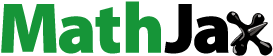
Abstract
The degradation and isomerization of all-trans-zeaxanthin in a model system during illumination, in the absence or presence of iodine, were investigated. A high-performance liquid chromatography method was developed using the C-30 column to separate all-trans-zeaxanthin and its cis-isomers. Three mono-cis-isomers and four di-cis-isomers were assigned by high-performance liquid chromatography-photo diode array detection-atmospheric pressure chemical ionization-mass spectrometry (HPLC-DAD-APCI-MS), namely, 15/15’-cis-, 13/13’-cis-, 9/9’-cis-13,15-di-cis-, 9,15-di-cis-, 9,13-di-cis-, and 9,9’-di-cis-zeaxanthin. 13/13’-cis- and 9, 13-di-cis-zeaxanthin were the major cis-isomers of zeaxanthin formed during illumination. Di-cis-zeaxanthin was favored during illumination in the presence of iodine. The degradation of total amount of all-trans-zeaxanthin during illumination was found to fit a first-order model. The degradation rate of iodine-catalyzed photoisomerization of all-trans-zeaxanthin was faster than that of non-iodine-catalyzed photoisomerization. Isomerization and degradation of all-trans-zeaxanthin and its cis-isomers during illumination proceeded simultaneously.
Introduction
Of the hundreds of carotenoids that exist in nature, only 20 are found inside the human body. Among the carotenoids present in the body, only zeaxanthin and its close relative lutein, are found in the highest concentrations in the different parts of the eye, including the lens, retina, and macula lutea.[Citation1,Citation2] Zeaxanthin, like other carotenoids, is not synthesized by the human body and must be consumed in the diet. It is naturally found in corn, egg yolks, and some of the orange and yellow vegetables and fruits, such as alfalfa and marigold flowers.[Citation3,Citation4] Numerous studies have shown that the carotenoids zeaxanthin and lutein may protect the human macula by absorbing blue light and quenching free radicals.[Citation5–Citation7] Studies in vivo have demonstrated that lutein and zeaxanthin have provided significant protection against light-induced skin damage, especially the ultraviolet wavelengths.[Citation6]
Carotenoids are highly conjugated polyene chromophores, and it is this conjugation which gives carotenoids much of their functionality. However, isomerization and oxidative degradation of carotenoids, influenced by temperature, light exposure, and other factors, are often encountered either in food processing or under improper storage conditions. The nutritional consequences of trans/cis isomerization and oxidative degradation are changes in bioavailability and physiological activity. Thus, studies on the stability of carotenoids were of great interest. Inbaraj et al.[Citation8] determined the carotenoids in Lycium barbarum fruits, and a total of 11 free carotenoids and 7 carotenoid esters were identified. Around 30 carotenoids in concentrated orange juice were detected by Melendez-Martinez et al.[Citation9] The increase of the number of carotenoids in concentrated orange juice in comparison with Lycium barbaricum may be due to the different processing way but also because of the difference of natural matrix. It was found that the activation energies for β-cryptoxanthin and the kinetic data on xanthophyll degradation were very different in various matrices.[Citation10–Citation12] These findings indicated that the stability of carotenoids is dependent on species of carotenoids and their matrix. Thus, to study the degradation and isomerization of carotenoids, a model system may overcome the matrix effect and monitor the formation of initial, intermediate, and final compounds more easily than the real system.
