Abstract
The texture profile analysis and functional properties of gelatin from fresh water fish (carps) and porcine skin were measured. Texture profile analysis revealed that the porcine gelatin showed significantly higher values for hardness, springiness, cohesiveness, gumminess, and chewiness than carp skin gelatin (p < 0.05). The bloom strength of gelatin from a porcine source was higher (466.40 g) than carp skin. The solubility profile of gelatin from carps and porcine as function of sodium chloride concentration indicated a maximum solubility at 0.3 M. The relationship between emulsion capacity values and concentration of proteins were found to be inversed in all of the gelatin samples.
INTRODUCTION
Gelatin is a denatured protein derived from collagen by thermo-hydrolysis and has a rheological property of thermo-reversible transformation between sol and gel.[Citation1] The unique characteristic of gelatin gel is its ‘melt-in-mouth’ texture that provides excellent mouth feel.[Citation2] Gelatin gels are quite soft and flexible, but their textural properties are very narrow and it is widely used in food applications, especially in the formulation of water-based dessert gels.[Citation3] Gelatin desserts made from various gelatins may differ in textural and gel melting properties, offering new product development opportunities.[Citation4] Gels have been used as model systems to study food texture as they can provide good descriptions of mechanical behavior of numerous food systems and, hence, provide valuable information about food texture.[Citation5]
Texture is defined as the sensory and functional manifestation of the structural, mechanical, and surface properties of foods that can be perceived by senses of sight (visual texture), sound (auditory texture), touch (tactile texture), and kinesthetics.[Citation6] The texture profile analysis (TPA) was first carried out using the General Food Corporation texturometer.[Citation7] The TPA includes application of controlled force to the product and recording its response with time. TPA is a technique commonly used in industry for the evaluation of food textural behavior, as it can give an indication of sensory properties.[Citation8] Instrumental TPA has been widely used to evaluate the texture of various protein gels, including gelatin and gel deserts,[Citation4] and gellan-gelatin.[Citation9]
TPA is useful for gel texture analysis because of the textural parameters obtained from the TPA curves have been well correlated with sensory evaluation of textural parameters.[Citation9,Citation10] The popularity of TPA for different foods is attributed to near simulated condition of oral mastication. The application of force to food during oral mastication is well obtained in TPA by force-time curves. The different parameters obtained during TPA include hardness, cohesiveness, springiness, and chewiness, and quality of gelatin can be assessed by using these parameters.[Citation11]
The raw material for gelatin production is mainly from the skins and bones of bovine and porcine sources. Gelatin obtained from bovine and porcine sources may not be preferred by a certain section of the population because of religious consideration.[Citation12] The processing of fish and fishery products will generate a large quantity of processing waste. A conservative estimate is that nearly 7.5 million tonnes of waste will be generated the world over from processing of fish.[Citation13] One of the effective ways of utilizing the fish processing waste is to utilize for a high value product like gelatin. However, the quality of gelatin will depend on the source and degree of freshness of raw material used for the process. It is important that the quality of gelatin to be assessed is produced from a different source of raw material. The Asian carps contribute 15% of total world fish production.[Citation13] The production of carps in South East Asia is increasing at 6–8% per annum. The likelihood of further increase in production of carps is foreseen with adoption of better culture practices. This necessitates better post harvest management by different processing techniques. Generally, any processing of fish will generate a substantial quantity of waste, such as skins, bones, and entrails. The processing waste generated needs to be discarded effectively so as to minimize the environmental pollution. Utilization of fish processing waste for gelatin production is one of the viable options to minimize the environmental pollution. However, the properties of gelatin need to be assessed whether it meets the prescribed standard or not. The textural properties of gelatin obtained from different sources of raw material will help the industry to decide on the utilization pattern.
With this rationale, the objectives of the present investigation were to prepare the gelatin from the skins of three species of Indian major carp and assess its textural and functional properties. The textural and functional properties of commercial porcine skin gelatin were evaluated and compared to the properties of gelatin from carp’s skin.
