ABSTRACT
Polylactide based films were formulated by incorporating polyethylene glycol, selected nanopowders (zinc oxide, silver-copper), and essential oils (cinnamon, garlic, and clove) by solvent casting method. Films were tested against three foodborne pathogens (one gram-positive and two gram-negative) for their antibacterial activity. The effectiveness of selected cinnamon oil-based film was ascertained by performing a challenge test with cheese as a food model. In vitro antibacterial efficacies of nanopowders and essential oils were also determined by the decimal reduction concentrations and the minimum bactericidal concentrations for those foodborne pathogens. It was observed that nanopowders exhibited considerably poorer decimal reduction concentrations and minimum bactericidal concentration values in comparison to the essential oils. Silver-copper alloy nanopowders exhibited lower decimal reduction concentrations and minimum bactericidal concentrations values than ZnO against tested pathogens whereas essential oils showed distinct antimicrobial effectiveness against all those pathogens with in vitro decimal reduction concentration values of 87–157 and 77–220 µg/mL for cinnamon and clove oils, respectively. Among the various formulations, it was observed that only essential oils (especially cinnamon and clove) incorporated films exhibited a significant antimicrobial activity against the selected microorganisms. These results indicate that the poor antibacterial activity of the nanopowders and the hydrophobicity of polylactide could be responsible for the ineffectiveness of nanopowders in polylactide based films. Furthermore, the challenge test indicated the polylactide/polyethylene glycol/cinnamon oil film was appropriate to inhibit the growth of L. monocytogenes and S. typhimurium on cheese up to 11 days at refrigerated storage.
Introduction
Polylactides (PLA) are relatively low cost polymers derived from renewable sources such as corn, sugar beet, wood residues, and other biomass are of current interest because of their many potentially useful physical and mechanical characteristics.[Citation1,Citation2] Over the last few decades, PLA has been extensively studied for use in medical applications because of its bioabsorbability, biocompatibilty, and controlled drug delivery capabilities.[Citation3] Currently, packaging material made with PLA is considered as an alternative solution for reducing the ecological problems that is generated by petroleum-based plastic packaging as it is a biodegradable material.
The use of PLA in food packaging has already received tremendous attention,[Citation4–Citation6] and presently, PLA-based packaging and containers are commercially available in many countries especially in Europe, Japan, and North America. Furthermore, PLA-based packaging materials have been generally recognized as safe (GRAS) which put it in a unique position for food applications.[Citation7] In general, PLA is a comparatively rigid and brittle polymer with a low deformation at break. Plasticizer like low molecular weight polyethylene glycol (PEG) has been added to improve the flexibility of the polymer by abruptly lowering the glass transition temperature (Tg). However, the low Tg of produced blends may affect the processing and molding of final products.[Citation8] Furthermore, introduction of particulate nanofillers (clay or metal-based) into polymer matrices is an elegant technique to improve/modify properties of polymer blends. Such nanoparticles offer enormous advantages over traditional macro- or micro-particles due to their higher surface area and aspect ratio, improved adhesion between nanoparticle and polymer, and lower amount of loading to achieve equivalent properties.
PLA is also considered as one of the most promising biocompatible materials for the creation of a new generation active and intelligent food packaging films with antibacterial properties. As a result, a wide variety of organic and inorganic additives or substances have been either incorporated or coated onto PLA based materials. Typically, substances such as silver (Ag), nisin, lemon extracts, thymol, and lysosyme have been explored for providing the antibacterial properties.[Citation9–Citation11]
Inorganic metal/metal oxide nanoparticles of silver (Ag), zinc oxide (ZnO) are thermally stable, non-volatile, exhibit excellent antibacterial activity against wide range of gram-positive and gram-negative bacteria including major foodborne pathogens like Escherichia coli O157:H7, Salmonella typhimurium, Listeria monocytogenes, and Staphylococcus aureus and have been approved by United States Food and Drug Administration for use in food packaging applications.[Citation12,Citation13] When incorporated in PLA-based films, these nanoparticles (Ag, ZnO) have shown inhibitory against gram-positive and gram-negative bacteria.[Citation14–Citation17] In particular, PLA/Ag films have shown excellent antibacterial activity against pathogenic strains of Salmonella enterica, Vibrio parahaemolyticus, and Staphylococcus aureus.[Citation18,Citation19] Nevertheless, information about the antibacterial activity of PLA/ZnO films especially against foodborne pathogens is lacking.
Single metal nanoparticles have been often alloyed to produce bimetallic nanoparticles with enhanced optical, electrical, and catalytic properties. For instance, Ag nanoparticles have been alloyed with copper (Cu) nanoparticles to enhance their interfacial and catalytic activity.[Citation20] Few studies have synthesized silver–copper (Ag-Cu) nanoparticles and investigated their antibacterial properties. It was observed that Ag-Cu alloy nanoparticles exhibited excellent antibacterial activity even at low concentrations in addition to being superior than single Ag or Cu nanoparticles.[Citation21–Citation24] However, to the best of our knowledge, there are still no studies on the use of Ag-Cu nanoparticles as antibacterial substances for the development of PLA-based films with antibacterial properties.
Organic substances such as essential oils (EOs) are categorized as GRAS by the U.S. Food and Drug Administration as well as the European Legislation for materials intended to be in contact with food. Thyme, cinnamon, clove, basil, oregano, garlic, and basil oils have been intensively explored for the development of food packaging films due to their excellent antibacterial properties against foodborne pathogens.[Citation25–Citation28] A range of both biodegradable and non-biodegradable polymer films, such as chitosan, fish skin, whey protein isolate, low-density polyethylene, ethylene vinyl alcohol, poly(ethylene terephthalate), and polypropylene films, have either coated or incorporated with EO and evaluated for their antibacterial effectiveness.[Citation29] Surprisingly, not many studies are available in the literature on the use of PLA-based films as carriers of EOs for antibacterial packaging applications. In addition, there is lack of information with regards to the application of PLA-based EO films, especially on food systems such as cheese and meats. Recently, antibacterial activity of PLA-based films incorporated with cinnamaldehyde against gram-negative E. coli and gram-positive S. aureus was revealed.[Citation30] Tests with these developed films were also found to be effective in reducing the mesophilic and psychrophilic bacterial counts of button mushrooms and extend its postharvest life.[Citation31] In another similar recent study, PLA films containing an extract of Allium spp. EO were shown to have inhibitory effect against several molds, yeast, and pathogenic bacteria and those films were also observed to be effective for inhibiting the growth of aerobic, enterobacteriaceae, yeast, and mold on ready-to-eat salads for up to 7 days under refrigerated storage conditions.[Citation32]
The main objective of this study was to evaluate the antibacterial effectiveness of plasticized PLA-based films prepared with metal nanopowders (NP), namely ZnO (metal oxide), Ag-Cu alloy, and EOs, namely cinnamon, garlic, and clove oils (CLOs), individual or in combination against three common foodborne pathogens (Listeria monocytogenes, Salmonella typhimurium, and E. coli O157:H7). Furthermore, the effectiveness of developed films was verified against two pathogens (Listeria monocytogenes and Salmonella typhimurium) with cheese as a food system for assessing their feasibility to be used in food packaging applications.
