Abstract
Low salt diets have become a trend due to the increase in health awareness. This study was carried out to investigate the quality and angiotensin I-converting enzyme inhibitory activity of low-salt sufu. Results showed that fermentation and the ripening process led to the release of peptides, greatly improving angiotensin I-converting enzyme inhibitory activity of low-salt sufu. Angiotensin I-converting enzyme inhibitory activity of low-salt sufu had thermostability, and its activity increased with a decrease in pH value. After in vitro protease digestion, angiotensin I-converting enzyme inhibitory activity increased significantly. When treated with protease alone, the sample digested with pepsin had the highest angiotensin I-converting enzyme inhibitory activity. Furthermore, the samples digested by gastrointestinal proteases in combination showed an even higher angiotensin I-converting enzyme inhibitory activity. Specifically, the sample digested by pepsin + α-chymotrypsin exhibited the lowest IC50 value of 0.015 mg/ml. These results suggested that low-salt sufu had a great potential to serve as a functional food in hypertension prevention.
INTRODUCTION
Angiotensin I-converting enzyme (ACE) is a dipeptidyl carboxypeptidase associated with the rennin-angiotensin system, which regulates peripheral blood pressure.[Citation1] ACE converts angiotensin I into angiotensin II (a potent vasoconstrictor), and it also inactivates bradykinin, which reduces blood pressure. Thus, inhibition of ACE in the organism generally results in an overall antihypertensive effect. Food-derived ACE inhibitors have been primarily attributed to bioactive peptides and many studies have focused on the production and isolation of ACE inhibitory peptides from food proteins.[Citation2] Fermentation has already been proved as one of the successful strategies to produce ACE inhibitory peptides. As a kind of protein-rich food, fermented soybean food has been widely reported to exhibit ACE inhibitory activity.[Citation3]
Sufu is a traditional fermented soybean food, which has been widely consumed as a condiment in China for centuries. It has been reported that sufu exhibited ACE inhibitory activity in vitro[Citation4] and antihypertensive activity in vivo.[Citation5] However, sufu is currently processed with high NaCl content (about 10% or higher), which is inconsistent with the health considerations of consumers. Moreover, some studies have indicated that higher NaCl content decreased some functional activities of fermented soybean foods.[Citation6] In our previous study, it was found that lower NaCl content could improve ACE inhibitory activity in sufu.[Citation7] Therefore, development of low-salt sufu is highly desired.
For the development of functional foods, it is necessary to examine the processing stability.[Citation8] Sufu has been consumed as a condiment in China since ancient times, and is sometimes used when cooking. During cooking, sufu always withstands heat treatment or varying pH conditions. Therefore, it is important to understand the processing stability of ACE inhibitory activity in low-salt sufu. Some food-derived peptides with ACE inhibitory activity in vitro have been well demonstrated to have ACE inhibitory activity in vivo and antihypertensive effects in animal experiments and clinical trials.[Citation9] However, some peptides with weak ACE inhibitory activity in vitro showed strong antihypertensive activity after oral or intravenous administration.[Citation10] One plausible explanation is that the peptide of weak ACE inhibitory activity may be converted into a stronger one by gastrointestinal proteases or by ACE in vivo, which is then absorbed intact to exhibit antihypertensive effects. Therefore, it is necessary to evaluate the impact of in vitro digestive protease on ACE inhibitory activity of low-salt sufu, which can provide a theoretical basis for further testing in vivo.[Citation11]
It should also be noted that, from the point of view of commercialization, it is a mixture of peptides, not a single, purified one that would be applied as a health-enhancing ingredient in “functional foods.”[Citation12] Li et al.[Citation13] found that ACE inhibitory peptides could be released from the inactive precursor proteins or oligopeptides during gastrointestinal digestion or food processing. An overall evaluation of the effects of processing and in vitro digestive protease on ACE inhibitory activity of low-salt sufu should be performed. The object of this study was to evaluate the quality properties (including chemical, microbiology, and sensory quantity) and ACE inhibitory activity of low-salt sufu. On this basis, the impact of processing and in vitro digestive protease on ACE inhibitory activity was investigated. Changes in the IC50 value before and after heat, pH, and digestive protease treatment (including pepsin, trypsin, and α-chymotrypsin alone and in combination) were examined.
