Abstract
Jumbo squid (Dosidicus gigas) is an underutilized fishery resource in the Gulf of California. Jumbo squid muscle could potentially be utilized to manufacture protein concentrates to be used as a base for surimi or as a food ingredient. However, the main problem is the low gelling ability of these protein concentrates compared those of with fish species. Consequently, the present study evaluated the effects of pH and the NaCl concentration on the gelling properties of protein concentrates from squid mantle. According to texture profile analysis, improved gelling properties were obtained at pH 6.0 and 1% of NaCl. During the sol-gel transition, decreased surface hydrophobicity that corresponded to an increased intensity of α-helix structures was detected. Moreover, the total sulfhydryl content decreased during gel formation. Viscoelasticity and water holding capacity analyses showed the formation of a more structured gel at pH 7.0. The higher enthalpy and denaturation temperature detected by differential scanning calorimetry at pH 7.0 and 1% NaCl showed that both variables (pH and NaCl concentration) affected the protein structure and conformation. The results demonstrated that varying pHs and NaCl concentrations improved the gelling properties of protein concentrates from squid.
INTRODUCTION
Jumbo squid is an abundant resource in the Mexican northwest. Its muscle characteristics and low market price make it an attractive species for the production of protein concentrates (PCs), allowing for more efficient use of underutilized species.[Citation1] Based on their gel-forming abilities, PCs can be used as a base for the preparation of seafood analogues or of other products such as imitation shrimp, lobster, and crab, among other seafood.[Citation2]
The most common method to obtain PCs is to perform a series of washes with water; the insoluble protein is regarded as the PC. This technology demands a large amount of water because the process includes two to five wash cycles at a 1:5–1:9 muscle:water ratio to completely remove sarcoplasmic protein, pigments, and lipids. However, in the case of the jumbo squid mantle, the application of several wash cycles is not necessary because the myofibrillar and sarcoplasmic proteins of this species are highly soluble in water.[Citation3,Citation4] Additionally, unlike fish species, the jumbo squid does not have pigments and is a much leaner animal.
Gómez-Guillén and Montero[Citation5] obtained a PC from the jumbo squid (Dosidicus gigas) using the traditional method and reported low functional quality of the extracted proteins. Therefore, they proceeded to study the addition of vegetable or animal protein as well as other natural carbohydrate based polymers to improve the gelling properties.[Citation6] However, Cortés-Ruiz et al.[Citation7] and Dihort-García et al.[Citation8] obtained PCs from jumbo squid (Dosidicus gigas) using acidic and alkaline solubilization, respectively, and evaluated the gelling capacity. These authors reported an improved gelling ability; nevertheless, this property was still inferior to that reported for several fish species.
Currently, 80% of jumbo squid production is designated for export, whereas the remaining 20% is sold in the domestic market as fresh-frozen products.[Citation9] Therefore, it is necessary to explore alternative options aimed at generating a value-added product such as PCs. In this sense, jumbo squid (Dosidicus gigas) is a species with outstanding characteristics for PCs or surimi because of its abundance, performance, white color, and low lipid content. However, jumbo squid proteins do not produce good gels compared with those of other marine sources. Consequently, it is necessary to study alternate methods to improve the gelling properties of squid-based PCs. In this sense, both the traditional method to obtain PCs and the acid/alkali dissolution method of protein extraction have been studied. However, no studies have assessed the effects of pH and salt concentration on the gelation of squid protein. Therefore, in this study, we evaluated the effect of these variables on the gelling properties and protein conformational changes to explain the textural characteristics of the obtained gels.
MATERIALS AND METHODS
Samples
Jumbo squid (D. gigas) was harvested off of the coast of Kino Bay, Mexico in May 2012. Ten specimens with an average mantle length of 31.6 ± 2.5 cm and a weight of 757 ± 7.50 g were decapitated, gutted on site, and then washed with fresh water at room temperature (25°C). The mantles (experimental samples) were bagged and placed in alternating layers of ice-squid-ice in a portable cooler for transport to the laboratory. The elapsed time between capture and reaching the laboratory did not exceed 12 h.