Materials and Methods
Materials
A commercial grade poly (lactic acid; PLA; Ingeo™ 4043D) was procured from NatureWorks (Minnetonka, MN, USA) and dried overnight at 60°C in a vacuum oven before use. Dichloromethane (DCM) was obtained from Fisher Scientific (Loughborough, LE, UK) and used as received. PEG (average MW = 1500 g/mol), zinc oxides [ZnO50 (particle size <50 nm) and ZnO100 (particle size <100 nm)], Ag-Cu (particle size <100 nm), cinnamon oil (CIN), garlic oil (GAR), CLO, Tween® 80, and ethanol were purchased from Sigma-Aldrich (St. Louis, MO, USA) and used as received. Processed cheddar cheese slices (Almarai Brand, KSA) were obtained locally and were used as it is.
Bacterial Strains and Media
Lyfo Disk pellets® of gram-positive Listeria monocytogenes (ATCC 19114) strain was obtained from MediMark Europe (Grenoble, CEDEX, France). Culti-loops® of gram-negative strains Escherichia coli O157:H7 (ATCC 43888) and Salmonella enterica sv thyphimurium (ATCC 14028) strains were purchased from Remel Europe Ltd. (Dartford, Kent, UK). Brain heart infusion agar (BHIA) was procured from Conda Laboratories (Torrejón de Ardoz, MD, Spain). Tryptic soya broth (TSB) and Muller Hinton agar (MHA) were obtained from TM Media (Bhiwadi, India). Polymyxin-Acriflavin-Lithium chloride-Ceftazidime-Aesculin-Mannitol (PALCAM) agar base, PALCAM selective supplement, and Xylose lysine deoxycholate agar (XLD) were purchased from Oxoid (Basingstoke, HM, UK).
Preparation of Inoculum
All strains were first revived in TSB at 37°C for 18–24 h before subcultures were made by streaking on BHIA (gram-positive bacteria) and MHA (gram-negative bacteria) plates. A standardized inoculum containing 108 CFU/mL was freshly prepared in buffered peptone water using the direct colony suspension method, with the aid of the 0.5 McFarland turbidity standards to perform the antibacterial tests.
Determination of the in Vitro Antibacterial Effectiveness of NP and EOs
Prior to incorporation of NP and EOs into PLA based films, in vitro antimicrobial properties of individual NP/EO were determined against L. monocytogenes, S. typhimurium, and E. coli O157:H7 using the macro broth-dilution method.[Citation33] Initially, stock solutions of NP and EO were prepared according to methods described by Tayel et al.[Citation34] and Kim et al.[Citation35] Briefly, NP were dispersed in distilled water, sterilized, vigorously vortexed for 10 min, and additionally sonicated for 1 h to avoid aggregation and deposition of particles. EO stocks were prepared by dissolving them in a mixture of sterile distilled water containing 1% Tween 80 and 1% of ethanol for enhancing the solubility of the EO compounds in aqueous solvent. The NP stocks solutions were stored at 4°C, whereas the EO stocks were freshly prepared prior to use.
Next, stock suspensions were diluted in sterile TSB and inoculated with an appropriate volume of 108 CFU/mL of bacteria cell cultures to achieve final concentrations ranging between 600 to 15,000 µg/mL for NP and 200 to 1600 µg/mL for EO, respectively. The inoculated suspensions with bacterial cultures were incubated at 37°C for 24 h and checked for counts by culturing onto BHIA plates. The viable cell survivors were calculated and expressed in CFU/mL. Suspensions without NP and EO were served as controls.
The survival of bacteria was analyzed based on a first order reaction kinetics indicating a logarithmic order of death, and expressed as Eq. (1).
where N is the number of surviving bacteria, N0 is the initial number of bacteria and decimal reduction concentrations (Dc) is the decimal reduction concentration which is defined as the concentration that results in the reduction of 90% of the existing microbial population. Dc values were obtained from the slope of the linear regression between log (N/N0) versus C (Eq. [2]).
Furthermore, the minimum bactericidal concentrations (MBC) of NP and EO were also recorded. The MBC was the lowest concentration of EO that resulted in more than 99.9% mortality of the bacterium relative to the concentration of microorganism that was present in the initial inoculum.[Citation36]
Preparation of PLA-Based Films
PLA based blended films (PLA/PEG, PLA/PEG/NP, PLA/PEG/EO, PLA/PEG/NP/EO) were prepared by the solvent casting method at room temperature (~25°C) conditions according to the method of Ahmed et al.[Citation8] Briefly, PLA, PEG, and/or NP was weighed separately, dry mixed and completely dissolved in DCM (30 mL DCM/g of PLA) by vigorous mixing for an hour on a magnetic stir plate. The resultant solution was sonicated (Branson Ultrasonics, CT, USA) for 30 min and stirred for 10 min. In the next step, EO was added (w/w based on the content of the PLA) to the mixture with gently stirring for an additional 10 min.[Citation37] The resultant solutions were casted evenly onto glass Petri dishes with a 100 mm diameter and 150 mm depth. The solvent was allowed to evaporate at room temperature under a fume hood. The films were peeled from the glass Petri dishes after 12 h, sealed in plastic pouches and stored in desiccator until used. Several films with diverse formulations were casted.
PLA/PEG films were prepared by addition of 0, 5, 10, 20, and 30 wt% PEG to PLA and these films were named as PLA/PEG: 100/0, 95/5, 90/10, 80/20, and 70/30, respectively.