Zepka and Mercadante[Citation13] have studied degradation reaction of carotenoids in aqueous-based and juice systems. Isomerizaiton of trans to cis-configuration and formation of oxidation compounds during carotenoids heating were found. Thermal and oxidative degradation of carotenoids in an oil model system were reported by Henry et al.[Citation14] The major isomers formed during heating of β-carotene were 13-cis, and 9-cis. For all-trans-zeaxanthin, 9-cis-, 13-cis-, and 15-cis- were identified in the light-induced and heat-induced isomerization of all-trans-zeaxanthin in an organic solvent environment.[Citation15] 13-cis- was the major isomer among the isomerization products. In addition, di-cis-zeaxanthin has been detected from postprandial human plasma by Melendez-Martinez et al.[Citation16] This data indicated that various cis-zeaxanthins occurred during degradation and isomerization of all-trans-zeaxanthin. But little information is available relative to the chromatographic and spectroscopic characteristics of the geometrical isomers of all-trans-zeaxanthin, especially for the di-cis-isomers of zeaxanthin. Thus, the aim of this study was to separate and identify the mono-cis- and di-cis-isomers of all-trans-zeaxanthin during illumination in a model system. Meanwhile, the stability of all-trans-zeaxanthin during illumination was analyzed. The identification and analysis of all-trans-zeaxanthin and its cis-isomers would provide original data for further understanding of the zeaxanthin degradation and prediction of their losses in a real matrix.
Materials and Methods
Materials
All-trans-zeaxanthin was purchased from Sigma Co. (St. Louis, MO, USA). The high-performance liquid chromatography (HPLC)-grade solvents, including methyl-tert-butyl ether (MTBE) and methanol (MeOH), were purchased from Tedia. Co. Inc. (Fairfield, OH, USA). Ammonium acetate (HPLC-grade) was purchased from Tianjin Kemiou Chemical Reagent Co. (Tianjin, China). Iodine, hexane, anhydrous sodium sulphate, and sodium thiosulfate were obtained from Sinopharm Chemical Reagent Co., Ltd (Beijing, China).
Instrumentation
The HPLC instrument consists of a vacuum degasser, a quaternary pump, a column oven, and a photodiode-array detector (Agilent Technologies, Palo Alto, CA, USA). 6530 QTOF-MS was purchased from Agilent. The nitrogen purging instrument (D10) was from Hangzhou All Sheng Instruments Co., Ltd. (Hangzhou, China), and the digital type of illuminance meter from TES Electrical Electronic Co. (Taiwan).
Separation and Identification of All-Trans-Zeaxanthin and Its Cis-Isomers by HPLC-Mass Spectra (MS)
A stationary phase (YMC C30 column, 5 μm, 4.6 mm × 250 mm) and mobile phase solvents (MTBE and MeOH) were used for the method development. The flow rate was set at 1.0 mL/min, the column temperature was maintained at 25°C, and the volume injection was 20 μL. MS analysis was performed using a 6530 QTOF-MS. MS were detected in positive ion mode in a mass range of m/z 80–1000. The HPLC-MS separations were monitored at 450 nm. Nitrogen was used as drying gas at a flow rate of 5.0 L/min and as APCI carrier gas at 20 psi. The corona discharge voltage was optimized to 2500V resulting in a current of 4 μA.
Iodine-Catalyzed Illumination of All-Trans-Zeaxanthin
One milligram of all-trans-zeaxanthin was dissolved in 25 mL MeOH and the mixture was poured into six tubes. The solution of each tube was evaporated to dryness with nitrogen. After that, iodine catalyst was added at a concentration of 2% (w/w) of the zeaxanthin weight in hexane. The tubes were placed above the fluorescent lamp with a distance of 10 cm and the illumination intensity was 1800 lux. Samples were collected at intervals, following illumination times of 1, 2, 3, 4, and 5 h. After illumination, the solution was filtered through a 0.45 μm membrane filter and collected for HPLC analysis.
Illumination of All-Trans-Zeaxanthin
All-trans-zeaxanthin samples were prepared as mentioned above. The tubes were placed above the fluorescent lamp with a distance of 10 cm and the illumination intensity was 1800 lux. Samples were collected at intervals, following illumination times of 2, 4, 6, 8, 10, 12, 16, 24, and 48 h. After illumination, the solution was filtered through a 0.45 μm membrane filter and collected for HPLC analysis.
Quantitation of All-Trans-Zeaxanthin and Its Cis-Isomers
The quantification of all-trans-zeaxanthin and its cis-isomers was achieved using an internal standard of all-trans-zeaxanthin and calibration curves. The solution was subjected to HPLC analysis and the calibration curve was obtained by plotting concentration ratio against area ratio. As no cis-zeaxanthin standards were available, cis-isomers of zeaxanthin were calculated as all-trans-zeaxanthin equivalents.
