ABSTRACT
Triglyceride composition and trans fatty acid content of corn oil subjected to microwave and conventional heating were evaluated with the aid of central composite design methodology. Oil samples with and without α-tocopherol were prepared on the basis of central composite design and, the effects of the main three factors (temperature, contact time, and the amount of α-tocopherol) were tired to investigate 10 at different five levels. Linoleic acid-linoleic acid-linoleic acid content in triglyceride structure of corn oil and the amount of trans fatty acids in the same oils were taken as response values for evaluation of central composite design assays. Based on the response surface, the optimum conditions (at which trans fatty acid formation and the change in the triglyceride composition are minimum) were determined for both heating procedures. The results showed that the increasing of the temperature and contact time increased the trans fatty acid formation and changed triglyceride structure of the oil in both heating techniques, although, the addition of α-tocopherol at high concentration caused pro-oxidant effect on triglyceride composition (bad effect), while reducing the formation of trans fatty acid (good effect). The results revealed that the heating 20 treatments caused significant changes in the triglyceride composition and trans fatty acid isomers of unsaturated fatty acids of the oil, while the saturated fatty acid contents did not change substantially. These alterations were much greater in microwave-heated samples (trans fatty acid ≤ 7.26%) than in Q3 corresponding samples heated in a conventional stove (trans fatty acid ≤ 0.56%).
Introduction
Today, different cooking processes such as hot pot (conventional; C), microwave (MW), barbecue, frying, and patty cooking involve the use of a wide variety of food and cooking oils. The most common of these processes are C and MW cooking methods. The C cooking method is the most preferred technique and can cause different chemical changes in the edible oil. Over the last 20 years, MW ovens have used in home and industry for baking, blanching, cooking, drying, pasteurization, sterilization, and thawing of various food products, because of their unique heating ability, offers many advantages, convenience, rapidity, and economy.[Citation1–Citation3] Although MW ovens have some advantages, they have also some disadvantages such as formation of free radicals, free fatty acids, and trans isomer in the edible oil.[Citation3–Citation5] In MW heating, foods are exposed to electromagnetic energy with the molecular constituents of food for heat transmission.[Citation6]
Edible oils which have predominantly unsaturated fatty acids are oxidized and their fatty acids in the form of cis were transformed to another structures (trans and conjugated fatty acid, etc.) under some condition such as heat, light, air, photosensitizing agents, free radicals, moisture, and heavy metals.[Citation7–Citation11] Oxidation products cause decrease in oxidative stability, quality and shelf life, loss of organoleptic characteristics as color, taste, and odor.[Citation7,Citation12] At the same time, oxidation products might causes cancer and effect of cytotoxicity and atherogenicity, hypertension.[Citation7,Citation9,Citation12–Citation14] Trans fatty acids (TFAs) are also dangerous for health in terms of they have higher melting point, more stable, higher total plasma and raise low density lipoprotein (LDL) cholesterol levels and lower high density lipoprotein (HDL) cholesterol levels,[Citation15] contribute to the risk of myocardial infarction.[Citation16] Some works reported that peroxide value, conjugated dienes (CD) and conjugated trienes (CT), p-anisidine value (p-AV), free fatty acids, and trans-isomer ratio[Citation1,Citation17–Citation19] was increased with the increasing of time and temperature for the oils heated by MW and C procedures. Besides, degree of oxidation is related to unsaturated fatty acid ratio. Higher unsaturated fatty acid ratio is increased the formation of the oxidized products.[Citation3,Citation18]
Tocopherols and tocotrienols, together abbreviated as tocols and summarized under the term vitamin E, are a group of fat soluble antioxidants with a chromanol ring and a hydrophobic side chain (phytyl in the case of tocopherols, isoprenyl in the case of tocotrienols).[Citation20,Citation21] Tocopherols and tocotrienols have different antioxidant and biological activities, such as reduce the risk of cardiovascular diseases and of certain types of cancer,[Citation20,Citation22–Citation24] decreasing platelet aggregation, LDL oxidation, and delaying intra-arterial thrombus formation,[Citation20,Citation25] inhibiting lipid peroxidation in biological membranes,[Citation26,Citation27] breaking free radical chain reactions and provides some natural oxidative protection to the oils,[Citation28,Citation29] and contribute to their nutritional value.[Citation5] Some works reported that MW and convectional heating decreased tocopherols with increasing of time and temperature.[Citation3,Citation5]
