × ananassa) polyphenol oxidase: A kinetic study. Journal of Food Biochemistry 2006, 30, 56–76.
- Zhang, W.J. Effect of high hydrostatic pressure on the quality of raspberry juice. Master’s thesis, China Agriculture University, 2010.
- Gimenez, J.; Kajda, P.; Margomenou, L.; Piggott, J.R.; Zabetakis, I. A study on the color and sensory attributes of high-hydrostatic-pressure jams as compared with traditional jams. Journal of the Science of Food and Agriculture 2000, 81, 1228–1234.
- Chandler, B.U.; Robertson, G.L. Effect of pectic enzymes on cloud stability and soluble limonin concentration in stored orange juice. Journal of the Science of Food and Agriculture 1983, 34, 599–611.
- Pan, J.; Zeng, Q.M.; Xie, H.M.; Yang, Y.; Xu, H.Q. Study on UHP treatment of microflora sterilization of strawberry juice. Journal of Food Science 2004, 25 (1), 31–34.
- Song, D.D.; Ma, Y.K.; Song, W.J. Effect of high pressure treatment on enzymes and bacterium of carrot-strawberry juice. Food Machinery 2007, 23 (6), 39–41.
- Houska, M.; Strohalm, J.; Kocurova, K.; Totusek, J.; Lefnerova, D.; Triska, J. High pressure and foods—Fruit/vegetable juices. Journal of Food Engineering 2005, 77 (3) 386–398.
- Corredig, M.; Wicker, L. Juice clarification by thermostable fractions of marsh grapefruit pectin methylesterase. Journal of Food Science 2002, 67 (5), 1668–1671.
- Corrales, M.; García, A.F.; Butz, P.; Tauscher, B. Extraction of anthocyanins from grape skins assisted by high hydrostatic pressure. Journal of Food Engineering 2009, 90, 415–421.
- Kalt, W.; Forney, C.F.; Martin, A.; Prior, R.L. Antioxidant capacity, vitamin C, phenolics, and anthocyanins after fresh storage of small fruits. Journal of Agriculture and Food Chemistry 1999, 47, 4638–4644.
- Perez, A.G.; Rios, J.J.; Sanz, C. Aroma components and free amino acids in strawberry variety Chandler during ripening. Journal of Agriculture and Food Chemistry 1992, 40, 2232–2235.
- Pogorzelski, E.; Wilkowska, A. Flavour enhancement through the enzymatic hydrolysis of glycosidic aroma precursors in juices and wine beverages: A review. Flavour and Fragrance Journal 2007, 22, 251–254.
- Li, C.; Hao, J.; Zhong, H.; Dang, M.; Xie, B. Aroma components at various of litchi juice processing. Journal of the Science of Food and Agriculture 2009, 89 (14), 2405–2414.
- Gimenez, J.; Kajda, P.; Margomenou, L.; Piggott, J.R.; Zabetakis, I. A study on the color and sensory attributes of high-hydrostatic-pressure jams as compared with traditional jams. Journal of the Science of Food and Agriculture 2000, 81, 1228–1234.