ABSTRACT
Protein extracted from Douchi was hydrolyzed by alcalase based on a single-factor experiment and response surface methodology was employed to optimize hydrolysis conditions. The optimum conditions were determined to be: 63℃ extraction temperature, 1.4% quantities of enzyme and substrate, and 1.7 h extraction time. The optimal protein hydrolysate was subsequently subjected to ultrafiltration, and the fraction of molecular weight 10–50 kDa was identified as the most potent contributor to antioxidant activity. Amino acid analysis indicated that acidic amino acids were the most abundant in all fractions of the protein hydrolysate. Infrared spectroscopy showed there were no significant differences in secondary structure between fractions.
Introduction
Oxidative damage is considered to play a causative role in several pathological processes, including cancer, cirrhosis, and emphysema.[Citation1,Citation2] Synthetic antioxidants, such as butylated hydroxyanisole (BHA) and butylated hydroxytoluene (BHT), have limited use in foods because they are suspected to be carcinogenic.[Citation3] As a result, there is increasing interest in identifying antioxidants from natural sources. In recent years, protein hydrolysates from many plants, such as wheat, soybean, and corn gluten meal, have been found to possess antioxidant activity.[Citation4–Citation6] Plant proteins are of particular interest as raw materials for the production of antioxidant peptides due to their low cost and widespread availability.
Membrane ultrafiltration technology represents an attractive means to fractionate bioactive protein hydrolysate. In recent years, this technology has been applied to the fractionation of many bioactive protein hydrolysates, such as rapeseed, yeast, and tuna dark muscle by-product.[Citation7–Citation9] Compared to conventional separation methods, such as gel chromatography, membrane ultrafiltration technology offers several advantages because it is low cost, energy saving, highly efficient, and environmentally friendly. Additionally, it is able to continuously operate at room temperature and avoid formation of unwanted precipitates during the separation.[Citation10]
Soybean is a well-known source of protein with a high nutritional value and many functional properties. Douchi, a seasoning food produced in China, is rich in nutrition and is obtained by fermenting steamed soybeans with microorganisms.[Citation11] It is similar to natto in Japan, tempeh in Indonesia and chungkookjang in Korea, which are all prepared by fermenting steamed soybeans.[Citation12–Citation14] Some researchers have evaluated the bioactive peptides of natto and tempeh after protease treatment.[Citation15] Bioactive peptides can be released from proteins by enzymatic proteolysis; protein hydrolysates exhibit strong antioxidant activity when treated with various proteases.[Citation16,Citation17] Douchi is especially promising because of its high protein content, which could enhance the yield of enzymatic protein hydrolysates. The bioactivity of protein hydrolysates could be affected by different parameters, such as the hydrolysis condition, molecular size, and amino acid composition. Box-Behnken design (BBD) of response surface methodology (RSM) has been successfully applied to optimize conditions in food research. This methodology is more efficient and easier to arrange and interpret experimental models compared to other methodologies.[Citation18,Citation19]
Although a few studies have performed comparisons between the molecular weight of plant hydrolysate and its antioxidant activity, the relationship between Douchi protein hydrolysate and antioxidant activity remains unclear. The optimization of antioxidant activity by enzymatic Douchi protein hydrolysate has not previously been reported nor have the ultrafiltration and secondary structures of Douchi protein hydrolysate been investigated. The objective of the present work was to optimize hydrolysis parameters to produce the highest antioxidant activity of Douchi protein hydrolysate using RSM. Then, the hydrolysate was separated by ultrafiltration membranes, and the antioxidant activity of each fraction was determined. Finally, the amino acid composition was determined by an automatic amino acid analyzer, and secondary structures of the collected hydrolysate fractions were investigated by infrared spectroscopy.
Materials and Methods
Materials and Reagents
Douchi was kindly provided by Yangjiang Yangfan Douchi Co., Ltd (Yangjiang, China) and identified as Aspergillus type. Alcalase (endoproteinase from Bacillus licheniformis) was purchased from Novozymes China Inc (Tianjin, China). 2,2-diphenyl-1-picrylhydrazyl (DPPH) was purchased from Sigma Chemical Co. (St. Louis, MO, USA). L-Ascorbic acid was purchased from Sinopharm Chemical Reagent Co., Ltd. (Shanghai, China). All other chemicals used in the experiments described below were of analytical grade and were purchased from Guangzhou Chemical Reagent factory (Guangzhou, China).