MATERIALS AND METHODS
Raw Material
Indian major carps, namely, Catla catla, Labeo rohita, and Cirrhinus mrigala, were procured from a private farm at Shimoga, Karnataka State, India and brought to the laboratory in iced condition. The fish were washed twice with chilled water and beheaded, gutted, and filleted. The skins from the fillets were removed manually and cleaned with tap water till the adhering meat was completely removed. The cleaned skins were stored at −20°C until further use. The frozen skin was used within 2 months of storage. The porcine skin type-A gelatin, was procured from Sigma-Aldrich Co. (St. Louis, MO, USA).
Preparation of Gelatin
The gelatin from the skins of fresh water carps viz., catla, mrigal, and rohu were produced according to the method described by Gudmundsson and Hafsteinsson.[Citation14] with a slight modification.
Proximate Composition
The moisture and ash content of the extracted gelatin were determined by the method of the AOAC.[Citation15] The crude protein content was determined by estimating the total nitrogen content by the method of the AOAC.[Citation15] The protein content of gelatin was obtained by multiplying the nitrogen value by a factor of 5.8.
Nitrogen Solubility Index (NSI)
The NSI of the gelatin samples was determined by the method of Binsi et al.[Citation16] The NSI was obtained by plotting pH values versus the percentage of the total nitrogen in the sample solubilized at each pH value measured.
Solubility of Proteins as a Function of Sodium Chloride Concentration
Gelatin samples were separately dissolved in phosphate buffer (pH 7.5), 50 mM containing different concentrations of sodium chloride (0–2.0 M). The ratio of buffer to gelatin was 1:100 (gelatin:buffer) homogenized at 9000 rpm for 3 min using an Ultra Turrax homogenizer (Ultra-Turrax, T 25, Janke & Kunkel GMBH & Co., KG Staufen, Germany). The homogenate was centrifuged at 9000× g for 15 min at 4°C using refrigerated centrifuge (Sorvall Legenda XTR, Thermo Fisher Scientific Inc, Osterode, Germany). The total nitrogen content in the clear supernatant was determined by the method as described by the AOAC.[Citation15] The nitrogen value obtained was multiplied by a factor of 5.8 to obtain the protein content and was expressed as the percentage of total gelatin.
Amino Acid Composition
The amino acid composition of the gelatin samples was determined by the method of Ishida et al.[Citation17] using HPLC (LC 10 AS, Shimadzu, Japan) fitted with a packed column (ISC-07/S1504-Na). A gradient buffer system of pH 3.2 to 12 was used and the individual amino acids were detected using a fluorescence detector. Tryptophan content of the gelatin sample was determined by the method of Sastry and Tummuru.[Citation18] The amino acid content was expressed as g amino acid per 100 g protein.
Bloom Strength (Bloom Value)
Bloom strength was determined by the method of GME.[Citation19] Gelatin (7.5 g) was weighed and transferred into a bloom jar. A known quantity of deionized water (105 mL) was added. The solution (6.67%) was stirred with a glass rod, covered, and allowed to stand at room temperature for 3 h. The solution was heated in a water bath at 65°C for 30 min with occasional stirring with a magnetic stirrer to dissolve the gelatin completely. The jar was covered and allowed to cool for 15 min at room temperature (21°C). The bloom jars were kept in a refrigerator at 4°C overnight (17 h), and immediately tested using a Brookfield texture analyzer (LFRA 1500 Brookfield Engineering Laboratories, Inc., Middleboro, MA, USA). The bloom jar was placed centrally under the standard probe and the penetration test was commenced. After attaining a 4 g trigger force, the probe proceeded to penetrate into the gel to a depth of 4 mm. At this depth, the maximum force reading (the resistance to penetration) was obtained and translated as the bloom value (g) of the gel.
Gel Preparation for TPA
Gelatin gels were prepared by dissolving the dry powder in distilled water. The concentration of gelatin used was 6.67% (w/v). The solution was heated on a water bath in the temperature range of 50–60°C for 30 min with occasional stirring using a magnetic stirrer to ensure complete dissolution of gelatin. The mixture was allowed to cool for 10 min and was kept in a refrigerator (4–6°C) for 24 h for gel formation. The gels were removed from the beaker using a spatula and were used for texture analysis immediately.