MATERIALS AND METHODS
Chemicals
Angiotensin I-converting enzyme (from rabbit lung, EC.3.4.15.1), Hippuryl-His-Leu (HHL), o-phthaldialdehyde (OPA), pepsin (from porcine stomach mucosa, EC.3.4.23.1), trypsin (from bovine pancreas, EC.3.4.21.4), and α-chymotrypsin (from bovine pancreas, EC.3.4.21.1) were purchased from Sigma-Aldrich, Inc. (St. Louis, MO, USA). All solvents used for free amino acid analysis were of HPLC grade. All other chemicals were of analytical grade.
Samples Preparation
Low-salt sufu was prepared at the Wang-Zhihe sufu manufacturing company in Beijing. Tofu was obtained by coagulation from soymilk, pressed to obtain a firm consistency, and then cut into pieces (3.2 × 3.2 × 1.6 cm3). The pieces were inoculated with Actinomucor elegans AS 3.227 by spraying inoculum to their surface. Fermentation was carried out at 28°C and a relative humidity of around 90% with air circulation to ensure adequate aeration. Pehtze was obtained after fermentation for 48 h. The pehtze was then transferred into a container and NaCl was spread between layers of pehtze as they were piled up in the container. Salted pehtze was obtained after salting for 5 days. The salted pehtzes were placed in a closed glass bottle with the dressing mixture consisting of alcohol (final concentration of 10%), red kojic rice, sugar, flour paste, and spices. The filled bottles were incubated at 25–28°C for 60 days. Tofu, pehtze (fermented for 48 h), salted pehtze (salted for 5 days), and low-salt sufu (ripened for 60 days) were sampled for analysis, respectively. Two popular commercial red sufu samples were purchased from a supermarket in Beijing, which were used as control samples in the sensory evaluation test.
Chemical Analysis
The pH was measured using a digital pH meter (F-23, Horiba, Japan). Amino nitrogen and total acid content were determined using a titration method.[Citation14] Water-soluble protein, moisture, and NaCl content were determined by the official methods of AOAC.[Citation15] Peptide content was determined by an OPA spectrophotometric assay, which was described by Church et al.[Citation16] with some modifications. Free amino acids were determined using a HPLC precolumn phenylisothiocyanate derivatization method described by Hu et al.[Citation17] The concentrations of amino acids in samples were calculated by calibrating with standard amino acids (Sigma Chemical Co., St. Louis, MO, USA). All determinations were performed in triplicate.
Microbiological Analysis
Ten grams of low-salt sufu samples were aseptically transferred into 90 mL of sterile physiological saline solution and sequentially diluted 10-fold. The total count of mesophilic aerobic bacteria (TMAB) was enumerated in pour plates containing plate count agar (PCA; CM325, Oxoid, England) after incubation at 30°C for 48 h. Lactic acid bacteria (LAB) was incubated at 30°C for 48 h on De Man Rogosa Sharper agar (MRS; AOBOX, Beijing, China) supplemented with 0.1% (w/v) natamycin to prevent fungal growth. Fungal populations were determined in pour plates of standard and salt-enriched oxytetracycline glucose-yeast extract agar (OGYE; CM545, Oxoid, England) to which 0 g and 25 g NaCl was added per liter, respectively, after incubation at 28°C for 5 days. The results were expressed as log cfu/g of sample.
Sensory Evaluation
Low-salt sufu samples fermented for 60 days were evaluated for sensory quality. Two popular commercial red sufu samples produced by different manufacturers served as controls. A panel was made up of 10 students from the College of Food Science and Nutritional Engineering, China Agricultural University, Beijing, China, and the panelists were all familiar with the food product, while they were also instructed on the use of sensory evaluation procedures. Each panelist was asked to rate the samples on the basis of color, aroma, taste, texture, and overall acceptability using a five-point hedonic scale. Each parameter was assigned a rating value: from 5 for the “like very much” level of acceptance down to 1 for the “dislike very much” level of acceptance (i.e., 5 = like extremely; 3 = neither like nor dislike; 1 = dislike extremely). Each sample was presented in round white ceramic plates at random, and was judged twice by each panelist. Panelists were asked to rinse their mouths between each sample. All sessions were held in a sensory panel booth room equipped with white fluorescent lighting.
Preparation of Water Extract
One gram of each dried sample was suspended in 10 ml of distilled water, and homogenized for 1 min. After sonication for 5 min, the suspension was shaken at room temperature for 1 h and then boiled for 15 min. The mixture was centrifuged at 12,000× g for 15 min and filtered using a 0.45-μm membrane. The filtrate was used as water extract with a concentration of 100 mg/ml.