Kinetics Analysis
The rate constant of degradation of all-trans-zeaxanthin and formation of cis-isomers was calculated by using the standard equation for a first-order reaction model:
where C represents the concentration at time t, C0 the initial concentration, k the rate constant (h–Citation1) and t the reaction time (h).
Statistical Analysis
All experiments were performed at least in triplicate. The data were subjected to analysis of variance (ANOVA) by using an Origin 8.0 program (OriginLab. Corp., Northampton, MA, USA). Kinetics data were analyzed by regression analysis using Microsoft Excel 2007 and Origin 8.0.
Results and Discussion
HPLC Analysis
A reversed phase C30 column was used to the determination of zeaxanthin and its cis-isomers as reported by our previous study.[Citation17] A gradient mobile phase based on two MeOH–MTBE–water mixtures of different ratios was tried. After various studies, a satisfactory separation of zeaxanthin and its cis-isomers was achieved. The mobile phase consisted of MeOH–MTBE–water (solvent A, 80:15:5, v/v/v) and MeOH–MTBE (solvent B, 1:10, v/v). The gradient program was as followed: 100% A to 70% in 10 min, 70% A to 50% in 16 min. All-trans-zeaxanthin and seven cis-isomers of zeaxanthin were completely separated in this solvent system within 16 min. This solvent system offered a significant improvement over the conventional HPLC separations, which typically take more time to run and resolve less peaks.[Citation18]
Identification of All-Trans Zeaxanthin and Its Cis-Isomers
shows the HPLC chromatogram and absorption spectra of cis-isomers of zeaxanthin as a result of iodine-catalyzed illumination. The characteristics of all-trans-zeaxanthin and its cis-isomers were shown in . Peak 6 was identified as zeaxanthin considering the absorption spectra and MS characteristics and confirmed by co-elution with the all-trans-zeaxanthin standard. Additionally, the characteristic ions [M+H]+ 569.4 and [M+H−18]+ 551.4 were the same as that reported by the previous studies.[Citation19]
FIGURE 1 HPLC chromatogram of all-trans-zeaxanthin and its cis-isomers; (a) HPLC chromatogram of all-trans-zeaxanthin and its cis-isomers obtained from iodine-catalyzed illumination at 450 nm; (b) Absorption spectrum of all-trans-zeaxanthin and its cis-isomers.
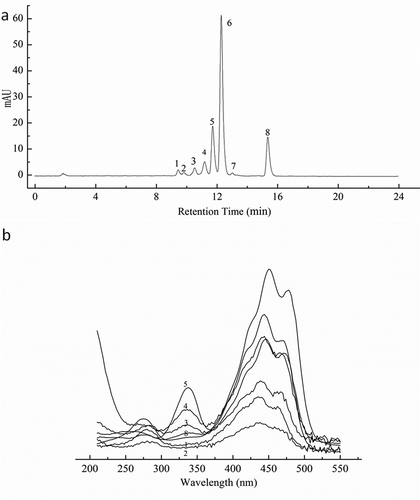
TABLE 1 Identification data for all-trans and cis-form of zeaxanthin
Because standards of cis-carotenoids are not commercially available, the differences that are observed consistently between the spectra of trans- and cis-isomers of carotenoids are, therefore, diagnostic for structural assignment. By comparison to the all-trans configuration, a small hypsochromic shift of λ max is usually observed for cis-isomers (approximately 4 nm for mono-cis and longer for di-cis isomers). A new absorption band appears at a characteristic position around 340 nm is often referred to as the cis-peak. The intensity of the cis-band is essentially greater when the cis double bond is nearer the center of the molecule. The di-cis carotenoid may feature a weak cis-band. In addition, as a derivative of β-carotene, the visible spectrum of zeaxanthin resembles that of β-carotene. Thus, the elution order of cis-isomers of β-carotene was used as a reference to locate the position of the cis double bond in the polyene chain of cis-zeaxanthins.