Instrumental Texture Profile Analysis (Two-Cycle Compression Test)
The size of the gel used for TPA was 3.0 cm × 4.0 cm (diameter × height). TPA was carried out using a TA-TX2 texture analyzer (Stable Micro Systems Ltd., Surrey, UK), attached with a 50-kg load cell. A 75-mm diameter compression platen was used to compress a cylindrical shape of the gels, which were compressed twice to 40% of the original height of the gel at a compression rate of 1.0 mm/s at room temperature. The TPA settings were as follows: pre-test speed: 1.5 mm/s; test speed: 1.0 mm/s; post-test speed: 1.5 mm/s; target mode distance: 10 mm; trigger force: 5 g; trigger type: Auto; data acquisition rate: 200 points per second (pps). The delay between the first and second compression was 5 s. The TPA analysis was carried out at ambient temperature (25°C) and the analysis was completed within 100 s. Three measurements were made for each sample in the same lot and average value was reported for each parameter. A force-time graph was generated and textural parameters, such as hardness (HA), cohesiveness (CO), adhesiveness (AD), springiness (SP), brittleness or fracturability (FA), resilience (RE), gumminess (GU), and chewiness (CH), were calculated with the help of software provided along with the instrument.[Citation20]
Emulsion Capacity (EC)
The emulsion capacity of the gelatin solution at different concentrations (0.05, 0.1, and 0.25% w/v) was determined by the method of Swift et al.[Citation21] Dry gelatin was dissolved in phosphate buffer (50 mM) containing 0.3 M sodium chloride, pH 7.5. About 25 ml of the gelatin solution at different concentrations were mixed thoroughly with 50 ml of refined sunflower oil (ITC, Mumbai, India) at 9000 rpm for 10 s using an Ultra Turrax homogenizer (Ultra-Turrax, T 25, Janke & Kunkel GMBH & Co. KG, Staufen, Germany).
Homogenization was continued at a high speed (23,000 rpm) with the addition of oil at a rate of 0.5–0.6 mL/s until phase inversion was observed visually. Protein content of the gelatin solutions was determined by the method of AOAC.[Citation15] The EC was expressed as ml oil/mg protein and the average of three replicates was taken as the emulsion capacity of the sample.
Foaming Capacity and Stability
The foam capacity and stability of gelatin solution at different concentrations (4, 10, and 20 mg/mL) were determined by the method of Sathe et al.[Citation22] The foam capacity and foam stability were calculated using the following equations:
Statistical Analysis
The data obtained was analyzed by one way analysis of variance. The textural attributes, bloom strength, and emulsion capacity values from different gelatin samples was used for one way analysis of variance. In order to assess the test of significance at a 5% level between source of gelatin and quality parameters of gelatin, Duncan’s Multiple Range test was used. The correlation factor (r) was assessed between bloom strength versus hardness, bloom strength versus cohesiveness, and bloom strength versus chewiness. The statistical analysis was carried out by using SPSS 16.0 for Windows (SPSS, Inc., Chicago, IL, USA).