Generation of PC
The squid mantle was defrosted at 5°C over the course of 12 h. Then, it was chopped into small pieces, homogenized with refrigerated water (4–6°C) at a 1:3 (mantle:water) ratio using a tissue homogenizer and centrifuged at 16,000 × g for 15 min at 2°C in a refrigerated centrifuge (Sorvall Biofugue Stratos, Thermo Scientific, Germany). The supernatant was discarded, and the precipitate was considered as the PC. The PC was packed in polyethylene bags and stored on ice in a refrigerated chamber until use on the same working day.
Viscoelasticity
Rheological changes in the sols were monitored using a rheometer with parallel plate geometry and a 1 mm gap (Rheometric Scientific, RFS III, New Jersey, Piscataway). To identify the linear viscoelastic region, a stress sweep (angular frequency 6.28 rad/s) was performed in the range of 10–4000 Pa at 10°C. Storage modulus (G´) changes were recorded. Frequency sweeps were performed on the samples in the 0.1–10 Hz range at 0.5% strain, and G´ and G´´ moduli changes were recorded from 5°C. For temperature sweeps, the oscillation temperature ramp mode (1°C/min) was used to heat the samples from 5 to 80°C. The frequency (0.5 Hz) and strain (c = 0.5%) were fixed.[Citation10]
Differential Scanning Calorimetry (DSC)
A 20 mg sample was placed in a calorimetric cell, and adequate contact between the sample and the lower face of the capsule was ensured. All samples were scanned at a heating rate of 5°C/min in the range of 10–90°C in a DSC (DSC 8000, Perkin Elmer, Shelton, USA). The total enthalpy of denaturation was estimated by measuring the area under the curve.[Citation11]
Determination of Total Sulfhydryl (TSH) Content
TSH content was measured according to the method of Patrick and Swaisgood.[Citation12] To 1 mL of protein solution (2%), 1 mL of 10 mM 5,5’-dithiobis(2-nitrobenzoic acid; DTNB) in 20 mM Tris-HCl buffer (pH 7.0) was added followed by the addition of 1.44 g of urea. After incubation at 40°C for 25 min, the absorbance was measured at 412 nm using a spectrophotometer (Varian, Cary 50, Walnut Creek, California). The TSH content was calculated using a molar extinction coefficient of 13,600 M–1cm–1. The reactive sulfhydryl (RSH) content was measured by mixing 5 mL of protein solution (2%) with 1 mL of 10 mM DTNB in 20 mM Tris-HCl buffer (pH 7.0). The reaction mixture was incubated at 40°C for 25 min, and the absorbance was measured at 412 nm using a spectrophotometer (Varian, Cary 50, Walnut Creek, California).
Fourier Transform Infrared Spectrometry (FTIR)
A total of 3 mg of lyophilized sols and gels (Labconco, Freezone 4.5 model Buenos Aires, Argentina) was taken for infrared analysis and then mixed with 35 mg of potassium bromide to form a pill. The analysis was performed in an infrared spectrophotometer (Perkin Elmer, Model Spectrum GX, Washington, DC) ranging from 400 to 4000 cm–1.[Citation13]
Heat-Set Gel Preparation
Different sols were prepared from the PCs by adding 1 and 3% NaCl. Then, the pH was adjusted to 6.0 and 7.0 using Na2CO3, allowing for the generation of four different PCs. The paste was homogenized at short intervals in a Model DLC-8 Plus Cuisinart Food Processor (Cuisinart Inc., Greenwich, CT). Each sol was packed into a Petri dish (1 cm height) and vacuum sealed in moisture/vapor-proof film bags (Cryovac Corp., Duncan, SC) with a Super Vac Smith vacuum machine (Smith Equipment Co., Clifton, NJ). Each sol was heat set in a water bath at 90°C for 30 min. Heat-set gels were immediately chilled to 5–10°C in an ice/water mixture and maintained at 2–4°C overnight prior to functional evaluation.