PLA/PEG/NP films (PLA/PEG/ZnO100, PLA/PEG/ZnO50, and PLA/PEG/Ag-Cu) with varying contents of NP ranging between 1–4 wt% (79/20/1, 78/20/2, 77/20/3, and 76/20/4) were obtained by combining PLA/PEG with ZnO100, ZnO50, and Ag-Cu NP.
Cinnamon, garlic, or clove EOs were combined with PLA/PEG at 100% (w/w, based on PLA content) to make PLA/PEG/EO films (PLA/PEG/CIN100, PLA/PEG/GAR100, and PLA/PEG/CLO100).
In one set of experiment the CIN concentration was varied from to 10 to 75% (w/w based on PLA content) to elucidate the effect of concentration on tested microorganism and these films were labeled as PLA/PEG/CIN10, PLA/PEG/CIN50, and PLA/PEG/CIN75 films.
To study the synergic effect between NP and EO, another set of PLA/PEG/NP/EO film was prepared with addition of CIN to both PLA/PEG/ZnO100 and PLA/PEG/Ag-Cu 78/20/2 blends at levels of 100% w/w PLA content and designated as PLA/PEG/ZnO100/CIN100 and PLA/PEG/Ag-Cu/CIN100 films.
Evaluation of Antibacterial Effectiveness of PLA-Based Films
The antimicrobial effectiveness of the PLA-based blend films were evaluated against L. monocytogenes (gram-positive), S. typhimurium (gram-negative), and E. coli O157:H7 (gram-negative) using the agar disc diffusion method.[Citation38] In addition selected formulated films were also tested for their antibacterial efficacy using the liquid culture test.[Citation30] Films were cut into a disc shape with a diameter of 20 mm using a metal hole-puncher and both the sides of the discs were sterilized under ultraviolet light for 15 min prior to testing.
In the agar disc diffusion method sterile film discs were placed on BHIA (gram-positive) or MHA (gram-negative) plates that had been previously smeared with standardized inoculums containing ~108 CFU/mL of bacteria. The plates were incubated at 37°C for 24 h. At the end of that period, the diameter of the clear zone around the disc was measured with a caliper (Mitutoyo, Japan) and expressed in millimeters (mm). Tests were carried out in with duplicate sampling and repeated two times.
For the liquid culture test, a film disc was placed in a test tube and filled with 10 mL TSB. The TSB was inoculated with 0.1 mL of an overnight culture of bacteria adjusted to a cell concentration of 108 CFU/mL. The test tubes were shaken at 200 rpm under room temperature (25ºC) conditions by using a shaker. After 24 and 48 h, aliquots (1 mL) from the test tubes were withdrawn and serially diluted with buffered peptone water and then spread plated onto BHIA plates. Plates were incubated at 37°C for 24 h and the colony-forming units (CFU) were counted. PLA/PEG film served as a control.
Verification Test with Processed Cheese
The antimicrobial effectiveness of PLA-based films against L. monocytogenes and S. typhimurium was tested using cheese as a model food. Two sets (eight discs per set) of sterilized discs of cheddar cheese with 20-mm diameter (prepared the same way as PLA-based films) were placed in two separate Petri dishes. To each Petri dish, a 5 mL aliquot of a 107 CFU/mL inoculum (prepared by diluting the standardized inoculum of 108 CFU/mL in peptone water) of L. monocytogenes and S. typhimurium bacteria were added separately, spread over the discs using sterile spreaders, and kept under the laminar hood for 15 min to allow attachment. Afterward, two inoculated cheese discs (weighing approximately 2 g) contaminated with L. monocytogenes or S. typhimurium were wrapped individually with PLA-based film (3 × 3 cm), heat sealed and stored in Petri dishes at 4°C. Wrapped samples were collected and checked for the existence of L. monocytogenes or S. typhimurium by enumeration at 0, 2, 4, 7, and 11 days during the storage period. Cheese samples wrapped with PLA/PEG films served as a control.
For enumeration, the films were carefully removed from cheese disc, and then homogenized for 2 min at in 10 mL of sterile peptone water (0.1% w/v) with a vortex. From this homogenate, serial dilutions were prepared in peptone water and spread-plated on PALCAM agar for L. monocytogenes and XLD agar for S. typhimurium, respectively. Plates were incubated at 37°C for 48 h before bacterial enumeration and expressed as log CFU/g of cheese. All samples were analyzed in duplicate and repeated two times (n = 4).
Results and Discussion
Antibacterial Effectiveness of NP and EOs (in Vitro)
The antimicrobial effectiveness of NP and EO were determined in terms of their Dc and MBC values. Commonly the minimal inhibitory concentrations (MIC) along with the MBC are standard measures of in vitro antimicrobial activities of NP and EO. MIC are is often defined as the lowest concentration of NP or EO that inhibit the visible growth of organism in the test media among several other definitions.[Citation26] In this study, it was not possible to determine the MIC from the visual appearance of the medium as the prepared NP and EO suspensions were cloudy. Therefore, the MIC of NP and EO were calculated from the survivor curves and reported as Dc values. The Dc value was equivalent to MIC90 and corresponded to the lowest concentration of NP or EO that were able to decrease the bacterial population by 90% (1 log reduction).[Citation39]
illustrates the survival curves of tested microorganisms at different NP concentrations. Logarithm of bacterial survival (N) decreased linearly with increasing EO and NP concentrations, and it was found that the microbial destruction followed the first-order reaction kinetics (RCitation2 > 0.90). The calculated Dc values from the survivor curves () were used to determine the antibacterial effectiveness of EO and NP. The lower Dc values confirmed the higher the antibacterial effectiveness. The Dc values of NPs were ranged between 139 and 1655 µg/mL. Among NPs, Ag-Cu was found to be the most effective than ZnO against test microorganisms (139–302 µg/mL). The higher antimicrobial efficacy of Ag-Cu in comparison to ZnO could be attributed by higher Ag content (~95%) in the bimetallic alloy mixture. It has been reported that Ag NP exhibited antibacterial activities at even very low concentrations, whereas the antibacterial activity of ZnO nanoparticles were dependent on the concentration and surface area of the particle.[Citation40] Furthermore, ZnO50 showed higher AMB efficacy (473–883 µg/mL) than ZnO100 (782–1656 µg/mL) against the test pathogens. It clearly demonstrated that the finer particle with larger surface to volume ratio had an added advantage in bacterial destruction. Similar antimicrobial activities attributed by finer ZnO have been reported earlier.[Citation41] Among NPs, the antibacterial effectiveness against the test microorganisms can be ordered as: Ag-Cu > ZnO50 > ZnO100.