The experimental design techniques are used in biological studies, process analysis and modeling are an alternative and more efficient approach.[Citation30,Citation31] The central composite design (CCD), which belongs to experimental design techniques, is used for multivariable systems such as method optimization and development, multivariable analysis and method condition.[Citation30,Citation32–Citation34] CCD has many advantages over the classical “one factor at a time” experiments because the factors involved in an experiment are being simultaneously changed, it requires fewer experiments than a full factorial design.[Citation32,Citation33] CCD gives almost as much information as a multilevel factorial, and thus CCD methodology can be easily used for identification of quality parameters in edible oils.[Citation30,Citation31,Citation35]
The aim of this study was to investigate the effects of the MW and C heating procedures on the TGC and TFA content of corn oil with the aid of CCD methodology. In order to understand the impacts of two heating procedures in the changes of the oil structure, three factors (temperature, contact time, and the amount of α-tocopherol) were evaluated and chemometric protocols of the response surface design were successfully employed.
Materials and methods
Chemicals and reagents
All chemicals and solvents were of high-performance liquid chromatography (HPLC) and gas chromatography (GC) grade and obtained from Merck (Darmstadt, Germany) and VWR International (USA). Corn oil sample was obtained from local market in Konya, Turkey.
Experimental design
CCD methodology with three factors including temperature, contact time, and the amount of α-tocopherol and five levels (–1.68, –1, 0, +1, +1.68) was applied for investigation of the MW and C heating techniques on corn oil. The CCD was constructed as in . and the actual design experiments were listed in . The total of 23 experiments were conducted, and response values were taken as the mean values of three results (relative standard deviation < 0.5%). The trial version 9 of the Design Expert software (Stat-Ease Corporation, USA) was utilized for the analysis of the CCD experiments.
Table 1. Experimental design scheme for optimization studies.
Table 2. CCD for the three independent variables.
MW and C heating procedure
Oil samples (25.0 ± 0.1 g) containing the different amounts of α-tocopherol according to the CCD ( and ) were weighted in glass flask for each process. The MW oven with rotating glass tray 27 cm was purchased from BEKO (MD 1510 model 1200 W manufactured Turkey). Samples were placed at equal distances on a rotary plate of the MW oven and heated at different times and levels according to the CCD ( and ). The same processes were carried out in a C heating on electrical convection heater (IKAMAG RCT Basic, IKA Labortechnik, Germany) having temperature controlled with thermocouple. Samples were placed on plate center of the heater and heated at different times and temperatures according to the CCD ( and ). After the heating process, the oil samples were cooled immediately, stored in amber glass flask at 4°C and brought to room temperature before use.
Analysis
HPLC conditions for triglyceride analysis
HPLC system was consisted of a 1200 series Agilent Technologies G1311A model quaternary pump, a G1315B model diode array detector (DAD), a G1329A model automatic injector system, a G1316A model thermostated column compartment. Data were recorded by a Chemstation B.03.02-2008 data processor (Agilent Technologies Inc., Wilmington, DE, USA). The triglycerides content were determined by direct injection of the oil samples into HPLC system. For this purpose, 1 g of oil was dissolved in 10 mL acetone, and 20 μL aliquot of it was injected into ACE 5 C18 column (250 × 4 mm, 5 µm particle size, Advanced Chromatography Technologies., Aberdeen, Scotland). A mixture of acetonitrile and acetone (1:1, v/v) was used as a mobile phase at a flow rate of 1.5 mL min–1 by an isocratic elution.[Citation36] The temperatures of column and detector were kept at 25°C. Obtained peaks were detected using a DAD detector set at wavelength of 215 nm. Triglyceride kits were purchased from Sigma-Aldrich (TRI19-1KT, Supelco® Triglycerides Kit, USA). Triglyceride composition (TGC) was identified by comparison of their retention times with those of authentic standards. All standard solutions were prepared weekly and stored in the dark at 0°C.
