Abstract
This study investigated the structural and physical properties of starches extracted from six varieties of millet mainly grown in China. The amylose content of millet starches ranged from 17.6 to 28.4 g/100 g (dry weight basis, db), and a variety of waxy proso millet starch (0.38 g/100 g, db) was identified. These starches exhibited a majority of large polygonal granules with several indentations, few small spherical granules, and A-type crystallinity. Proso millet starch showed prominent paste clarity and freeze-thaw stability, whereas finger millet starch exhibited the highest solubility. Moreover, barnyard millet starch had the lowest sediment volume. The gelatinization enthalpy of proso millet starch was the highest (5.16 J/g). For non-waxy millet starch, the ratio of retrogradation (R%) was positively correlated with amylose content. Among all starches, the storage modulus (G′) was higher than the loss modulus (G″) during heating and cooling. The G′ and G″ of proso millet starch and barnyard millet starch pastes were significantly lower than other starches during cooling. The pasting properties of six millet starches significantly differed, had positive correlation with amylose content, and were affected by NaCl, sucrose, and pH values.
INTRODUCTION
Millet is one of the oldest human foods and believed to be the first domesticated cereal grain.[Citation1] India, Nigeria, Niger, and China are the world’s largest growers of millet.[Citation2] The millet crop can be produced on light, well-drained soils that are poor in organic matter and low rainfall, as well as, on the arid or the semi arid areas and poor sandy or saline-alkali soil areas. Moreover, millet crops provide high biomass production.[Citation3,Citation4] This crop is used primarily for human food and remains a major source of calories and a vital component of food security in harsh natural environments areas in the developing world. As the desert encroachment and environmental protection persist, more states may be involved in the production of millet, being a resistant crop. To date millet ranks as the sixth most important cereal grain worldwide, sustaining more than one-third of the world’s population.
Millet is receiving increasing attention as a grain crop because of its resistance to poor growth stresses and its high nutritional value. Millet is a gluten-free and typically allergy-friendly food. It can be recommended for use in the management of cardiovascular diseases and diabetes in human beings.[Citation5,Citation6] Barnyard millet has been identified as highly nutritious, rich in proteins, lipids, and vitamins B1 and B2 compared with other cereals, such as rice and wheat grain. Japanese barnyard millet grains have been used as a functional food for patients with allergic diseases, including atopic dermatitis.[Citation7]
The presence of all the required nutrients in the millets leads to their suitability for large-scale utilization in the manufacture of various food products.[Citation8,Citation9] Millet is a starchy cereal that usually contains 50–70 g/100 g of starch (dry weight basis, db). Starch constitutes the main component of millet grain and has an important role in its food applications, such as in breakfast cereals, muffins, cookies, snacks, pastas, and health foods. The properties of millet starch have been characterized in some studies. Single-millet starch, including those from proso, foxtail, pearl, and finger millets, has already been studied.[Citation10–Citation14] The grain has variable shape, size, and color. Several studies have been also reported on the physicochemical properties of varieties of millet starches.[Citation5,Citation15] However, obtaining an adequate understanding of the physical properties of different millet starches and their distinctions are difficult. In the present study, six varieties of millet mainly cultivated in China were selected to characterize its structural and physical properties of starches. The data from the investigation would be relevant for various food and non-food utilizations of the starches.
MATERIALS AND METHODS
Materials
Six varieties of millet were mainly cultivated in China and harvested in 2011, which were provided by Liaoning Academy of Agricultural Sciences (Liaoning Province, China). The isolated starches are proso millet starch (Panicum miliaceum, PSMS), hybrid barnyard millet starch (Echinochloa frumentacea, HBMS), barnyard millet starch (Echinochloa frumentacea, BYMS), finger millet starch (Eleusine coracana, FGMS), foxtail millet starch (Setaria italica, FTMS), and pearl millet (Pennisetum glaucum, PLMS).
