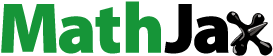
Abstract
The optimum extraction conditions of phenolic compounds from ginger were evaluated with respect to antioxidant activity and angiotensin converting enzyme and α-glucosidase inhibitory activities. Free phenolics were extracted under conditions that varied according to extraction time, temperature, and solvent type (water, acetone, and methanol). Acid and base hydrolysis reactions were used to obtain bound phenolic compounds from ginger. The results showed that the type of solvent used and the temperature and time of extraction needed for maximal total phenolic content, antioxidant activity, and angiotensin converting enzyme inhibitory activity differed greatly from solvent conditions and showed the greatest α-glucosidase inhibitory activity. The predominant free phenolics in the methanol extracts included diosmin, thymol, and carvacrol, which varied greatly according to solvent extraction conditions (i.e., time and temperature). Diosmin was the predominant bound phenolic compound of the methanol extracts. The present study findings indicate that differing solvent extraction protocols involving extraction time and temperature for ginger need to be explored to generate specific optimal bioactivities of the extracts, which are related to the pattern of predominant phenolics in those extracts.
Introduction
The Mediterranean area, including Jordan, Turkey, Greece, and Palestine, is well known for its biodiversity of wild medicinal plants such as ginger, thyme, spearmint, and rosemary.[Citation1–Citation5] Novel bioactive compounds with pharmaceutical and nutraceutical properties have been identified from such plants.[Citation1–Citation8] There is great ongoing research interest in identifying bioactive molecules from medicinal plants and investigating their pharmaceutical and nutraceutical properties due to the rising incidence of chronic diseases such as coronary vascular diseases and type 2 diabetes.[Citation9] In that regard, health benefits have been associated with the consumption of volatile oils and phenolic compounds from wild medicinal plants.[Citation8–Citation10] Phenolic compounds possess antioxidant properties that protect against free radical-generated oxidation by-products.[Citation11]
Ginger (Zingiber officinale) is a medicinal plant that has been widely used by Chinese, Ayurvedic, and Tibb-Unani populations.[Citation12–Citation16] Rhizome ginger belongs to the family Zingiberaceae, and it is mainly grown in East Asia in areas such as Taiwan, Malaysia, India, and Bangladesh, as well as in Jamaica and Nigeria.[Citation12] The ginger cultivar with well-known putative medicinal properties is Jamaica ginger, which has been used historically as a stimulant and for its carminative properties against dyspepsia and colic.[Citation13] Traditionally, ginger has been widely used as a spice with proposed health benefits.[Citation14–Citation16] Ginger has been indicated to exhibit antioxidant, anti-inflammatory, anti-ulcer, hypolipidemic, and hypoglycemic properties.[Citation13,Citation17–Citation20] Approximately 3% of ginger oil is composed of the primary bioactive compounds zingiberene, sesquiterpenoids, and β-sesquiphellandrene.[Citation21] The aim of this study was to identify the impact of extraction solvent type, extraction time, and temperature on the extraction of phenolic compounds from ginger, as well as to assess of the antioxidant and angiotensin converting enzyme (ACE) and α-glucosidase inhibitory activities of these extracts.
Materials and methods
Sample Preparation
The samples were prepared according to the method described by Alu’datt[Citation22] with some modifications. The medicinal plant (Zingiber officinale Z.) was harvested during the maturation season of 2009. Zingiber officinale Z. was washed to remove soil residues followed by freeze drying at –55ºC and 10 mTorr for 48 h. The dried plants were ground and stored in plastic bags at –18°C for further analysis.
Optimization of Extraction Conditions
The phenolic compounds were extracted based on the procedure described by Preva-Uzunalic et al.[Citation23] with modifications. The optimization of phenolic extraction was evaluated via consideration of the extraction parameters of temperature, time, and solvent type. One gram of ginger was subjected to various extraction conditions and extraction times (1, 3, 6, and 8 h) using 25 mL of different extraction solvents (methanol [ME], acetone [AC], distilled water [DW]) and solvent combinations (1:1 or 1:1:1 v/v) at different extraction temperatures (40, 50, and 60ºC). Each extract was filtered into 25 mL volumetric flasks using Whatman No.1 filter paper. The extract volume was brought to the final mark of the volumetric flask (25 mL) and stored at –18ºC for further measurement.
Extraction of Free Phenolic Compounds
Total free phenolic content was measured according to the method described by Alu’datt et al.[Citation22] One gram of ginger was mixed with 25 mL of ME for 1 h at 30°C in a water bath followed by centrifugation (10,000 × g, 10 min) to generate the supernatant containing free phenolic compounds. To obtain free phenolic compounds using ME extraction at 50°C, the residue remaining from the above ME extraction was mixed with 25 mL of ME for 1 h at 50°C in a water bath followed by centrifugation (10,000 × g, 10 min). The supernatants from all of the above methanolic extractions were filtered using cheesecloth and stored at –18°C for further analysis. The residue from the ME extraction at 50°C was kept for further extraction of bound phenolics.
