Abstract
An ion chromatographic method for the quantitative determination of free cyanide in bottled natural mineral waters were measured in terms of selectivity, linearity, the limit of detection, limit of quantification, repeatability, precision, and accuracy. Chromatographic separation of free cyanide ions was accomplished with an anion-exchange column and detected by pulsed amperometric detection with a silver working electrode. The method was found to be selective, linear (r2 = 0.999) at a concentration range of 0.5 to 134 μg L−1, precise, and accurate. Recovery values of free cyanide in all classes of natural mineral water varied from 65.9 ± 1.6 to 95.2 ± 0.7 at different spiking levels (5–70 μg L−1). Parameters (total dissolved solids, mineral interferences, and added sodium hydroxide) affecting the recovery values were studied in this project. The limit of detection and limit of quantification were found to be 0.295 and 0.983 μg L−1, respectively. The proposed method was applied to 27 different brands of commercially available bottled natural mineral water products sold in Turkish markets. These natural mineral waters were classified as: (i) very low mineral concentration, (ii) low mineral concentration, (iii) intermediate mineral concentration, and (iv) high mineral concentration based on their total dissolved solids contents according to European Union Directive (Directive 80/777/EEC). Levels of free cyanide residues in the samples ranged from > limit of detection to 6.12 μg L−1. The highest average concentration of free cyanide residues was found in the class of “high mineral concentration waters.” However, the determined free cyanide values in all of the tested natural mineral water samples were found to be within the limits of European Union legislation.
INTRODUCTION
Cyanide is an important environmental contaminant that occurs in surface and ground waters as a result of agricultural discharge and/or industrial waste, such as from the metal finishing industries, iron and steel mills, runoff from the disposal of cyanide waste in landfills, pesticides, and the use of cyanide-containing road salts.[Citation1,Citation2] Furthermore, cyanide is widely used in the gold-mining sector and approximately 2500 tons of sodium cyanide is used annually in Turkey for gold and silver recovery.[Citation3]
The presence of cyanide in drinking water, food, and in other environmental sources is a matter of great concern, since it could have harmful effects on human health. Cyanide is acutely toxic to mammalians by all routes of administration. Acute cyanide toxicity is a result of cellular hypoxia and cytotoxic anoxia, which is potentially fatal.[Citation4] Long-term ingestion of cyanide is responsible for various toxic effects, including weight loss, impaired thyroid function, and neuronal disturbances.[Citation5]
Due to the negative health effects of cyanide, international authorities have established regulations and minimum-level requirements, which are continuously updated as new knowledge becomes available. According to the current regulations, the highest concentration of cyanide allowed in drinking water by the US Environmental Protection Agency (EPA) is 200 μg L−1.[Citation6] Directive 1998/83 of the European Union on the quality of drinking water sets an even lower limit of 50 μg L−1.[Citation7] The maximum concentration of cyanides allowed in mineral water according to Directive 2003/40/EU is 70 μg L−1.[Citation8]
A number of methods have been published to quantify free cyanide, including (the values in parentheses correspond to the reported detection limits of the methods) capillary electrophoresis (CE) after single-drop micro extraction (2 μg L−1);[Citation9] chemiluminescence (0.1 μg L−1);[Citation10] stopped-flow spectrophotometric detection (0.5 μg L−1);[Citation11] headspace single-drop micro extraction involving a reaction with ninhydrin;[Citation12] and ion chromatography (IC) with pulsed amperometric detection (PAD) using standard (2 μg L−1)[Citation13] or disposable silver electrodes (1 μg L−1).