Isolation of Starch
The starch was isolated according to the following process. The grain was washed, and then steeped in 0.2% (w/v) aqueous NaOH solution at 4 to 6°C for 24 h. The softened grains were ground to slurry and sieved through 100, 250, and 400 mesh screens, respectively. The filtrate was placed in 4°C for 12 h to allow the starch layer to settle. The supernatant was discarded, and the top yellow protein layer was removed. The lower starch layer was subsequently washed with 0.2% (w/v) NaOH and 0.5% (w/v) sodium dodecyl sulfonate to discard remnant protein, and then resuspended in double deionised water. This procedure was repeated twice. The prime starch was obtained and dried at 40°C, and then stored in a desiccator. The contents of moisture, ash, protein, amylose, and starch purity were determined using standard procedures.[Citation16]
Scanning Electron Microscopy (SEM)
The native starch granules were mounted on double-sided adhesive tape and attached to specimen stubs. Samples were coated with a thin layer of carbon in vacuo and approximately 150 Å of gold. Afterward, the samples were viewed, examined, and photographed using an ETEC-autoscan scanning electron microscope (Sirion 200, FEI Company, Holland) at an accelerating voltage of 20 kV. Every variety of starch granules was observed at two SEM magnifications 5000 and 10000 ×, respectively.
X-ray Diffractometry
X-ray powder diffraction measurements were conducted using an X-ray diffractometer (D/max 2500, Japan). Each sample of millet starches were packed tightly in a rectangular glass cell (15 × 10 mm, thickness 0.15 cm). The samples were exposed to the X-ray beam from the X-ray generator running at 40 kV and 250 mA. The scanning regions of the diffraction angle 2θ were 5 to 40°, which covered most of the significant diffraction peaks of the starch crystallites. MDI Jade 5.0 software was used to analyze the diffractograms.
Water Solubility Index (WSI) and Swelling Power (SP)
Starch (1 g) was heated with 30 mL of water at 95°C for 1 h with constant stirring. After cooling to room temperature, the dispersion was centrifuged at 3000 rpm for 15 min. Starch sediment was weighed. Supernatant was carefully dumped into a preweighed petri dish and dried at 130°C until a constant weight is achieved, and then weighed. WSI and SP were calculated as follows:
Paste Clarity
A 0.5% (dry basis, w/v) aqueous suspension of starch was heated in a boiling water bath for 30 min with constant stirring, and then cooled to room temperature. The transmittance of the paste was measured at 650 nm against a water blank using 722 s spectrophotometer.
Sediment Volume
A 2% (dry basis, w/v) aqueous suspension of starch was heated in a boiling water bath for 30 min, moved into measuring cylinder (100 mL) with plug, and then left to cool at room temperature for 24 h. Finally, its sediment volume was measured and expressed with mL/100 mL.
Freeze-Thaw Stability
A 3% (dry basis, w/v) aqueous suspension of starch was heated in a boiling water bath for 30 min and cooled to room temperature. The paste (Wp) was then weighed and stored in freezer at –20 to –15°C. After 24 h, the sample was thawed naturally, and then centrifuged at 3000 rpm for 20 min. The upper layer water was discarded and the sediment (Ws) was weighed. The separated water is expressed as follows:
Thermal Properties
The thermal characteristics of the isolated starches were analyzed using a differential scanning calorimeter (DSC1/200/TC45, Mettler Toledo Company, Switzerland). Each millet starch (2 mg, dry weight) was accurately weighed into aluminum DSC pans, and distilled water was added by micropipette to achieve a water-sample ratio of 2:1 (v/w). The samples were hermetically sealed and allowed to stand for 24 h at room temperature before heating in the DSC. The DSC analyzer was calibrated using indium, and an empty aluminum pan was used as reference. Sample pans were heated at a rate of 10°C /min from 25 to 95°C. The carrier gas was nitrogen with flow velocity of 20 mL/min. The onset temperature (To), peak temperature (Tp), conclusion temperature (Tc), and gelatinization enthalpy (ΔHg) were calculated automatically. The gelatinization temperature range (Tr) was computed as Tc–To. Enthalpies were calculated on a starch dry basis. After gelatinization, the samples were stored at 4°C for 10 days for the following retrogradation test. After storage, the samples were then reheated in DSC under the same conditions. Retrogradation enthalpy (ΔHr) and the ratio of retrogradation (R%, ΔHr/ΔHg) were calculated from the second thermalgram.
Rheological Properties
A small amplitude oscillatory rheological measurement was conducted for millet starches using a dynamic rheometer (ARES G2 Rheometer, TA Instruments Ltd., America) equipped with a parallel plate system (25 mm dia.). The gap size was set at 1 mm .The strain and frequency were 2% and 5 rad/s, respectively. Starch suspensions with 6% (w/v) concentration were loaded on the plate of rheometer and covered with a thin layer of low-density silicone oil (to minimize evaporation losses). The starch samples were heated from 28 to 100°C at a rate of 5°C/min, and then cooled from 100 to 28°C at a rate of 5°C/min. The dynamic rheological parameters of the storage modulus (G′) and loss modulus (G″) of millet starches were recorded as a function of temperature. Rheological viscograms were drafted using an Excel software package (Microsoft Corporation).