Extraction of Bound Phenolic Compounds
The total content of bound phenolic compounds was measured according to the method of Alu’datt et al.[Citation22] The residue from the ME extraction at 50°C as described above was subjected to either acid or base hydrolysis to liberate the bound phenolic compounds. Base hydrolysis consisted of using 25 mL of pH 12.0 (0.1 M NaOH) at 35°C for 12 h in a shaking water bath, whereas acid hydrolysis involved using 25 mL of HCl of pH 2.0 (0.1 M) at 30°C for 12 h in shaking water bath. The liberated phenolic compounds from the base and acid hydrolysis reactions were extracted with 25 mL ME at 30°C for 1 h in a water bath and then centrifuged (10,000 × g, 10 min). The supernatants were filtered through cheesecloth and stored at –18°C for further analysis.
Phenolic Content Measurement
The Folin–Ciocalteu spectrophotometric method was used to measure the phenolic content of each extract.[Citation22] One milliliter of phenolic extract was diluted in 50 mL of DW and 1 mL of diluted phenolic extract was mixed with 7.5 mL of DW and 0.5 mL of Folin–Ciocalteu. The mixture was left for 4 min, and then 1 mL of 5% sodium carbonate (Na2CO3) was added to neutralize the reaction. The green color was developed after 1 h and was measured at a wavelength of 725 nm using a spectrophotometer to determine the phenolic content in each extract. The content of phenolic compounds in each extract was expressed as milligram of gallic acid equivalents per gram of dry matter (mg of GAE/g).
Antioxidant Activity
Antioxidant activity of the phenolic extracts was determined according to Alu’datt et al.[Citation22] Βeta-carotene stock solution was prepared by mixing of 5 mg in 50 mL chloroform. A 3 mL aliquot of the β-carotene stock solution was mixed with 50 µL of linoleic acid and 400 mg Tween 20. Chloroform was evaporated using nitrogen, and the residue was mixed with 100 mL of DW followed by vortexing to mix with oxygen. The β-carotene/linoleic acid emulsion was mixed with 100 µL extract solution at 50ºC for 60 min in a water bath. The antioxidant activity was evaluated by measuring the color absorbance at 470 nm over 60 min using a spectrophotometer. The antioxidant activity was expressed using the following Equation (1):
ACE Inhibitory Activity
ACE inhibition was determined using the method previously described by Cushman and Cheung[Citation24] with some modifications. Hippuryl-L-histidyl-L-leucine (HHL; Sigma Chemical Co.) solution was dissolved in 50 mM HEPES-HCl buffer (Sigma Chemical Co.) containing 300 mM NaCl (pH 8.3). A total of 200 µL of HHL solution (0.3% w/v) was mixed with 80 µL of phenolic extracts. 0.33 U of ACE enzymes (Sigma Chemical Co.) were dissolved in 1 mL of DW. Fifty microliters of diluted ACE was mixed to initiate the reaction followed by incubation for 15 min at 37ºC. The reaction was stopped by addition of 250 µL of HCl (1 M). The hippuric acid liberated from the reaction was removed from the solution by using 2 mL of ethyl acetate. One milliliter of ethyl acetate layer was separated by centrifugation and evaporated by heating in boiling water for 15 min followed by addition of 3 mL of DW. The absorbance of the sample was measured by UV spectrophotometry at 228 nm. The blank was prepared by adding 250 µL HCl (1 M) before incubation. The amount of hippuric acid liberated from the reaction in the absence of inhibitor was defined as 100% ACE activity. The ACE activity and ACE inhibitory activity were calculated by the following equations:
Inhibition of α-Glucosidase Activity
α-Glucosidase inhibition was determined using the colorimetric method described by McCue et al.[Citation25] with modifications. The α-glucosidase solution was prepared by mixing 1 mg of α-glucosidase (Sigma Chemical Co.) dissolved in 5.7 mL of phosphate buffer (pH 6.9). Five mM 4-nitrophenyl α-D-glucopyranoside (Sigma Chemical Co.; 0.151 g) was dissolved in 100 mL phosphate buffer (PH 6.9) in volumetric flask. Fifteen microliters of sample extract was mixed with 100 µL of α-glucosidase solution and left for 10 min at room temperature. Thereafter, 50 µL of 4-nitrophenyl α-D-glucopyranoside was added and absorbance measured at 405 nm using a spectrophotometer (Dynatech, MR5000). The extract was replaced with extraction solvent for the control samples. The mixture was incubated for 5 min and absorbance was read at the same wavelength against the control. Percentage of inhibition of α-glucosidase was calculated using the following Equation (6):
RP-HPLC Analysis of Individual Phenolic Compounds