[Citation14] From among these methods, IC has been known to be a simple and reliable method for the determination of free cyanide residues in water samples. The most important advantages of IC are the possibility of a simultaneous determination of several ions, the short analysis time, low detection limits, the small amount of sample needed for analysis, and the possibility of using various detectors. IC also involves a simple method for sample preparation (in the case of water, it is usually sufficient to filter the water through a filter with a pore diameter of 0.45 μm) and this enables the simultaneous detection of both cations and anions.[Citation15] In spite of these advantages, the determination of free cyanide may be significantly affected due to the influence of high ionic strength matrices with a high mineral content and/or total dissolved solids. These factors could cause potential interference and the loss of analyte sensitivity due to the overloading of the exchange sites on the analytic column and this may significantly reduce the target peak area of the analyte. The causes of possible interference that have been cited are nitrate, bromide, nitrite, chloride, chlorine, sulphide-generating compounds, and carbonyl compounds.[Citation14,Citation16,Citation17] Natural mineral water can typically contain elevated levels of these substances. According to European legislation (Directive 80/777/EEC), natural mineral waters are classified into four major classes based on their mineral compositions: (i) very low mineral concentration (total dissolved solids (TDS) <50 mg L−1); (ii) low mineral concentration (TDS 50–500 mg L−1); (iii) intermediate mineral concentration (TDS 500–1500 mg L−1); and (iv) high mineral concentration (TDS >1500 mg L−1).[Citation18] Natural mineral water with a high ionic strength has concentrations of ions that extend over several orders of magnitude and, therefore, there has been an increasing need for comprehensive information on the possible effects of the interferents in order to enable a more accurate detection of trace levels of free cyanide in natural mineral water.
Recently, there has been a tremendous increase in consumer demand for bottled drinking water all over the world. People choose to drink bottled water for many reasons, such as a concern about the microbiological quality of municipal water supplies, to avoid chemicals such as chlorine used in water treatment, a better taste, and a perception that bottled water is in some way healthier than tap water.[Citation19] Over the past 5 years, consumption of tap water in Turkey has declined as sales of commercially available bottled water have risen. According to the sales data in Turkey, a total of 8.7, 9, 9.5, 9.9, and 10.2 billion liters of bottled drinking water were sold in 2008, 2009, 2010, 2011, and 2012, respectively. It is expected that bottled water sales will reach 10.5 billion liters in 2013.[Citation20] As drinking water may be an important source of cyanide intake, the shift in consumption to bottled natural drinking water may have important implications for health and disease. Therefore, the aims of this study were (1) to improve an in-house validated ion chromatographic method for the determination of free cyanide and (2) to determine whether differences in the free cyanide content of commercially available bottled natural mineral water consumed in Turkey could be classified based on the EU legislation.
MATERIALS AND METHODS
Chemicals Standards and Samples
Sodium hydroxide, sodium acetate, and ethylene diamine were purchased from Merck (Darmstadt, Germany). Methane sulfonic acid was obtained from Fluka (St. Louis, MO, USA). Anions (chlorine, nitrate, nitrite, and sulphate), cations (sodium and calcium), and free cyanide analytical standards were obtained with a purity certificate from Inorganic Ventures (Christiansburg, VA, USA). With the exception of the reference standards, all chemicals used were of analytical grade. Standard solutions were prepared by dilution in ultrapure water to achieve the required concentrations and were stored in a refrigerator (+4°C). The water was produced by an ultrapure (18.2 MΩ cm at 25°C) purification system from Millipore (Bedford, MA, USA). Twenty-seven different brands of bottled natural mineral water were collected from local supermarkets and chain stores between February 2012 and April 2012 in Antalya, Turkey. All of the collected samples were transferred to the laboratory and analyzed within the sampling day. The bottles were kept sealed and refrigerated at +4°C until the time of analysis. The samples were classified based on the relevant EU legislation, as shown in .