Pasting Properties
The pasting properties of starches were determined using a Rapid Visco Analyzer (RVA, Super-4, Newport Scientific, Warriewood, Australia). Starch (3 g, 14% moisture basis) was mixed with 25 g of deionised water, different concentrations (0, 1, 2, 3, 4, and 5% w/v) of sodium chloride (NaCl) solution, different concentrations (0, 3, 7, 11, and 15% w/v) of sucrose solution, and different pH value (pH 2, 3, 5, 7, 9, and 11) solutions adjusted with 0.1 N NaOH and HCl solutions in the RVA sample canister. The procedure used was Standard profile 1. The analysis was conducted for 13 min with the following conditions: An equilibration for 1 min at 50°C, a linear temperature increase to 95°C in 3 min 42 s, holding at 95°C for 2 min 30 s, a cooling step (3 min 48 s) with a linear temperature decrease to 50°C, and a final isothermal step at 50°C (2 min). An RVA plot of viscosity (cP) versus time (s) was used to determine the peak viscosity (PV), holding viscosity (hot paste viscosity, HPV), cool paste viscosity (CPV), breakdown (BD = PV–HPV), and setback (SB = CPV–HPV). RVA viscograms were drafted using Origin Pro8.0 software.
Statistical Analysis
All analyses were conducted in triplicate. The data analysis was conducted using SPSS version 18.0 software. The differences were reported as significant by analysis of variance (ANOVA) at a confidence level of 95%.
RESULTS AND DISCUSSION
Chemical Composition and Purity
The moisture content of starches ranged from 7.64 to 11.48 g/100 g (db), whereas their ash content varied from 0.39 to 1.60 g/100 g (db). The protein residual was from 0.31 to 0.58 g/100 g (db). Low residual protein contents in the isolated starches indicated the successful isolation of starches from millets. shows that amylose content of every millet starch was, respectively, the lowest for one waxy variety PSMS with 0.38 g/100 g (db), medium for BYMS with 17.60 g/100 g (db), higher for FTMS with 25.68 g/100 g (db), and highest for HBMS, FGMS, and PLMS with about 28.00 g/100 g (db). Amylose content is responsible for a number of factors that affect the SP, solubility, and gel forming property of the starch. These samples provided a good platform for studying the relationships between the amylose content and physicochemical properties of starch. The purity of starches from six millet cultivars ranged from 80.61 to 92.27 g/100 g (db). The waxy PSMS had the lowest purity because of its combination with higher lipid content.
Table 1 Chemical composition and purity of starches isolated from six varieties of millet (dry weight basis, db).
Granular Morphology and Crystalline Structure
The scanning electron micrographs of native millet starch granules at two magnifications (viz.5000 × and 10000 ×) are presented in . These micrographs showed majority of large polygonal and few small spherical granules. In addition, numerous large polygonal granules of millet starch showed deep indentations, which may be attributed to the pressure exerted by protein bodies or the dense packing of the endosperm.[Citation10] Several pores exist on the surface of a few granules, as shown in . Granules of sorghum, millet, and corn starches have openings (pores) on the surface of the granules.[Citation17] These pores are normal structures related to the genetic make-up of the source plant and are not artifacts of the isolation, preparation, or observation techniques.[Citation14] By contrast, potato, arrow-root, and canna starch granules have smooth, non-porous surfaces. The granule sizes of the PSMS, HBMS, BYMS, and FGMS were similar ranging from 3 to 10 μm in diameter. Meanwhile, the granule sizes of FTMS and PLMS were a little larger than the other four varieties, ranging from 3 to 15 μm in diameter. Fujita reported that the average granule size of 53 foxtail millet varieties ranged from 6.8 μm to 11.8 μm in diameter.[Citation11] The granule size of FGMS was consistent with the results of FGMS reported by Adebowale.[Citation18] However, the result of PLMS was smaller than the starch granules (10 to 16 μm) reported by Wankhede et al.[Citation19] The variation in size and shape of starch granules may be caused by their biological origin.