Gel-Forming Ability (GFA)
Gels were tempered for 60 min at room temperature (25°C) prior to analysis. The GFA was evaluated by the folding test (FT) and texture profile analysis (TPA). The FT was performed as described by Tanikawa et al.[Citation14] TPA was conducted on cylinder-shaped samples of uniform dimensions (1-cm diameter and 1-cm height) obtained from each gel using a sharp-edged plastic tube. Texture was measured using a TA-XT2 Plus Texturometer (Food Technology Corp., Sterling, VA) with a 3.8-cm diameter compression plunge attached to a 100 N load cell. Compression forces at 75% of the original gel sample height were used to calculate compression hardness, gel strength, fracture, cohesiveness, and elasticity.
Water Holding Capacity (WHC)
The WHC was measured using the methodology reported by Cheng et al.[Citation15] A 5-g portion of each gel was centrifuged in a refrigerated centrifuge at 3000 × g for 20 min at 4°C (Sorvall Biofugue Stratos, Thermo Scientific, Germany). The WHC was expressed as “water retained,” representing the percentage of sample weight loss with regards to the initial weight.
Statistical Analysis
In this experiment, a factorial design with two main effects was used: main effect A, pH, with 2 levels (6.0 and 7.0); and main effect B, NaCl concentration, with 2 levels (1.0 and 3.0%). The response variables used were the viscoelastic properties (G’), texture (hardness, fracture, adhesiveness, springiness, and cohesiveness), WHC, thermal protein denaturation (Td and ΔH), TSH, and RSH contents and surface hydrophobicity. When required, Tukey’s multiple comparisons tests were performed using a significance level of 95%. Data were analyzed using Jump software version 5.0.1. The infrared data and electrophoretic profiles were not subjected to any statistical analysis for either variable; only descriptive analysis was performed.
RESULTS AND DISCUSSION
Protein Concentrate Yield
A PC yield of 58.68 ± 5.72% was obtained using a modified conventional method (only one wash cycle) with a humidity of 90.58 ± 2.62%. The standard process for producing PC was based on the use of several washing cycles with the purpose of a better removal of sarcoplasmic proteins, pigments, lipids, and impurities. However, due to the inherent characteristics of the squid muscle such as high protein solubility (easy removal of sarcoplasmic protein), the absence of pigments, lean muscle, and lack of leaves or thorns, we used a single wash cycle.[Citation4] The protein yield can vary depending on the extraction method used; in this sense, Dihort-Garcia et al.[Citation8] using alkaline dissolution of squid mantle (Dosidicus gigas), reported a yield of 43.87 ± 2.11%, which was a similar recovery to that obtained by Cortes-Ruiz et al.[Citation7] who reported a recovery of 48.2 ± 6.0% using acid dissolution of squid mantle protein. Therefore, modifying the traditional PC procurement process resulted in enhanced protein recovery compared with other extraction methods used for the same species.
Viscoelasticity
shows the effects of pH and the NaCl concentration on changes in the storage modulus (G’) as a function of temperature. The heating cycle began at 5°C. A continuous increase in the elastic modulus was then detected from 21.75 to 37.64°C with a subsequent immediate decrease in G’ to a minimum value within the range of 41.83–58.52°C. The first increase in the elastic modulus could be attributed to protein unfolding caused by the disruption of electrostatic interactions, whereas subsequent reductions could be due to the denaturalization of light meromyosin, causing fluidity. Similarly, Tolano-Villaverde et al.[Citation13] found that a small drop of G’ at 25°C was indicative of unfolding of the α-helix from the myosin heavy chain. Moreover, Siegler and Acton[Citation16] reported that heating actomyosin at temperatures below 40°C generally results in the dissociation of proteins, thereby affecting the dissociation of tropomyosin from the F-actin backbone. This unfolding could be a consequence of the effects of temperature, pH, and ionic strength.