Determination of ACE Inhibitory Activity in Vitro
ACE inhibitory activity was measured by using the modified method described by Horie.[Citation18] An amount of 20 μl of aqueous extract was mixed with 40 μl of ACE solution (12.5 munits/ml) and 40 μl of substrate solution (4.66 mM HHL in 400 mM phosphate buffer containing 600 mM NaCl, pH 8.3) and then incubated at 37°C for 1 h. The reaction was terminated by adding 150 μl of 1.2 M NaOH solution. The mixture was incubated for 20 min at room temperature after the addition of 40 μl of 2% OPA dissolved in methanol. The derivation reaction was terminated by adding 40 μl of 6 M HCl solution. The fluorescence intensity was measured using a spectrofluorophotometer (RF-5300PC, Shimadzu Co., Kyoto, Japan) under the following conditions: Ex, 340 nm; Em, 455 nm; slit width, 5 nm. ACE inhibitory activity percentage (ACEi%) was calculated as follows: ACEi% = [1 − (A − C) ÷ (B − D)] × 100, where A is the intensity with the sample, B is the intensity without the sample, C is the intensity without ACE, and D is the intensity of the buffer. The median inhibitory concentration (IC50) value is defined as the sample concentration that inhibits 50% of ACE activity under the assay conditions.
Heat Treatment and pH Stability Test
Effects of heat treatment and pH on ACE inhibitory activity of low-salt sufu were evaluated based on the methods of Wu and Ding[Citation12] with some minor modifications. Water extracts were incubated at temperatures of 40, 60, 80, 100, and 120°C for 2 h. The water extracts were also incubated at pH values of 2, 4, 6, 8, and 10 for 2 h. After the solutions were acclimated to room temperature, pH was adjusted to 8.3 for the measurement of ACE inhibitory activity. The water extract of low-salt sufu before heat treatment and undergoing a change in pH served as control.
In Vitro Gastrointestinal Digestion
The enzymatic digestion of sample extract was carried out according to the method of Parrot et al.[Citation19] with some modifications. To start, 300 μl of water extract were mixed with 1200 μl of 0.1 M Tris-HCl buffer (pH 2.0, for pepsin), 0.1 M phosphate buffer (pH 8.5, for trypsin), and 0.1 M phosphate buffer (pH 8.5, for α-chymotrypsin), respectively. Pepsin, trypsin, and α-chymotrypsin (1%, w/v) were then added to the corresponding mixture. The reaction mixtures were incubated at 37°C for 5 h, and boiled for 10 min to stop the reaction. Next, 200 μl of reaction solution treated with pepsin was mixed with 800 μl of phosphate buffer. Trypsin (1%, w/v), α-chymotrypsin (1%, w/v), or trypsin (0.5%, w/v) and α-chymotrypsin (0.5%, w/v) were added separately to three aliquots of the same mixture. The reaction mixtures were incubated at 37°C for 5 h, and boiled for 10 min to stop the reaction. After cooling to room temperature, the six reaction mixtures were centrifuged (12,000× g, 10 min), and the supernatant was filtered using a 0.45-μm membrane for measuring ACE inhibitory activity. The water extract of low-salt sufu before protease treatment served as the control.
Statistical Analysis
Experiments were performed in triplicate and repeated at least three times. All statistical calculations were performed using SPSS version 16.0, and the obtained values were presented as mean ± SD. Significant differences were determined by analysis of variance, followed by Duncan’s multiple-range tests (p < 0.05).
Table 1 Chemical properties for low-salt sufu during the production process
RESULTS AND DISCUSSION
Quality Properties of Low-Salt Sufu during Production Process
The quality properties of low-salt sufu, including chemical, microbiological, and sensory properties, were evaluated. shows the chemical properties for low-salt sufu during the production process; it was found that the tofu cube displayed an initial pH value and acidity of 6.35 and 0.67%, respectively. Hereafter, the pH decreased slightly while the acidity increased after the pehtze stage. Although there was no significant difference among pH values during the production process, the differences among total acid contents were significant. Amino nitrogen content is often used as a chemical indicator to determine the fermentation degree of sufu in China.[Citation20] During the production process, an increase in the amino nitrogen content was noted except in the pehtze stage. A similar phenomenon was observed in the changes of water-soluble protein content. In addition, it was also noted that low-salt sufu showed moisture and NaCl content of 62.42 and 4.65%, respectively ().