Figure 1 The scanning electron micrographs of starches isolated from six varieties of millet at different magnifications: PSMS: proso millet starch; HBMS: hybrid barnyard millet starch; BYMS: barnyard millet starch; FGMS: finger millet starch; FTMS: foxtail millet starch; PLMS: pearl millet starch.
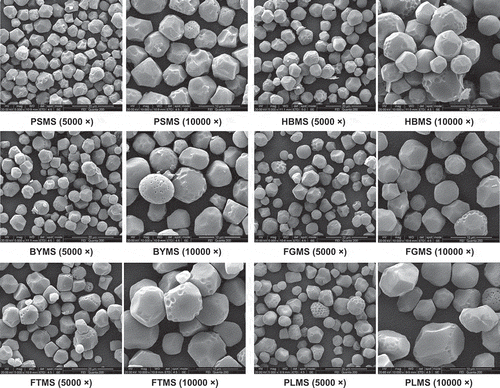
According to the the x-ray pattern (in ), all six millet starches possess a typical A-type crystallinity, with strong reflection at 2θ about 15, 17, 18, and 23°, and weaker peak at 2θ around 20°. The calculated degrees of crystallinity of the six starches from different millets are also shown in . Among the six starches, PSMS had the lowest amylose content (0.38 g/100 g, db), but with highest crystalline degree (30.89%). The crystalline degree of other starches ranged from 23.69 to 25.91%. The differences in relative crystallinity among starches can be attributed to the following: (1) crystal size, (2) amount of crystalline regions (influenced by amylopectin content and amylopectin chain length), (3) orientation of the double helices within the crystalline domains, and (4) extent of interaction between double helices.[Citation20]
Several Basic Physical Properties
The basic physical properties in terms of WSI, SP, paste clarity, sediment volume, and freeze-thaw stability of starches separated from different millet cultivars are presented in . The WSI for PLMS was the lowest, as shown in . FGMS with the highest amylose content had the highest solubility. Meanwhile, the other millets had similar solubility. Solubility corresponds to hydrophilicity and amylose content.[Citation21] Starch molecules can hardly be soluble in free water but imbibed water as the temperature increases above the critical value. The amylose and linear branches of amylopectin dissociate in suspension and increase the solubility of starch.[Citation22]
In , six millet starches with different amylose content distribution were observed to have almost similar SP at approximately 23 to 27%. However, the previous report showed that waxy starches usually swell to a greater extent than their normal-amylose counterparts.[Citation23] SP can be influenced by strongly amylopectin molecular structure.[Citation24] Hydrogen bonds that stabilize the structure of the double helices in crystallites were broken during gelatinization and replaced by the hydrogen bonds with water and swelling was regulated by the crystalline properties of the starch.[Citation25] According to Beleia et al.,[Citation26] starches containing a higher proportion of amorphous material would presumably imbibe more water.
PSMS presented excellent paste clarity of about half of the transmittance of distilled water, as shown in . Waxy starches are notable for paste clarity. Such value is much higher than all other millet starches. This result may be due to the presence of less granule remnants in the starch paste.[Citation27] The other five millet starches exhibited similar paste clarity. Starch paste clarity is among the important attributes of starch, which is essential in foods, such as jellies and fruit pastes, to obtain the desired consistency. Paste clarity varies considerably with the starch source, amylose/amylopectin ratio, chemical or enzymatic modifications, and addition of solutes.[Citation28] Therefore, PSMS with significantly high transmittance can be suitable for application in the jelly, drink, and fruit paste industries.
Among the six millet starches, the sediment volume of PSMS was the highest. Similarly, other millet starches presented relatively high sedimentation values, except for BYMS (). The higher the sediment volume of the aqueous starch dispersion, the stronger ability of the starch paste to form gel. shows the freeze-thaw stability of millet starches in terms of syneresis. PSMS had separated water of 17%, which is much lower than the other five millet starches. Other millet starches showed more than 45% separated water, which is an adverse property in the frozen food industry. This was because that the separated water was lower, the freeze-thaw stability of starch was higher, and the tendency to retrograde was smaller. Syneresis increased with the number of freeze-thaw cycles. Abdel-Aal et al.[Citation29] also illustrated that waxy starch gels exhibit greater refrigeration and freeze-thaw stability than the non-waxy wheat starch gels, as indicated by significantly lower values of net syneresis. Based on the above results, PSMS showed prominent characteristics, including the significant paste clarity and freeze-thaw stability, whereas FGMS had the highest solubility, which may have applications in relative starch fields other than the food industry.