RESULTS AND DISCUSSION
Proximate Composition
The protein content of the dried gelatin obtained from the skin of catla, mrigal, and rohu was in the range of 92 to 94% (). The moisture content of gelatin was less than 6%, which was within the limit prescribed for edible gelatin.[Citation23] The ash content of fish gelatin was in the range of 1.37–1.87% (). The ash content of gelatin depends on the source of raw material used and generally it will be higher if bone is used as raw material.[Citation24]
TABLE 1 Proximate composition of gelatin from the skin of porcine and carp’s skin
Nitrogen Solubility Index (NSI)
The NSI of gelatin samples is given in . The pH at which minimum solubility occurred may be considered as the iso-electric point (pI). The lower pI of 6.17 was recorded from gelatin from the skin of mrigal. The pI of four gelatin samples varied from 6.17–6.42. At pI, the net charge on the protein molecule will be zero and favors protein precipitation from the solvent.[Citation16] The maximum solubility occurred in the acidic pH range than on the alkali side in all the gelatin samples. Gelatin is an amphiphatic molecule with isoelectric point in the range of 5–9 depending on raw material and method of extraction.[Citation3]
Solubility of Protein as a Function of Sodium Chloride Concentration
The solubility profile of gelatin from carp’s skin and porcine as a function of sodium chloride concentration indicated a maximum solubility at 0.3 M concentration (). Nearly 80% of total protein of gelatin samples was soluble in 0 M sodium chloride concentration with the exception of gelatin from rohu. With an increase in sodium chloride concentration, the solubility of gelatin increased up to 0.3 M, beyond which solubility decreased. Generally, solubility increases with an increase in salt concentration up to a certain level (salting ‘in’) and with further increase in salt concentration, the solubility decreases (salting ‘out’).[Citation25] The decrease in solubility with the increase of sodium chloride concentration is due to the increase of the hydrophobic interactions and the competition of ionic salts for water.[Citation16] At high sodium chloride concentration, salting out effect might occur as a result of salt binding with water and gelatin molecules undergo precipitation. The difference in amino acid composition and structure may impact solubility, especially at high sodium chloride concentration.[Citation26]
Amino Acid Composition
Amino acid composition of gelatin prepared from the skin of carps and porcine is given in . The main difference in the amino acid profile is the lower content of imino acid in gelatin from carp skin than porcine gelatin. Glycine is the most dominant amino acid residue in gelatin from different sources.[Citation27] The imino acids (proline + hydroxy proline) content of the gelatin samples in the present study was 28.5, 27.1, 26.44, and 32.05% for catla, rohu, mrigal, and porcine, respectively. Gelatin with high levels of imino acids tends to have high gel strength and melting point.[Citation28] Imino acids are important in the renaturation of gelatin subunits during gelling.[Citation3] In general, gelatin with high proline, hydroxyproline, and alanine contents shows better viscoelastic properties.[Citation29] The amino acid residues, such as arginine and cysteine, were not present in the gelatin samples.
TABLE 2 Amino acid composition of gelatin from skin of porcine and fresh water fishes
Bloom Strength (g)
The porcine gelatin had a higher bloom strength of 466.4 g, which was significantly higher (p < 0.05) than that of carp skin gelatin (). The bloom strength for gelatin from catla was higher (367.66 g) followed by mrigal and rohu gelatin. Gelatin is capable of forming and stabilizing hydrogen bonds with water molecules to form a stable three-dimensional gel. Bloom strength is an important criteria employed for grading of gelatin. Typically, gelatin from a fish source will have lower bloom strength than from a mammalian source.[Citation30] Several studies have indicated that the gel strength is related to imino acid content of gelatin samples.[Citation14] In the present investigation, the imino acid content of gelatin from carp’s skin was lower than that of porcine skin and is responsible for lower bloom strength.
TABLE 3 Bloom strength and texture profile of 6.67% (w/v) porcine and Indian major carp’s skin gelatin
Texture Profile Analysis (TPA)
The force-deformation curves of four gelatin samples are given . The TPA attributes of four gelatin gels carried out at a concentration of 6.67% (w/v) is given in . The porcine gelatin gel had a hardness value of 14.60 N followed by gels of catla, mrigal, and rohu with a value of 12.84, 8.41, and 7.05 N, respectively. Hardness of porcine gelatin gels was significantly higher compared to carp’s skin gelatin (p < 0.05). The hardness is related to the strength of gel structure under compression and is the peak force during the first compression cycle. The hardness value of gelatin gel from Nile perch skin was found to be 20.16 N.[Citation31] The high degree of correlation between bloom strength and hardness value has been obtained in the present study for all four gelatin samples (p < 0.05) (r = 0.934). The sensory meaning of hardness is the maximum force required to compress a food between the molar teeth.[Citation6]
FIGURE 3 Texture profile of gelatin gels from fresh water carp’s skin and porcine. (a) Porcine; (b) Catla catla; (c) Cirrhinus mrigala; (d) Labeo rohita. (Hardness: F1; Cohesiveness: A2/A1; Springiness: d2/d1; Adhesiveness: A3; Gumminess: Hardness × Cohesiveness; Chewiness: Gumminess × Cohesiveness.)