Table 1. Decimal reduction concentrations (Dc, µg/mL) values of for nanopowders and essential oils for selected microorganisms.
Figure 1. Typical survival curves of food borne pathogens at different concentrations of nanopowders (A: L. monocytogenes; B: S. typhimurium; C: E. coli O157:H7).
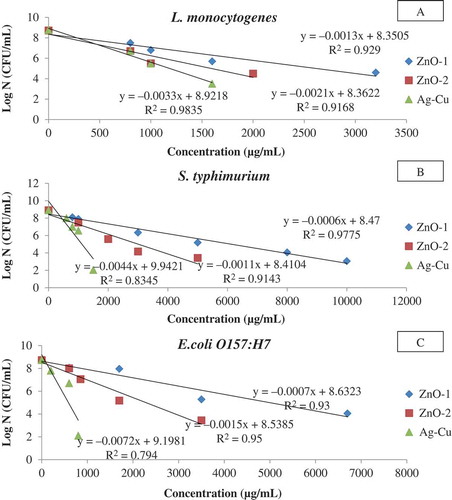
Among tested NPs, it was observed that Ag-Cu had a higher effect against tested gram-negative strains with lower Dc values. The Dc values were 140 and 227 µg/mL for E. coli O157:H7 and S. typhimurium, respectively, while the corresponding Dc value for the gram-positive bacteria (L. monocytogenes) was 302.26 µg/mL. A higher biocidal efficiency of Ag-Cu and Ag against gram-negative bacteria has been reported.[Citation23,Citation42] It was opined that the higher effectiveness of Ag nanoparticles against gram-negative bacteria has been attributed by the difference in cell wall structure between gram-negative and gram-positive microorganisms. Both ZnO NPs showed stronger antibacterial effectiveness against gram-positive (473–782 µg/mL) than gram-negative bacterial strains (646–1656 µg/mL). These observations are supported by earlier study carried out by Tayel et al.[Citation34] Those authors investigated the antibacterial action of ZnO against two gram-positive and seven gram-negative foodborne pathogens and reported that the gram-positive strains were more susceptible to ZnO than the gram-negative strains.
Three selected EOs namely cinnamon, garlic, and CLOs were tested against the test organisms, and it was observed that the Dc values were ranged from 87–157, 81–159, and 77–220 µg/mL, respectively (, ). Garlic and CLOs were more effective against gram-positive than gram-negative strains, whereas CIN showed the best result for E. coli O157:H7 (87.05 µg/mL). It can be seen from that S. typhimurium (157–220 µg/mL) showed the maximum resistance against EOs. It has been reported that EO including garlic, and clove are active against gram-positive than gram-negative bacteria due to the absence of external lipopolysaccharide wall surrounding the peptidoglycan cell membrane of the gram-positive bacteria; however, conflicting results have also been found.[Citation27]
Figure 2. Typical survival curves of foodborne pathogens at different concentrations of essential oils (A: L. monocytogenes; B: S. typhimurium; C: E. coli O157:H7).
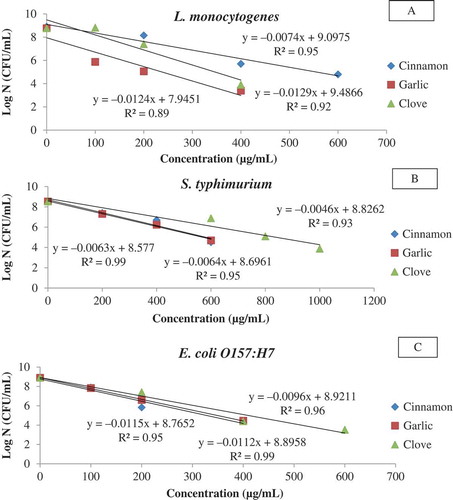
In addition to the Dc values, the antimicrobial efficiency of NP and EO were determined using the MBC, as an indication of total bacterial death (). The MBC showed the same trends as Dc values with respect to antimicrobial effectiveness for both NP and EO, against gram-positive L. monocytogenes and gram-negative strains of E. coli O157:H7 and S. typhimurium. The MBC values of NP and EO against the tested gram-positive L. monocytogenes and gram-negative strains of E. coli O157:H7 and S. typhimurium ranged from 850 to 15,000 and 600 to 1200 µg/mL, respectively. In general, the MBC values of NP and EO obtained in this study were slightly higher (particularly for NP) than the reported literature. The differences in values may be attributed to the different experimental methods, physical and chemical characteristics of NP and EO, species and strain of microorganism, as well as culture conditions that have been used to determine the antibacterial effectiveness of NP and EO.[Citation26,Citation27,Citation41,Citation43]
Table 2. Minimum bactericidal concentrations (MBC, µg/mL) of nanopowders and essential oils for selected microorganisms.
Several mechanisms of antibacterial action of NP and EO have been proposed in the literature; however, not fully elucidated. ZnO nanoparticles are believed to produce of reactive oxygen species (ROS; such as hydroxyl radicals, superoxides, and H2O2), release zinc ions (ZnCitation2+) or mechanically damage cell walls upon contact or interaction with bacterial cell leading its death.[Citation41] Cinnamon, garlic, and CLOs contain cinnamaldehyde, allicin, and eugenol, respectively, as major constituents which are responsible for providing the antibacterial activity. These major components have the ability to affect the enzyme activity (particularly those associated with energy production), cause protein denaturation and alter microbial cell wall permeability, thereby permitting the leakage of cell components leading to death.[Citation27,Citation44]
Selection of PLA-Based Films for Evaluation of Antibacterial Effectiveness
The inherent brittleness of PLA makes it necessary for the addition of plasticizers to improve their ductile properties and to get the flexibility required for casting of films. In this study PLA was blended with PEG as a plasticizer in order to improve its flexibility.[Citation8] Results showed that PLA/PEG (80/20) and PLA/PEG/NP (78/20/2) blend films appeared to be plasticized with more flexibility than those with different blend ratios and, therefore, those blends were selected in this study. Similarly, preliminary tests were performed with the addition of EO (<10% w/w based on PLA content) to PLA based films to obtain PLA/PEG/EO films. Results showed that these films were ineffective against test microorganisms. Thus, PLA/PEG/EO and PLA/PEG/EO/NP films prepared with higher oil concentrations were selected for evaluation of antibacterial effectiveness against the foodborne pathogens. Furthermore, a verification study with a selected PLA/PEG/EO film was performed against L. monocytogenes and S. typhimurium inoculated cheese samples for feasibility in food packaging applications.