Preparation of fatty acid methyl esters (FAME)
Corn oil samples were converted to their respective methyl esters by base-catalyzed procedure.[Citation37–Citation39] As per protocol of procedure, 1 g of oil samples was methylated with 1 mL of 2 N methanolic KOH for 10 min at room temperature. Methyl esters were extracted with 7 mL of hexane. After centrifugation (4000 rpm/min), hexane layer containing FAME was collected and injected to GC.[Citation37,Citation38]
GC conditions for FAME analysis
In the present study, Agilent 7890 Series GC-flame ionization detector (FID) fitted with HP-88 column (100 m, 0.25 mm, 0.25 µm, Agilent Technologies, ABD) was used for fatty acid composition (FAC) analysis of corn oil samples. Helium was used as carrier gas. The temperature of the injection port and detector were maintained at 250°C. The oven temperature was programmed to initiate at 50°C for 10 min, then the temperature was raised to 240°C at a rate of 4°C min–1, held there for 10 min. The FAME dissolved in hexane was injected (1 μL) in a split mode of injection at a split ratio of 100:1. Flow rates of H2 and air were adjusted to 30 and 300 mL min−1, respectively. Chromatograms were recorded using Agilent 1200 Series-B.03.02 software program. Identification of the individual FA was accomplished by comparing GC retention time with that of the authorized pure commercial standards of Supelco 37-Component FAME Mix. Area under each FA peak relative to the total area of all FA peaks was used to quantify the FAs identified and the obtained results are reported as percentage of FA.
Results and discussions
CCD
CCD that developed by Box and Wilson[Citation40] is widely used on experimental design to investigated the main influences of different factors with minimum experimental runs. In this study, CCD concluding 23 experimental points (see ) which were much fewer experiments than a full factorial design was used for investigation of the effects of MW and C heating techniques on corn oil. For this purpose, the combined effects of the temperature, contact time and, the amount of α-tocopherol taken as factors were examined in the experiments. Linoleic acid-linoleic acid-linoleic acid (LLL) content present in the corn oil as the most abundant and the amount of TFA were taken as response values for CCD evaluation. After conducting of all experiments, the best fitting model was determined via regression. The information obtained from Design Expert software program showed that our results were fitted to quadratic polynomial model. When considering the relation between the response and the variables described, quadratic polynomial model was adapted as in the following equation.[Citation41]
where Y represents the response value, β0 is a constant, βi, βii, and βij are the linear, quadratic, and interactive coefficients, respectively.
Evaluation of the TGC and TFA content for MW heating
The final estimative response model equation based on the actual value by which the effects of MW heating parameters were predicted for LLL (Eq. [Citation2]) and total TFA content (Eq. [Citation3]), as follows:
By Eqs. (2) and (3), the response surfaces modeling of LLL% and total TFA% were created as the three-dimension (3D) and normal plot of the residuals and shown in and , respectively. The 3D plots indicate the interaction between the factors and responses of LLL% and total TFA% for MW heating.
Figure 1. The tree-dimension response surface plots (3D) and normal plot of the residuals of percentage of LLL (Y) for microwave heating, X1: temperature; X2: the amount of α-tocopherol; X3: contact time.
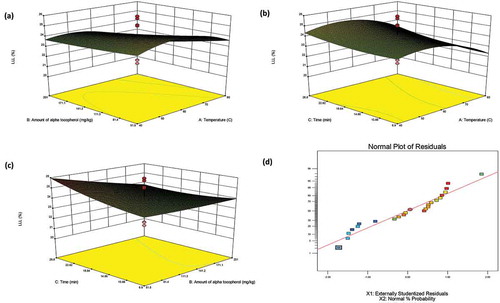
Figure 2. The tree-dimension response surface plots (3D) and normal plot of the residuals of total trans fatty acid (Y) for microwave heating, X1: temperature; X2: the amount of α-tocopherol; X3: contact time.