Individual phenolic compounds were separated and evaluated according to the method described by Alu’datt[Citation22] with some modifications. Phenolic extracts from ginger were evaporated under a stream of nitrogen and then dissolved in 1 mL of ME. The sample was mixed with ME, centrifuged (5000 × g, 10 min), flushed under a stream of nitrogen and stored at –18ºC for HPLC analysis. The supernatant was filtered through 0.45 µm MilliporeTM membrane filters prior to RP-HPLC analysis. HPLC analysis was carried out using the Agilent 1100 series HPLC system (Agilent Technologies, Waldbronn, Germany) for liquid chromatography equipped with a quaternary gradient pumping system (Agilent, G1312) for high-pressure solvent delivery, an automatic injector (Agilent, G1313A, Agilent Technologies) with a 100 μL loop and a variable wavelength UV-detector (Agilent, G1314A). Spectral and chromatographic data were analyzed using Agilent ChemStation software. For chromatographic separation, 100 μL of sample was injected into a C18 reversed phase column (5 micron, 250 × 4.6 mm, Varian, US). Elution was carried out at a flow rate of 0.75 mL/min with the following two-buffer gradient system: solvent A, 0.2% triflouroacetic acid (TFA) in water (v/v) and solvent B, 100% ME, with a linear gradient starting at 5–95% ME within 60 min, initial conditions were then re-established over 10 min. The UV detector was calibrated and the eluate was monitored at 280 nm at 1 s intervals. The stock solution of (20 µL) was prepared at 1 μg/mL in ME. The standards were purchased from Sigma Chemical Company (St. Louis, MO, USA).
Statistical Analyses
All experiments were performed at least in duplicate. Statistical analyses were performed using factorial analysis with the generalized linear model (GLM) procedure and correlation (R2) analysis with the SAS program version 8.2 (SAS 2002 Institute Inc., Cary, NC, USA). Statistical significance was accepted at a level of p ≤ 0.05.
Results and discussion
Effect of Extraction Solvent on Total Phenolic Content
shows the effect of different extraction solvents on phenolic content. Generally, the phenolic content was affected greatly by the type of extraction solvent. Overall, AC was the most efficient solvent for phenolic extraction from ginger, followed by ME. Marja et al.[Citation26] reported that either the mixture of 70% AC or 80% ME in water extracted the highest amount of phenolic compounds in selected medicinal plants. Badami et al.[Citation27] found that the phenolic content extracted from ginger was 3.35 mg/g using water extraction, which is similar to our findings with DW extraction (). Jensen et al.[Citation28] found that the extraction temperature, time and ratio of solvent affected the content of extracted phenolic compounds from red grapes. Tlili et al.[Citation29] reported that the content of phenolic compounds in the ME extract from Thymus vulgaris was 11.80 mg/g, whereas ginger methanolic extract had a phenolic content of 8.71 mg/g in the present study.
Table 1 Effect of extraction solvents on content (mg/g), antioxidant activity (%) ACE inhibitory activity (%), and α-glucosidase inhibitory activity (%) of phenolic compounds at 25°C/1 h for ginger*
Effect of Extraction Time and Temperature on Total Phenolic Content
indicates the effect of time and temperature of extraction on the content of phenolic compounds using ME extraction. The content of phenolic compounds extracted from ginger generally showed no significant variations with temperature and time of extraction; however, the highest total phenolic content was observed with extraction at 60ºC for 1 h. Likewise, the optimum temperature for extraction of phenolic compounds has previously been noted to be 60ºC in olive cake[Citation23] and rice.[Citation30] Hodzic et al.[Citation31] has reported that raising the temperature of extraction increases the extractability of phenolic compounds from rice, rye, oats, buckwheat, barley, wheat, and corn. Onofre and Hettiarachchy[Citation30] showed that the phenolic yield from rice bran increased with a longer time of extraction at 95°C. As the time of extraction increased from 0.5 to 1 h, the content of extracted phenolic compounds was shown to increase in rice bran.[Citation30] Jensen et al.[Citation28] also found that the extraction temperature and time increased the total content of phenolic compounds in red grapes. In the present study, however, an increase in extraction time ranging from 1 to 8 h at all the tested temperatures was not associated with an increase in extraction of phenolics from ginger.