Peaks 4, 5, and 8 exhibited a hypsochromic shift of 4–6 nm and a pronounced cis-peak (), suggesting that they were the mono-cis-isomers. Comparison with the literature assignments allowed a tentative assignment of these peaks. Aman et al.[Citation18] have identified 13-cis- and 9-cis-zeaxanthin by nuclear magnetic resonance spectroscopy. 13-cis- has been shown to elute before all-tran- and 9-cis- has been shown to elute after all-trans-zeaxanthin. 15-cis-β-carotene eluting before 13-cis- and 9-cis-β-carotene has been observed.[Citation19] Based on order of elution and absorption spectra, the peaks marked 4, 5, and 8 were identified as 15/15’-cis-, 13/13’-cis-, and 9/9’-cis-zeaxanthin, respectively. It has been shown that 13, 15-di-cis- eluted before 15-cis-β-carotene.[Citation20] A tentative assignment of peak 1 was 13, 15-cis-zeaxanthin because it eluted first from the column and because it exhibited a hypsochromic shift of 11 nm and a small cis-band at 338 nm. Based on order of elution and the hypsochromic shift of λ max, the peaks 2 and 3 could be 9, 15-di-cis- and 9, 13-di-cis-zeaxanthin, respectively. 9, 9-di-cis- has been found to elute between all-trans- and 9-cis-β-carotene.[Citation21] Peak 7 eluted between all-trans- and 9/9’-cis-, and showed a hypsochromic shift of 10 nm when compared to the all-trans-zeaxanthin. Thus, it was tentatively identified as 9, 9-di-cis-zeaxanthin. All the identified compounds and their structures were shown in .
Iodine-Catalyzed Illumination of All-Trans-Zeaxanthin
HPLC analysis indicated a pronounced degradation of all-trans-zeaxanthin, accompanied by the increment of cis-isomers (). However, analysis of the total all-trans-zeaxanthin content demonstrated that only a fraction of the degraded all-trans-zeaxanthin can be associated with the formation of its cis-isomers. Indeed, a gas chromatography (GC)-MS study involving the heating of cashew apple carotenoids had been reported by Zepka et al.[Citation22] They found that more than 10 volatile compounds were generated during the process, including low molecular weight aromatic volatiles such as p-xylene, p-cymene, p-cresol, and naphthalene, as well as nonaromatic volatiles such as n-dodecane and 1-tetradecene. Thus, the disappearance of all-trans-zeaxanthin in present study may be attributed to the generation of both volatile and low molecular weight compounds not detected by high performance liquid chromatography - photo diode array detection - atmospheric pressure chemical ionization-mass spectrometry (HPLC-DAD-APCI-MS). Results of order determination showed that the first order kinetic model was the most appropriate for modeling all-trans-zeaxanthin degradation (). Indeed, the correlation coefficients of zero order, first order, and second order were 0.940, 0.958, and 0.941, respectively, and the coefficient of variation was 11, 9, and 13%, respectively. The rate constant was about five-fold lower than that of all-trans-β-cryptoxanthin in the same model system.[Citation23] Zeaxanthin includes the presence of two hydroxyl groups on carbons C-3 and C-3’ in terms of structure. This symmetry in the molecule is possibly the reason for its lower degradation. Furthermore, zeaxanthin showed a different behavior under various conditions. The natural environment of zeaxanthin could provide protection from pH value, phytochemical compounds, temperature, and so on.[Citation24] It has been reported that zeaxanthin degraded four- to five-fold faster in the neutral system than in the acid system. The phytochemicals such as flavonoids or reducing sugars may lower the degradation rate of zeaxanthin in real food system. In addition, zeaxanthin esters responded differently to the oxidation process, and were degraded more quickly than free zeaxanthin.[Citation25]
FIGURE 3 Iodine-catalyzed illumination of all-trans-zeaxanthin; (a) Concentration changes of all-trans-zeaxanthin and its cis-isomers during iodine-catalyzed illumination for the indicated time periods; (b) First-order plot for the degradation of total all-trans-zeaxanthin during iodine-catalyzed illumination; (c) Concentration changes of cis-isomers derived from all-trans-zeaxanthin during iodine-catalyzed illumination for the indicated time periods.