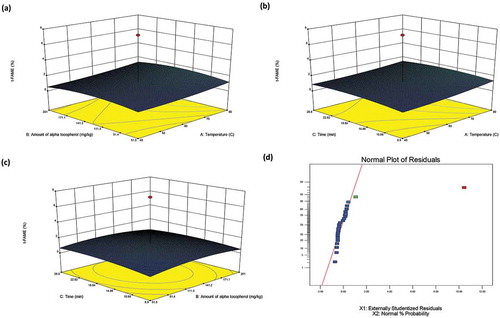
It can be seen from and , the samples including more α-tocopherol were found to have lower LLL% content in MW heating experiments. Although, LLL% decreased for long-term heating procedures throughout the contact time, it did not change for samples containing less α-tocopherol with contact time ( and ). This means that decrease of the LLL% was due to the pro-oxidant effect of α-tocopherol at high concentrations in foods and oils,[Citation42] and hydrolysis of triglycerides and their fatty acids. Also, temperature did not affect the changing of the LLL% of oil.
As for total TFA content, it also decreased when α-tocopherol increased in the sample while it increased at high temperatures in MW heating procedures ( and ). Also, the increasing of the contact time increased the formation of TFAs. (). Here, the most remarkable point is that the addition of the α-tocopherol demonstrated a supportive impact with the reducing capability of TFA formation, in contrast that of the triglyceride analysis.
Evaluation of the TGC and TFA content for convectional heating
Similar to the MW heating procedure, the final conjectural response model equation based on the actual value by which the effects of C heating parameters were predicted for LLL (Eq. [Citation4]) and total TFA content (Eq. [Citation5]), as follows:
By Eqs. (4) and (5), the response surfaces modeling of LLL% and total TFA% were created as the 3D and normal plot of the residuals and shown in and respectively. The 3D plots indicate the interaction between the factors and responses of LLL% and total TFA% for convectional heating.
Figure 3. The tree-dimension response surface plots (3D) and normal plot of the residuals of percentage of LLL (Y) for conventional heating, X1: temperature; X2: the amount of α-tocopherol; X3: contact time.
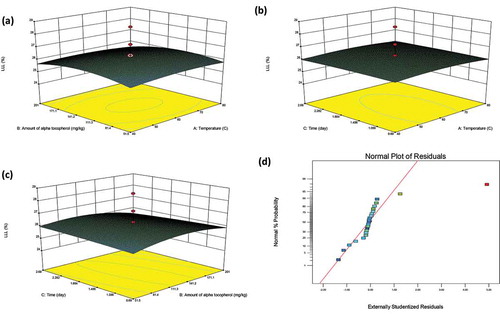
It can be seen from , the samples added more α-tocopherol had a lower LLL% content due to the prooxidan effect of the tocopherol. However, LLL% did not change significantly for samples subjected to longer heating contact time ( and ). Total TFA content decreased with the more addition of α-tocopherol ( and ), whereas it increased when the contact time was increased and heating temperature was decreased ().
Comparison of the MW and C heating techniques
The TGC results obtained for MW and C heating experiments are shown in and respectively. The data proved once again that LLL, OLL, and PLL are major triglyceride components for corn oil.[Citation43,Citation44] Therefore, for both heating procedures, the biggest variation in the triglyceride structures occurred in the linoleic acid parts which are the major constituent of corn oil. However, the significant changes were not observed for other triglyceride components which don`t contain linoleic acid such as OOO and POP.
Table 3. Triglyceride composition (%) values for microwave heating experiments of corn oil.
Table 4. Triglyceride composition (%) values for conventional heating experiments of corn oil.
For MW heating experiments, the highest variation in LLL, OLL, and PLL contents was observed for MW11 sample which was subjected to the highest temperature, whereas the significant changes were not observed for MW10 sample which was subjected to the lowest temperature. At the same time LLL, OLL, and PLL contents did not change significantly experiments for MW13 and MW15 samples that had the highest α-tocopherol content and contact time, respectively. Similar results were obtained for repeat experiments (9 and 16–23) performed under the same conditions.
For C heating experiments, except for LLL, OLL, and PLL contents, any significant changes in the triglycerides components was not observed. The highest variation in LLL, OLL, and PLL contents was observed for C12 sample which had the lowest α-tocopherol content, whereas the limited changes were monitored for C13 sample which had the highest α-tocopherol content. Beside, LLL, OLL and PLL contents did not significantly change for C11 sample that subjected to the highest temperature. For repeat experiments which are performed under the same conditions, close results were also obtained (9 and 16–23).