The second increase in G’ might be due to the formation of a continuous protein network, mainly driven by the crosslinking of myosin.[Citation17] An increased G’ offers a more rigid system, which is advantageous in the thermal gelation process. G’ values rapidly increased in the treatments after 60°C and reached a value between 1026 and 11,606 Pa by the end of the trial. Egelandsdal et al.[Citation18] suggested that the increase in G’ after 47°C is most likely due to the irreversible formation of a gel network. Differences in the magnitude of G’ were due to different pH values and NaCl concentrations; however, the NaCl concentration appears to have a greater impact than the pH because increased G’ values were detected in sols with 3% NaCl and were higher at pH 7.0 than at pH 6.0. The subsequent decrease is presumably related to the dissociation of actin-myosin complexes and unfolding of the myosin α-helix rod portion[Citation19] because actin-myosin complex dissociation and light meromyosin denaturation led to increased fluidity.[Citation18]
DSC
The temperature (Tm) and energy (ΔH) of protein denaturation found in the sols of PCs are shown in . Two endothermic peaks were detected, which might correspond to the transitions of myosin and actin. The endothermic transitions of myosin and actin from the muscle of giant squid (Dosidicus gigas) are present at 50 and 79°C, respectively.[Citation20] Thus, a greater effect was found in myosin; this difference may be due to variations in the extraction procedure. These proteins were important for thermally induced gelation and were denatured at different temperatures. Therefore, the variations found in the present experiment can be related to a combined effect of the pH and salt concentration because these variables can shift the denaturation temperature.[Citation11]
TABLE 1 Effect of pH and NaCl concentration on Tm and ΔH of protein concentrates
shows that myosin denaturation is pH dependent (p ˂ 0.05) because no significant differences (p ≥ 0.05) were detected for the effect of NaCl. This result could be attributed to increased availability of the ionizable groups of the protein at pH 7.0. These ionizable groups confer an enhanced ability to interact with other proteins, NaCl ions and water, yielding increased thermal stability.[Citation21] For the Tm of actin, no differences were found related to the pH (p ≥ 0.05) at the same NaCl concentration; however, differences were detected according to the NaCl concentration (p < 0.05). The enthalpy of denaturation of both peaks at pH 6.0 at any NaCl concentration was lower compared with that at pH 7.0. This outcome indicates that less energy is required for thermal denaturation at pH 6.0, which is attributable to pH 6.0 being near the pI of inter- and intramolecular salt bridges compared with pH 7.0.
TSH Content
SH groups are exposed during heat treatment as a result of protein unfolding; this is the same process that occurs during gelation. During the sol-gel transition, the exchange of -SH by S-S takes place. A decrease in the total TSH content indicates the formation of disulfide bridges. The results of TSH quantification in the sols and gels of PCs are shown in . The obtained results are lower compared with other species such as burbot (Lota lota) and Alaska pollock (Gadus morhua), which have 5.2 and 8.2 mol/105 g, respectively,[Citation22] and could be due to the inherent characteristics of each protein system such as the high paramyosin concentration in squid muscle, which is found in a very similar proportion. The low myosin level could be the main cause of the lower TSH content in Dosidicus gigas muscle.[Citation4]
FIGURE 2 Effect of pH and NaCl concentration on the TSH content of sols and gels. Values are mean ± SD of n = 4. Different letters on bars indicate significant differences between treatments (sol or gel; p < 0.05).
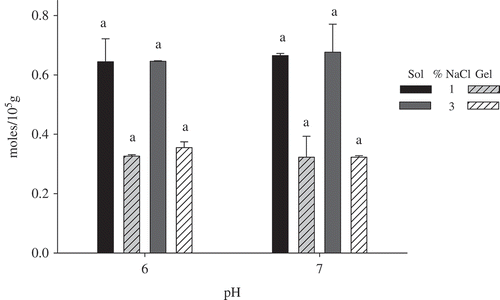
Due to the thermal treatment applied during gelation, we expected a decrease in the TSH found in gels in relation to the sols (p < 0.05) because the heat supplied during gelation exposes the reactive SH groups and leads to the consequent formation of S-S, thereby reducing the TSH.[Citation23] Similar behavior has been reported by Ingadottir and Kristinsson,[Citation24] who compared the paste protein TSH content before and after cooking and reported a decreased TSH content.