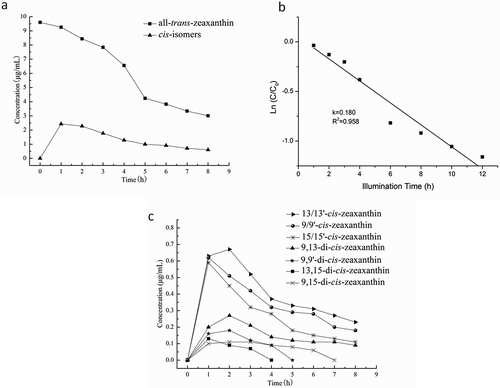
shows the concentration changes of cis-isomers of all-trans-zeaxanthin during iodine-catalyzed illumination. The 15/15’-cis-, 13/13’-cis-, and 9/9’-cis- zeaxanthin were observed to get into significant production after 1 h illumination. After illumination time reached 2 h, the levels of all the mono-cis isomers of all-trans-zeaxanthin began to decrease. The amounts of 15/15’-cis-, 13/13’-cis-, and 9/9’-cis-zeaxanthin decreased by 81.4, 71.0, 65.7%, respectively, after 8 h exposure to light. These results indicated the degradation rates of 15/15’-cis-, 13/13’-cis-, and 9/9’-cis-zeaxanthin might be greater than the formation rates. In addition, the conversion between all-trans-β-carotene and its mono-cis isomers has been reported to be reversible.[Citation26] Thus, the decreased in 15/15’-cis-, 13/13’-cis-, and 9/9’-cis- zeaxanthin suggested that all these mono-cis isomers may be converted to all-trans-zeaxanthin or undergo degradation simultaneously during illumination. For 15-cis-, it formed at a concentration less than that of 13/13’-cis- and 9/9’-cis-zeaxanthin. This finding is similar to that in the study of Chen et al.,[Citation27] who reported that 15-cis- was formed in smallest amount among all the cis-isomers of β-carotene. On one hand, it is possible that it was difficult to form because of a steric hindrance effect. On the other hand, the photosensitivity of 15-cis- is greater than that of 9-cis and 13-cis-.[Citation28] The unstable nature of 15-cis- implied that it can be degraded or converted to the other forms of cis-isomers more easily than either 9-cis- or 13-cis-. No significant change (p > 0.05) was observed for 15/15’-cis-, 13/13’-cis-, or 9/9’-cis-zeaxanthin after illumination time reached 7 h, this result implied that the whole system reached equilibrium.
Chen and Huang[Citation27] have reported that the large amount of 13, 15-di-cis- was formed during iodine-catalyzed illumination of all-trans-β-carotene. In present study, the formation of 13, 15-di-cis-, 9, 15-di-cis-, 9, 13-di-cis- and 9, 9’-di-cis- zeaxanthin was found during iodine-catalyzed illumination of all-trans-zeaxanthin. As shown in , the formation levels and rates of 9, 13-di-cis- were greatest, followed by 9, 9’-di-cis-, 13, 15-di-cis- and 9, 15-di-cis- zeaxanthin. It was reported that the formation of di-cis isomers may due to conversion of mono-cis isomers.[Citation27] 9-cis- and 13-cis- exhibited higher stability compared to the 7-cis- and 15-cis-.[Citation29] It is likely that the formation condition of di-cis isomers was associated with that of the mono-cis isomers. Thus, the formation levels and rates of 9, 13-di-cis- were related to that of 9-cis- or 13-cis-zeaxanthin.
Illumination of All-Trans-Zeaxanthin
shows the concentration changes of all-trans-zeaxanthin and cis-isomers during illumination. showed the experimental and model fit results for all-trans-zeaxanthin degradation. The all-trans-zeaxanthin degradation during non-iodine illumination in model system followed a first order kinetic model with a variation coefficient of 8%. The degradation rate constant of all-trans-zeaxanthin during iodine-catalyzed illumination was two-fold faster than that of all-trans-zeaxanthin during non-iodine-catalyzed illumination, indicating that the iodine probably accelerated the degradation rate of all-trans-zeaxanthin.