As stated previously, while the most effective factor for MW heating procedure was temperature; the addition of α-tocopherol into oil samples was the most influential factor for C heating procedures in determination of the TGC. These results are due to the effect of the MW energy. This energy potentiates the effects of the temperature, because the heating is made as a consequence, mainly, of the internal friction of the molecules.[Citation45] Therefore, temperature rise is very fast in MW ovens and generated heat can easily permeate into the food. The data obtained by the researchers showed that severe the hydrolytic and oxidative degradations took place in the oil during the MW heating.[Citation3,Citation6] Since oil oxidation involves free radicals, this heating process could accelerate all of the oxidative reactions at a higher rate than C oven heating or frying processes. For this reason, temperature showed a predominant effect and located in front of the other factors in MW heating procedure.[Citation46]
The FAC results obtained for MW and C heating experiments are shown in and , respectively. As can be seen from the data, linoleic, oleic, palmitic, and stearic acids were found to be major fatty acids for corn oil.[Citation47,Citation48] When comparing the FAC data for two different heating procedures, while the significant change was observed in the FAC for MW heating, it was not observed for convectional heating. The changes observed in the MW heating were occurred on unsaturated fatty acids, particularly in the percentage of the linoleic acid.
Table 5. Fatty acid composition (%) values for microwave heating experiments of corn oil.
Table 6. Fatty acid composition (%) values for conventional heating experiments of corn oil.
The MW heating experiments revealed that maximum TFA formation was observed for the samples of MW11 (7.26%) and MW8 (3.41%) which subjected to the higher heating temperature. TFA content was also found to be more for the samples of MW15 (%1.08) treated in long contact time. However, TFAs formation in the sample of MW3 (0.28%) containing high amount of α-tocopherol was decreased. The majority of the total TFAs consist from trans linoleic acid because of the linoleic acid is the major fatty acids of corn oil.
As for C heating experiments, total TFA content was increased at higher temperature and longer contact time such as the experiment of C11 (0.56%) and C15 (0.48%), although the total TFA content was decreased with the increasing of the amount of added α-tocopherol such as the experiment of C13 (0.35%). When considering the results obtained from two heating procedures, the TFA formation was found to be more for oil samples heated at high temperature and long contact time while it was determined lower for the samples containing high α-tocopherol. But of course, TFA formation has been much more in the MW heating procedure (7.26%) compared to the C heating procedure (0.56 %)[Citation1,Citation49] due to the effect of the MW energy.[Citation4,Citation6]
Conclusion
In this study, the effects of the MW and C heating techniques on the changing of the TGC and the formation of TFAs in corn oil samples were investigated via CCD methodology. The results of the CCD experiments examining the effect of three factors (temperature, contact time, and the amount of α-tocopherol) showed that the effectiveness of the selected factors varied depending on the type of heating procedure. Namely, for the change in the TGC of corn oil the most effective factor was determined as temperature in the MW heating procedure, while the addition of α-tocopherol into oil samples was the most effective factor in C heating procedures. For the change in the TFA content of corn oil all factors were found to be highly effective for both heating procedures, however the high temperature and contact time caused the increasing of the TFAs formation, while the addition of the high amount of α-tocopherol significantly inhibited this formation. Also, the increase of TFAs content was observed much more in MW heating procedure compared to the C heating procedure. As a conclusion, this study demonstrated that CCD methodology can be successfully used for modeling the some operating parameters of heating procedure for vegetable oil because of the good way of obtaining the useful information in a short period of time and with the minimum number of the experiments.
Funding
This study was supported by Selcuk University Coordinators of Scientific Research. The authors wish to thank the principal of Selcuk University and Scientific Research Projects Coordination.
References
- Caponio, F.; Pasqualone, A.; Gomes, T. Changes in the Fatty Acid Composition of Vegetable Oils in Model Doughs Submitted to Conventional ir Microwave Heating. International Journal of Food Science Technology 2003, 38, 481–486.