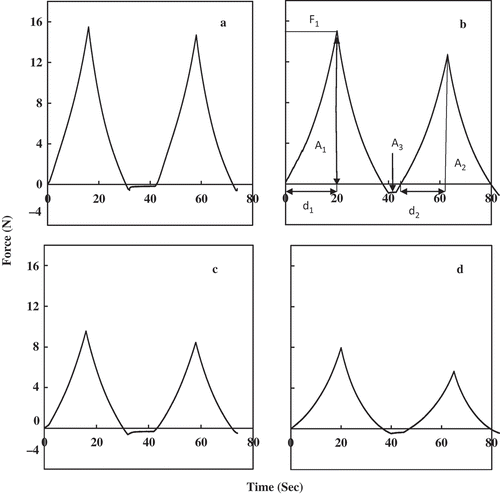
The adhesiveness parameter in TPA analysis for the four gelatin samples is represented in . Adhesiveness is defined as the negative force area for the first bite and represents the work required to overcome the attractive forces between the surface of a food and the surface of other materials with which the food comes into contact.[Citation2] From , it can be seen that the maximum adhesiveness value has been obtained for gelatin from rohu skin, whereas the minimum adhesiveness value (−198.72 g.s) has been obtained for gelatin from catla. The adhesiveness of all four types of gelatin samples showed no significant differences (p < 0.05). These results are in agreement with Rahman and Al-Mahrouqi.[Citation1] The higher value of adhesiveness implies soft texture and can be made use of for certain dessert preparations.
The cohesiveness (consistency) indicates the strength of internal bonds making up the body of food and the degree to which a food can be deformed before it ruptures (breaks).[Citation32] The cohesiveness values of the four gelatin samples were in the range of 0.64 to 0.9. The highest value obtained was for porcine gelatin and the lowest value obtained was for gelatin from rohu. Cohesiveness is defined as the ratio of the positive force area during the second compression to that of the first compression. It may be measured as the rate at which the material is disintegrated under mechanical action. Tensile strength is a manifestation of cohesiveness. The cohesiveness indicates the ability of the product to hold together. Among the four gelatin samples tested, the porcine gelatin had a higher cohesiveness value of 0.924. There is a good correlation between bloom strength versus hardness (r = 0.934) and bloom strength versus cohesiveness (r = 0.886) in the four gelatin samples studied.
The springiness values of the four gelatin samples are given in . Rohu fish skin gelatin gel showed the lowest springiness value compared with other samples. The springiness is a textural parameter, which is related to elasticity of the sample. Springiness in TPA is related to the height that the food recovers during the time that elapses during the end of first bite and the start of the second bite. If springiness is high, it requires more mastication energy in the mouth.[Citation1] A higher springiness value of 0.943 was obtained from gelatin from porcine. There was no significant difference in the springiness value of gelatin from porcine catla and mrigal (p > 0.05).
The values for gumminess of the four gelatin samples are given in . Gumminess is defined as the product of hardness and cohesiveness. From the table it can be seen that porcine gelatin had a higher gumminess value and that from rohu had a lower value. The higher gumminess has arisen from a higher hardness value.[Citation1] Gumminess is a characteristic of semisolid foods with a low degree of hardness and high degree of cohesiveness. Gumminess is a more important textural parameter of semisolids than solids like gelatin. In the present study, the concentration of gelatin used for all TPA measurements was 6.67%. At this concentration, the gelatin was visco-elastic in nature and showed gumminess behavior. Similar results were reported for gelatin from the skin of grouper and commercial gelatin gels.[Citation1]
The values for chewiness of four gelatin sample gels followed the same trend as gumminess attribute. Chewiness is a measure of energy required to masticate the food and is normally reported for solid foods. Chewiness is defined as the product of gumminess and springiness, which is equal to the product of hardness × cohesiveness × springiness. The chewiness value of four gelatin samples varied from 3.86 to 12.45 N. Chewiness is most difficult to measure precisely, because mastication involves compressing, shearing, piercing, grinding, tearing, and cutting along with adequate lubrication by saliva at body temperatures.[Citation33] There is a good correlation between the bloom strength versus chewiness values in the four gelatin samples (r = 0.949).