TABLE 1 Classification of the samples
Instrumentation
Conductivity, TDS, salinity, resistance, and pH values of the samples were measured by a conductivity meter (Mettler Toledo S230, Switzerland) and a pH meter (Mettler Toledo S220, Switzerland). Chromatographic analyses were performed on an ICS-5000 IC system (Sunnyvale, CA, USA) that included a dual isocratic pump with automated membrane eluent degassing, an electrochemical detector (ED), and an AS40 auto sampler. The ED module contained an electrochemical cell with a combination pH–Ag/AgCl reference electrode and a silver working electrode. A Chromeleon 6.80 Data Management System® (Dionex) was used for instrument control and data handling. All measurements were carried out isocratically and at room temperature. The column and compartment temperatures were thermostatted at 30°C. The other chromatographic and detection conditions are summarized in . The waveform used for this study was selected from another publication where it was optimized for iodide, sulphide, cyanide, and thiosulfate detection using a silver disposable working electrode.[Citation21]
TABLE 2 Ion chromatographic conditions for the analysis of cyanide
The analysis of the anions and cations in the samples was carried out using the same IC system with some modifications, such as a new module. This module consisted of an Eluent Generator with a Dionex EGC III KOH Cartridge, an Anion Self-Regenerating Suppressor (ASRS® Ultra II), and a conductivity detector in order to determine the anions that were present. Cations were analyzed in the same module by using a cation column and conductivity detector. Dionex IonPac™ AS19 (250 mm × 2 mm i.d.) and IonPac™ CS12A (250 mm × 2 mm i.d.) columns were used for the separation of all anions and cations, respectively. The mobile phases were 12–45 mM KOH produced by the Dionex EGC III KOH cartridge for anion analysis and 20 mM methane sulfonic acide for the cation analysis. The other chromatographic parameters (flow rate, injection volume, and temperatures) were the same as for the cyanide analysis. Three replications were undertaken for each experiment.
Interference Study
The interference study was carried out with a constant free cyanide concentration and different interferents at variable concentrations in ultrapure water. The possible interferents were specified in accordance with the literature study as calcium, sodium, chlorine, carbonate, sulphate, nitrate, and nitrite.[Citation13,Citation14,Citation22] All of the bottled mineral water samples were analyzed using the IC system and the concentration patterns of the interferents were determined. Solutions of 70 μg L−1 cyanide in ultrapure water were prepared and additionally spiked with a known concentration of the interferents ions according to their patterns that were found in the samples. The solutions were analyzed and percentage recovery values of free cyanide were calculated. The effects of each ion on free cyanide recovery are discussed.
Method Validation
The validation of the analytical method was performed using the following parameters: linearity over a 250-fold concentration range, selectivity, limit of detection (LOD), limit of quantification (LOQ), recovery (precision and accuracy), and repeatability for the peak area and retention time.
Statistical Analyses
The obtained results were statistically analyzed with ANOVA. All statistical analyses were performed using the Statistical Analysis System (SAS).[Citation23]
RESULTS AND DISCUSSION
Data-Method Validation
The method demonstrated an acceptable performance for the analysis of free cyanide in bottled natural mineral water samples. The linearity of the assay was checked by calculating the regression line using the least squares method and this was expressed by the coefficient of determination. The calibration curve was linear (r2 = 0.999) in the range of 0.5–134 μg L−1. The linear-range mathematical equation (y = 11.93x – 0.66) was used for all tested natural mineral water samples, since their free cyanide levels were in the linear range of the calibration curve.
The selectivity of the method was checked by injecting blank natural mineral water, a mobile phase, a free cyanide standard, and a sample (spiked) with free cyanide into the ion chromatograph for detection. shows a representative chromatogram for a blank natural mineral water sample, a spiked natural mineral water sample with 70 μg L−1 of cyanide, and a natural mineral water sample with a free cyanide residue. The chromatograms in the figure show that free cyanide was well separated with no interfering peaks and the noise was similar regardless of matrices, suggesting the excellent selectivity of this method.
FIGURE 1 (A) Chromatogram of natural mineral water sample (no cyanide residue was detected); (B) Chromatogram of natural mineral water sample spiked with 70 μg L−1 of free cyanide standard; (C) Chromatogram of natural mineral water sample with free cyanide residue (3.47 μg L−1).