Thermal Properties
The gelatinization characteristics of starches separated from the six millets are presented in , in which the waxy PSMS yielded the highest Tp of 74.53°C, Tr of 13.87°C, and ΔHg of 5.16 J/g. This result may be attributed to the highest amylopectin content and degree of crystallinity of PSMS among the six millet starches. There are believed to result from high degree of crystallinity, which imparts structural stability, making the granules more resistant to gelatinization.[Citation30] The gelatinization of starch leads to the process of the melting of the double helices and loss of crystallinity.[Citation31] It also indicated that waxy starch has higher gelatinization temperatures and enthalpy than their normal amylose starch.[Citation32] The gelatinization enthalpy varied from 2.65 to 3.98 J/g for the other five millet starches. It has similar gelatinization temperature and significant different gelatinization enthalpy of PSMS and FTMS, respectively, compared to previous reported data of some varieties, and the differences in gelatinization enthalpy may be attributed to the differences in amylose content.[Citation10,Citation12]
Table 2 Thermal properties of starches isolated from six varieties of millet.
The retrogradation characteristics of gelatinized millet starches after storage at 4°C for 10 days are shown in . The enthalpy value of the retrograded starch reflects the melting of the crystallites formed through the association between the adjacent double helices during gel storage.[Citation33] This endothermic peak was caused by the melting of retrograded amylopectin instead of amylose.[Citation34,Citation35] However, in a dispersed starch system, the proportion of amylose and the structure of amylopectin play significant roles in the rate and degree of amylopectin retrogradation.[Citation36] And the leading role of the two factors is different under different conditions. The retrogradation enthalpy (ΔHr) of gelatinized millet starches ranged between 0.93 J/g and 1.74 J/g. R% (the ratio of ΔHr/ΔHg) is the retrogradation rate, which was the highest for FGMS (46.08%) with the highest amylose content, but lowest for BYMS (28.35%) with the lowest amylose content, except for waxy PSMS. By correlation analysis using SPASS software, it found that R% of non-waxy millet starch was positively correlated with their amylose content (Pearson’s correlation coefficient r = 0.974, P < 0.01, by t-test), which means the non-waxy millet starch with higher amylose content may retrograded quicker. Meanwhile, waxy PSMS had almost no amylose, but had relatively high ΔHr (1.74 J/g) and R% (33.72%). The results were agreed with the previous investigation, which showed that the normal-amylose and the waxy barley starches indeed retrograded to the same level, but that the high-amylose barley starch retrograded to a higher extent.[Citation37]
Rheological Properties
Temperature sweep of native millet starch suspensions
The variations of the dynamic rheological parameters of millet starches during heating are presented in and , which differ significantly. In particular, for PSMS at 6% concentration, G′ and G″ remained nearly zero at the whole stages of heating, indicating that the paste in this concentration had become a very weak gel. For the other five millet starches, the G′ and G″ curves rose to maximum, dropped gradually, and had similar tendency of change. Meantime, in the whole heating phase, G′ of the five millet starches was always higher than G″. It was accordance with the results of barnyard, finger, proso, and foxtail millets flours in a previous study, but this result was not implied “a tendency to behave like weak gel” as the researcher considered.[Citation38] It indicated that the elasticity of the gel is larger than adhesiveness, showing that the paste has a tendency to behave as a solid-like gel. This phenomenon is similar to that obtained for corn and potato starch.[Citation30] According to the former report,[Citation39] this trend is caused by the elastic nature of the material that exceeds the viscous nature. In the dynamic measurement with rheometer, the changes of G′ can reflect the changes in hardness and strength of the gel, in which higher G′ indicates higher hardness and strength.[Citation40]
Temperature sweep of native millet starch pastes
During cooling, the starch pastes showed continuous rise in G′ among all starch and G″ of PSMS and BYMS ( and ). However, G″ of other millet starch pastes increased first, and then immediately decreased. In addition, waxy PSMS and BYMS with lower amylose content (17.60 g/100 g, db) both showed significantly lower G′ and G″ compared with the other starches. This result may be attributed to the lower tendency on retrogradation of amylose of the two-mentioned starch gels during cooling compared with the other four starch pastes. Amylose affected the rate of short-term retrogradation and viscoelastic characteristics of starches, in which the starches with high amylose exhibited greater G′ of the cooked starch pastes.[Citation41]
Pasting Properties of Native Millet Starches
shows the pasting characteristics of millet starches. The pasting characteristics of various starches are affected by amylose and amylopectin content and by their arrangement structure in the granules.[Citation42] The PV of millet starches varied from 2372 to 4522 cP. Waxy PSMS had the lowest PV, whereas FGMS had the highest value. Lower PV indicates lower water binding capacity of the starch.[Citation21] The difference in BD was related to the variations in rigidity or fragility of the swollen granules, and was an indication of the degree of organization of the molecules.[Citation5] The PV and BD values of millet starches had positive correlation with amylose content of millet starches, respectively (Pearson’s correlation coefficient r = 0.815 and 0.847, P < 0.05, by t-test). The final viscosity of the millet starches were lowest for PSMS (1792 cP) and highest for BYMS. The difference value between the final and holding viscosities is defined as SB. The secondary increase in viscosity (SB) during the cooling phase, associated with the amylose retrogradation phenomenon and related to amylose content, was minimum for waxy PSMS (582 cP) compared with the other millet starches. Eliminating unreasonable final viscosity and SB values of BYMS, the final viscosity and SB values of other millet starches also had positive correlation with amylose content of millet starches, respectively (Pearson’s correlation coefficient r = 0.890 and 0.958, P < 0.05, by t-test).
