ABSTRACT
In the present study, a total of used six lactic acid bacterial strains that isolated from children’s feces and milk products. This study investigated the exopolysacch aride production of these strains. In the culture broth, highest exopolysaccharide production capacity was detected in GD-11, LB-69, and B-3 strains. Monosaccharide compositions of the exopolysaccharides that were purified and lyophilized in the culture medium were investigated and dominant monomer was found to be mannose in all strains. The biofilm-forming capacity of 13 pathogen bacteria was determined and four strains with the highest biofilm-forming capacity were selected as test bacteria for anti-biofilm studies. Different concentrations of l-exopolysaccharides of three lactic acid bacteria were tested for anti-biofilm effect. Highest anti-biofilm effects were exhibited by the L. fermentum LB-69 (90%) against B. cereus RSKK 863. In the statistical analyses performed using the available data, a strong correlation was found between the amount of mannose present in l-exopolysaccharides and the anti-biofilm activity exhibited by l-exopolysaccharides (p < 0.01). In this study, it was concluded that the l-exopolysaccharides of the L. fermentum LB-69 strain could be used as efficient agents with both bifidogenic growth stimulator effect and anti-biofilm effect. If these agents are taken via oral route, it would be possible to provide beneficial effects for the host by increasing the development of bifidobacteria present in the gastrointestinal tract as well as to prevent and treat the diseases by hindering the biofilm formation by harmful bacteria.
Introduction
Bacteria from the lactic acid bacteria (LAB) group have mostly been used in the production and protection of foods. Some species are acknowledged as probiotics, which according to the definition recommended by the World Health Organization (WHO) meet a health benefit on the host when leaded in satisfactory amounts.[Citation1,Citation2] In addition LAB are identified as generally recognized as safe (GRAS) foods by the Food Drug Administration (FDA). As the resident microbiota of the intestinal track the microorganisms that are closely related to human beings.[Citation3]
Exopolysaccharides (EPSs) are long-chain high-molecular-mass polymers that are primarily produced by the metabolic pathways of LAB.[Citation4] In LAB, many species have been indicated to produce EPS molecules. The diversity of the produced molecules is huge in terms of monomer composition, molecular mass, degree of branching, and structure.[Citation5] The EPS can also consist in different forms. Many probiotic bacteria (FAO/WHO, 2002) with diverse informed health effects have been indicated to produce EPS, including the well-known Lactobacillus rhamnosus strain GG,[Citation6] which produces two types of molecules: one galactose-rich (the most abundant)[Citation7] and the other glucose-rich.[Citation8] The defined roles of EPS in probiotic actions are numerous,[Citation9] including prebiotic potential, possible involvement in the adhesion to the intestinal epithelium, and modulation of host immunity.[Citation10–Citation12]
EPS-producing LAB are generally regarded as safe due to their long history of safe use in substances for human consumption.[Citation13] In recent years, an enhancing body of evidence has displayed that EPSs produced by different LAB strains have potential biological activities such as antioxidant,[Citation14] immunomodulating,[Citation15] anti-tumor, anti-biofilm,[Citation16] and antibacterial properties.[Citation15] EPSs are also involved in the formation of biofilms,[Citation17,Citation18] which may develop colonization and assist survival.[Citation19,Citation20]
Biofilm is the predominant mode of growth for bacteria in clinical, industrial, and most natural environments.[Citation16,Citation21] Biofilm formation by bacterial pathogens has been indicated to enhance their tolerance to exogenous stress and their ability to escape from the host immune system, causing many serious problems such as food spoilage, biofouling, and ineffective treatment for chronic and repetitive infections.[Citation22] Biofilms are sustainable sources of contaminations that are accountable of food-related illness and substantial economic losses. This lifestyle negotiates protection to bacterial cells and reduces the efficiency of cleaning and disinfection procedures.[Citation23] As a consequence, the need for the progress of new strategies and anti-biofilms agents allowing the suppression and control of biofilm-formation by various pathogens is of major importance in order to diminish the risk of contamination in food sector and human health.