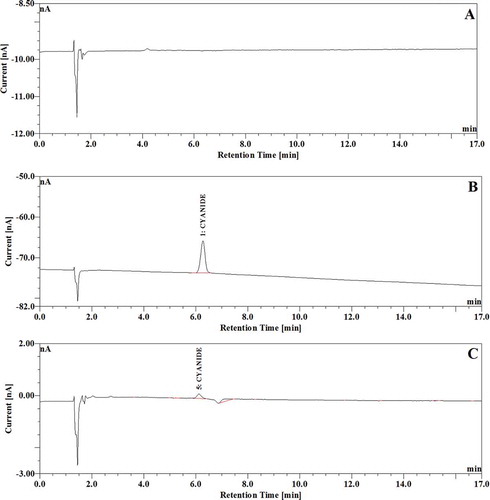
The method’s LOD, LOQ, repeatability, and recovery were conducted according to the Eurachem guide.[Citation24] The LOD was estimated as 3 times the standard deviation, while the LOQ was estimated as 10 times the standard deviation, which was derived from the analyses of 10 independent samples spiked with the lowest calibrated level. The LOD and LOQ were estimated to be 0.295 and 0.983 μg L−1, respectively. The obtained LOD value was lower than those reported for UV-Vis methods[Citation25,Citation26] and some other ion chromatographic methods.[Citation13,Citation14]
Repeatability of the method was checked by assaying six replicates of blank natural mineral water samples spiked with free cyanide at a concentration of 70 μg L−1 and was expressed as the relative standard deviation (RSD; %). The free cyanide concentration was selected as 70 μg L−1 based on the maximum contaminant level (MCL), as described by the EU legislation. The obtained RSD values were 0.70, 0.74, 0.98, and 1.77 for ultrapure water as a very low mineral concentration, low mineral concentration, intermediate mineral concentration, and high mineral concentration, respectively. In addition, there was a negligible retention time drift. The repeatability of the retention time was 0.954% (RSD). Repeatability was also checked using lower concentrations because determined free cyanide concentrations of the samples were lower than the permissible level by the EU legislation. Thus, RSD value was estimated as 2.41% for 5 μg L−1 in a sample with intermediate mineral concentration.
TABLE 3 Recoveries of cyanide in natural mineral water samples spiked with various concentration of cyanide
TABLE 4 Effect of interference on determination of cyanide (single compound interference)
For the recovery study, the cyanide standard was spiked at a final concentrations of 5, 10, 25, and 70 μg L−1 in natural mineral water samples classified as TDS 2, TDS 3, and TDS 4. Blank values for each class of natural mineral water were determined prior to the study. The obtained recovery values and some other chemical specifications for all classes of natural mineral water samples are illustrated in . According to the results from , TDS 2 exhibited the highest recovery value, while more complex matrices, such TDS 3 and TDS 4, did not exhibit a similar recovery. Due to the nature of their composition, the dissolved compounds may potentially act as interference substances during cyanide detection and, thus, the recovery value was reduced. It has been reported that the recovery of cyanide from different real matrices is always cumbersome, as it may undergo precipitation, complexation, and oxidation reactions.[Citation13]
Interference Study
From among the interference compounds, calcium, sodium, chlorine, and sulphate were selected for the interference study, since these compounds were detected in all bottled natural mineral water samples. In addition, it was found that none of our samples contained nitrate or nitrite. Therefore, these compounds were not included in the study. The concentrations of the other interferents in the tested natural mineral waters were found to be 43.06 ± 41.03, 60.84 ± 58.67, 6.75 ± 7.68, and 7.85 ± 9.32 for calcium, sodium, chloride, and sulphate, respectively. Two different concentrations of calcium, sodium, chlorine, and sulphate were selected based on their detected concentration patterns in the samples. Carbonate was also included in the study, since most natural mineral water may contain high levels of carbonate. However, the concentration of carbonate could not be determined in the ion chromatographic system. Therefore, the concentrations of carbonate, which were declared on the label of the water samples, were used for the selection of carbonate concentrations in the study. To evaluate the effects of these major ions on free cyanide determination, a 70 μg L−1 free cyanide standard was treated with each of the major ions at two concentrations and then compared with an untreated standard. shows the recovery values of free cyanide concentrations in the presence of a single interferent.