Table 2 Content (mg/g), antioxidant activity (%) ACE inhibitory activity (%) and α-glucosidase inhibitory activity (%) of phenolic compounds for methanolic extraction using different time and temperature of extractions from ginger*
Table 3a Phenolic content (mg/g), antioxidant activity (%), ACE-inhibitory activity (%), and α-glucosidase inhibitory activity (%) of extracted free and bound phenolic compounds from ginger*
Effect of Extraction Solvents on Antioxidant Activity, Ace Inhibitory Activity, and α-Glucosidase Inhibition
demonstrates the effect of solvent extraction using ME, AC, DW, and mixtures of these solvents on the antioxidant activity, ACE inhibitory activity, and α-glucosidase inhibitory activity of the phenolic compounds extracted from ginger. The results revealed that the solvent extraction associated with the highest antioxidant activity was found with the combination of ME, AC, and water solvents (1:1:1), as this combination was more effective than the extractions performed with DW, AC, or ME alone. Likewise, a 1:1 combination of DW with either AC or ME was more effective than either AC or ME alone. Both AC and ME extracts showed higher antioxidant activities compared to DW extracts. Badami et al.,[Citation27] studying Zingiber officinale Z., reported that the minimum antioxidant activity among medicinal extracts was obtained using a water extract with a value of 15.64%. Hernandez et al.[Citation32] found that the antioxidant activity of Rosmarinus officinalis L. extracts was higher compared to other medicinal plants. Arumugam et al.[Citation33] reported that the antioxidant activities of hexane, chloroform, ethyl acetate, and water extracts from Mentha spicata L. had values ranging from 8 to 95%. Similarly, Haddad and Dezashibi[Citation34] found that the ME extraction of henna leaves gave a higher antioxidant activity compared with water extraction. The combination of AC and ME, however, lowered antioxidant activity relative to the single solvent extractions. The optimum activity of ACE inhibition of phenolic compounds in ginger was obtained in an extraction mixture of ME, AC, and water, which is similar to the observations on antioxidant activity. As observed with antioxidant activity, the combination of water with either AC or ME showed more effective ACE inhibitory activity than either AC or ME alone. In contrast to the antioxidant activity results, AC and ME extracts had lower ACE inhibitory activity than DW extracts, but the AC and ME combination increased ACE inhibitory activity. The antihypertensive properties were obtained in water and ME extracts of Gynura procumbens.[Citation35] Pinto et al.[Citation36] reported that a water extract of Brazilian strawberry inhibited the activity of ACE. As opposed to antioxidant and ACE-inhibitory activities, the highest α-glucosidase inhibitory activities were obtained via the mixture of AC and water extraction. As opposed to the greatly increased α-glucosidase inhibitory activities shown by the addition of DW with AC, the combination of DW with ME lowered α-glucosidase inhibitory activities relative to ME alone. The water extract of Azadirachta indica and ME extract of potatoes (Solanum tuberosum L.) showed inhibitory effects for α-amylase enzyme.[Citation37,Citation38] McCue et al.[Citation39] reported relatively low inhibitory activities of α-glucosidase in water extracts of soybean over a range of 1.00 to 1.20%. A similar degree of inhibition of α-glucosidase activity (82.3%) was observed in the present study with the ME extract of ginger as with methanolic extracts of Eucalyptus, which gave a dose dependent range of inhibitory activities of α-glucosidase with a range of values from 20.00 to 93.00%.[Citation40] Similar to the relatively low antioxidant activities shown with DW extracts, the lowest value of inhibitory activity for α-glucosidase was observed with the water extraction of ginger. Overall, the present findings indicate major solvent dependent differences in bioactivities among the ginger extracts, as the different solvent extraction protocols were optimal for different bioactivities.
Effect of Extraction Time and Temperature of Phenolic Compounds on Antioxidant Activity
shows the effect of extraction time and temperature for phenolic compounds on the antioxidant activity, ACE-inhibitory activity, and α-glucosidase inhibitory activity of ginger. Generally, the antioxidant activities of phenolic compounds in ginger showed no consistent variations as the temperature and time of extraction gradually increased, although ME extraction at 60ºC had higher antioxidant activity at 3 h relative to 40ºC. Likewise, higher antioxidant activity was noted at 50ºC than 40ºC after 6 h methanolic extraction. As opposed to antioxidant activity, both temperature, and time were associated with major variations in the ACE inhibitory and α-glucosidase inhibitory activities of the extracted phenolic compounds. Higher ACE inhibitory activity was associated with 60ºC than 40ºC extraction after 1 and 3 h. Several studies have demonstrated that extraction of phenolic compounds at high temperature for a shorter time provides a higher ACE inhibitory activity due to an increase in the solubility and rate of diffusion of phenolic compounds. Conversely, the maximum value of ACE inhibitory activity with ME extraction was observed at 40ºC for 6 h. Both 40 and 60ºC were associated with a major drop in ACE inhibitory activity at 8 h. The latter observations are in concert with studies showing the use of higher temperatures for a more prolonged time may denature the chemical structure of biologically active compounds.[Citation30] The present findings show relatively higher ACE inhibitory effects than those previously noted with ethanolic extracts of wild plants. The highest value of ACE inhibitory activity in Rhodiola species was extracted using ethanol at 40°C for 3 h with a value of 38.5%, while the lower values of ACE inhibitory activities were extracted in ethanol extracts of oregano, lemon balm, and Melissa officinalis with 18.5, 0.5, and 0.5%, respectively.[Citation41]