- Tan, C.; Man, Y.C.; Jinap, S.; Yusoff, M. Effects of Microwave Heating on Changes in Chemical and Thermal Properties of Vegetable Oils. Journal of the American Oil Chemists’ Society 2001, 78, 1227–1232.
- Yoshida, H.; Hirakawa, Y.; Abe, S.; Mizushina, Y. The Content of Tocopherols and Oxidative Quality of Oils Prepared from Sunflower (Helianthus Annuus L.) Seeds Roasted in a Microwave Oven. European Journal of Lipid Science and Technology 2002, 104, 116–122.
- Mahmoud, A.E.E.D.J.; Pokorný, J.; Lukešová, D.; Doležal, M. Oxidation of Olive Oils During Microwave and Conventional Heating for Fast Food Preparation. Czech Journal of Food Sciences. Food Chemistry 2009, 27, 173–177.
- Malheiro, R.; Oliveira, I.; Vilas-Boas, M.; Falcão, S.; Bento, A.; Pereira, J.A. Effect of Microwave Heating with Different Exposure Times on Physical and Chemical Parameters of Olive Oil. Food Chemistry 2009, 47, 92–97.
- El-Abassy, R.M.; Donfack, P.; Materny, A., Assessment of Microwave versus Conventional Heating Induced Degradation of Olive Oil by VIS Raman Spectroscopy and Classical Methods. In Advances in Induction and Microwave Heating of Mineral and Organic Materials, Grundas, S.; Ed.; Intech: Germany, 2011, 585–597.
- Erkan, N.; Ayranci, G.; Ayranci, E. A Kinetic Study of Oxidation Development in Sunflower Oil Under Microwave Heating: Effect of Natural Antioxidants. Food Research International 2009, 42, 1171–1177.
- Isabei, P.M.M. Study of the Thermal Stability of Edible Vegetable Oils in Different Environments. Afinidad, 2001, 58, 190–196.
- Naz, S.; Sheikh, H.; Siddiqi, R.; Sayeed, S.A. Oxidative Stability of Olive, Corn, and Soybean Oil Under Different Conditions. Food Chemistry 2004, 88, 253–259.
- Matalgyto, F.S.; Al-Khalifa, A.S. Effect of Microwave Oven Heating on Stability of Some Oils and Fats. Arab Gulf Journal of Scientific Research 1998, 16, 431–450.
- Fukumoto, Y.; Iibuchi, S. Temperature Dependency of Rate Constants of Edible Oil’s Autoxidation. Nippon Nogeikagaku Kaishi-Journal of the Japan Society for Bioscience Biotechnology and Agrochemistry 2000, 74, 769–773.
- Azeez, O.T.E.K.O.; Frank, E.O.; Gerald, N.E. Effects of Antioxidants on the Oxidative Stability of Vegetable Oil at Elevated Temperature. International Journal of Applied Science and Technology 2013, 3, 107–115.
- Carla, R.O.O.; Domenico, G.; Giovanni, F.; Zenari, M.L.; Sara, L.; Roberto, C. Antioxidant Status and Lipid Peroxidation in Patients with Essential Hypertension. Journal of Hypertension 1998, 16, 1267–1271.
- White, P.J. Flavor Quality of Fats and Oils. In Introduction to Fats and Oils Technology, 2nd Ed; O’Brien, R.D.; Farr, W.E.; Wan, P.J.; Eds.; AOCS Press: Champaign, IL, 2000; 341–370.
- Mensink, R.P.; Katan, M.B. Effect of Dietary trans Fatty Acids on High-Density and Low-Density Lipoprotein Cholesterol Levels in Healthy Subjects. The New England Journal of Medicine, 1990, 323, 439–445.
- Ascherio, A.; Hennekens, C.H.; Buring, J.E.; Master, C.; Stampfer, M.J.; Willett, W. Trans-Fatty Acids Intake and Risk of Myocardial Infarction. Circulation 1994, 89, 94–101.
- Poiana, M.A. Enhancing Oxidative Stability of Sunflower Oil During Convective and Microwave Heating Using Grape Seed Extract. International Journal of Molecular Sciences 2012, 13, 9240–9259.