The recovery values of free cyanide were good in the presence of 10 mg L−1 calcium and 20 mg L−1 sodium. However, cyanide recoveries decreased at a higher concentration of sodium and calcium due to high ionic strength. According to the results, sulphate and chloride significantly affected free cyanide recovery. The recovery of 70 μg L−1 free cyanide in the presence of 10 mg L−1 sulphate was 90.70 ± 1.69. It has been reported that sulphur compounds react rapidly with cyanide. These compounds can convert cyanide to thiocyanate, as shown in the reaction: (8CN− + S8 → SCN−). Thus, the recovery values of cyanide can be reduced by sulphur-compound interference. Christison and Rohrer[Citation14] reported that the recovery of cyanide (10 μg L−1) in water samples was 95.9 ± 1.7 when spiked with sulphide at 20 μg L−1. In addition, there are reports that chloride can produce low readings during cyanide determination.[Citation27] The silver chloride precipitation reaction is well known and, therefore, chloride is expected to reach the electrode and could affect the electrode’s response to free cyanide.[Citation14] We also used a silver working electrode for the determination of free cyanide. In addition, chloride reacts with free cyanide to form cyanogen chloride (CNCl), which, under alkaline conditions, hydrolyzes to cyanate (CNO−).[Citation17] The observed decrease in the recovery values in natural mineral water could be attributed to the chlorine concentration of natural mineral water. We also observed a small increase in cyanide recovery in the carbonate interference experiments. All of the carbonate interferents produced positive errors and the recoveries were over 100%. It has been reported that carbonate can accumulate by adherence to the anion-exchange resin of the analytical column. This may eventually lead to an unstable baseline and a reduction in column capacity and analyte retention.[Citation22]
It was reported that solutions containing cyanide must be stabilized with sodium hydroxide when pH > 9, because cyanide generates poisonous hydrogen cyanide gas at neutral or acidic pH.[Citation14] It is possible that the determination of free cyanide could be affected by the addition of NaOH. To evaluate the effects of NaOH on free cyanide determination, we prepared 70 μg L−1 cyanide standards in solutions including various levels of NaOH (0, 12.5, 25, 50, and 100 mM NaOH) and the recovery values were calculated. The results showed that there was no statistically significant difference among the recovery values obtained from the concentrations of 12.5, 25, 50, and 100 mM NaOH (p > 0.05). However, a recovery value of 92.10 ± 0.35 was obtained in the study without NaOH addition. A decrease in the recovery value could be explained by the variation in the pH value of the solution. The use of NaOH prevents the loss of hydrogen cyanide gas by increasing the pH level of the solution. Furthermore, hydrogen cyanide gas is highly poisonous by all routes of administration.[Citation28] Therefore, it is recommended that samples with free cyanide residues must be stabilized with sodium hydroxide to a pH > 9 for analytical requirements and health reasons.
Application to Real Samples
The method was applied to the determination of free cyanide in natural mineral water samples. Different brands of water samples from various manufacturers were purchased from supermarkets in Antalya, Turkey. Collected water samples were classified according to their TDS value as recommended European legislation (Directive 80/777/EEC). Although, most of the samples were classified in the class of “low mineral concentration,” there was no sample in the class of “very low mineral concentration.” It has been reported that the mineral concentrations of bottled natural mineral waters consumed in Turkey could be higher levels than those of very low mineral concentration. Güler[Citation29] announced that the mean calcium and magnesium levels of 24 different brands of bottled natural mineral waters were found to be 179.8 and 92.9 mg L−1, respectively.