Antibacterial Effectiveness of PLA-Based Films Incorporated with NP and EO
The zone of inhibition (ZoI) of the PLA-based films (PLA/PEG, PLA/PEG/NP, PLA/PEG/EO, and PLA/PEG/NP/EO) against the tested microorganisms are shown in . All PLA-based film containing nanoparticles did not show any antimicrobial effects against the tested bacterial pathogens since no ZoI were detected. The poor antibacterial effectiveness of NP in films was expected as they had been characterized with very low Dc and MBC values (in vitro studies). In addition, the hydrophobic nature of PLA enhanced the ineffectiveness of the films against bacteria. Rhim et al.[Citation45] reported PLA/nanoclay films were ineffective against most of the selected foodborne pathogens due to the hydrophobic nature of PLA. Similarly, Jin et al.[Citation46] investigated the antibacterial activity of hydrophobic polystyrene containing ZnO against L. monocytogenes and found there was no inhibition. Those authors opined that ZnO were tightly bound within the polystyrene film which prevented its release and expression for the antibacterial action. However, Raquez et al.[Citation10] found PLA/ZnO or PLA/Ag films were effective against both gram-positive and gram-negative bacteria. So, there was no consensus on antimicrobial activity of NP containing PLA films.
Table 3. Measured zones of inhibition (mm) of PLA based films against three foodborne pathogens.
Among oil-based PLA films, PLA/PEG/CIN100 and PLA/PEG/CLO100 films were effective against all the tested pathogens as evidenced by measuring the ZoI (). The ZoI ranged from 10 to 14 mm and 8 to 15 mm, respectively, and it is worth noting that gram-positive bacteria exhibited higher range of ZoI than gram-negative bacteria. The PLA/PEG/CIN100 film showed stronger inhibitory activity against E. coli O157:H7 compared to other two bacteria. These trends were similar to the results reported for in vitro studies. Most investigations have reported that the gram-positive bacteria to be more sensitive than the gram-negative ones while using different EOs in films.[Citation47–Citation50] Contrary to other two oil-based films, the PLA/PEG/GAR100 film was found to be active only against L. monocytogenes.
An attempt was made to reduce the EO content in the film and its effect on ZoI. CIN was chosen as the test oil for the film. It was observed that the ZoI reduced significantly from 10 to 1 mm when the CIN content reduced by 35%. The lower diffusion of oil into the media and the hydrophobic nature of PLA could be responsible factors for the partial or complete ineffectiveness of films (PLA/PEG/CIN10 and PLA/PEG/CIN50) with low concentration of oil. A low inhibitory effect of olive leaf extract incorporated PLA films against S. aureus has been reported by Erdohan et al.[Citation51] who argued that the antimicrobial ineffectiveness was due to less released amounts of olive leaf extracts from hydrophobic PLA film matrixes. Qin et al.[Citation30] prepared Poly(lactic acid)/poly(trimethylene carbonate/cinnamaldehyde films (PLA/PTMC/cinnamaldehyde) with 3, 6, 9 and 12 wt% cinnamaldehyde and showed that only the films containing 9 or 12 wt% cinnamaldehyde were significantly effective against both gram-positive S. aureus and gram-negative E. coli.
Furthermore, the synergistic effects of NP and EO against the three pathogens in PLA films were tested. ZoI values of PLA/PEG/ZnO100/CIN100 and PLA/PEG/Ag-Cu/CIN100 films were similar to the values that were obtained with PLA/PEG/CIN100 films indicating that there were no synergistic effects of NP and EO against the tested bacteria. Other than the hydrophobic nature of PLA and poor antimicrobial efficacy of NP factors including incorporation methods of NP and EO play an important role for producing films with antimicrobial properties. Recently, nanoclays (montmorillonite)/EO (carvacrol) were combined to produce novel hybrids and incorporated into hydrophobic low density polyethylene films to obtain films with excellent antimicrobial effectiveness against gram-positive and gram-negative bacteria.[Citation52,Citation53]
The antibacterial effectiveness of PLA-based films were also determined by the liquid culture tests as the agar diffusion method is a qualitative method and can only indicate the antibacterial activity of the film.[Citation28] The survival counts of the three foodborne pathogens in comparison to the control film after incubation in TSB for 24 h and 48 h is shown in . Their corresponding log reduction was also obtained by subtracting with the survivor counts of the control films and is depicted in .
TAble 4. Survivor counts (CFU/mL) and of three foodborne pathogens after 24 and 48 h of incubation of PLA based films in TSB.
Figure 3. Log reductions of food borne pathogens after 24 and 48 h incubation of PLA based films in TSB (A: L. monocytogenes; B: S. typhimurium; C: E. coli O157:H7).
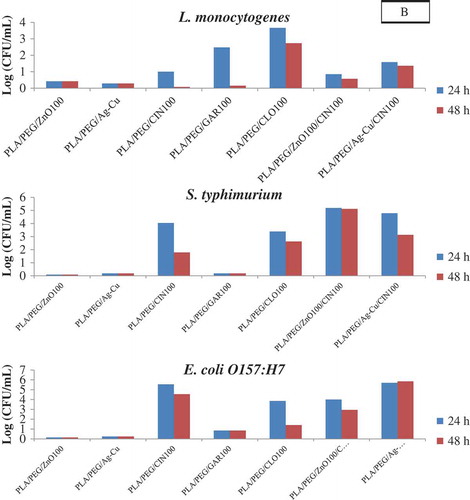
After 24 h of incubation, the PLA/PEG/ZnO100, PLA/PEG/ZnO50, and PLA/PEG/Ag-Cu films showed no reductions in the bacterial counts (<0.5 log reduction) when compared to the control films indicating that these films were ineffective against all the three test organisms.