Prebiotics such as fructooligosaccharides (FOS) and inulin, are designated as “a dietary prebiotic is a selectively fermented ingredient that results in specific changes in the composition and/or activity of the gastrointestinal microbiota, thus conferring benefits upon host health” by the International Association of Probiotics and Prebiotics.[Citation24] Prebiotic oligosaccharides and polysaccharides are employed during different stages of gut transit, generally the proximal and distal colon, respectively. This is thought to be due to the fact that long chain carbohydrates are metabolized more slowly than oligosaccharides.[Citation25] As inulin is recently the only commercially present prebiotic polysaccharide, it may be of interest to find new polymeric substances which could be delivered in dissimilar food vectors, such as dairy or bakery products with EPS producing adjuncts, to improve the distal colonic microbiome. It is only in the last few years that LAB EPS have taken attention with regard to prebiotic potential.[Citation26] Due to the lack of wide information on EPSs from the food-associated and human originated LABs to determine this research investigated the use of lyophilized EPS (l-EPS) synthesized by LAB as potential prebiotics. The aim of the present work was determined the EPS production of six lactobacilli strains, monosaccharide composition of the l-EPSs, regulatory effects of the l-EPSs on bifidobacteria development and their anti-biofilm abilities against some pathogens.
Materials and methods
Bacterial strains media and culture conditions
This research comprised Bifidobacterium breve BASO-1 and six strains of lactobacilli, of which these strains were conducted at Gazi University, Biotechnology Laboratory Collection for Type Cultures. The six lactobacilli species utilized in the research were isolated from children’s feces and milk products. The lactobacilli were classified by their morphological and cultural properties, catalase test, and the API 50 CHL kit (BioMerieux, France) analyzed by API LAB plus software version 4.0 databases (data not show). In addition, the strains were approved using 16S rDNA sequence analysis. Uni27F (20 bp) 5′ AGAGTTTGATCCTGGCTCAG 3′ and Uni1492R (19 bp) 5′ GGTTACCTTGTTACGACTT 3′ were used in PCR amplification of 16S rDNA gene regions and sequencing (). The sequences were compared with the sequences deposited in the GenBank database using the BLAST algorithm. Lactobacilli were inoculated in de Man Rogosa Sharpe (MRS) broth (Oxoid, Istanbul, Turkey). All of the lactobacilli strains were stored at –40°C in MRS broth with 10% glycerol, and regenerated twice before the use in the manipulations.
Table 1. Origin and molecular identification results of lactobacilli in this study by sequencing the 16S rRNA gene region of lactobacilli in this study.
To detect the effect of l-EPSs on bifidobacteria, Bifidobacterium breve BASO-1 strain were utilized. The strain were cultured under anaerobic conditions for 24–48 h at 37°C in modify MRS (Merck) broth supplemented with 0.05% w/v L-cysteine-HCl (Merck; MRS-C).[Citation27]
Thirteen pathogen bacteria (Bacillus cereus RSKK 863, Listeria monocytogenes ATCC 7644, Enterococcus fecalis ATCC 25175, Staphylococcus aureus EB-1, S. aureus EB-12, S. aureus ATCC 25923, S. aureus ATCC 2392, S. aureus EB-36, S. aureus EB-18, Escherichia coli 0157:H7, E. coli ATCC 35218, Pseudomonas aeruginosa ATCC 72853, Salmonella typhimurium BAST-01) were used to research biofilm production. The strains were stored at –40°C in Nutrient broth plus 10% (v/v) glycerol. Before experimental use the cultures were propagated twice in nutrient broth at 37°C; the transfer inoculum was 2% (v/v) of a 24 h culture grown in fresh medium.
Isolation and quantification of EPS
EPS was isolated with a method of Frengova et al.[Citation28] Cultures were boiled at 100°C for 15 min. After cooling, they were treated with 17% (v/v) of 85% trichloracetic acid solution and centrifuged at 13,000 rpm for 20 min. Removal of cells and protein was done by centrifugation. The EPS was precipitated with 1 volume of cold absolute ethanol and then centrifuged at 13,000 rpm for 15 min. The pellet containing EPS was dissolved in deionized water. EPS was precipitated with ethanol again, and the EPS that dissolved in deionized water was lyophilized (Christ alpha 2- 4 LD Plus freeze Dryer, UK). The total amount of carbohydrate in the l-EPS was described using the phenol-sulfuric acid method[Citation29] with glucose as standard.