Effect of NaCl on Pasting Properties of Native Millet Starches
The addition of NaCl can influence the pasting properties of millet starch, as shown in . For PSMS, the changes of various viscosities were insignificant at different NaCl concentration of the solutions compared with the other millet starch, indicating that the pasting properties of waxy PSMS paste remained less effect by this range concentration of NaCl. On the other hand, peak, holding, and final viscosities of PSMS was higher, whereas its BD and SB values lowered as NaCl concentration increased, except at 1% NaCl concentration. On the contrary, adding salt in the solution significantly affected the pasting properties of other millet starches. Specifically, NaCl decreased the peak viscosities of HBMS, FGMS, FTMS, and PLMS. The holding viscosities of these millet starches increased, whereas their BD values decreased, with the increase of the NaCl concentration. However, the counterpart peak viscosities of these starches showed an increasing tendency, whereas their SB values showed a decreasing tendency by adding NaCl concentration from 1 to 5%. The final viscosity of BYMS sharply reduced to almost zero under the influence of salt.
Effect of Sucrose on Pasting Properties of Native Millet Starches
The addition of sucrose can significantly and regularly influence the pasting properties of millet starches according to . The total tendency of RVA curves consistently moved up with the increase of sucrose concentration. All viscosities, including the BD and SB values, increased with the increase in sucrose concentration. This result may be attributed to the sucrose combined with the starch by hydrogen bond, which was connected between starch and water. Moreover, the sucrose-starch combination increased the pasting viscosity.[Citation43]
Effect of pH on Pasting Properties of Native Millet Starches
No significant change was identified for the pasting viscosity of various starches when the pH value was increased from 5 to 9, as shown in . In particular, the pasting properties of PSMS and FTMS remained relatively stable at pH 3 to 11. However, when the pH value decreased to 2, the viscosity of all starches rapidly changed significantly than at pH 11 alkaline conditions, and then decreased quickly, except for BYMS and PLMS. Such results may be attributed to the more easily hydrolyzed starches in such acidic environment. By contrast, the PV of BYMS and PLMS increased obviously at pH 11, which reveals the higher water binding capacity of the starch.
CONCLUSIONS
The isolation, structural, and physical properties of different millet starches were analyzed. Starches isolated from six kinds of millets showed significant difference in composition and physical properties. The waxy starch obtained from proso millet exhibited more outstanding physical properties, especially paste clarity, freeze-thaw stability, rheological properties, and relative viscosity stability at different NaCl concentrations and pH values. However, these starches showed similar granular morphology and crystalline structure. The information obtained in this study would be significant in the applications of these millets in starch industry fields other than food. Essentially, the potentials of a relatively new, cheap and underutilized source of starches are underscored.
FUNDING
This study was supported by a grant from the National 12th Five-year Key Technology R&D Program (Grant No. 2012BAD34B0202), the Ministry of Science and Technology of the People’s Republic of China, and National Natural Science Foundation of P.R. China (Grant No. 31250005).
REFERENCES
- Stewart, G.F.; Amerine, M.A. Evolution of food processing and preservation. In: Introduction to Food Science and Technology; Academic Press: New York, 1982; 6.