PLA/PEG/CIN100, PLA/PEG/CLO100 films were effective against all the three pathogens, whereas the PLA/PEG/GAR100 film was effective only against the gram-positive L. monocytogenes. The counts of L. monocytogenes in TSB broth PLA/PEG/GAR100 films were 6.65 log CFU/mL after 24 h, 8.98 log CFU/mL after 48 h, while the control values were 9.23 log CFU/mL. The PLA/PEG/CIN100 films showed superior activity against both gram-negative pathogens (4–6 log reductions) when compared to the gram-positive pathogen (1 log reduction). After 48 h of incubation an increase in counts or decrease in log reductions were observed for the test strains indicating that the efficacy of the PLA/PEG/EO films decreased with incubation time. Similar patterns of reductions of test pathogens were also obtained in the case PLA/PEG/ZnO100/CIN100 and PLA/PEG/Ag-Cu/CIN100 as compared to PLA/PEG/CIN100 films indicating no synergistic effects of NP (ZnO100 or Ag-Cu) and cinnamon. Overall, the results were similar to the observations made by the agar diffusion method. Llana-Ruiz-Cabello et al.[Citation32] used both agar diffusion and liquid incubation methods to evaluate the antimicrobial activity for PLA films incorporated with 2, 5, and 6.5% Proallium® (Allium sativum EO) against several yeast, molds, and pathogenic bacteria (including L. monocytogenes, Salmonella enterica, and E. coli O157:H7). They observed no antimicrobial activity for any active films in the diffusion assay in contrast to the liquid incubation assay which showed that films containing 5 and 6.5% of Proallium® were able to significantly reduce the growth of all organisms. Thereby they suggested that complementary studies to the diffusion assay are required for confirming the antimicrobial activity of films.
Verification Test with Cheese
PLA/PEG/CIN100 film showed an excellent activity against both gram-negative and gram-positive pathogens, and therefore, it was selected for the challenge study. illustrates the growth of L. monocytogenes and S. typhimurium, respectively, on artificially contaminated cheese samples packaged in PLA/PEG/CIN100 films in comparison with samples packed in control (PLA/PEG) film. The initial bacterial load was 7 log CFU/g of cheese at day 0 for both samples. A decrease in bacterial concentration was noticed on day 2 which continued until day 11. The bacterial concentration reduced to 4.47 log CFU/g and 3.17 log CFU/g for L. monocytogenes and S. typhimurium, respectively, after 11 days of storage at 4°C. The microbial concentration in control sample also decreased, but the counts were higher. These results suggest that PLA/PEG/CIN100 induced an inhibition for both L. monocytogenes and S. typhimurium in cheese samples, and therefore, it demonstrated an in situ antimicrobial activity. Suppakul et al.[Citation54] showed a similar inhibitory effects of polyethylene-based films containing EO from basil against L. innocua contaminated cheddar cheese. Inhibitory effects of poly(lactic acid; PLA) cellulose nanocrystals (CNC) and oregano films against L. monocytogenes in mixed vegetables has also been reported.[Citation55]
Conclusion
In vitro studies indicated that both metal-based NP and EOs were effective against tested microorganisms; however, EO exhibited its superiority over nanoparticles. Following in vitro studies, no ZoI or log reductions of test organisms were detected for nanoparticle incorporated PLA films. PLA-based films formulated with cinnamon and CLOs showed excellent antibacterial efficacy against both gram-positive and gram-negative pathogens, whereas the GAR was effective against gram-positive pathogen only. Artificially contaminated cheese samples packed with PLA/PEG/CIN100 film showed inhibition against L. monocytogenes and S. typhimurium until 11 days of storage at 4°C. This indicates that plasticized PLA/EO films could be used to enhance the shelf life of food under refrigerated condition. However, further research is needed to evaluate the diffusion and release kinetics for the NP and EO from PLA films to ensure their suitability in food packaging applications.
Funding
The authors express their gratitude to the Kuwait Foundation for Advancement of Sciences (KFAS) and the Kuwait Institute for Scientific Research (KISR) for providing the grant for the research work (Grant number FB 087C).
Additional information
Funding
References
- Plackett, D.V.; Holm, V.K.; Johansen, P.; Ndoni, S.; Nielsen, P.V.; Sipilainen-Malm, T.; Södergård, A.; Verstichel, S. Characterization of L-Polylactide and L-Polylactide–Polycaprolactone Co-Polymer Films for Use in Cheese-Packaging Applications. Packaging Technology and Science 2006, 19(1), 1–24.
- Ahmed, J.; Varshney, S.K. Polylactides—Chemistry, Properties, and Green Packaging Technology: A Review. International Journal of Food Properties 2011, 14, 37–58.
- Zhang, L.; Gu, F.X.; Chan, J.M.; Wang, A.Z.; Langer, R.S.; Farokhzad, O.C. Nanoparticles in Medicine: Therapeutic Applications and Developments. Clinical Pharmacology & Therapeutics 2008, 83(5), 761–769.
- Auras, R.; Harte, B.; Selke, S. An Overview of Polylactides As Packaging Materials. Macromolecular Bioscience 2004, 4(9), 835–864.
- Sinclair, R.G. The Case for Polylactic Acid As a Commodity Packaging Plastic. Journal of Macromolecular Science, Part A 1996, 33(5), 585–597.
- Almenar, E.; Samsudin, H.; Auras, R.; Harte, J. Consumer Acceptance of Fresh Blueberries in Bio-Based Packages. Journal of the Science of Food and Agriculture 2010, 90(7), 1121–1128.
- Jin, T.; Zhang, H. Biodegradable Polylactic Acid Polymer with Nisin for Use in Antimicrobial Food Packaging. Journal of Food Science 2008, 73(3), M127–134.
- Ahmed, J.; Varshney, S.K.; Auras, R.; Hwang, S.W. Thermal and Rheological Properties of L-Polylactide/Polyethylene Glycol/Silicate Nanocomposites Films. Journal of Food Science 2010, 75(8), N97–N108.
- Jamshidian, M.; Tehrany, E.A.; Imran, M.; Jacquot, M.; Desobry, S. Poly-Lactic Acid: Production, Applications, Nanocomposites, and Release Studies. Comprehensive Reviews in Food Science and Food Safety 2010, 9(5), 552–571.
- Raquez, J.M.; Habibi, Y.; Murariu, M.; Dubois, P. Polylactide (PLA)-Based Nanocomposites. Progress in Polymer Science 2013, 38(10–11), 1504–1542.
- Tawakkal, I.; Cran, M.J.; Miltz, J.; Bigger, S.W. A Review of Poly(Lactic Acid)-Based Materials for Antimicrobial Packaging. Journal of Food Science 2014, 79(8), R1477–R1490.