No differences (p ≥ 0.05) in TSH reduction were found for any of the treatments, demonstrating that neither the pH nor the salt concentration has a significant role in disulfide bond formation during the gelation of PCs from squid mantle. Consequently, the differences detected in the rheological study and the ability to form gels (TPA) could not be attributed to this variable.
Infrared Spectrometry
The types of conformations that determine secondary structure can be estimated by the peak intensity at 1658–1654 cm–1 for α-helices and 1642–1624 cm–1 for β-sheets.[Citation25] Infrared (IR) studies in sols and gels are shown in . In general, each of the IR spectra show a broad band between 3314–3294 and 3298–3281 cm–1 (both sols and gels) that can be attributed to the stretching of OH groups. During the sol-gel transition, we found that the pH and the NaCl concentration influenced the IR spectrum. The pH and salt concentration caused variations in the band intensity related to the presence of S-S bonds. Certain chemical conditions may or may not induce the formation of disulfide bonds, which could influence rheological properties. However, in this study, no significant differences were observed for the treatments used. According to Venugopal,[Citation26] gelation involves the aggregation of head and tail portions due to hydrophobic interactions and disulfide bonds. Therefore, S-S formation is expected, as evidenced by the decreased TSH content ().
The structural changes of proteins during gel formation must allow for the development of a three-dimensional network that supports the formed gel. This phenomenon can be established by monitoring certain bands such as the characteristic α-helix structure band (1658–1654 cm–1), for which an increase in intensity was observed during the sol-gel transition. The intensity of this band increased together with the pH at the same salt concentration, except at 3% NaCl. This outcome may be due to changes in the protein conformation due to myosin dissociation.[Citation27]
The characteristic band for the α-helix structure exhibits an increased intensity in gels compared with sols, showing that the sol-gel transition from squid mantle proteins promotes the formation of α-helical regions. Jang et al.[Citation28] studied pH and temperature effects and established that as temperature increased, the peak intensity associated with α-helix conformation was higher. Hence, the conformational changes observed in this study could be a result of the effects of pH and NaCl as well as of the thermal treatment applied involved in protein unfolding.
Persic et al.[Citation29] studied the effects of the salt concentration on the protein structure and hydration properties of beef (Longissimus dorsi) and found a band at ~1631 cm–1 and a small band near 1693 cm–1 at high salt concentrations. These bands correspond to β-sheet structure aggregates; thus, high salt concentrations favored protein aggregation, which explained the decreased intensity of the band corresponding to the α-helix structures of myofibrillar proteins. In our study, upon comparing band intensities during the sol-gel transition, the band corresponding to β-sheets was found to have a greater intensity than the band corresponding to α-helices. Likewise, the band intensity characteristic of the β-sheet (1537 cm–1) tends to increase with increasing pH during the sol-gel transition, except at 3% NaCl, whereas increasing salt concentrations tend to decrease the β-sheet content. Therefore, the decrease in the α-helix content observed in this study suggests the aggregation of myofibrillar proteins. Similar results were reported by Liu et al.[Citation30] who studied the protein conformation changes from silver carp (Hypophthalmichthys molitrix) during the gel formation.
GFA
Texture changes in the gels were evaluated by the FT and TPA (); significant differences (p < 0.05) in all evaluated parameters were found. Although the FT is subjective, it showed a good relationship with the TPA. According to the FT, treatment with 3% NaCl at pH 6.0 (3/6.0) produced better quality gels (AA quality). According to TPA, the same treatment (3/6.0) showed enhanced hardness, elasticity, cohesiveness, and resistance against breaking. This outcome is not in agreement with the rheological study, which showed that treatment with 3% salt at pH 7.0 (3/7.0) was optimal. The previous results could be because the study shows viscoelastic deformation as a function of the temperature; that is, the 3/7.0 treatment was more elastic from 55 to 90°C. This increased elasticity could be attributed to better interactions between the hydrophobic regions of the protein matrix because hydrophobic interactions are favored at increased temperatures.[Citation31] However, at lower temperatures (25°C, temperature at which TPA was conducted), the protein system appears to become unstable, thereby explaining why the 3/7.0 treatment was less elastic and less cohesive. Our results were consistent with those reported by Kim and Park,[Citation32] who found that the textural properties of PCs from Alaska pollock (Theraga chalcogramma) were affected by increasing NaCl concentrations, especially from 2 to 3%, because salt promotes hydrophobic interactions. Therefore, the addition of salt in PCs can cause protein aggregation prior to network formation during heat treatment. Consequently, according to the viscoelastic study, treatments with higher salt concentrations showed higher G´ values due to increased hydrophobic interactions resulting from the temperature and NaCl concentration effects.