- Dharmaraj, U.; Ravi, R.; Malleshi, N.G. Physicochemical and textural characteristics of expanded finger millet. International Journal of Food Properties 2012, 15, 336–349.
- Badau, M.H.; Nkama, I.; Ajalla, C.O. Physico-chemical characteristics of pearl millet cultivars grown in Northern Nigeria. International Journal of Food Properties 2002, 5, 37–47.
- Baryeh, E.A. Physical properties of millet. Journal of Food Engineering 2002, 51, 39–46.
- Kumari, S.K.; Thayumanavan, B. Characterization of starches of proso, foxtail, barnyard, kodo, and little millets. Plant Foods for Human Nutrition 1998, 53, 47–56.
- Asharania, V.T.; Jayadeepa, A.; Malleshia, N.G. Natural antioxidants in edible flours of selected small millets. International Journal of Food Properties 2010, 13, 41–50.
- Kim, J.Y.; Jang, K.C.; Park, B.R.; Han, S.I.; Choi, K.J.; Kim, S.Y.; Oh, S.H.; Ra, J.E.; Ha, T.J.; Lee, J.H.; Hwang, J.; Kang, H.W; Seo, W.D. Physicochemical and antioxidative properties of selected barnyard millet (Echinochloa utilis) species in Korea. Food Science and Biotechnology 2011, 20, 461–469.
- Subramanian, S; Viswanathan, R. Thermal properties of minor millet grains and flours. Biosystems Engineering 2003, 84, 289–296.
- Taylor, J.R.N.; Schober, T.J.; Bean, S.R. Novel food and non-food uses for sorghum and millets. Journal of Cereal Science 2006, 44, 252–271.
- Yañez, G.A.; Walker, C.E.; Nelson, L.A. Some chemical and physical properties of proso millet (Panicum milliaceum) starch. Journal of Cereal Science 1991, 13, 299–305.
- Fujita, S.; Sugimoto, Y.; Yamashita, Y.; Fuwac, H. Physicochemical studies of starch from foxtail millet (Setaria italica Beauv.). Food Chemistry 1996, 55, 209–213.
- Kim, S.K.; Sohn, E.Y.; Lee, I.J. Starch properties of native foxtail millet, Setaria italica Beauv. Journal of Crop Science and Biotechnology 2009, 12, 59–62.
- Ojijo, N.K.O.; Shimoni, E. Influence of xanthan gum and tapioca starch on the retrogradation and gelation of finger millet (Eleusine coracana L. Gaertner) starch pastes. Journal of Texture Studies 2007, 38, 100–115.
- Hoover, R.; Swamidas, G.; Kok, L.S.; Vasanthan T. Composition and physicochemical properties of starch from pearl millet grains. Food Chemistry 1996, 56, 355–367.
- Malleshi, N.; Desikachar, H.; Tharanathan, R. Physico-chemical properties of native and malted finger millet, pearl millet, and foxtail millet starches. Starch/Stärke 1986, 38, 202–205.
- AOAC. In: Official Methods of Analysis of AOAC International; 17th ed. AOAC International: Maryland-Gaithersburg, USA, 2000.
- Fannon, J.E.; Hauber, R.J.; BeMiller, J.N. Surface pores of starch granules. Cereal Chemistry 1992, 69, 284–288.
- Adebowale, K.O.; Afolabi, T.A.; Olu-Owolabi, B.I. Hydrothermal treatments of finger millet (Eleusine coracana) starch. Food Hydrocolloids 2005, 19, 974–983.
- Wankhede, D.B.; Rathi, S.S.; Gunjal, B.B.; Patil, H.B.; Walde, S.G.; Rodge, A.B.; Sawate, A.R. Studies on isolation and characterization of starch from pearl millet (Pennisetum americanum (L.) Leeke) grains. Carbohydrate Polymers 1990, 13, 17–28.
- Hoover, R.; Ratnayake, W.S. Starch characteristics of black bean, chick bean, lentil, navy bean, and pinto bean cultivars grown in Canada. Food Chemistry 2002, 78, 489–498.
- Lawal, O.S. Starch hydroxyalkylation: Physicochemical properties and enzymatic digestibility of native and hydroxypropylated finger millet (Eleusine coracana) starch. Food Hydrocolloids 2009, 23, 415–425.
- Balasubramanian, S.; Sharma, R.; Kaur, J.; Bhardwaj, N. Characterization of modified pearl millet (Pennisetum typhoides) starch. Journal of Food Science and Technology–Mysore 2011, 17, 12–17.