Monomer analysis of l-EPS
The l-EPS was analyzed for carbohydrate content. l-EPS was dissolved with ultra-pure water. Hydrolysis of the EPS was performed with 4 M HCl at 120°C for 15 min. Determination of monosaccharides was made by HPLC (Varian ProStar) with Carbohydrates Ca (300 × 6.5 mm) CP28351 column using water as mobile phase at a flow rate of 0.6 mL/min and the eluted were conformed with a detector (VARIAN 350 RI).
l-EPS fermentation by Bifidobacterium breve BASO-1
For this study, l-EPS belonging GD-11 strain was selected among the six strains according to their capacity of EPS production. Ten milliliters of modified carbohydrate-free basal medium with 1% (wt/vol) l-EPS was inoculated (2% [vol/vol]) with culture of Bifidobacterium breve BASO-1 and incubated for 48 h at 37°C under anaerobic conditions. The number of live (cfu/mL) bacteria was designated by total plate count after 48 h of cultivation.
Effect of l-EPSs on Bifidobacterium breve BASO-1 development
l-EPSs added to the fresh MRS-C broth at final concentrations of 0.5 and 1 mg/mL. A negative control (without l-EPS) and a positive control (0.5 and 1 mg/mL inulin) were also used simultaneously. Bifidobacterium breve BASO-1 strain was inoculated with an initial turbidity at 107–108 CFU/mL. After 48 h incubation at 37°C under anaerobic conditions, the number of viable bacteria was calculated as follows:
where A is the number of colonies, B is dilution factor = 1 /ratio of dilution, C is volume of planted.
Microtiter plate biofilm production assay
The method used was a modified version of that defined by Djordjevic et al.[Citation30] Overnight cultures of pathogen bacteria strains grown at 37°C in nutrient broth were refreshed in nutrient broth. Two hundred microliters of inoculum were transferred in the 96-well polystyrene microtiter plates with an initial turbidity at 600 nm of 0.05 OD. The microtiter plate was then left at 37°C for 24 h in static condition. To analysis the biofilm formation, the remaining medium in the incubated microtiter plate was taken out and the wells were washed five times with sterile distilled water to remove loosely associated bacteria. Plates were air dried for 45 min and each well was stained with 200 μL of 1% crystal violet solution for 45 min. After staining, plates were washed with sterile distilled water five times. The quantitative analysis of biofilm production was made by adding 200 μL of ethanol-acetone solution (4:1) to de-stain the wells. The level (OD) of the crystal violet present in the de-staining solution was measured at OD570 and the cell turbidity was monitored using a microtiter plate reader (Multiscan Spectrum, Thermo Electron Corporation). The rate of biofilm formation was designated by the value of OD. Five replicate wells were performed for each experimental parameter and each data point was averaged from these five. Strains were divided into three; weak biofilm formation, middle biofilm formation and strong biofilm formation. Four strains that were the highest biofilm forming were utilized for test anti-biofilm experiment.
In vitro anti-biofilm effect of l-EPS on pathogen bacteria
The highest biofilm forming pathogen bacteria (L. monocytogenes ATCC-7644, B. cereus RSKK-863, E. fecalis ATCC-25175, and P. aeruginosa ATCC-72853) were grown at 37°C in nutrient broth. The culture, grown overnight, was diluted to 0.1 OD with nutrient broth at 620 nm and then added to a 96-well polystyrene microtiter plate together with different concentrations of l-EPS (0.1 and 1.0 mg/mL). The plate was incubated for 24 h (100 rpm, 37°C) and a biofilm developed by strain in the absence of l-EPS was used as the control. Wells were washed, dried and then, plates were stained for 5 min with 0.2 mL of 2% crystal violet. To correlate biofilm formation with planktonic growth in each well, the planktonic cell fraction was transferred to a new microtiter plate and the OD570 was monitored using a microtiter plate reader (Multiscan Spectrum, Thermo Electron Corporation). The level (OD) of the crystal violet present in the de-staining solution was measured at 570 nm.[Citation31,Citation32] Percent of biofilm inhibition was calculated according to the following formula:
where a is biofilm OD 570 nm, b is planktonic OD 570 nm.
Statistics
All experiments were realized in triplicate, and mean values are presented. The results were stated as means ± standard deviations (SD). Statistical analyses were made using SPSS version 16.0 (SPSS, Chicago, 182 IL, USA). Pearson’s correlation analysis was used to designate the statistical significance of differences between the values.
Results and Discussion
In the present study, six Lactobacillus spp. were isolated from human feces and milk products. The isolates were identified by sequencing of their 16S rRNA gene region which amplified a 1492 bp fragment. They identified as lactobacilli and displayed a similarity of ≥99% to type strains deposited in the GenBank database. As results of sequence analysis; isolates identified as Lactobacillus fermentum, Lactobacillus rhamnosus, Lactobacillus casei, Lactobacillus delbrueckii ssp. bulgaricus, and Lactobacillus plantarum ().