TABLE 2 Effect of pH and NaCl concentration on folding test and TPA parameters of gels
The oscillatory dynamic measurements () showed that the main factor affecting gel elasticity was the salt concentration, with 3/7.0 being the best treatment. However, the FT and TPA showed that pH was the most important factor for obtaining higher quality gels; that is, the gels prepared at pH 6.0 were the best regardless of the NaCl concentration as these gels had the greatest hardness, elasticity and cohesiveness, with 1/6.0 being the best treatment. This outcome is interesting because we often want to explain the gel quality only through rheological studies although this method only provides information about gelation related to a temperature sweep and not to the textural characteristics of the gel formed.
Moreover, it is difficult to compare our results with other studies because of differences in conducting TPA, for which variables such as sample size and geometry (height and width, cylinder or cube) as well as other factors such as the moisture content, protein type, pH, and ionic strength influence the results. Nevertheless, our hardness values were lower than those reported by Encinas-Arzate et al.[Citation4] and Dihort-Garcia et al.,[Citation8] who prepared gels at pH 7.0 and 2.5% NaCl from PCs from jumbo squid (Dosidicus gigas; size and geometry identical to this study). However, the previous result was due to those researchers being able to obtain a protein concentrate with more protein (11.5%) than in our study.
WHC
shows the WHC of gels made from jumbo squid PC at different concentrations of NaCl (1 to 3%) and pH (6.0 and 7.0). All treatments showed good WHC (over 95% in all cases), indicating that the problem of squid mantle protein gelation lies in the protein-protein interactions and not in the protein-water interactions. Therefore, a three-dimensional network was formed; however, the network formed was easily deformed upon applying a deformation force, producing weak gels compared with those of other aquatic species.
CONCLUSIONS
The application of one wash cycle to recover myofibrillar proteins from jumbo squid resulted in improved gelation performance. Both pH and NaCl concentration caused conformational changes in the protein matrix, which affected gelation and thus, the characteristics of the obtained gel. According to rheological analysis, squid protein gelation was most strongly affected by the ionic strength because a more elastic gel was obtained when the ionic strength was increased. However, according to the texture analysis, gel characteristics were largely influenced by pH because a lower pH produced gels with increased hardness, elasticity, cohesiveness, and resistance against breaking.
REFERENCES
- Marmon, S.K.; Undeland, I. Protein Isolation from Gutted Herring (Clupea harengus) Using pH-Shift Processes. Journal of Agricultural and Food Chemistry 2010, 58(19), 10480–10486.
- Félix-Armenta, A.; Ramirez-Suarez, J.C.; Pacheco-Aguilar, R.; Díaz-Cinco, M.E.; Cumplido-Barbeitia, G.; Carvallo-Ruiz, G. Jumbo Squid (Dosidicus gigas) Mantle Muscle Gelled-emulsified Type Product: Formulation, Processing and Physicochemical Characteristics. International Journal of Food Science & Technology 2009, 44, 1517–1524.
- Sánchez-Alonso, I.; Careche, M.; Borderías, A.J. Method for Producing a Functional Protein Concentrate from Giant Squid (Dosidicus gigas) Muscle. Food Chemistry 2007, 100, 48–54.
- Encinas-Arzate, J.J.; Ezquerra-Brauer, J.M.; Ocano-Higuera, V.M.; Ramirez-Wong, B.; Armenta-Villegas, L.; Torres-Arreola, W.; Marquez-Rios, E. Effect of Ionic Strength on Soluble Protein Removal from Giant Squid Mantle (Dosidicus gigas) and Functional Evaluation of Protein Recovery. Food Science and Biotechnology 2014, 23(2), 401–407.