- Tester, R.F.; Morrison, W.R. Swelling and gelatinization of cereal starches. 1. Effects of amylopectin, amylose, and lipids. Cereal Chemistry 1990, 67, 551–557
- Tester, R.F.; Morrison, W.R.; Schulman, A.H. Swelling and gelatinization of cereal starches. V. Risomutants of Bomi and Carlsberg II Barley cultivars. Journal of Cereal Science 1993, 17, 1–9.
- Tester, R.F.; Karkalas, J. Swelling and gelatinization of oat starches. Cereal Chemistry 1996, 73, 271–273.
- Beleia, A.; Varriano-marston, E.; Hoseney, R.C. Characterization of starch from pearl millets. Cereal Chemistry 1980, 57, 300–303.
- Lovedeep, K.; Jaspreet, S.; Narpinder, S. Effect of glycerol monostearate on the physico-chemical, thermal, rheological, and noodle making properties of corn and potato starches. Food Hydrocolloids 2005, 19, 839–849.
- Achille, T.F.; Georges, A.; Alphonse, K. Contribution to light transmittance modeling in starch media. African Journal of Biotechnology 2007, 6, 569–575.
- Abdel-Aal, E.S.M.; Hucl, P.; Chibbar, R.N.; Han, H.L.; Demeke, T. Physicochemical and structural characteristics of flours and starches from waxy and non-waxy wheats. Cereal Chemistry 2002, 79, 458–464.
- Barichello, V.; Yada, R.Y.; Coffin, R.H.; Stanley, D.W. Low temperature sweetening in susceptible and resistant potatoes: Starch structure and composition. Journal of Food Science 1990, 55, 1054–1057.
- Cooke, D.; Gidley, M.J. Loss of crystalline and molecular order during starch gelatinization: Origin of the enthalpic transition. Carbohydrate Research 1992, 227, 103–112.
- Sasaki, T.; Yasui, T.; Matsuki, J. Effect of amylose content on gelatinization, retrogradation, and pasting properties of starches from waxy and non-waxy wheat and their F1 Seeds. Cereal Chemistry 2000, 77, 58–63.
- Hoover, R.; Senanayake, S.P.J.N. Composition and physicochemical properties of oat starches. Food Research International 1996, 29, 15–26.
- Fearn, T.; Russell, P.L. A kinetic study of bread staling by differential scanning calorimetry. The effect of loaf specific volume. Journal of the Science of Food and Agriculture 1982, 33, 537–548.
- Karim, A.A.; Norziah, M.H.; Seow, C.C. Methods for the study of starch retrogradation. Food Chemistry 2000, 71, 9–36.
- Matalanis, A.M.; Campanella, O.H.; Hamaker, B.R. Storage retrogradation behavior of sorghum, maize, and rice starch pastes related to amylopectin fine structure. Journal of Cereal Science 2009, 50, 74–81.
- Fredriksson, H.; Silverio, J.; Anderssona, R.; Eliassonb, A.C.; Åmana, P. The influence of amylose and amylopectin characteristics on gelatinization and retrogradation properties of different starches. Carbohydrate Polymers 1998, 35, 119–134.
- Shinoj, S.; Viswanathan, R.; Sajeev, M.S.; Moorthy, S.N. Gelatinisation and rheological characteristics of minor millet flours. Biosystems Engineering 2006, 95, 51–59.
- Garcia, A.M.; Walter, W.M. Physicochemical characterization of starch from Peruvian sweetpotato selectionsASA Alimentos S.A., Lima, Peru. Starch/Stärke 1998, 50, 331–337.
- Rao, M.A.; Okechukwu, P.E.; da Silva, P.M.S.; Oliveira, J.C. Rheological behavior of heated starch dispersions in excess water: Role of starch granule. Carbohydrate Polymers 1997, 33, 273–283.
- Singh, N.; Singh, S.; Shevkani, K. Maize: Composition, bioactive constituents, and unleavened bread. In: Flour and Breads and Their Fortification in Health and Disease Prevention; Academic Press: Elsevier, London, 2011; 89–99.
- BeMiller, J.; Whistler, R. Starch Chemistry and Technology, Academic Press: Elsevier, London, 2009; 195–196.
- Eliasson, A.C. Starch in food: Structure, function, and applications, Woodhead Publishing Ltd.: London, 2004; 140–142