Changes in microbiological properties are presented in , which showed that TMAB, LAB, and fungal counts were low in tofu, while a considerable increase was observed in pehtze as expected. Significant declines in microbial count occurred after salting. There were lower levels of TMAB and LAB in the low-salt sufu product than in the salted pehtze. This could be due to the use of ethanol and red kojic rice in the dressing mixture of low-salt sufu. Ethanol has been found to be widely used in the production of low-salt fermented soybean foods to inhibit microbial overgrowth.[Citation21] Antimicrobial effect of red yeast rice has also been reported.[Citation22] Almost no fungal (<1 log cfu/g) was detected in the low-salt sufu product, which was consistent with previous reports on sufu. It is likely that the fungal, particularly the mold starters, did not survive in the sufu product due to the effects of the ingredients used in the dressing mixtures.
Figure 1 Microbial counts for low-salt sufu during production process. TMAB: total count of mesophilic aerobic bacteria; LAB: lactic acid bacteria. Error bars show the standard deviations. Values are the means of triplicate analyses. Different letters (a, b, c, and d) at the top of the bars indicate significant differences (p < 0.05).
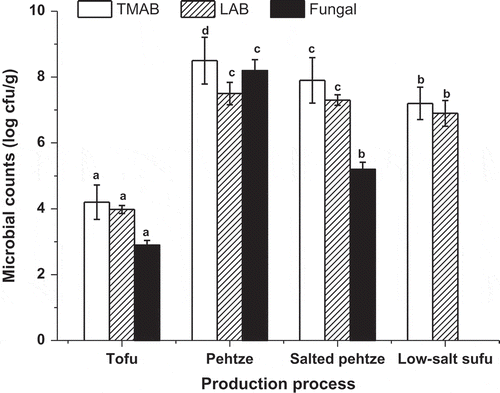
The sensory quality ratings of low-salt sufu and two kinds of commercial sufu samples are presented in , which showed that low-salt sufu was rated highest in terms of aroma and taste, while having a lower score than that of the commercial products in terms of color and texture. Results of overall acceptability showed that the panelists considered low-salt sufu equal to or even better, when compared with the control samples (P < 0.05).
Figure 2 Sensory evaluation for two commercial sufu samples (Control A, Control B) and low-salt sufu product. Control A and Control B are two popular commercial red sufu samples produced by different manufacturers. Values are mean values of panelists’ ratings. Error bars show the standard deviations. Different letters (a, b, and c) at the top of the bars indicate significant differences (p < 0.05).
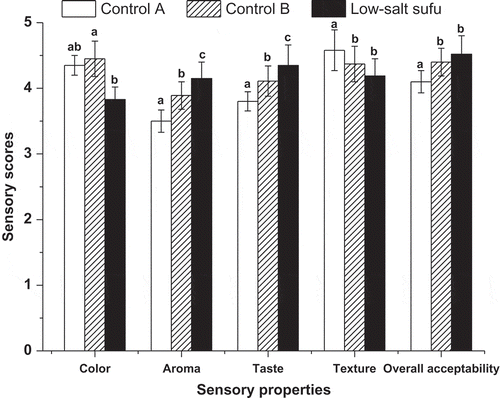
Changes in ACE Inhibitory Activity and Peptide Content of Low-Salt Sufu during the Production Process
The ACE inhibitory activity of low-salt sufu during the production process, expressed as ACEi%, is presented in , which showed that ACE inhibitory activity of tofu was low, but after 48 h fermentation, it increased by 2.1 times. During the salting process, however, ACE inhibitory activity experienced a slight decrease. The highest ACE inhibitory activity was observed in the low-salt sufu product, which was 4.4 times higher than that in tofu. These results suggested that the production process, particularly the ripening process, led to the generation of ACE inhibitors in low-salt sufu. Many fermented soybean foods exhibited ACE inhibitory activity and antihypertensive effect.[Citation23] The ACE inhibitory activity of fermented soybean foods has been mainly attributed to bioactive peptides. Changes in peptide content are also presented in , which showed that there was a similar trend between peptide content and ACE inhibitory activity. Compared with tofu, the peptide content of low-salt sufu had a significant increase, which could be due to the hydrolysis of soybean proteins by proteases derived from microorganisms.