The rheological properties of EPS produced by LAB have determined a major application in the manufacture of fermented dairy products such as yogurt, cheese, or fermented milks, although they also play a role in the elaboration of fermented meats and vegetables.[Citation33–Citation35] The EPSs will remain for longer in the gastrointestinal tract, thus increasing colonization by probiotic bacteria[Citation36] and the prebiotic effect will be in direct or indirect role.
The l-EPSs of Lactobacillus spp. content ranged between 255–320 mg/L (). The l-EPS of L. rhamnosus GD-11 strain produced the highest amount 320 mg/L. A considerable variation can be observed in EPS quantifications. In previous studies, the amount of EPS informed varies from 25 to 132 mg/L for L. lactis ssp. cremoris[Citation37] and L. rhamnosus C83,[Citation38] and from 130–250 mg/L for Lactobacillus casei CG11 and L. delbrueckii ssp. bulgaricus NCFB 2772,[Citation39] respectively. When we compared our results with these findings; Lactobacillus spp. produced comparatively higher quantities of EPS.
Table 2. The amount and carbohydrate composition of l-EPS isolated from lactobacilli and carbohydrate composition of l-EPSs.
The monomer compositions of EPSs that lyophilized and produced by the strains displaying the EPS production were analyzed by high-performance liquid chromatography (HPLC). The l-EPSs have the same monomers in their composition, however, the ratio among the two polymers were different. The l-EPSs defined in samples consist of mannose and glucose monosaccharides. Mannose was the major component of l-EPS from all strains (). The l-EPS produced by these closely related strains also differed in their monosaccharide ratio composition. Other studies declare on the presence of mannose in exocellular polymers in lactobacilli, which was identified as a main monomer in the polysaccharides analyzed in our study.[Citation40–Citation42]
The EPS fermentation capability of the B. breve BASO-1 was evaluated by using the l-EPS of the L. rhamnosus GD-11 strain which is the highest EPS producer. B. breve BASO-1 was able to grow in both MRS-C medium and carbohydrate-free basal medium with 1% (wt/vol) l-EPS (Fig. 1). .B. breve BASO-1 was tested in vitro for its ability to use all the l-EPSs studied. The l-EPSs were added to the medium to a final concentration of 0.5 and 1 µg/mL. Bifidogenic growth stimulator (BGS) activities of l-EPSs were compared with inulin. Inulin enhanced the number of bifidobacteria cell viability, and this stimulation was not higher than BGS activities of the l-EPSs (p < 0.05; ). After 2 days of incubation, all l-EPSs tested, as well as inulin, promoted higher raises in levels of bifidobacteria populations than those occurring in control cultures without carbohydrates addition. Even though an interindividual variability in BGS activities was found, the highest stimulation of bifidogenic growth was promoted by l-EPS from L. fermentum LB-69 (10.70 log cfu/mL at 1 mg/mL; ). Our results exhibited that the l-EPSs produced by these lactobacilli had the ability to modulate the intestinal microbiota, promoting a bifidogenic effect comparable to that of the prebiotic inulin. Also, our results defined that EPS which has different types of monosaccharide composition stimulated the growth of bifidobacteria at different rates.
Table 3. Effect of l-EPS on Bifidobacterium breve BASO-1 development.
Figure 1. EPS fermentation by Bifidobacterium breve BASO-1. (1) The viability in MRS-C medium. (2) The viability in carbohydrate-free basal medium with 1% (wt/vol) l-EPS.
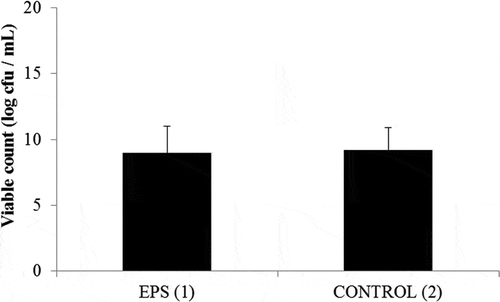
Ruas-Madiedo et al.[Citation43] reported that the susceptibility of the LAB to biological degradation is directly related to the EPSs. The Bifidobacterium strains characterized in their study possess degradative extracellular enzymes belonging to glycoside hydrolase families 2, 13, 36, and 42, such as α-galactosidases, β-galactosidases, and an enzyme active toward glucooligosaccharides. These results exhibited that; EPS synthesized by Lactobacillus spp. could act as fermentable substrates as BGS.[Citation44]
We initially tested the ability of 13 pathogen bacteria to form biofilms in vitro in a classic micro well assay. The highest biofilm forming pathogen bacteria (B. cereus RSKK 863, L. monocytogenes ATCC 7644, E. fecalis ATCC 25175, and P. aeruginosa ATCC 72853) were selected to determine the anti-biofilm effect of l-EPSs (). EPSs, as one of the primary metabolic products of LAB, were strongly associated with functional properties such as antioxidant, antimicrobial and cholesterol-lowering effects due to their unique characteristics and strong biological activities.[Citation45] However, so far few studies have been reported on the characterization and biological activities (e.g., antioxidant, anti-biofilm, and anti-tumor activities) of the l-EPS from lactobacilli.