- Hassanein, M.M.; El-Shami, S.M.; El-Mallah, M.H. Changes Occurring in Vegetable Oils Composition Due to Microwave Heating. Grasas Aceites 2003, 54, 343–349.
- Jittrepotch, N.K.T.; Rojsuntornkitti, K. Influence of Microwave Irradiation on Lipid Oxidation and Acceptance in Peanut (Arachis Hypogaeal.) Seeds. International Food Research Journal 2010, 17, 173–179.
- Schwartz, H.; Ollilainen, V.; Piironen, V.; Lampi, A.-M. Tocopherol, Tocotrienol, and Plant Sterol Contents of Vegetable Oils and Industrial Fats. Journal of Food Composition and Analysis 2008, 21, 152–161.
- Ayyildiz, H.F.; Topkafa, M.; Kara, H.; Sherazi, S.T.H. Evaluation of Fatty Acid Composition, Tocols Profile, and Oxidative Stability of Some Fully Refined Edible Oils. International Journal of Food Properties 2015, 18, 2064–2076.
- Burton, G.W. Vitamin-E—Molecular and Biological Function. Proceedings of the Nutrition Society 1994, 53, 251–262.
- Burton, G.W.; Traber, M.G. Vitamin-E—Antioxidant Activity, Biokinetics, and Bioavailability. Annual Review of Nutrition 1990, 10, 357–382.
- Zhou, X.; Zhou, M.; Liu, Y.; Ye, Q.; Gu, J.; Luo, G. Isolation and Identification of Antioxidant Compounds from Gynura Bicolor Stems and Leaves. Internation Journal of Food Properties 2016, 19, 233–241.
- Li, D.S., T.; Romeo, F.; Mehta, J.L. Relative Effects of Alpha- and Gamma-Tocopherol on Low-Density Lipoprotein Oxidation and Superoxide Dismutase and Nitric Oxide Synthase Activity and Protein Expression in Rats. Journal of Cardiovascular Pharmacology and Therapeutics 1999, 4, 219–226.
- Amaral, J.S.; Casal, S.; Torres, D.; Seabra, R.M.; Oliveira, B.P.P. Simultaneous Determination of Tocopherols and Tocotrienols in Hazelnuts by a Normal Phase Liquid Chromatographic Method. Analytical Sciences 2005, 21, 1545–1548.
- Bruscatto, M.H.; Zambiazi, R.C.; Sganzerla, M.; Pestana, V.R.; Otero, D.; Lima, R.; Paiva, F. Degradation of Tocopherols in Rice Bran Oil Submitted to Heating at Different Temperatures. Journal of Chromatographic Science, 2009, 47, 762–765.
- Sanagi, M.M.; See, H.; Ibrahim, W.A.W.; Naim, A.A. Determination of Carotene, Tocopherols, and Tocotrienols in Residue Oil from Palm Pressed Fiber Using Pressurized Liquid Extraction-Normal Phase Liquid Chromatography. Analytica Chimica Acta 2005, 538, 71–76.
- Vieira, T.M.; Regitano-D’arce, M.A. Canola Oil Thermal Oxidation During Oven Test and Microwave Heating. LWT–Food Science and Technology 2001, 34, 215–221.
- Zhang, X.; Wang, R.; Yang, X.; Yu, J. Central Composite Experimental Design Applied to the Catalytic Aromatization of Isophorone to 3,5-Xylenol. Chemometrics and Intelligent Laboratory Systems 2007, 89, 45–50.
- Muthukumar, M.; Sargunamani, D.; Selvakumar, N.; Venkata Rao, J. Optimisation of Ozone Treatment for Colour and COD Removal of Acid Dye Effluent Using Central Composite Design Experiment. Dyes and Pigments 2004, 63, 127–134.
- Box, G.E.P.; Hunter, J.S. Multifactor Experimental Designs for Exploring Responses Surfaces. The Annals of Mathematical Statistics, 1957, 28 (1), 195–241.
- Hashemi, P.; Raeisi, F.; Ghiasvand, A.R.; Rahimi, A. Reversed-Phase Dispersive Liquid-Liquid Microextraction with Central Composite Design Optimization for Preconcentration and HPLC Determination of Oleuropein. Talanta, 2010, 80, 1926–1931.