The estimated cyanide concentrations in the tested samples ranged from > LOD to 6.12 μg L−1. The results are given in . The obtained results were consistent with those reported by Güler,[Citation29] who found that free cyanide levels ranged from 0 to 10 μg L−1 in Turkish drinking water.
TABLE 5 Cyanide concentrations in analyzed natural mineral water
The MCLs of free cyanide in natural mineral water samples following the European Union requirements and Turkish legislation are 70 μg L−1.[Citation8] Although some of the tested natural mineral water samples contained various levels of free cyanide residues, none exceeded the levels outlined in the legislation. A comparison of the mean values of free cyanide in different classes of natural mineral water showed that the concentration of cyanide is higher in the high mineral content samples. This difference in the mean free cyanide concentration is expected since these samples are mostly produced from areas close to geothermal regions with deep ground-water circulation patterns.
CONCLUSIONS
Our results show that the method used in the study adequately met the validation criteria and was successfully used to analyze free cyanide residues in natural mineral water. The method is linear across a wide range, with high repeatability and good recovery. The LOQ found here was much lower than the MCL established by the European legislation, indicating that the method may be convenient for the determination of free cyanide residues in natural mineral water. The method may be a better choice due to its simplicity, low solvent requirement, and faster processing time. The sample-preparation time was as brief as 2 min. As a result, this method can be considered for the routine analysis of trace levels of free cyanide in water samples. However, this method faced interference problems during the analysis of natural mineral water containing high levels of chloride and sulphate. Therefore, further research is recommended in the area of the interferent’s removal techniques.
ACKNOWLEDGMENT
The authors are grateful to Food Security and Agricultural Research Centre of Akdeniz University for their help in IC analysis.
FUNDING
The authors wish to thank the Scientific Projects Coordination Unit of Akdeniz University (Antalya, Turkey) for financial support.
REFERENCES
- Dash, R.R.; Gaur, A.; Balomajumder, C. Cyanide in industrial wastewaters and its removal: A review on biotreatment. Journal of Hazardous Materials 2009, 163, 1–11.
- Miralles, E.; Prat, D.; Compano, R.; Granados, M. Assessment of different fluorimetric reactions for cyanide determination in flow systems. Analyst 1997, 122, 553–558.
- Akcil, A. Managing cyanide: Health, safety and risk management practices at Turkey’s Ovacik gold–silver mine. Journal of Cleaner Production 2006, 14, 727–735.
- Ballantyne, B.; Marrs, T.C. Clinical and Experimental Toxicology of Cyanides; Wright: Bristol, UK, 1987.
- Riet-Correa, F. Poisoning By Plants, Mycotoxins, and Related Toxins; CAB International: Oxfordshire, UK, 2011.
- US EPA. Methods for Chemical Analysis of Water and Wastes; United States Environmental Protection Agency (EPA) Environmental Monitoring and Support Laboratory: Cincinnati, OH, 1983.
- EU. Official Journal of the European Union, Commission Directive 1998/83/EC; EC of the European Parliament: Brussels, Belgium, 1988.
- EU. Official Journal of the European Union, Commission Directive 2003/40/EC; EC of the European Parliament: Brussels, Belgium, 2003.
- Jermak, S.; Pranaityte, B.; Padarauskas, A. Headspace single-drop microextraction with in-drop derivatization and capillary electrophoretic determination for free cyanide analysis. Electrophoresis 2006, 27, 4538–4544.
- Gavrilov, A.V.; Druzhinin, A.A.; Zakharov, K.I.; Ishutin, V.A.; Nemkov, S.A.; Pushkin, I.A. Chemiluminescence determination of cyanide ions. Journal of Analytical Chemistry 2005, 60, 1029–1034.
- Sun, B.; Noller, B.N. Simultaneous determination of trace amounts of free cyanide and thiocyanate by a stopped-flow spectrophotometric method. Water Research 1998, 32, 3698–3704.