FIGURE 4 Illumination of all-trans-zeaxanthin; (a) Concentration changes of all-trans-zeaxanthin and its cis-isomers during illumination without iodine as catalyst for the indicated time periods; (b) First-order plot for the degradation of total all-trans-zeaxanthin during non-iodine-catalyzed illumination; (c) Concentration changes of cis-isomers derived from all-trans-zeaxanthin during non-iodine-catalyzed illumination for the indicated time periods.
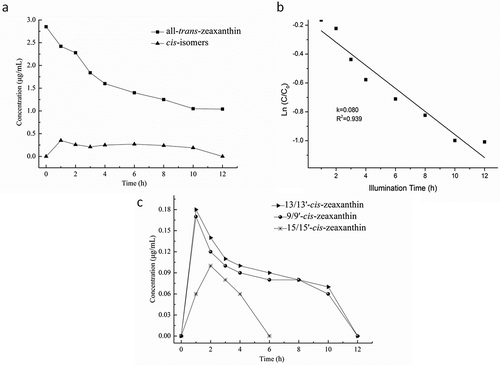
shows the concentration changes of cis-isomers of all-trans-zeaxanthin during non-iodine illumination. 15/15’-cis-, 13/13’-cis-, and 9/9’-cis- zeaxanthin reached their highest levels after 1 h illumination. 13/13’-cis- was formed in largest amount, followed by 9/9’-cis- and 15/15’-cis-zeaxanthin. By comparison of iodine-catalyzed illumination, the formation of di-cis-zeaxanthin was not observed during non-iodine illumination. It is likely that the contents of di-cis isomers were under the detection threshold. Furthermore, it is presumably due to the absent of iodine. It was found that iodine was not covalently bound to the carotene.[Citation30] A mixture of solutions of iodine and β, β-carotene in hexane provided a β, β-carotene-iodine complex with cationic/radical cationic properties, containing iodine in a π-complex.[Citation31] The major quenching product treated with acetone or thiosulfate furnished isocarotene. All quenching products obtained from the iodine complexes are strongly E/Z isomerized, consistent with cationic intermediates. Thus, the presence of iodine may facilitate formation of di-cis-zeaxanthin during illumination. Moreover, the activation energy for formation of di-cis-zeaxanthin should be higher than that of mono-cis-zeaxanthin. The energy supplement to rotation might be different during two treatments. The conversion energy for formation of di-cis-zeaxanthin was lowered because of the presence of catalyst iodine.
Conclusion
A C30-HPLC method that allowed to analyze all-trans-zeaxanthin and its cis-isomers in a model system had been developed. A total of three mono-cis-isomers and four di-cis-isomers were detected during illumination by HPLC-DAD-APCI-MS. Among the formed cis-isomers of all-trans-zeaxanthin, 13/13’-cis-zeaxanthin was the major mono-cis-isomer. The formation of di-cis isomers may prefer in the present of iodine. 9,13-di-cis-zeaxanthin was the major di-cis-isomer of the iodine-catalyzed photo-isomerization. The degradation rate of all-trans-zeaxanthin during iodine-catalyzed illumination was faster than that of all-trans-zeaxanthin during non-iodine-catalyzed illumination. These findings greatly contribute to the understanding of the stability of all-trans-zeaxanthin during illumination. Much more work will be done to study the mechanisms of all-trans-zeaxanthin degradation and isomerization in various real food matrices and in various processing and storage conditions.
Funding
The authors would like to thank Jiangsu Academy of Agricultural Sciences for Innovation in Agriculture Science and Technology [project no. CX (14) 2055] for the financial support.
Additional information
Funding
References
- Snodderly, D.M. Evidence for Protection Against Age-Relatedmacular Degeneration by Carotenoids and Antioxidant Vitamins. The American Journal of Clinical Nutrition 1995, 62, 1448S–1461S.
- Sajilata, M.G.; Singhal R.S.; Kamat M.Y. The Carotenoid Pigment Zeaxanthin—A Review. Comprehensive Reviews in Food Science and Food Safety 2008, 7, 29–49.