Table 4. Biofilm formation of some pathogenic bacteria.
A biofilm formation assay with varying concentration of l-EPSs (0.1 and 1 mg/mL) was made in order to study the anti-biofilm potential of the l-EPSs against four pathogen bacteria. As portray in , the inhibitory activities of the l-EPS from three lactobacilli strains on biofilm formation by the four pathogens B. cereus RSKK 863, L. monocytogenes ATCC 7644, E. fecalis ATCC 25175, and P. aeruginosa ATCC 72853 were concentration dependent. The l-EPSs of three lactobacilli indicated potent anti-biofilm activities by inhibiting the four biofilm forming pathogen bacteria such as the abilities of BGS. The inhibition abilities of l-EPSs on biofilms forming pathogen bacteria were in a concentration dependent way. Biofilm formation in B. cereus RSKK 863, L. monocytogenes ATCC 7644, E. fecalis ATCC 25175, and P. aeruginosa ATCC 72853 diminished significantly (p < 0.01) with enhancing concentrations of l-EPSs (). The l-EPSs of L. fermentum LB-69 exhibited significant biofilm inhibition, especially with four pathogen bacteria. At a concentration of 1 mg/mL, L. fermentum LB-69 l-EPS significantly inhibited 90% of biofilm formation by B. cereus RSKK 863 followed by L. monocytogenes ATCC 7644 (88%) and P. aeruginosa ATCC 72853 (88%) among these pathogen bacteria. These results are similar to those reported previously for the lactobacilli in the studies of Kim et al.[Citation31] and Li et al.[Citation16] Kim et al.[Citation31] declared that enterohemorrhagic E. coli biofilms were dramatically diminished by 87% in plastic 96-well microplates that contained 1 mg/mL of released EPS (r-EPS) by the Lactobacillus acidophilus A4 strain. Li et al.[Citation16] indicated that, the EPS from Lactobacillus helveticus MB2-1 had anti-biofilm activity against several biofilm-forming bacteria.
Table 5. Anti-biofilm activity of lyophilized EPSs from the exopolysaccharide (EPS)-produced lactobacilli strains.
In the present study, it was also found that the level of biological activity was depended on the type of EPS (p < 0.01). It was found remarkable that the l-EPSs of L. fermentum LB-69 had high level of mannose (96%). Bifidobacteria are very important microorganisms for taking part in the health of the human gastrointestinal tract. In order to depict these health-beneficial effects, bifidobacteria should raise their population at a definite number. So, the growth stimulator factors of bifidobacteria are very important for stabilizing the microflora in the gut.[Citation26] Prebiotics stimulate the growth of probiotic bacteria in the colon; especially inulin is used for bifidobacteria. This prebiotic carbohydrate is obtained from chicory plants. However, it is very difficult to get this plant. So, BGS molecules should be utilized as a probable prebiotic in food, and microorganisms are easier than plants. Since this activity is not influenced by heat, a wide pH range, and storage conditions, BGS would not lose influence in the gastrointestinal conditions and can be used as a prebiotic alternative to inulin in food products.[Citation44] Microbial EPSs that produced by the probiotic bacteria very important for human health. As well as EPS has immunomodulating, antiulcer, antitumor, cholesterol-lowering effects; prebiotic activity of the EPS is a new found and important feature.[Citation46]
Conclusion
This is the first article giving a detailed anti-biofilm activity and potential prebiotic application of l-EPSs from the lactobacilli. The l-EPS produced by L. fermentum LB-69, which may make it potential in the design of new therapeutic strategies for bacterial biofilm-associated infections and limiting the biofilm formation on medical indwelling devices. The investigation displayed that the l-EPSs produced by lactobacilli had potentials as a multi-functional natural ingredient for therapeutic and health foods. These results may also promote a new recognition about the functions of bacterial EPSs. The prebiotic effect and anti-biofilm activity of the EPSs may contribute to its possible pharmaceutical applications. Also, this is due to their properties, EPSs may be a new potential in the development of functional foods.