- Jain, A.; Pillai, A.K.K.V.; Sharma, N.; Verma, K.K. Headspace single-drop microextraction and cuvetteless microspectrophotometry for the selective determination of free and total cyanide involving reaction with ninhydrin. Talanta 2010, 82, 758–765.
- Giuriati, C.; Cavalli, S.; Gorni, A.; Badocco, D.; Pastore, P. Ion chromatographic determination of sulfide and cyanide in real matrices by using pulsed amperometric detection on a silver electrode. Journal of Chromatography A 2004, 9, 105–112.
- Christison, T.T.; Rohrer, J.S. Direct determination of free cyanide in drinking water by ion chromatography with pulsed amperometric detection. Journal of Chromatography A 2007, 1155, 31–39.
- Michalski, R. Environmental applications of ion chromatography in Eastern and Central Europe. Journal of Chromatographic Science 2010, 48, 559–565.
- US EPA. Potentiometric determination of cyanide in aqueous samples and distillates with ion-selective electrode. EPA Method 9213. EPA: Washington, DC, 1996.
- US EPA. Free cyanide in water, soils and solid wastes by microdiffusion. EPA Method 9016. EPA: Washington, DC, 2010.
- EC. Council directive 80/777/EEC of 15 July 1980 on the approximation of the laws of the Member States relating to the exploitation and marketing of natural mineral waters. Official Journal of the European Community 1980, L 229 (23), 1–10.
- Cochrane, N.J.; Saranathan, S.; Morgan, M.V.; Dashper, S.G. Fluoride content of still bottled water in Australia. Australian Dental Journal 2006, 51, 242–244.
- SUDER. About sector–general structure and the size in figures. Association of Packaged Water Manufacturers Bylaw Code: 34-101/114. 2013. http://www.suder.org.tr ( accessed September 4, 2013).
- Cheng, J.; Jandik, P.; Avdalovic, N. Pulsed amperometric detection of sulfide, cyanide, iodide, thiosulfate, bromide and thiocyanate with microfabricated disposable silver working electrodes in ion chromatography. Analytica Chimica Acta 2005, 536, 267–274.
- US EPA. Metal cyanide complexes by anion exchange chromatography and UV detection. EPA Method 9015-1. EPA: Washington, DC, 2004.
- SAS. The SAS system for Windows. SAS Institute, Inc.: Cary, NC, 1998.
- EURACHEM. The Fitness for Purpose of Analytical Methods: A Laboratory Guide to Method Validation and Related Topics; EURACHEM: Uppsala, Sweden, 1998; 75 pp.
- Hamza, A.; Bashammakh, A.S.; Al-Sibaai, A.A.; Al-Saidi, H.M.; El-Shahawi, M.S. Dual-wavelength beta-correction spectrophotometric determination of trace concentrations of cyanide ions based on the nucleophilic addition of cyanide to imine group of the new reagent 4-hydroxy-3-(2-oxoindolin-3-ylideneamino)-2-thioxo-2H-1,3-thiazin-6(3H)-one. Analytica Chimica Acta 2010, 657, 69–74.
- Afkhami, A.; Sarlak, N.; Zarei, A.R. Simultaneous kinetic spectrophotometric determination of cyanide and thiocyanate using the partial least squares (PLS) regression. Talanta 2007, 71, 893–899.
- Heckenberg, A.; Cheng, J.; Jandik, P.; Cavalli, S.; Abballe, F. Determination of sulfide and cyanide using integrated pulsed amperometric detection on a disposable silver electrode. In: The Application Notebook; Dionex Corp.: Sunnyvale, CA, 2004, 32.
- Magnusson, R.; Nyholm, S.; Åstot, C. Analysis of hydrogen cyanide in air in a case of attempted cyanide poisoning. Forensic Science International 2012, 222, e7–e12.
- Güler, C. Evaluation of maximum contaminant levels in Turkish bottled drinking waters utilizing parameters reported on manufacturer’s labeling and government-issued production licenses. Journal of Food Composition and Analysis 2007, 20, 262–272.