- Khachik, F.; Nir, Z.; Ausich, R.; Steck, A.; Pfander, H. Distribution of Carotenoids in Fruits and Vegetables As a Criterion for the Selection of Appropriate Chemopreventative Factors. In Food Factors for Cancer Prevention; H. Ohigashi, T. Osawa, J. Terao, S. Watanabe, T. Yoshikawa (Eds.); Tokyo, Japan: Springer, 1997; 204–208.
- Sommerburg, O.; Keunen, J.; Bird, A.; van Kuijk, F. Fruits and Vegetables That Are Sources of Lutein and Zeaxanthin: The Macular Pigment in Human Eyes. The British Journal of Ophthalmology 1998, 82, 907–910.
- Bian, Q.; Gao, S.; Zhou, J.; Qin, J.; Taylor, A.; Johnson, E.J.; Tang, G.; Sparrow, J.R.; Gierhart, D.; Shang, F. Lutein and Zeaxanthin Supplementation Reduces Photooxidative Damage and Modulates the Expression of Inflammation-Related Genes in Retinal Pigment Epithelial Cells. Free Radical Biology & Medicine 2012, 53, 1298–1307.
- Roberts, R.L.; Green, J.; Lewis, B. Lutein and Zeaxanthin in Eye and Skin Health. Clinics in Dermatology 2009, 27, 195–201.
- Ma, L.; Lin, X.M. Effects of Lutein and Zeaxanthin on Aspects of Eye Health. Journal of the Science of Food and Agriculture 2010, 90, 2–12.
- Inbaraj, B.S.; Lu, H.; Hung, C.F.; Wu, W.B.; Lin, C.L.; Chen, B.H. Determination of Carotenoids and Their Esters in Fruits of Lycium Barbarum Linnaeus by HPLC-DAD-APCI-MS. Journal of Pharmaceutical and Biomedical Analysis 2008, 47, 812–818.
- Meléndez-Martínez, A.J.; Britton, G.; Vicario, I.M.; Heredia, F.J. The Complex Carotenoid Pattern of Orange Juices from Concentrate. Food Chemistry 2008, 109, 546–553.
- Aparicio-Ruiz, R.; Minguez-Mosquera, M.I.; Gandul-Rojas, B. Thermal Degradation Kinetics of Lutein, β-Carotene, and β-Cryptoxanthin in Virgin Olive Oils. Journal of Food Composition and Analysis 2011, 24, 811–820.
- Dhuique-Mayer, C.; Tbatou, M.; Carail, M.; Caris-Veyrat, C.; Dornier, M.; Amiot, M.J. Thermal Degradation of Antioxidant Micronutrients in Citrus Juice: Kinetics and Newly Formed Compounds. Journal of Agricultural and Food Chemistry 2007, 55, 4209–4216.
- Hidalgo, A.; Brandolini, A. Kinetics of Carotenoids Degradation During the Storage of Einkorn (Triticum Monococcum L. ssp Monococcum) and Bread Wheat (Triticum Aestivum L. ssp Aestivum) Flours. Journal of Agricultural and Food Chemistry 2008, 56, 11300–11305.
- Zepka, L.Q.; Mercadante, A.Z. Degradation Compounds of Carotenoids Formed During Heating of a Simulated Cashew Apple Juice. Food Chemistry 2009, 117, 28–34.
- Henry, L.K.; Catignani, G.L.; Schwartz, S.J. Oxidative Degradation Kinetics of Lycopene, Lutein, and 9-Cis and All-Trans β-Carotene. Journal of the American Oil Chemists Society 1998, 75, 823–829.
- Milanowska, J.; Gruszecki, W.I. Heat-Induced and Light-Induced Isomerization of the Xanthophyll Pigment Zeaxanthin. Journal of Photochemistry and Photobiology B: Biology 2005, 80, 178–186.
- Melendez-Martinez, A.J.; Stinco, C.M.; Liu, C.; Wang, X.D. A Simple HPLC Method for the Comprehensive Analysis of Cis/Trans (Z/E) Geometrical Isomers of Carotenoids for Nutritional Studies. Food Chemistry. 2013, 138, 1341–1350.