References
- FAO-WHO. Report of a Joint FAO/WHO Expert Consultation on Guidelines for the Evaluation of Probiotics in Food; Canada World Health Organization/Food and Agriculture Organization of the United Nations: London, Ontario, Canada, 2002.
- Wyszyńska, A.; Kobierecka, P.; Bardowski, J.; Jagusztyn-Krynicka, E.K. Lactic Acid Bacteria—20 Years Exploring Their Potential as Live Vectors for Mucosal Vaccination. Applied Microbiology and Biotechnology 2015, 99, 2967–2977.
- Li, J.Y.; Jin, M.M.; Meng, J.; Gao, S.M.; Lu, R.R. Exopolysaccharide from Lactobacillus Plantarum LP6: Antioxidation and the Effect on Oxidative Stress. Carbohydrate Polymers 2013, 98, 1147–1152.
- Guo, Y.; Pan, D.; Li, H.; Sun, Y.; Zeng, X.; Yan, B. Antioxidant and Immunomodulatory Activity of Selenium Exopolysaccharide Produced by Lactococcus Lactis Subsp. Lactis. Food Chemistry 2013, 138, 84–89.
- Cerning, J. Production of Exopolysaccharides by Lactic Acid Bacteria and Dairy Propionibacteria. Lait 1995, 75, 463–472.
- Doron, S.; Snydman, D.R.; Gorbach, S.L. Lactobacillus GG: Bacteriology and Clinical Applications. Gastroenterology Clinics of North America 2005, 34, 483–498.
- Landersjo, C.; Yang, Z.N.; Huttunen, E.; Widmalm, G. Structural Studies of the Exopolysaccharide Produced by Lactobacillus Rhamnosus Strain GG (ATCC 53103). Biomacromolecules 2002, 3, 880–884.
- Francius, G.; Lebeer, S.; Alsteens, D.; Wildling, L.; Gruber, H.J.; Hols, P.; De Keersmaecker, S.; Vanderleyden, J.; Dufrêne, Y.F. Detection, Localization, and Conformational Analysis of Single Polysaccharide Molecules on Live Bacteria. ACS Nano 2008, 2, 1921–1929.
- Lebeer, S.; Vanderleyden, J.; De Keersmaecker, S.C.J. Genes and Molecules of Lactobacilli Supporting Probiotic Action. Microbiology and Molecular Biology Reviews 2008, 72, 728–764.
- Lebeer, S.; Claes, I.J.; Verhoeven, T.L.; Vanderleyden, J.; De Keersmaecker, S.C. Exopolysaccharides of Lactobacillus rhamnosus GG Form a Protective Shield Against Innate Immune Factors in the Intestine. Microbiology and Biotechnology 2010, 4, 368–374.
- Ruas-Madiedo, P.; Gueimonde, M.; Margolles, A.; de los Reyes-Gavilán, G.C.; Salminen, S. Exopolysaccharides Produced by Probiotic Strains Modify the Adhesion of Probiotics and Enteropathogens to Human Intestinal Mucus. Journal of Food Protection 2006, 69, 2011–2015.
- Yasuda, E.; Serata, M.; Sako, T. Suppressive Effect on Activation of Macrophages by Lactobacillus Casei Strain Shirota Genes Determining the Synthesis of Cell Wall-Associated Polysaccharides. Applied and Environmental Microbiology 2008, 74, 4746–4755.
- Nikolic, M.; López, P.; Strahinic, I.; Suárez, A.; Kojic, M.; Fernández-García, M.; Topisirovic, L.; Golic, N.; Ruas-Madiedo, P. Characterisation of the Exopolysaccharide (EPS)-Producing Lactobacillus Paraplantarum BGCG11 and Its Non-EPS Producing Derivative Strains as Potential Probiotics. International Journal of Food Microbiology 2012, 158, 155–162.
- Li, S.; Shah, N.P. Antioxidant and Antibacterial Activities of Sulphated Polysaccharides from Pleurotus Eryngii and Streptococcus Thermophilus ASCC 1275. Food Chemistry 2014, 165, 262–270.
- Wu, M.H.; Pan, T.M.; Wu, Y.J.; Chang, S.J.; Chang, M.S.; Hu, C.Y. Exopolysaccharide Activities from Probiotic Bifidobacterium: Immunomodulatory Effects (on J774A.1 Macrophages) and Antimicrobial Properties. International Journal of Food Microbiology 2010, 144, 104–110.