- Duncan, T.V. Applications of Nanotechnology in Food Packaging and Food Safety: Barrier Materials, Antimicrobials, and Sensors. Journal of Colloid and Interface Science 2011, 363(1), 1–24.
- Llorens, A.; Lloret, E.; Picouet, P.A.; Trbojevich, R.; Fernandez, A. Metallic-Based Micro and Nanocomposites in Food Contact Materials and Active Food Packaging. Trends in Food Science & Technology 2012, 24(1), 19–29.
- Martinez-Abad, A.; Ocio, M.J.; Lagaron, J.M. Morphology, Physical Properties, Silver Release, and Antimicrobial Capacity of Ionic Silver-Loaded Poly(L-Lactide) Films of Interest in Food-Coating Applications. Journal of Applied Polymer Science 2014, 131(21). DOI: 10.1002/app.41001.
- Erem, A.D.; Ozcan, G.; Erem, H.H.; Skrifvars, M. Antimicrobial Activity of Poly(L-Lactide Acid)/Silver Nanocomposite Fibers. Textile Research Journal 2013, 83(20), 2111–2117.
- Doumbia, A.S.; Vezin, H.; Ferreira, M.; Campagne, C.; Devaux, E. Studies of Polylactide/Zinc Oxide Nanocomposites: Influence of Surface Treatment on Zinc Oxide Antibacterial Activities in Textile Nanocomposites. Journal of Applied Polymer Science 2015, 132(17). DOI: 10.1002/app.41776.
- Pantani, R.; Gorrasi, G.; Vigliotta, G.; Murariu, M.; Dubois, P. PLA-ZnO Nanocomposite Films: Water Vapor Barrier Properties and Specific End-Use Characteristics. European Polymer Journal 2013, 49(11), 3471–3482.
- Martinez-Abad, A.; Ocio, M.J.; Lagaron, J.M.; Sanchez, G. Evaluation of Silver-Infused Polylactide Films for Inactivation of Salmonella and Feline Calicivirus in Vitro and on Fresh-Cut Vegetables. International Journal of Food Microbiology 2013, 162(1), 89–94.
- Shameli, K.; Bin Ahmad, M.; Yunus, W.; Ibrahim, N.A.; Rahman, R.A.; Jokar, M.; Darroudi, M. Silver/Poly (Lactic Acid) Nanocomposites: Preparation, Characterization, and Antibacterial Activity. International Journal of Nanomedicine 2010, 5, 573–579.
- Tan, K.S.; Cheong, K.Y. Advances of Ag, Cu, and Ag-Cu alloy Nanoparticles Synthesized Via Chemical Reduction Route. Journal of Nanoparticle Research 2013, 15(4). DOI: 10.1007/s11051-013-1537-1.
- Taner, M.; Sayar, N.; Yulug, I.G.; Suzer, S. Synthesis, Characterization, and Antibacterial Investigation of Silver-Copper Nanoalloys. Journal of Materials Chemistry 2011, 21(35), 13150–13154.
- Zain, N.M.; Stapley, A.G.F.; Shama, G. Green Synthesis of Silver and Copper Nanoparticles Using Ascorbic Acid and Chitosan for Antimicrobial Applications. Carbohydrate Polymers 2014, 112, 195–202.
- Valodkar, M.; Modi, S.; Pal, A.; Thakore, S. Synthesis and Anti-Bacterial Activity of Cu, Ag, and Cu-Ag Alloy Nanoparticles: A Green Approach. Materials Research Bulletin 2011, 46(3), 384–389.
- Taner, M.; Sayar, N.; Yulug, I.G.; Suzer, S. Zn Prolongs the Stability of Antibacterial Silver‐Copper Nanoalloys. Biochemistry and Biophysics 2013, 1(4), 70–77.
- Sung, S.Y.; Sin, L.T.; Tee, T.T.; Bee, S.T.; Rahmat, A.R.; Rahman, W.; Tan, A.C.; Vikhraman, M. Antimicrobial Agents for Food Packaging Applications. Trends in Food Science & Technology 2013, 33(2), 110–123.
- Seow, Y.X.; Yeo, C.R.; Chung, H.L.; Yuk, H.G. Plant Essential Oils As Active Antimicrobial Agents. Critical Reviews in Food Science and Nutrition 2014, 54(5), 625–644.
- Burt, S. Essential Oils: Their Antibacterial Properties and Potential Applications in Foods—A Review. International Journal of Food Microbiology 2004, 94(3), 223–253.
- Tajkarimi, M.M.; Ibrahim, S.A.; Cliver, D.O. Antimicrobial Herb and Spice Compounds in Food. Food Control 2010, 21(9), 1199–1218.
- Kuorwel, K.K.; Cran, M.J.; Sonneveld, K.; Miltz, J.; Bigger, S.W. Essential Oils and Their Principal Constituents As Antimicrobial Agents for Synthetic Packaging Films. Journal of Food Science 2011, 76(9), R164–R177.
- Qin, Y.Y.; Yang, J.Y.; Xue, J. Characterization of Antimicrobial Poly(Lactic Acid)/Poly(Trimethylene Carbonate) Films with Cinnamaldehyde. Journal of Materials Science 2015, 50(3), 1150–1158.
- Qin, Y.Y.; Liu, D.; Wu, Y.; Yuan, M.L.; Li, L.; Yang, J.Y. Effect of PLA/PCL/cinnamaldehyde Antimicrobial Packaging on Physicochemical and Microbial Quality of Button Mushroom (Agaricus Bisporus). Postharvest Biology and Technology 2015, 99, 73–79.
- Llana-Ruiz-Cabello, M.; Pichardo, S.; Baños, A.; Núñez, C.; Bermúdez, J.M.; Guillamón, E.; Aucejo, S.; Cameán, A.M. Characterisation and Evaluation of PLA Films Containing An Extract of Allium spp. to Be Used in the Packaging of Ready-to-Eat Salads Under Controlled Atmospheres. LWT–Food Science and Technology 2015, 64(2), 1354–1361.
- NCCLS. National Committee for Clinical Lab Standards: Methods for Dilution and Antimicrobial Susceptibility Tests for Bacteria That Grow Aerobically. Approved Standard 2000, 20, M7–A5.
- Tayel, A.A.; El-Tras, W.F.; Moussa, S.; El-Baz, A.F.; Mahrous, H.; Salem, M.F.; Brimer, L. Antibacterial Actin of Zinc Oxide Nanoparticles Against Foodborne Pathogens. Journal of Food Safety 2011, 31(2), 211–218.