- Kumar, R.; Kumar, S.; Sinha, V. Evaluation and Optimization of Water in Oil Microemulsion Using Ternary Phase Diagram and Central Composite Design. Journal of Dispersion Science and Technology, 2016, 37 (2), 166–172.
- Irandoust, M.; Shariati-Rad, M.; Haghighi, M. Nitrite Determination in Water Samples Based on a Modified Griess Reaction and Central Composite Design. Analytical Methods 2013, 5, 5977–5982.
- AOCS. Official Methods and Recommended Practices of the American Oil Chemists’ Society, 4th Ed; AOCS Press: Champaign, IL, 1998.
- Suzuki, R.; Noguchi, R.; Ota, T.; Abe, M.; Miyashita, K.; Kawada, T. Cytotoxic Effect of Conjugated Trienoic Fatty Acids on Mouse Tumor and Human Monocytic Leukemia Cells. Lipids 2001, 36, 477–482.
- Wetherilt, H.; Pala, M. Spices, Herbs, and Edible Fungi, Charalambous, G.; Ed.; Elsevier Science Publishers: BV, Amsterdam, Netherlands, 1994; 285–307.
- Topkafa, M. Investigation of Refining Properties for Edible Pomegranate Seed Oil, Doctor of Philosophy, Selçuk University, 2013.
- Box, G.E.; Wilson, K. On the Experimental Attainment of Optimum Conditions. Journal of the Royal Statistical Society. Series B (Methodological) 1951, 13, 1–45.
- Tarhan, İ.; Kara, H. A Chemometric Study: Automated Flow Injection Analysis Method for the Quantitative Determination of Humic Acid in Ilgın Lignite. Arabian Journal of Chemistry, in press, DOI:10.1016/j.arabjc.2014.09.002
- Mukai, K.; Sawada, K.; Kohno, Y.; Terao, J. Kinetic Study of the Prooxidant Effect of Tocopherol. Hydrogen Abstraction From Lipid Hydroperoxides by Tocopheroxyls in Solution. Lipids 1993, 28, 747–752.
- Van Der Klift, E.J.C.; Vivó-Truyols, G.; Claassen, F.W.; Van Holthoon, F.L.; Van Beek, T.A. Comprehensive Two-Dimensional Liquid Chromatography with Ultraviolet, Evaporative Light Scattering and Mass Spectrometric Detection of Triacylglycerols in Corn Oil. Journal of Chromatography 2008, 1178, 43–55.
- Neff, W.E.; List, G.R.; Byrdwell, W.C. Effect of Triacylglycerol Composition on Functionality of Margarine Basestocks. LWT–Food Science and Technology 1999, 32, 416–424.
- Schiffmann, R.F. Microwave technology in baking. In Advances in Baking Technology, Kamel, B.; Stauffer, C.; Eds.; Blackie Academic & Professional: London, 1993; pp. 292–315.
- Albi, T.; Lanzón, A.; Guinda, A.; León, M.; Pérez-Camino, M.C. Microwave and Conventional Heating Effects on Thermoxidative Degradation of Edible Fats. Journal of Agricultural and Food Chemistry 1997, 45, 3795–3798.
- Mondello, L.; Tranchida, P.Q.; Costa, R.; Casilli, A.; Dugo, P.; Cotroneo, A.; Dugo, G. Fast GC for the Analysis of Fats and Oils. Journal of Separation Science 2003, 26, 1467–1473.
- Lay, J.O.; Liyanage, R.; Durham, B.; Brooks, J. Rapid Characterization of Edible Oils By Direct Matrix-Assisted Laser Desorption/Ionization Time-of-Flight Mass Spectrometry Analysis Using Triacylglycerols. Rapid Communications in Mass Spectrometry 2006, 20, 952–958.
- Daglioglu, O.; Tasan, M.; Tuncel, B. Effects of Microwave and Conventional Baking on the Oxidative Stability and Fatty Acid Composition of Puff Pastry. Journal of the American Oil Chemists’ Society 2000, 77, 543–545.