- Li, W.; Ji, J.; Rui, X.; Yu, J.; Tang, W.; Chen, X.; Jiang, M.; Dong, M. Production of Exopolysaccharides by Lactobacillus Helveticus MB2-1 and Its Functional Characteristics in Vitro. LWT–Food Science and Technology 2014, 59, 732–739.
- Vu, B.; Chen, M.; Crawford, R.J.; Ivanova, E.P. Bacterial Extracellular Polysaccharides Involved in Biofilm Formation. Molecules 2009, 14, 2535–2554.
- Dertli, E.; Mayer, M.J.; Narbad, A. Impact of the Exopolysaccharide Layer on Biofilms, Adhesion and Resistance to Stress in Lactobacillus Johnsonii FI9785. BMC Microbiology 2015, 15, 1–9.
- Jones, S.E.; Versalovic, J. Probiotic Lactobacillus Reuteri Biofilms Produce Antimicrobial and Anti-Inflammatory Factors. BMC Microbiology 2009, 9, 35.
- Walter, J.; Schwab, C.; Loach, D.M.; Ganzle, M.G.; Tannock, G.W. Glucosyltransferase A (GtfA) and Inulosucrase (Inu) of Lactobacillus Reuteri TMW1.106 Contribute to Cell Aggregation, in Vitro Biofilm Formation, and Colonization of the Mouse Gastrointestinal Tract. Microbiology 2008, 154, 72–80.
- Stoodley, P.; Sauer, K.; Davies, D.G.; Costerton, J.W. Biofilms as Complex Differentiated Communities. Annual Review of Microbiology 2002, 56, 187–209.
- Wang, J.; Zhao, X.; Yang, Y.; Zhao, A.; Yang, Z. Characterization and Bioactivities of an Exopolysaccharide Produced by Lactobacillus Plantarum YW32. International Journal of Biological Macromolecules 2015, 74, 119–126.
- Ouali, F.A.; Kassaa, I.A.; Cudennec, B.; Abdallah, M.; Bendali, F.; Sadoun, D.; Chihib, N.E.; Drider, D. Identification of Lactobacilli with Inhibitory Effect on Biofilm Formation by Pathogenic Bacteria on Stainless Steel Surfaces. International Journal of Food Microbiology 2014, 191, 116–124.
- ISAPP. 6th Meeting of the International Scientific Association of Probiotics and Prebiotics. London, Ontario, Canada, 2008.
- Salazara, N.; Gueimondea, M.; De Los Reyes-Gavilána, C.G.; Ruas-Madiedo, P. Exopolysaccharides Produced by Lactic Acid Bacteria and Bifidobacteria as Fermentable Substrates by the Intestinal Microbiota. Critical Reviews in Food Science Nutrition 2016, 56, 1440–1453. DOI:10.1080/10408398.2013.770728
- Ryan, P.M.; Ross, R.P.; Fitzgerald, G.F.; Caplicee N.M.; Stanton, C. Sugar-Coated: Exopolysaccharide Producing Lactic Acid Bacteria for Food and Human Health Applications. Food and Function 2015, 6, 679–693.
- Beerens H. Year Elective and Selective Insulation Medium for Bifidobacterium sp. Letters in Applied Microbiology 1990, 11, 155–157.
- Frengova, G.I.; Simova, E.D.; Beshkova, D.M.; Simov, Z.I. Production and Monomer Composition of Exopolysaccharides by Yogurt Starter Cultures. Canadian Journal of Microbiology 2000, 46, 1123–1127.
- Dubois, M.; Gilles, K.A.; Hamilton, J.K.; Roberts, P.A.; Smith, F. Colorimetric Determination of Sugars and Related Substances. Analytical Chemistry 1956, 28, 350–356.
- Djordjevic, D.; Wiedmann, M.; McLandsborough, L.A. Microtiter Plate Assay for Assessment of Listeria Monocytogenes Biofilm Formation. Applied and Environmental Microbiology 2002, 68, 2950–2958.
- Kim, Y.; Oh, S.; Kim, S.H. Released Exopolysaccharide (r-EPS) Produced from Probiotic Bacteria Reduce Biofilm Formation of Enterohemorrhagic Escherichia Coli O157:H7.Biochemical and Biophysical Research Communication 2009, 379, 324–329.