The resilience value of four gelatin samples is given in . A higher value was obtained for gelatin from the skin of catla and mrigal. Resilience is a measurement of how the sample recovers from deformation both in terms of speed and force.[Citation34] In simple terms, it is the elastic recovery of the sample. The ability of elastic recovery is related to nature of network formed during gelatin gel, which is, in turn, related to the imino acid content, molecular weight distribution, and the extraction procedure followed.[Citation35] From the TPA analysis, it is clear that the textural property of gelatin is in the following order: porcine > catla > mrigal > rohu. The TPA of porcine and carp’s skin gelatin revealed that all TPA attributes except adhesiveness showed significant differences (p < 0.05).
Emulsion Capacity (EC)
The emulsifying capacity of all the gelatin samples studied was found to be decreasing with an increase in concentration (). Emulsifiers are surface-active materials that adsorb to interfaces and facilitate the production of small droplets by lowering the interfacial tension during homogenization.[Citation36] Similar results were reported for bigeye snapper skin gelatin.[Citation16] The higher value of EC recorded for a lower concentration may be due to a higher degree of unfolding of polypeptides during the shearing involved in the emulsifying process.[Citation37] The results of EC revealed that a lower concentration of 0.05% of gelatin was sufficient to prepare oil-in-water emulsions (p < 0.05).
TABLE 4 Emulsion capacity of gelatin from porcine and Indian major carp’s skin
Foam Capacity and Stability
Foam capacity and foam stability of gelatin from carp’s skin and porcine was depicted in . Foam formation ability is another important property of gelatin for commonly used food, such as marshmallows.[Citation38] At a concentration of 4 mg/mL the foam capacity of porcine gelatin is 80%, while that from catla, mrigal, and rohu was 90, 80, and 92%, respectively. The foam capacity of gelatin from giant catfish was higher than that of calf skin gelatin, which was 130 and 110%, respectively.[Citation39] The results of foam capacity revealed that the higher the gelatin concentration, the higher the foam capacity (). Foam formation is generally controlled by transportion, penetration, and reorganization of protein molecules at the air-water interface. The results indicate that the difference in foam capacity between gelatin samples could be due to the higher content of hydrophobic amino acid residues. In the present investigation, results revealed that the lower the gelatin concentration, the higher the foam stability. At a gelatin concentration of 4 mg/mL, the foam stability of porcine gelatin was 85.5% while that from catla, mrigal, and rohu was 61.8, 77.1, and 72.9%, respectively. In the present study, the relationship between gelatin concentration and foam stability was found to be inverse.
CONCLUSION
The bloom strength of gelatin gel from the carp’s skin was in the range of 367–258 g, which was significantly lower than porcine skin gelatin (p > 0.05). The difference in textural properties of porcine and carp skin gelatin is mainly related to amino acid content. The amino acid profile of gelatin samples revealed that gelatin porcine had higher levels of imino acids than carp skin gelatin. The carp skin gelatin had lower hardness value than the porcine skin gelatin. The hardness is related to the strength of gel structure, which in turn is dependent on imino acid composition. Among the three species of carp studied, the quality of gelatin from the skin of Catla catla is almost on par with porcine skin gelatin. Higher emulsion capacity was obtained at a lower concentration of gelatin from both carp skin and porcine sources. The foam capacity of gelatin was concentration dependent while that of foam stability was inversely proportional to concentration of protein.
FUNDING
The authors gratefully acknowledge the funding received from the National Agriculture Innovation Project (NAIP component-II), Indian Council of Agricultural Research, New Delhi, India.