The highest values of α-glucosidase inhibitory activity were obtained in extractions of phenolic compounds at 40ºC for 3 h from Zingiber officinale Z. with a value of 73.70%, which showed a significant drop in activity at 8 h. In contrast, the lowest value of inhibitory activity for α-glucosidase was 22.91% observed at 50°C after 1 h extraction that increased significantly after a 3 h extraction. The above α-glucosidase inhibitory activities are within the range of previous observations with ethanol extracts of other wild plant species. For example, Kwon et al.[Citation41] reported that the α-glucosidase inhibitory activity of ethanol extracts from oregano and lemon balm at 40°C for 3 h were 80 and 75%, respectively. The α-glucosidase inhibitory activity of ethanol extracts from Saliva species at 40°C ranged from 52.24 to 24.95%.[Citation42]
Content and Biological Properties of Free and Bound Phenolic Compounds
demonstrates the phenolic content and bioactivities of extracted free and bound phenolic compounds from ginger using: (a) ME extract obtained at 30°C (FP-30°C extract); (b) ME extract generated at 50ºC (FP-50°C extract); (c) base hydrolysis followed by ME extraction (BP-base extract); and (d) acid hydrolysis followed by ME extraction (BP-acid extract). The total content of free phenolic compounds was higher in the FP-30°C extract versus the FP-50°C extract, which was associated with significantly higher antioxidant and α-glucosidase inhibitory activity. In contrast, ACE inhibitory activity was associated with the lower free phenolic content observed in the FP-50°C extract. Similar associations were observed with the bound phenolics as the BP-base extract had markedly higher bound phenolic content than the BP-acid extract along with greater α-glucosidase inhibitory activity, although antioxidant activity did not vary. As observed with the free phenolic acid extracts, higher ACE inhibitory activity was associated with lower total phenolic content of the BP-base extract. The above results indicate that higher total phenolic content with various extractions does not necessarily relate to an increase in bioactivity. It is conceivable that more selective extractions of phenolic compounds with certain solvent extraction conditions could lead to higher bioactivities regardless of lower total phenolic content as observed with the above ACE inhibitory activities. shows the correlation coefficients between phenolic content and the tested bioactivities associated with the extracted phenolic compounds from ginger. Antioxidant activity was strongly positively correlated with the content of extracted phenolic compounds in ginger, whereas ACE inhibitory activity of phenolic compounds was negatively correlated with antioxidant activity in Zingiber officinale with value of –0.85. Previous studies have shown plant food and wild plant variations in terms of the relationship between phenolic content and bioactivities. Karadenuz et al.[Citation43] found positive correlations between antioxidant activity and content of phenolic compounds in potato, onion, spring onion, red radish, and red cabbage; however, no correlation between phenolic acid content and antioxidant activity was obtained in strawberries.[Citation36] In the present work, a negative correlation was observed between antioxidant and ACE inhibitory activity, while a positive relationship was observed between the ACE inhibitory and α-glucosidase inhibitory activities. Ahmed et al.[Citation44] found a strong positive correlation (0.893) between antioxidant activity and ACE inhibitory activity in stem bark of Ficus racemosa. Nho et al.[Citation45] reported that the correlation between total phenols and ACE inhibitory activity in Boehmeria nivea was 0.689. A strong positive correlation between total content of phenolic compounds and ACE inhibitory activity was obtained in Thymus vulgaris and Mentha spicata with values of 0.71 and 0.27, respectively. On the other hand, Nho et al.[Citation45] found strong negative correlations between antioxidant activity and ACE inhibitory activity in Boehmeria nivea extracts, similar to those observed in our present study.
Table 3b Correlation coefficients (r) between total phenolic content, antioxidant activity, ACE inhibitory activity, and α-glucosidase inhibitory activity of extracted phenolic compounds from ginger
Effect of Extraction Solvent, Time, Temperature, Acid, and Base on Contents of Individual Phenolics by RP-HPLC
and illustrate that the concentrations of major phenolics were altered depending on extraction conditions including solvent type, time, and temperature. Carvacrol was the predominant phenolic compound detected in extractions involving AC, DW, a 1:1 combination of ME and DW, and the combination of ME, AC, and DW solvents (1:1:1; ). In contrast, carvacrol was not detectable in the ME extract and diosmin was the major predominant phenolic compound. Thymol was predominant for the 1:1 combination of ME and AC, as well as for the 1:1 AC and DW combination (). Although diosmin was predominant in ME extracts obtained from extractions at 40 and 60°C for 1 h, the predominant phenolic compound from ME extraction at 50°C for 1 h was kaempferol. A more prolonged extraction for 3 h, however, was associated with major increases in thymol in extracts generated at all temperatures of 40, 50, and 60°C, followed by subsequent decreased thymol concentrations at 6 and 8 h. The profile of the major phenolic in the ME extracts was altered after the 6 h extraction as diosmin was once again predominant at all temperatures of extraction (). At 8 h of extraction, diosmin was also the predominant phenolic in methanolic extracts generated at temperatures of 40 and 50°C, whereas carvacrol was the major phenolic observed at 60°C (). RP-HPLC profiles showed that the major free predominant phenolic compound was diosmin in the FP-30°C extract and carvacrol in the FP-50°C extract. No phenolic compound predominated in the BP-base extract as relatively low concentrations of several phenolics were observed, whereas diosmin was the major phenolic component in the BP-acid (). In light of the above, it is likely that the varying bioactivities observed among different types of extracts could be explained by the patterns in the appearance of predominant phenolics that are dependent on solvent type and the extraction conditions of time and temperature.