Extraction of Protein Hydrolysate
Douchi was dried in an oven at 40°C, then was ground in a high speed disintegrator. The powder was diluted with deionized water using a range of ratios of water to raw material from 6 to 10 (v/w), protein samples were extracted in a thermostat-controlled water bath ranging from 40 to 80℃, and the pH value was adjusted to a value ranging from 8 to 10 with enzyme and substrate (E/S) ranging from 1.1 to 1.9% for 1 to 5 h. The mixtures were then heated in a boiling water bath at 100°C for 10 min to inactive enzyme activity. After centrifuging at 4°C, 2057 × g for 5 min, the mixtures were vacuum filtrated until they had no residue. Then, the pH values of the mixtures were adjusted by adding 0.25 M NaOH. The precipitate was obtained by centrifugation at 4°C, 9800 × g for 10 min after pH adjustment to 4.35. The obtained precipitate was then lyophilized for further use.[Citation20]
Experimental Design and Statistical Analysis
On the basis of a single-factor experiment for the protein hydrolysate, proper ranges of extraction temperature, pH, ratio of water to raw material, extraction time, and E/S were preliminarily determined. To optimize the DPPH scavenging activity of the Douchi protein hydrolysate, three independent variables at three levels were employed in a 17-run BBD, coded 1, 0, and -1 for high, intermediate and low values, respectively. The variables were E/S (X1: –1–1.3%, 0–1.5%, 1–1.7%), extraction time (X2: –1–1 h, 0–2 h, 1–3 h), and extraction temperature (X3: –1–50°C, 0–60°C, 1–70°C). The DPPH scavenging activity value was selected as the experimental response Y. This experimental design was also used to estimate the interactions between response function and factors. The experimental design was analyzed and the predicted data were calculated using the Design-Expert software (v.8.0.6, Stat-Ease, Inc, Minneapolis, USA) to estimate the responses of independent variables.
Determination of the degree of hydrolysis
The DH value is equal to the number of hydrolyzed peptide bonds divided by the number of peptide bonds in the substrate (htot). The DH values were calculated using the following formula:
where B is the amount of alkali consumed to keep the pH constant during the reaction, Nb is the normality of the alkali, Mp is the mass of the protein in the reaction, andαis the average degree of dissociation ofα-NH2 groups released during hydrolysis. The value of htot is the total amount of peptide bonds in the protein substrate determined from the amino acid composition.[Citation21]
DPPH Radical Scavenging Activity
The DPPH radical scavenging activity was assayed according to the methods described by Blois,[Citation22,Citation23] with a slight modification. Three milliliters of samples of varying concentrations was mixed with 3 mL of DPPH in ethanol solution (0.16 mmol/L) and incubated at 25°C for 10 min; upon reaction completion, the absorbance of each sample was read at 517 nm. A blank was prepared from the reaction mixture without DPPH solution. For an absorbance negative control, deionized water replaced the sample in the reaction mixture. Ascorbic acid was used as a positive control. All measurements were performed in triplicate. The IC50 value was defined as the concentration at which 50% of DPPH radicals were scavenged, representing a parameter widely used to measure the antioxidant activity, and was calculated from a calibration curve by linear regression. The scavenging activity was expressed as a percentage using the following formula:
Ultrafiltration
Douchi protein hydrolysate that was obtained under optimized conditions and demonstrated the highest activity was selected for further analysis. The lyophilized protein hydrolysate was dissolved in deionized water and fractioned according to molecular weight (Mw) using three different cut-off membranes (50, 10, and 5 kDa; Millipore, USA). Four fractions were collected: Mw: >50 kDa, Mw: 10–50 kDa, Mw: 5–10 kDa, Mw: <5 kDa. The fractions were freeze-dried to measure the antioxidant activity.[Citation24]