- Gómez-Guillén, M.C.; Montero, P. Improvement of Giant Squid (Dosidicus gigas) Muscle Gelation by Using Gelling Ingredients. Zeitschrift fur Lebensmittel-Untersuchung und-Forxhung A 1997, 204, 379–384.
- Gómez-Guillén, M.C.; Montero, P.; Solas, M.T.; Borderías, A.J. Thermally Induced Aggregation of Giant Squid (Dosidicus gigas) Mantle Proteins. Physicochemical Contribution of Added Ingredients. Journal of Agricultural and Food Chemistry 1998, 46(9), 3440–3446.
- Cortés-Ruiz, J.; Pacheco-Aguilar, R.; Lugo-Sánchez, M.; Carvallo-Ruiz, G.; García-Sánchez, G. Production and Functional Evaluation of a Protein Concentrate from Giant Squid (Dosidicus gigas) by Acid Dissolution and Isoelectric Precipitation. Food Chemistry 2008, 110, 486–492.
- Dihort-Garcia, G.; Ocano-Higuera, V.M.; Ezquerra-Brauer, J.M.; Lugo-Sanchez, M.E.; Pacheco-Aguilar, R.; Barrales-Heredia, S.M.; Marquez-Rios, E. Production and Functional Evaluation of a Protein Concentrate from Giant Squid (Dosidicus gigas) Obtained by Alkaline Disolution. CyTA-Journal of Food 2012, 9, 171–179.
- Moreno-Martínez, A.G.; Ramírez-Olivas, R.; Marina-Ezquerra, J.M.; Ocano-Higuera, V.M.; Cárdenas-López, J.L. A Review of Jumbo Squid (Dosidicus gigas) Enzymes with Emphasis in Hydrolases. Biotecnia 2012, XIV(2), 18–25.
- Campo-Deaño, L.; Tovar, C.A.; Jesús Pombo, M.; Teresa Solas, M.; Borderías, J. Rheological Study of Giant Squid Surimi (Dosidicus gigas) Made by Two Methods with Different Cryoprotectants Added. Journal of Food Engineering 2009, 94, 26–33.
- Paredi, M.E.; Tomas, M.C.; Crupkin, M. Thermal Denaturation of Myofibrillar Proteins of Striated and Smooth Adductor Muscles of Scallop (Zygochlamys patagonica). A Differential Scanning Calorimetric Study. Journal of Agricultural and Food Chemistry 2002, 50(4), 830–834.
- Patrick, P.S.; Swaisgood H.E. Sulfhydryl and Disulfide Groups in Skim Milk as Affected by Direct Ultra-High-Temperatures Heating and Subsequent Storage. Journal of Dairy Science 1976, 59, 594–600.
- Tolano-Villaverde, I.J.; Ezquerra-Brauer, J.M.; Ocaño-Higuera, V.M.; Ramírez-Wong, B.; Armenta-Villegas, L.; Herrera-Urbina, R.; Magaña-Barajas, E.; Márquez-Ríos, E. A Jumbo Squid (Dosidicus gigas) Protein Concentrate Obtained by Alkaline Dissolution and Its Conformational Changes Evaluation. Food Science and Technology Research 2013, 19(4), 601–608.
- Tanikawa, E.; Motohiro, T.; Akiba, M. Marine Products in Japan; Koseicha Koseicha Co. Ltd: Tokyo, Japan, 1985; 506 p.
- Cheng, C.S.; Haman, D.D.; Webb, N.B. Effects of Species and Storage Time on Minced Fish Gel Texture. Journal of Food Science 1979, 44, 1087–1092.
- Siegler, G.R.; Acton, J.C. Heat-Induced Transition in the Protein-Protein Interaction of Bovine Natural Actomyosion. Journal of Food Biochemistry 1984, 8, 25–38.