Figure 3 ACE inhibitory activity and peptide content for low-salt sufu during production process. The concentration of water extract used for ACE inhibitory activity test was 10 mg/ml. Values are the means of triplicate analyses. Error bars show the standard deviations. Different letters (a, b, c, and d) at the top of the bars indicate significant differences (p < 0.05).
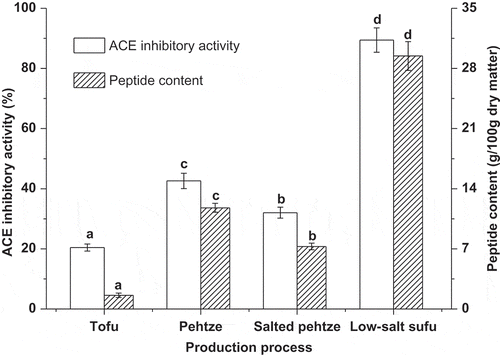
Effects of Heat Treatment and pH on ACE Inhibitory Activity of Low-Salt Sufu
In order to understand the processing stability of ACE inhibitory activity in low-salt sufu, changes in the IC50 value before and after heat and pH treatment were examined. Effects of heat treatment on ACE inhibitory activity are presented in , which showed that heat treatment caused a slight decrease in ACE inhibitory activity, but there were no significant differences among the control sample and samples treated at different temperatures (p < 0.05). This suggested that ACE inhibitory activity of low-salt sufu showed thermostability. As a condiment, sufu was sometimes used during cooking, so it was of practical significance to examine the thermal stability of its ACE inhibitory activity. At present, peptide is considered to be an ACE inhibitor.[Citation24] ACE inhibitory activity of different peptides exhibited different thermal stability. Wu and Ding[Citation12] demonstrated that soy-protein-derived ACE inhibitory peptides retained activity under a series of temperature treatments. However, Tsuda and Miyamoto[Citation25] found that ACE inhibitory activity of some peptides derived from fermented milk reduced with an increase in treatment temperature and time.
Figure 4 Effect of heat treatment and pH on ACE inhibitory activity in low-salt sufu. The water extract of low-salt sufu before heat treatment was served as control. Values are the means of triplicate analyses. Error bars show the standard deviations. Different letters (a, b, c, d, and e) at the top of the bars indicate significant differences (p < 0.05).
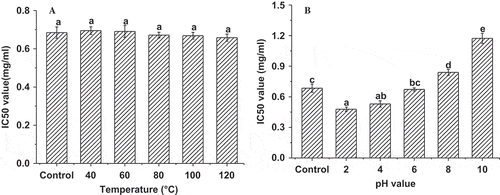
It is important to know the effect of pH on ACE inhibitory activity as sufu is often subjected to pH changes during processing or digestion. As shown in , ACE inhibitory activity increased with a decrease in pH value, suggesting that ACE inhibitory activity of low-salt sufu increased under acidic conditions, while it decreased under alkaline conditions. Compared with the control, samples at pH 2 and pH 4 conditions showed considerable ACE inhibitory activities with IC50 values of 0.48 and 0.53 mg/ml, respectively. It has been shown that some ACE inhibitory activity peptides displayed stability under varying pH conditions,[Citation26] which was inconsistent with the results of this present study. This was likely due to the fact that ACE inhibitory activity peptides of low-salt sufu involved complex components, generated by a variety of protease secreted by micro-organisms. In addition, the hydrolysis extent of protein or peptide was different under different acidic or alkali conditions, which might impact ACE inhibitory activity of low-salt sufu.
Effect of in Vitro Digestion on ACE Inhibitory Activity in Low-Salt Sufu
Changes in IC50 value before and after in vitro digestion with gastrointestinal proteases, including pepsin, trypsin, and α-chymotrypsin alone and in combination, were examined. As shown in , ACE inhibitory activity was 6.2, 4.7, and 1.8 times higher than the control sample after digestion by pepsin, trypsin, and α-chymotrypsin, respectively. ACE inhibitory activity after in vitro protease digestion in combination increased notably. The highest ACE inhibitory activity was observed in a low-salt sufu sample digested by pepsin and α-chymotrypsin in combination, with an IC50 value of 0.015 mg/ml. These results suggested that in vitro protease digestion greatly enhanced ACE inhibitory activity of low-salt sufu, which was also found in other foods.[Citation27]
Figure 5 Effect of in vitro protease digestion on ACE inhibitory activity in low-salt sufu. The water extract of low-salt sufu before protease treatment was served as control. Values are the means of triplicate analyses. Error bars show the standard deviations. Different letters (a, b, c, and d) at the top of the bars indicate significant differences (p < 0.05).