REFERENCES
- Rahman, M.S.; Al-Mahrouqi, A.I. Instrumental texture profile analysis of gelatin gel extracted from grouper skin and commercial (bovine and porcine) gelatin gels. International Journal of Food Sciences and Nutrition 2009, 60, 229–242.
- Kasapis, S. Developing minced fish products of improved eating quality: An interplay of instrumental and sensory texture. International Journal of Food Properties 2009, 12, 11–26.
- Johnston-Banks, F.A. Gelatin. In: Food Gels; Harris, P.; Ed.; Elsevier Applied Science: New York, 1990; 233–289..
- Ninan, G.; Zynudheen, A.A.; Joseph, J. Physico-chemical and texture properties of gelatin and water gel desserts prepared from the skin of freshwater carps. Fishery Technology 2011, 48, 67–74.
- Jones, R.R.; Steffe, J.F.; Harte, J.B. Sensory firmness scale based on gelatin gels. Journal of Texture Studies 2003, 33, 543–558.
- Szczesniak, A.S. Texture is sensory property. Food Quality Prefer 2002, 13, 215–225.
- Friedman, H.H.; Whitney, J.; Szczesniak, A.S. The texturometer—A new instrument for objective texture measurement. Journal of Food Science 1963, 28, 390–396.
- Burey, P.; Bhandari, B.R.; Rutgers, R.P.G.; Halley, P.J.; Torley, P.J. Confectionery gels: A review on formulation, rheological and structural aspects. International Journal of Food Properties 2009, 12, 176–210.
- Lau, M.; Tang, J.; Paulson, A.T. Texture profile and turbidity of gellan/gelatin mixed gels. Food Research International 2000, 33, 685–671.
- Sanderson, G.R. Gellan gum. In: Food Gels; Harris, P.; Ed.; Elsevier Science Publishing Co., Inc.: New York, 1990; 201–232.
- Yang, H.; Wang, Y.; Jiang, M.; Oh, J.; Herring, J.; Zhou, P. 2-Step optimization of the extraction and subsequent physical properties of channel catfish (Ictalurus punctatus) skin gelatin. Journal of Food Science 2007, 72, 188–195.
- Karim, A.A.; Bhat, R. Fish gelatins: Properties, challenges and prospects as alternative to mammalian gelatine. Food Hydrocolloids 2009, 43, 161–165.
- FAO. The state of world fisheries and aquaculture 2012/FAO annual report. Food and Agricultural Organization: Rome, Italy, 2012; 3–100.
- Gudmundsson, H.; Hafsteinsson, H. Gelatin from cod skins as affected by chemical treatments. Journal of Food Science 1997, 52, 37–39.
- AOAC. Official Methods of Analysis of AOAC International, Vol. II, 16th Edition; Association of Official and Analytical Chemists International: Arlington, VA, 2000.
- Binsi, P.K.; Shamasundar, B.A.; Dileep, A.O.; Badii, F.; Howell, N.K. Rheological and functional properties of gelatin from the skin of bigeye snapper (Priacanthus hamrur) fish: Influence of gelatin on the gel-forming ability of fish mince. Food Hydrocolloids 2009, 23, 132–145.
- Ishida, Y.; Fugita, T.; Asai, K. New detection and separation method for amino acid by high performance liquid chromatography. Journal of Chromatography 1981, 204, 143–148.
- Sastry, C.S.P.; Tummuru, M.K. Spectrophotometer determination of tryptophan in protein. Journal of Food Science and Technology 1985, 22, 146–147.
- GME. Standardized Methods for the Testing of Edible Gelatin; Gelatin Monograph Versions: Brussels, 2000.
- Rahman, M.S.; Al-Farsi, S. Instrumental texture profile analysis (TPA) of date flesh as a function of moisture content. Journal of Food Engineering 2005, 66, 505–511.
- Swift, C.E.; Lockett, C.; Fryer, A.S. Comminuted meat emulsions: The capacity of meats for emulsifying fat. Food Technology 1961, 15, 468–469.