Table 4 Effect of extraction solvent on individual phenolic compounds contents obtained using RP-HPLC technique from ginger
Table 5 Effect combination of extraction time and temperature on the contents of phenolic compounds obtained using RP-HPLC technique from ginger (%)*
Table 6 Content of free and bound phenolic compound obtained using RP-HPLC technique from ginger (%)
Conclusions
The present study has shown that optimum extraction conditions in ginger vary greatly in terms of maximum phenolic content, antioxidant activity, ACE inhibitory activity, and α-glucosidase inhibitory activity. In general, extraction conditions of the solvent used and the temperature and time of extraction needed for maximal antioxidant and ACE inhibitory activity differed substantially from the solvent conditions associated with the highest α-glucosidase inhibitory activity. In that regard, negative correlations were observed between antioxidant activity and ACE inhibitory activity. Thus, differing solvent extraction protocols involving extraction time and temperature for ginger are needed to generate specific optimal bioactivities of these extracts. In support of these observations, the major phenolics diosmin, thymol, and carvacrol in the ME extracts showed a varying pattern of appearance that was dependent on temperature and time of extraction. Further studies are needed to determine the specific functionalities of the major phenolics toward the antioxidant, ACE inhibitory and α-glucosidase inhibitory activities of the ginger extracts.
Acknowledgments
The authors would like to extend their sincere appreciation to the Deanship of Scientific Research at Jordan University of Science and Technology and Deanship of Scientific Research at King Saud University for its funding of this research through Research Group Project number 193.
References
- Nawash, O.; Shudiefat, M.; Al-Tabini, R.; Al-Khalidi, K. Ethnobotanical Study of Medicinal Plants Commonly Used by Local Bedouins in the Badia Region of Jordan. Journal of Ethnopharmacology 2013, 148, 921–925.
- Alzweiri, M.; Al Sarhan, A.; Mansi, K.; Hudaib, M.; Aburjai, T. Ethnopharmacological Survey of Medicinal Herbs in Jordan, the Northern Badia Region. Journal of Ethnopharmacology 2011, 137(1), 27–35.
- Kaval, I.; Behçet, L.; Cakilcioglu, U. Ethnobotanical Study on Medicinal Plants in Geçitli and Its Surrounding (Hakkari-Turkey). Journal of Ethnopharmacology 2014, 155(1), 171–184.
- Mukemre, M.; Behçet, L.; Çakılcıoglu, U. Ethnobotanical Study on Medicinal Plants in Villages of Çatak (Van-Turkey). Journal of Ethnopharmacology 2015, 166(26), 361–374.
- Pirbalouti, A.G.; Neshat, S.H.; Rahimi, E.; Hamedi, B.; Malekpoor, F. Chemical Composition and Antibacterial Activity of Essential Oils of Iranian Herbs Against Staphylococcus Aureus Isolated from Milk. International Journal of Food Properties 2014, 17, 2063–2071.
- Kingston, D.G.I. Natural Products As Pharmaceuticals and Sources for Lead Structures. In The Practice of Medicinal Chemistry; Wermuth, C.G.; Ed.; Academic Press Harcourt Brace: London, UK, 1996; 101–116 pp.
- Bordia, A.; Verma, S.K.; Srivastava, K.C. Effect of Ginger (Zingiber Officinale) and Fenugreek (Trigonella Foenumgraecum) on Blood Lipids, Blood Sugar, and Platelet Aggregation in Patients with Coronary Artery Disease. Prostaglandins, Leukotrienes, and Essential Fatty Acids 1997, 56(5), 379–384.
- Eric, Y.; Kathy, A.; Hooper, C.G. Chronic Sinusitis. Journal of Alternative and Complementary Medicine 2003, 9(1), 39–41.
- Kalra, E.K. Nutraceutical: Definition and Introduction. The American Association of Pharmaceutical Scientists 2003, 5, 2–3.
- Han, X.; Shen, T.; Lou, H. Dietary Polyphenols and Their Biological Significance. International Journal of Molecular Sciences 2007, 8, 950–988.
- Gutteridge, J.M.C.; Halliwell, B. Antioxidants in Nutrition, Health, and Disease. Oxford University Press: New York, NY, 1994; 111–123 pp.