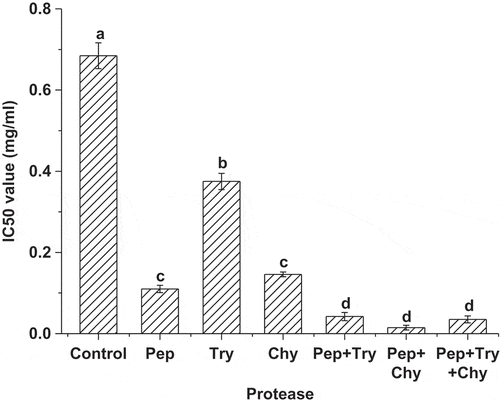
Pepsin, trypsin, and α-chymotrypsin were also used in the enzymatic hydrolysis of food protein to release ACE inhibitory activity peptides.[Citation28] Zhang et al.[Citation29] observed that ACE inhibitory activity of douchi qu increased greatly after preincubation with gastrointestinal proteases. It has been demonstrated that ACE inhibitory activity could be generated from muscle proteins by hydrolysis of gastrointestinal proteases.[Citation30] Lignitto et al.[Citation31] also observed that short peptides with higher ACE inhibitory activity could be generated by gastrointestinal digestion. The present results were in accordance with previous reports. Soy protein in low-salt sufu further digested by gastrointestinal proteases may possibly produce active peptides of small molecular weight, further leading to the significant increase of ACE inhibitory activity.
In the present study, in the case of single protease treatment, it was found that the low-salt sufu sample digested by pepsin showed the highest ACE inhibitory activity, which was related to enzymatic properties. Gibbs et al.[Citation32] found that pepsin had a low specificity and could hydrolyze protein to produce more oligopeptides and active peptides, while trypsin had a relatively high specificity. It is typically noted that pepsin has the capability of hydrolyzing peptide bonds from the carboxyl terminus of hydrophobic and aromatic amino acids, thus generating peptides with a C-terminus that has hydrophobic and aromatic amino acid residues. Studies have shown that this structure was conducive to the combination of peptides and ACE to generate strong ACE inhibitory activity.[Citation13] The samples treated with proteases in combination showed higher ACE inhibitory activity than those treated with protease alone (), which might be the effect of enzyme synergy. Overall, ACE inhibitory activity was significantly improved after in vitro protease digestion, which suggested that ACE inhibitory activity of low-salt sufu had practical value, and also providing the theoretical basis for further animal experimentation.
Since protein is the major component related to ACE inhibitory activities of sufu, it is necessary to investigate the changes in individual free amino acids contents of low-salt sufu with different protease treatments. The results are presented in , which showed that total free amino acids and most of individual amino acid generally increased in low-salt sufu with different protease treatment. The level of total free amino acid increased from 50 to 65 mg/g dry matter (1.3-fold) treated with pepsin, whereas 1.5- and 1.6-fold increases were observed in samples treated with trypsin and α-chymotrypsin. It was also shown that total free amino acid content of samples treated with proteases in combination were higher than that treated with single protease. Among the various free amino acids examined, the six highest concentrations of free amino acids, except for glutamic, were all hydrophobic amino acids. After protease treatment, the hydrophobic amino acid content increased significantly. Studies had shown that hydrophobic amino acids in ACE inhibitors played an important role on ACE inhibitory activity.[Citation33] In the present study, high content of hydrophobic amino acids was observed in samples, which might be related with their ACE inhibitory activities.
Table 2 Changes in individual free amino acids contents of low-salt sufu with different protease treatments
In the present study, low-salt sufu with good quality and high ACE inhibitory activity was produced. ACE inhibitory activity of low-salt sufu was mainly generated during the fermentation and ripening processes, which was closely related to the release of peptides. ACE inhibitory activity of low-salt sufu was stable under heat treatment, while ACE inhibitory activity increased with a decrease in pH value. These results provided references for practical production. ACE inhibitory activity of low-salt sufu drastically increased after in vitro digestive protease, which suggested that low-salt sufu was expected to have anti-hypertensive effects, and also provided a theoretical basis for further animal testing. Thus, it could be concluded that low-salt sufu had the potential to develop as a functional food capable of lowering blood pressure. However, ACE inhibitors in low-salt sufu should be isolated, purified, and compared with well-known ACE-inhibiting compounds, such as captopril, when developing a new ACE inhibitor or antihypertensive drug.