- Kim, J.M.; Marshall, M.R.; Wei, C. Antibacterial Activity of Some Essential Oil Components Against 5 Foodborne Pathogens. Journal of Agricultural Food Chemistry 1995, 43(11), 2839–2845.
- NCCLS. National Committee for Clinical Lab Standards: Methods for Determining Bactericidal Activity of Antimicrobial Agents. Approved Standard 1999, M26–A.
- Arfat, Y.A.; Benjakul, S.; Prodpran, T.; Sumpavapol, P.; Songtipya, P. Properties and Antimicrobial Activity of Fish Protein Isolate/Fish Skin Gelatin Film Containing Basil Leaf Essential Oil and Zinc Oxide Nanoparticles. Food Hydrocolloids 2014, 41, 265–273.
- NCCLS. National Committee for Clinical Lab Standards: Performance Standards for Antimicrobial Disk Susceptibility Tests. Approved Standard 2003, M2–A8.
- Pasquet, J.; Chevalier, Y.; Couval, E.; Bouvier, D.; Noizet, G.; Morliere, C.; Bolzinger, M.A. Antimicrobial Activity of Zinc Oxide Particles on Five Micro-Organisms of the Challenge Tests Related to Their Physicochemical Properties. Int J Pharm 2014, 460(1–2), 92–100.
- Dizaj, S.M.; Lotfipour, F.; Barzegar-Jalali, M.; Zarrintan, M.H.; Adibkia, K. Antimicrobial Activity of the Metals and Metal Oxide Nanoparticles. Materials Science & Engineering C-Materials for Biological Applications 2014, 44, 278–284.
- Shi, L.E.; Li, Z.H.; Zheng, W.; Zhao, Y.F.; Jin, Y.F.; Tang, Z.X. Synthesis, Antibacterial Activity, Antibacterial Mechanism, and Food Applications of Zno Nanoparticles: A Review. Food Additives and Contaminants Part A—Chemistry Analysis Control Exposure & Risk Assessment 2014, 31(2), 173–186.
- Kim, J.S.; Kuk, E.; Yu, K.N.; Kim, J.H.; Park, S.J.; Lee, H.J.; Kim, S.H.; Park, Y.K.; Park, Y.H.; Hwang, C.Y.; Kim, Y.K.; Lee, Y.S.; Jeong, D.H.; Cho, M.H. Antimicrobial Effects of Silver Nanoparticles. Nanomedicine-Nanotechnology Biology and Medicine 2007, 3(1), 95–101.
- Seil, J.T.; Webster, T.J. Antimicrobial Applications of Nanotechnology: Methods and Literature. International Journal of Nanomedicine 2012, 7, 2767–2781.
- Tiwari, B.K.; Valdramidis, V.P.; O’Donnell, C.P.; Muthukumarappan, K.; Bourke, P.; Cullen, P.J. Application of Natural Antimicrobials for Food Preservation. Journal of Agricultural Food Chemistry 2009, 57(14), 5987–6000.
- Rhim, J.W.; Hong, S.I.; Ha, C.S. Tensile, Water Vapor Barrier, and Antimicrobial Properties Of PLA/Nanoclay Composite Films. LWT–Food Science and Technology 2009, 42(2), 612–617.
- Jin, T.; Sun, D.; Su, J.Y.; Zhang, H.; Sue, H.J. Antimicrobial Efficacy of Zinc Oxide Quantum Dots Against Listeria Monocytogenes, Salmonella Enteritidis, and Escherichia Coli O157:H7. Journal of Food Science 2009, 74(1), M46–M52.
- Ramos, O.L.; Silva, S.I.; Soares, J.C.; Fernandes, J.C.; Pocas, M.F.; Pintado, M.E.; Malcata, F.X. Features and Performance of Edible Films, Obtained from Whey Protein Isolate Formulated with Antimicrobial Compounds. Food Research International 2012, 45(1), 351–361.
- Sung, S.Y.; Sin, L.T.; Tee, T.T.; Bee, S.T.; Rahmat, A.R. Effects of Allium Sativum Essence Oil As Antimicrobial Agent for Food Packaging Plastic Film. Innovative Food Science & Emerging Technologies 2014, 26, 406–414.
- Ramos, M.; Jimenez, A.; Peltzer, M.; Garrigos, M.C. Characterization and Antimicrobial Activity Studies of Polypropylene Films with Carvacrol and Thymol for Active Packaging. Journal of Food Engineering 2012, 109(3), 513–519.
- Torlak, E.; Sert, D. Antibacterial Effectiveness of Chitosan-Propolis Coated Polypropylene Films Against Foodborne Pathogens. Int J Biol Macromol 2013, 60, 52–55.
- Erdohan, Z.O.; Cam, B.; Turhan, K.N. Characterization of Antimicrobial Polylactic Acid Based Films. Journal of Food Engineering 2013, 119(2), 308–315.
- Shemesh, R.; Goldman, D.; Krepker, M.; Danin-Poleg, Y.; Kashi, Y.; Vaxman, A.; Segal, E. LDPE/Clay/Carvacrol Nanocomposites with Prolonged Antimicrobial Activity. Journal of Applied Polymer Science 2015, 132(2). DOI: 10.1002/app.41261.
- Shemesh, R.; Krepker, M.; Goldman, D.; Danin-Poleg, Y.; Kashi, Y.; Nitzan, N.; Vaxman, A.; Segal, E. Antibacterial and Antifungal LDPE Films for Active Packaging. Polymers for Advanced Technologies 2015, 26(1), 110–116.
- Suppakul, P.; Sonneveld, K.; Bigger, S.W.; Miltz, J. Efficacy of Polyethylene-Based Antimicrobial Films Containing Principal Constituents of Basil. LWT–Food Science and Technology 2008, 41(5), 779–788.
- Salmieri, S.; Islam, F.; Khan, R.; Hossain, F.; Ibrahim, H.M.; Miao, C.; Hamad, W.; Lacroix, M. Antimicrobial Nanocomposite Films Made of Poly(Lactic Acid)–Cellulose Nanocrystals (PLA–CNC) in Food Applications—Part B: Effect of Oregano Essential Oil Release on the Inactivation of Listeria Monocytogenes in Mixed Vegetables. Cellulose 2014, 21(6), 4271–4285.