- Xiong, Y.L. Structure-Function Relationships of Muscle Proteins. In Food Proteins and their Applications; Damodaran, S.; Paraf, A.; Eds.; Marcel Dekker Inc.: New York, NY, 1997; 341–392 pp.
- Egelandsdal, B.; Fretheim, K.; Harbitz, O. Dynamic Rheological Measurements on Heat-Induced Myosin Gels: An Evaluation of the Methods Stability for the Filamentous Gels. Journal of the Science of Food and Agriculture 1986, 37, 944–954.
- Xiong, Y.L.; Blanchard, S.P. Myofibrillar Protein Gelation: Viscoelastic Changes Related to Heating Procedures. Journal of Food Science 1994, 59(4), 734–738.
- Ramírez-Olivas, R.; Rouzaud-Sánchez, O.; Haard, N.; Pacheco-Aguilar, R.; Ezquerra-Brauer, J.M. Changes in Firmness and Thermal Behavior of Ice-Stored Muscle of Jumbo Squid (Dosidicus gigas). European Food Research and Technology 2004, 219, 312–315.
- Gill, T.; Conway, J.; Evrovski, J. Changes in Fish Muscle Proteins at High and Low Temperature. In Advances in Seafood Biochemistry. Composition and Quality; Flick, G.J.; Martin, R.E.; Eds.; Technomic Publishing: Lancaster, PA, 1992; 213–231 pp.
- Riebroy, S.; Benjakula, S.; Visessanguanb, W.; Eriksonc, U.; Rustadd, T. Acid-Induced Gelation of Natural Actomyosin from Atlantic Cod (Gadus morhua) and Burbot (Lota lota). Food Hydrocolloids 2009, 23, 26–39.
- Yongsawatdigul, J.; Park, J.W. Effects of Alkali and Acid Solubilization on Gelation Characteristics of Rockfish Muscle Proteins. Journal of Food Science 2004, 69(7), 499–505.
- Ingadottir, B.; Kristinsson, H.G. Gelation of Protein Isolates Extracted from Tilapia Light Muscle by pH Shift Processing. Food Chemistry 2010, 118, 789–798.
- Kong, J.; Yu, S. Fourier Transform Infrared Spectroscopic Analysis of Protein Secondary Structures. Acta Biochimica et Biophysica Sinica 2007, 39(8), 549–559.
- Venugopal, V. Functionality and Potential Applications of Thermostable Water Dispersions of Fish Meat. Trends Foods Science and Technology 1997, 8(8), 271–276.
- Weeds, A.G.; Pope, P. Studies on the Chymotryptic Digestion of Myosin. Effects of Divalent Cations on Proteolytic Susceptibility. Journal of Molecular Biology 1977, 111, 129–157.
- Jang, J.H.; Choi, Y.M.; Choi, Y.Y.; Joo, M.K.; Park, M.H.; Choi, B.G.; Kang, E.Y.; Jeong, B. pH/Temperature Sensitive Chitosan-G-(PA-PEG) Aqueous Solutions as New Thermogelling Systems. Journal of Materials Chemistry 2011, 21, 5484–5491.
- Perisic, N.; Afseth, N.K.; Ofstad, R.; Kohler, A. Monitoring Protein Structural Changes and Hydration in Bovine Meat Tissue Due to Salt Substitutes by Fourier Transform Infrared (FTIR) Microspectroscopy. Journal of Agricultural and Food Chemistry 2011, 59, 10052–10061.
- Liu, H.; Gao, L.; Ren, Y.; Zhao, Q. Chemical Interactions and Protein Conformation Changes During Silver Carp (Hypophthalmichthys Molitrix) Surimi Gel Formation. International Journal of Food Properties 2014, 17(8), 1702–1713.
- Liu, H.; Nie, Y.; Chen, H. Effect of Different Starches on Colors and Textural Properties of Surimi-Starch Gels. International Journal of Food Properties 2014, 17(7), 1439–1448.
- Kim, Y.S.; Park, J.W. Negative Roles of Salt in Gelation Properties of Fish Protein Isolate. Journal of Food Science 2008, 73(8), C585–C588.