ACKNOWLEDGMENT
The authors are grateful to the Wang-Zhihe sufu manufacturing company.
FUNDING
This research was financially supported by Beijing Ershang Wangzhihe Food Co., Ltd. This research was also carried out with the financial support of the National Key-Technologies R&D Program (No. 2012BAD34B03) and National Science Foundation of China (No. 31171739). The authors also acknowledge the support from the Program for New Century Excellent Talents in University (NCET-10-0776).
REFERENCES
- Erdös, E. The angiotensin I converting enzyme. Federation Proceedings 1977, 36, 1760–1765.
- Yu, Z.; Liu, B.; Zhao, W.; Yin, Y.; Liu, J.; Chen, F. Primary and secondary structure of novel ACE-inhibitory peptides from egg white protein. Food Chemistry 2012, 133, 315–322.
- Li, F.J.; Yin, L.J.; Lu, X.; Li, L.T. Changes in angiotensin I-converting enzyme inhibitory activities during the ripening of douchi (a Chinese traditional soybean product) fermented by various starter cultures. International Journal of Food Properties 2010, 13, 512–524.
- Wang, L.; Saito, M.; Tatsumi, E.; Li, L. Antioxidative and angiotensin I-converting enzyme inhibitory activities of sufu (fermented tofu) extracts. Japan Agricultural Research Quarterly 2003, 37, 129–132.
- Kuba, M.; Shinjo, S.; Yasuda, M. Antihypertensive and hypocholesterolemic effects of tofuyo in spontaneously hypertensive rats. Journal of Health Science 2004, 50, 670–673.
- Chen, J.; Cheng, Y.Q.; Yamaki, K.; Li, L.T. Anti-α-glucosidase activity of Chinese traditionally fermented soybean (douchi). Food Chemistry 2007, 103, 1091–1096.
- Ma, Y.; Cheng, Y.; Yin, L.; Wang, J.; Li, L. Effects of processing and NaCl on angiotensin i-converting enzyme inhibitory activity and γ-aminobutyric acid content during sufu manufacturing. Food and Bioprocess Technology 2012. DOI:10.1007/s11947-012-0852-3.
- Shimoni, E. Stability and shelf life of bioactive compounds during food processing and storage: Soy isoflavones. Journal of Food Science 2004, 69, R160–R166.
- Shin, Z.I.; Yu, R.; Park, S.A.; Chung, D.K.; Ahn, C.W.; Nam, H.S.; Kim, K.S.; Lee, H.J. His-His-Leu, an angiotensin I converting enzyme inhibitory peptide derived from Korean soybean paste, exerts antihypertensive activity in vivo. Journal of Agricultural and Food Chemistry 2001, 49, 3004–3009.
- Kuwabara, Y.; Nagai, S.; Yoshimitsu, N.; Nakagawa, I.; Watanabe, Y.; Tamai, Y. Antihypertensive effect of the milk fermented by culturing with various lactic acid bacteria and a yeast. Journal of Fermentation and Bioengineering 1995, 80, 294–295.
- Ruiz, J.Á.G.; Ramos, M.; Recio, I. Angiotensin converting enzyme-inhibitory activity of peptides isolated from Manchego cheese. Stability under simulated gastrointestinal digestion. International Dairy Journal 2004, 14, 1075–1080.
- Wu, J.; Ding, X. Characterization of inhibition and stability of soy-protein-derived angiotensin I-converting enzyme inhibitory peptides. Food Research International 2002, 35, 367–375.
- Li, G.H.; Le, G.W.; Shi, Y.H.; Shrestha, S. Angiotensin I-converting enzyme inhibitory peptides derived from food proteins and their physiological and pharmacological effects. Nutrition Research 2004, 24, 469–486.
- Judoamidjojo, M. The studies on kecap: Indigenous seasoning of Indonesia. Memoirs of the Tokyo University of Agriculture 1986, 28, 97–159.
- Horwitz, W.; Latimer, G.W. Official Methods of Analysis of AOAC International; AOAC International: Gaithersburg, MD, 2000.
- Church, F.C.; Swaisgood, H.E.; Porter, D.H.; Catignani, G.L. Spectrophotometric assay using o-phthaldialdehyde for determination of proteolysis in milk and isolated milk proteins. Journal of Dairy Science 1983, 66, 1219–1227.