- Song, J.F.; Li, D.J.; He, M.J.; Chen, J.Q.; Liu, C.Q. Comparsion of Carotenoid Composition in Immature and Mature Grains of Corn (Zea Mays L.) Varieties. International Journal of Food Properties 2016, 19(2), 351–358.
- Aman, R.; Biehl, J.; Carle, R.; Conrad, J.; Beifuss, U.; Schieber, A. Application of HPLC Coupled with DAD, APcI-MS and NMR to the Analysis of Lutein and Zeaxanthin Stereoisomers in Thermally Processed Vegetables. Food Chemistry 2005, 92, 753–763.
- Kao, T.H.; Loh, C.H.; Inbaraj, B.S.; Chen, B.H. Determination of Carotenoids in Taraxacum Formosanum by HPLC-DAD-APCI-MS and Preparation by Column Chromatography. Journal of Pharmaceutical and Biomedical Analysis 2012, 66, 144–153.
- Glaser, T.; Lienau, A.; Zeeb, D.; Krucker, M.; Dachtler, M.; Albert, K. Qualitative and Quantitative Determination of Carotenoid Stereoisomers in a Variety of Spinach Samples by Use of MSPD Before HPLC-UV, HPLC-APCI-MS, and HPLC-NMR On-Line Coupling. Chromatographia 2003, 57, S19–S25.
- Mortensen, A. Analysis of a Complex Mixture of Carotenes from Oil Palm (Elaeis Guineensis) Fruit Extract. Food Research International 2005, 38, 847–853.
- Zepka, L.Q.; Borsarelli, C.D.; Silva, M.A.A.P.D.; Mercadante, A.Z. Thermal Degradation Kinetics of Carotenoids in a Cashew Apple Juice Model and Its Impact on the System Color. Journal of Agricultural and Food Chemistry 2009, 57, 7841–7845.
- Li, D.J.; Xiao, Y.D.; Zhang, Z.Y.; Liu, C.Q. Analysis of (All-E)-Lutein and Its (Z)-Isomers During Illumination in a Model System. Journal of Pharmaceutical and Biomedical Analysis 2014, 100, 33–39.
- Hadjala, T.; Dhuique-Mayerb, C.; Madania, K.; Dornierc, M.; Achir, N. Thermal Degradation Kinetics of Xanthophylls from Blood Orange in Model and Real Food Systems. Food Chemistry 2013, 138(4), 2442–2450.
- Perez-Galvez, A.; Minguez-Mosquera, M.I. Degradation of Non-Esterified and Esterified Xanthophylls by Free Radicals. Biochimica et Biophysica Acta 2002, 1569, 31–34.
- Chen, B.H.; Chen, T.M.; Chien, J.T. Kinetic Model for Studying the Isomerization of α-and β-Carotene During Heating and Illumination. Journal of Agricultural and Food Chemistry 1994, 42, 2391–2397.
- Chen, B.H.; Huang, J.H. Degradation and Isomerization of Chlorophyll α- and β-Carotene As Affected by Various Heating and Illumination Treatments. Food Chemistry 1998, 62, 299–307.
- Zechmeister, L. Cis-Trans Isomeric Carotenoids, Vitamins A and Arylpolyenes; Springer: Vienna, 1962.
- Doering, W.vE.; Sotiriou-Leventis, C.; Roth, W.R. Thermal Interconversions Among 15-Cis-,13-Cis-, and All-Trans-β-Carotene: Kinetics, Arrhenius Parameters, Thermochemistry, and Potential Relevance to Anticarcinogenicity of All-Trans-β-Carotene. Journal of the American Chemical Society 1995, 117, 2747–2757.
- Lutnaes, B.F.; Krane, J.; Liaaen-Jensen, Sv. On the Structure of Carotenoid Iodine Complexes. Organic & Biomolecular Chemistry 2004, 2, 2821–2828.
- Molnár, P. Research of the (E/Z)-Isomerization of Carotenoids in Pécs Since the 1970s. Archives of Biochemistry and Biophysics 2009, 483, 156–164.