- Mustafa, T.; Sirivastav, K.V.; Jensen, K.B. Drug Development Report 9: Pharmacology of Ginger, Zingiber Officinale. Journal of Drug Development 1993, 6(1), 25–39.
- Ernst, E.; Pittler, M.H. Efficacy of Ginger for Nausea and Vomiting: A Systematic Review of Randomized Clinical Trials. British Journal of Anesthesia 2000, 84(3), 367–371.
- Cao, Z.F.; Chen, Z.G.; Guo, P.; Zhang, S.M.; Lian, L.X.; Luo, L.; Hu, W.M. Scavenging Effects of Ginger on Superoxide Anion and Hydroxyl Radical. Chung-Kuo Chung Yao Tsa Chih 1993, 18, 750–764.
- Krishnakantha, T.P.; Lokesh, B.R. Scavenging of Superoxide Anions by Spice Principles. Indian Journal of Traditional Knowledge 1993, 30, 133–134.
- Reddy, A.A.; Lokesh, B.R. Studies on Spice Principles As Antioxidants in the Inhibition of Lipid Peroxidation of Rat Liver Microsomes. Molecular and Cellular Biochemistry 1992, 111, 117–124.
- Al-Yahya, M.A.; Rafatullah, S.; Mossa, J.S.; Ageel, A.M. Gastroprotective Activity of Ginger Zingiber Officinale Rosc., in Albino Rats. American Journal of Chinese Medicine 1989, 17, 51–56.
- Kim, Y.M.; Jeong, Y.K.; Wang, M.H.; Lee, W.Y.; Rhee, H.I. Inhibitory Effect of Pine Extract on Alpha-Glucosidase Activity and Postprandial Hyperglycemia. Nutrition 2005, 21, 756–761.
- Yoshikawa, M.; Yamaguchi, S.; Kunimi, K.; Matsuda, H. Stomachic Principles in Ginger III. An Anti-Ulcer Principle, 6-Gingesulfonic Acid, and Three Monoacyl Digalactosyl Glycerols, Gingerglycolipids A, B, and C, from Zingiberis Rhizoma Originating in Taiwan. Chemical Pharmaceutical Bulletin 1994, 42, 1226–1230.
- Badreldin, A.H.; Blunden, G.; Tanira, M.O.; Nemmar, A. Some Phytochemical, Pharmacological and Toxicological Properties of Ginger (Zingiber Officinale Roscoe): A Review of Recent Research. Food and Chemical Toxicology 2008, 46(2), 409–420.
- Verma, S.K.; Bordia, A. Ginger, Fat, and Fibrinolysis. Indian Journal of Medical Sciences 2001, 55(2), 83–86.
- Alu’datt, M.H.; Alli, I.; Ereifej, K.; Alhamad, M; Al-Tawaha, A.R.; Rababah, T. Optimisation, Characterization, and Quantification of Phenolic Compounds in Olive Cake. Food Chemistry 2010, 123, 117–122.
- Preva-Uzunalic, A.; Skerget, M.; Knez, Z.; Weinreich, B.; Otto, F.; Gruner, S. Extraction of Active Ingredients from Green Tea (Camellia Sinensis): Extraction Efficiency of Major Catechins and Caffeine. Food Chemistry 2007, 96, 597–605.
- Cushman, D.W.; Cheung, H.S. Spectrometric Assay and Properties of Angiotensin-Converting-I-Enzyme of Rabbit Lung. Biochemical Pharmacology 1971, 20, 1637–1648.
- McCue, P.; Kwon, Y.; Shetty, K. Anti-Amylase, Anti-Glucosidase, and Antiangiotensin I-Converting Enzyme Potential of Selected Foods. Journal of Food Biotechnology 2005, 29, 278–294.
- Marja, P.K.; Anu, I.H.; Heikki, J.V.; Jussi, P.R.; Kalevi, P.; Tytti, S.K.; Marina, H. Antioxidant Activity of Plant Extracts Containing Phenolic Compounds. Journal of Agricultural and Food Chemistry 1999, 47, 3954–3962.
- Badami, S.; Sangeetha, M.; Latha, V.; Archana, N.; Suresh, B. Antioxidant Potential of Five Ksheerapaka’s and Kashaya’s, Ayurvedic Decoctions. Indian Journal of Traditional Knowledge 2007, 6(3), 423–425.
- Jensen, J.S.; Blachez, B.; Egebo, M.; Meyer, A.S. Rapid Extraction of Polyphenols from Red Grapes. American Journal of Enology and Viticulture 2007, 58(4), 451–461.
- Tlili, N.; Elfalleh, W.; Hannachi, H.; Yahia, Y.; Khaldi, A.; Ferchichi, A.; Nasri, N. Screening of Natural Antioxidants from Selected Medicinal Plants. International Journal of Food Properties 2013, 16, 1117–1126.