- Sathe, S.K.; Deshpande, S.S.; Salunkhe, D.K. Functional properties of lupin seed (Supinus mutabilis) proteins and protein concentrates. Journal of Food Science 1982, 7, 191–197.
- GME. Gelatin manufacturers of Europe. 2008. http://overview/127.htm (accessed March 15, 2008).
- Jones, R.N. Use of gelatin in edible products. In: The Science and Technology of Gelatin; Ward, A.; Courts, A.; Eds.; Academic Press Inc.: London, UK, 1977; 366–394.
- Damodaran, S.; Kinsella, J.E. Effects of hydrocolloids on protein conformation and functionality. In: Food Protein Deterioration: Mechanisms and Functionality; Cherry, J.P.; Ed.; ACS Symposium Series No. 206. American Chemical Society: Washington, 1982; 327–330.
- Ahmad, M.; Benjakul, S. Extraction and characterization of pepsin solubilized collagen from the skin of unicorn leatherjacket (Aluterus monocerous). Food Chemistry 2010, 120, 817–824.
- Arnesen, J.A.; Gildberg, A. Preparation and characterization of gelatin from the skin of harp seal (Phoca groendlandica). Bioresource Technology 2002, 82, 191–194.
- Haug, I.J.; Draget, K.I.; Smidsrod, O. Physical and rheological properties of fish gelatin compared to mammalian gelatin. Food Hydrocolloids 2004, 18, 203–213.
- Gomez-Guillen, M.C.; Turnay, J.; Fernandez-Diaz, M.D.; Ulmo, N.; Lizarbe, M.A.; Montero, P. Structural and physical properties of gelatin extracted from different marine species: a comparative study. Food Hydrocolloids 2002, 16, 25–34.
- Gilsenan, P.M.; Ross-Murphy, S.B. Visco-elasticity and thermo reversible gelatin gels from mammalian and piscine collagens. Journal of Rheology 2000, 44, 871–883.
- Muyonga, J.H.; Cole, C.G.B.; Duodu, K.G. Extraction and physico-chemical characterization of nile perch (Lates niloticus) skin and bone gelatin. Food Chemistry 2004, 86, 325–332.
- Radocaj, O.F.; Dimic, E.B.; Vujasinovic, V.B. Optimization of the texture of fat-based spread containing hull-less pumpkin (Cucurbita pepo L.) seed press-cake. Acta Periodica Technologica 2011, 42, 131–143.
- Bhale, S.D. Effect of Ohmic Heating on Color, Rehydration and Textural Characteristics of Fresh Carrot Cubes. Master’s Dissertation, Louisiana State University and Agricultural and Mechanical College: Baton Rouge, LA, 2004.
- Brenda, C.; Fermin, T.S.; Hahm, J.A.; Radinsky, R.J.; Kratochvil, J.E.; Hall, M.L.Y. Effect of proline and glutamine on the functional properties of wheat dough in winter wheat varieties. Journal of Food Science 2005, 70, E273–E278.
- Liu, H.Y.; Han, J.; Guo, S.D. Characteristics of the gelatin extracted from Channel Catfish (Iactalurus punctatus) head bones. Food Science Technology 2009, 42, 540–544.
- Walstra, P. Physical Chemistry of Foods; Marcel Dekker: New York, 2003; 834 pp.
- Kinsella, J.E. Functional properties of proteins in foods: A Survey. Critical Review Food Science 1976, 7, 219–280.
- Cho, S.M.; Kwak, K.S.; Park, D.C.; Gu, Y.S.; Ji, C.I.; Jang, D.H.; Lee, Y.B.; Kim, S.B. Processing optimization and functional properties of gelatin from shark (Isurus oxyrinchus) cartilage. Food Hydrocolloids 2004, 18, 573–579.
- Jongjareonrak, A.; Rawdkuen, S.; Chaijan, M.; Benjakul, S.; Osako, K.; Tanaka, M. Chemical compositions and characterization skin gelatin from farmed giant catfish (Pangasianodon gigas). LWT–Food Science and Technology 2010, 43, 161–165.