- Hu, H.P.; Hao, J.X.; Cheng, Y.Q.; Yin, L.J.; Ma, Y.L.; Yu, Z.W.; Li, L.T. Effect of fermented rice culture on the microbiological, biochemical and sensory characteristics of low-salt douchi, a traditional Chinese fermented soybean condiment. International Journal of Food Science and Technology 2012, 47, 689–695.
- Horie, H. Handbook of Functional Evaluation on Food; Japanese Ministry of Agriculture, Forestry and Fisheries. Agriculture, Forestry and Fishery Research Council, National Food Research Institute: Tokyo, Japan, 1999; 117–121.
- Parrot, S.; Degraeve, P.; Curia, C.; Martial-Gros, A. In vitro study on digestion of peptides in Emmental cheese: Analytical evaluation and influence on angiotensin I converting enzyme inhibitory peptides. Food/Nahrung 2003, 47, 87–94.
- Wu, B.; Li, T.; Chen, H.; Shen, J. Cost-effectiveness of nucleoside analog therapy for hepatitis B in China: A Markov analysis. Value in Health 2010, 13, 592–600.
- Chiou, R.Y.Y.; Ferng, S.; Beuchat, L. Fermentation of low-salt miso as affected by supplementation with ethanol. International Journal of Food Microbiology 1999, 48, 11–20.
- Kalaivani, M.; Sabitha, R.; Kalaiselvan, V.; Rajasekaran, A. Health benefits and clinical impact of major nutrient, red yeast rice: A review. Food and Bioprocess Technology 2010, 3, 333–339.
- Oh, Y.S.; Hwang, J.H.; Lim, S.B. Physiological activity of tofu fermented with mushroom mycelia. Food Chemistry 2012, 133, 728–734.
- Ren, F.; Zhang, S.; Guo, H.; Jiang, L. Systemic screening of milk protein-derived ACE inhibitors through a chemically synthesised tripeptide library. Food Chemistry 2011, 128, 761–768.
- Tsuda, H.; Miyamoto, T. Angiotensin I-converting enzyme inhibitory peptides in skim milk fermented with Lactobacillus helveticus 130B4 from camel milk in Inner Mongolia, China. Journal of the Science of Food and Agriculture 2008, 88, 2688–2692.
- Hwang, J.S. Impact of processing on stability of angiotensin I-converting enzyme (ACE) inhibitory peptides obtained from tuna cooking juice. Food Research International 2010, 43, 902–906.
- Akıllıoğlu, H.G.; Karakaya, S. Effects of heat treatment and in vitro digestion on the angiotensin converting enzyme inhibitory activity of some legume species. European Food Research and Technology 2009, 229, 915–921.
- Chiang, W.D.; Tsou, M.J.; Tsai, Z.Y.; Tsai, T.C. Angiotensin I-converting enzyme inhibitor derived from soy protein hydrolysate and produced by using membrane reactor. Food Chemistry 2006, 98, 725–732.
- Zhang, J.H.; Tatsumi, E.; Ding, C.H.; Li, L.T. Angiotensin I-converting enzyme inhibitory peptides in douchi, a Chinese traditional fermented soybean product. Food Chemistry 2006, 98, 551–557.
- Arihara, K.; Nakashima, Y.; Mukai, T.; Ishikawa, S.; Itoh, M. Peptide inhibitors for angiotensin I-converting enzyme from enzymatic hydrolysates of porcine skeletal muscle proteins. Meat Science 2001, 57, 319–324.
- Lignitto, L.; Cavatorta, V.; Balzan, S.; Gabai, G.; Galaverna, G.; Novelli, E.; Sforza, S.; Segato, S. Angiotensin-converting enzyme inhibitory activity of water-soluble extracts of Asiago d’allevo cheese. International Dairy Journal 2010, 20, 11–17.
- Gibbs, B.F.; Zougman, A.; Masse, R.; Mulligan, C. Production and characterization of bioactive peptides from soy hydrolysate and soy-fermented food. Food Research International 2004, 37, 123–131.
- Alemán, A.; Giménez, B.; Pérez-Santin, E.; Gómez-Guillén, M.C.; Montero, P. Contribution of Leu and Hyp residues to antioxidant and ACE-inhibitory activities of peptide sequences isolated from squid gelatin hydrolysate. Food Chemistry 2011, 125, 334-341.