- Onofre, F.O.; Hettiarachchy, N.S. Extraction, Quantification, and Characterization of Phenolics Extracted with the Aid of Sonication from Rice Bran. Cereal Chemistry 2007, 84(4), 337–342.
- Hodzic, Z.; Pasali, H.; Memisevi, A.; Srabovi, M.; Saletovi, M.; Poljakovic, M. The Influence of Total Phenols Content on Antioxidant Capacity in the Whole Grain Extracts. European Journal of Scientific Research 2009, 28(3), 471–477.
- Hernandez, E.; Alquicira, E.P.; Jaramillo-Flores, M.E.; Legarreta, I.G. Antioxidant Effect Rosemary (Rosmarinus Officinalis L.) and Oregano (Origanum Vulgare L.) Extracts on TBARS and Colour of Model Raw Pork Batters. Meat Science 2009, 81(2), 410–417.
- Arumugam, P.; Ramamurthy, P.; Thyagarajan, S.S.; Ramesh, A. Antioxidant Activity Measured in Different Solvent Fractions Obtained from Mentha Spicata Linn. : An Analysis by ABTS and Decolorization Assay. Asia Pacific Journal of Clinical Nutrition 2006, 15(1), 119–124.
- Haddad, K.M.H.; Dezashibi, Z. Phenolic Compounds and Antioxidant Activity of Henna Leaves Extracts (Lawsonia Inermis). World Journal of Dairy and Food Sciences 2007, 2(1), 38–41.
- Puang-Pronpitag, D.; Chaichanadee, S.; Naowaratwattana, W.; Sittiwet, C.; Thammasarn, K.; Luerang, A.; Kaewseejan, N. Evaluation of Nutritional Value and Antioxidative Properties of the Medicinal Plant Gynura Procumbens Extract. Asian Journal of Plant Science 2010, 9, 146–151.
- Pinto, M.S.; Kwon, Y.; Apostolidis, E.; Lajolo, F.M.; Genovese, M.; Shetty, K. Functionality of Bioactive Compounds in Brazilian Strawberry (Fragaria Ananassa Duch). Cultivars: Evaluation of Hyperglycemia and Hypertension Potential Using in Vitro Models. Journal of Agricultural and Food Chemistry 2008, 56, 4386–4392.
- El-Shraiy, A.M.; Hegazi, A.M. Influence of JA and CPPU on Growth, Yield and α-Amylase Activity in Potato Plant (Solanum Tuberosum L.). Australian Journal of Basic and Applied Sciences 2010, 4(2), 160–170.
- Dineshkumar, B.; Mitra, A.; Manjunatha, M. A Comparative Study of Alpha Amylase Inhibitory Activities of Common Anti-Diabetic Plants at Kharagpur 1 Block. International Journal of Green Pharmacy 2010, 4, 115–121.
- McCue, P.; Kwon, Y.I.; Shetty, K. Anti-Diabetic and Anti-Hypertensive Potential of Sprouted and Solid-State Bioprocessed Soybean. Asia Pacific Journal of Clinical Nutrition 2005, 14(2), 145–152.
- Basak, S.S.; Candan, F. Chemical Composition and in Vitro Antioxidant and Antidiabetic Activities of Eucalyptus Camaldulensis Dehnh. Journal of the Iranian Chemical Society 2010, 7(1), 216–226.
- Kwon, Y.I.; Vattem, D.A.; Shetty, K. Evaluation of Conal Herbs of Lamiaceae Species for Management of Diabetes and Hypertension. Asia Pacific Journal of Clinical Nutrition 2006, 15(1), 107–118.
- Nickavar, B.; Abolhasani, L.; Izadpanah, H. α-Amylase Inhibitory Activities of Six Salvia Species. Iranian Journal of Pharmaceutical Research 2008, 7(4), 297–303.
- Karadenuz, F.; Burdurlu, H.S.; Koca, N.; Soyer, Y. Antioxidant Activity of Selected Fruits and Vegetables Grown in Turkey. Turkish Journal of Agriculture and Forestry 2005, 29, 297–303.
- Ahmed, F.; Siddesha, J.M.; Urooj, A.; Vishwanath, B.S. Radical Scavenging and Angiotensin Converting Enzyme Inhibitory Activities of Standardized Extracts of Ficus Racemosa Stem Bark. Phytotherapy Research 2010, 24, 1839–1843.
- Nho, J.W.; Hwang, I.G.; Kim, H.Y.; Lee, Y.R.; Woo, K.S.; Hwang, B.Y.; Chang, S.J.; Lee, J.; Jeong, H.S. Free Radical Scavenging, Angiotensin I-Converting Enzyme (ACE) Inhibitory, and in Vitro Anticancer Activities of Ramie (Boehmeria Nivea) Leaves Extracts. Food Science and Biotechnology 2010, 19(2), 383–390.