- Sayem, S.M.A.; Manzo, E.; Ciavatta, L.; Tramice, A.; Cordone, A.; Zanfardino, A.; De Felice, M.; Varcamonti, M. Anti-Biofilm Activity of an Exopolysaccharide from a Sponge-Associated Strain of Bacillus Licheniformis. Microbial Cell Factories 2011, 10, 74.
- Van den Berg, D.J.C.; Smits, A.; Pot, B.; Ledeboer, A.M.; Kersters, K.; Verbakel, J.M.A.; Verrips, C.T. Isolation, Screening, and Identification of Lactic Acid Bacteria from Traditional Food Fermentation Processes and Culture Collections. Food Biotechnology 1993, 7, 189–205.
- Tieking, M.; Korakli, M.; Ehrmann, M.A.; Ganzle, M.G.; Vogel. R.F. In Situ Production of Exopolysaccharides During Sourdough Fermentation by Cereal and Intestinal Isolates of Lactic Acid Bacteria. Applied and Environmental Microbiology 2003, 69, 945–952.
- Sánchez, J.I.; Martìnez, B.; Guillén, R.; Jiménez-Díaz, R.; Rodríguez, A. Culture Conditions Determine the Balance Between Two Different Exopolysaccharides Produced by Lactobacillus Pentosus LPS26. Applied and Environmental Microbiology 2006, 72, 7495–7502.
- Welman, A.D.; Maddox, I.S. Exopolysaccharides from Lactic Acid Bacteria: Perspectives and Challenges. Trends Biotechnology 2003, 21, 269–274.
- Marshall, V.M.; Courie, E.N.; Moreton, R.S. Analysis and Production of Two Exopolysaccharides from Lactobacillus Lactis Subsp. Cremoris LC330. Journal of Dairy Research 1995, 62, 621–628.
- Gamar, L.; Blondeau, K.; Simonet, J.M. Physiological Approach to Extracellular Polysaccharide Production by Lactobacillus Rhamnosus Strain C83. Journal of Applied Microbiology 1997, 83, 281–287.
- Grobben, G.J.; Sikkema, J.; Smith, M.R.; de Bont, J.A.M. Production of Extracellular Polysaccharides by Lactobacillus Delbrueckii Spp. Bulgaricus NCFB 2772 Grown in a Chemically Defined Medium. Journal of Applied Bacteriology 1995, 79, 103–107.
- Cerning, J.; Bouillanne, C.; Desmazeaud, M.J.; Landon, M. Isolation and Characterization of Exocellular Polysaccharide Produced by Lactobacillus Bulgaricus. Biotechnology Letters 1986, 8, 625–628.
- Bouzar, F.; Cerning, J.; Desmazeaud, M. Expoloysaccharide Production in Milk by Lactobacillus Delbrueckii Ssp. Bulgaricus CNRZ 1187 and by Two Colonial Variants. Journal of Dairy Science 1996, 79, 205–211.
- Aslim, B.; Beyatli, Y.; Yuksekdag, Z.N. Productions and Monomer Compositions of Exopolysaccharides by Lactobacillus Delbrueckii Subsp Bulgaricus and Streptococcus Thermophilus Strains Isolated from Traditional Home-Made Yoghurts and Raw Milk. International Journal of Food Science Technology 2006, 41, 973–979.
- Ruas-Madiedo, P.; Hugenholtz, J.; Zoon, P. An Overview of the Functionality of Exopolysaccharides Produced by Lactic Acid Bacteria. International Dairy Journal 2002, 12, 163–171.
- Pokusaeva, K.; Fitzgerald, G.F.; van Sinderen, D. Carbohydrate Metabolism in Bifidobacteria. Genes and Nutritions 2011, 6, 285–306.
- Kim, Y.; Oh, S.; Yun, H.S.; Oh, S.; Kim, S.H. Cell-Bound Exopolysaccharide from Probiotic Bacteria Induces Autophagic Cell Death of Tumor Cells. Letters in Applied Microbiology 2010, 51, 123–130.
- Rios-Covian, D.; Arboleya, S.; Hernandez-Barranco, A.M.; Alvarez-Buylla, J.R.; Ruas-Madiedo, P.; Gueimonde, M.; de los Reyes-Gavilan, C.G. Interactions Between Bifidobacterium and Bacteroides Species in Co-Fermentations are Affected by Carbon Sources, Including Exopolysaccharides Produced by Bifidobacteria. Applied and Environmental Microbiology 2013, 79, 7518–7524.