Analysis of Amino Acid Composition
The amino acid composition of each sample was analyzed according to published methods.[Citation25] The protein hydrolysate and ultrafiltration fractions were hydrolyzed with 6 M HCl at 110°C for 14 h. The acidolysis solution was diluted to 4 mL with 0.02 mol/L HCl, and 20 μL of the sample was injected into the automatic amino acid analyzer (L-8800, HITACHI, Japan) for the detection of amino acids. The content of the individual amino acids were expressed as:
Infrared Spectroscopy
The samples were fully dried in a dryer containing P2O5, mixed with potassium bromide powder and pressed into tablets. They were then analyzed by infrared spectroscopy using a Nicolet Nexus 470 FT-IR spectrometer (Thermo, USA) at a resolution of 2 cm−Citation1, over a range of 4000 to 400 cm−Citation1.[Citation26]
Results and Discussion
The effects of the extraction temperature, pH, ratio of water to raw material, extraction time, and E/S on DPPH scavenging activity were systematically investigated. As shown in , to investigate the effect of the extraction temperature on the DPPH scavenging activity, the extraction was carried out at temperatures of 40, 50, 60, 70, and 80°C, while the other extraction parameters were fixed as follows: pH 9, extraction time 3 h, extraction ratio of water to raw material 8 and E/S 1.5%. The optimum temperature for hydrolysis was found to be 60°C. The hydrolysate showed maximum DPPH scavenging activity at 60°C. The DH increased at first and then decreased. These results could be due to the high temperature reducing enzyme activity. Therefore, 60°C was selected as center-point with 10°C as a step change.
Figure 1. Effect of A: extraction temperature, B: pH, C: ratio of water to raw material, D: extraction time, and E: E/S on the DPPH scavenging activity.
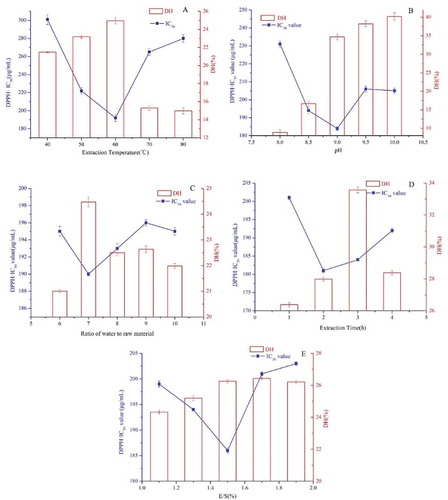
The pH was another factor that could influence the extraction efficiency. The effect of pH on the DPPH scavenging activity was investigated by varying the pH (8, 8.5, 9, 9.5, and 10) while other extraction variables were set as follows: extraction temperature 60°C, extraction ratio of water to raw material 8 and E/S 1.5%. The results are shown in ; the DH increased when the pH increased, and the optimum pH for the highest DPPH scavenging activity was at 9. Thus, pH 9 was used in subsequent experiments.
The ratio of water to raw material was set to 6, 7, 8, 9, and 10 to investigate the effect on DPPH scavenging activity, as shown in . For ratios of water to raw material from 6 to 10, the DH increased at first and reached the highest value at a ratio of 7, and then the DH decreased significantly when the ratio of water to raw material increased thereafter. The DPPH scavenging activity changed steadily. For the purpose of saving energy and lowering cost, the ratio of water to raw material was set to 7 in all subsequent experiments.
The effect of extraction time on DPPH scavenging activity was investigated using different extraction times (1, 2, 3, 4, and 5 h) while other extraction variables were set as follows: extraction temperature 60℃, ratio of water to raw material 7, E/S 1.5% and pH 9. The results showed () the best DH and the highest DPPH scavenging activity at 2 h. Hence, 2 h was chosen as the center-point with 1 h as a step change.
We next examined the effect of hydrolysis E/S from 1.1% to 1.9% () while the other extraction variables were set as follows: 60℃ extraction temperature, a ratio of water to raw material of 7, 2 h extraction time and pH 9. The results showed the DH changed steadily, and the IC50 value of the DPPH scavenging activity was lowest when the E/S was at 1.5%. The center point for E/S was 1.5% with a step change of 0.2%. showed that hydrolysis extraction temperature, pH, extraction time, ratio of water to raw material and E/S had a great effect on the DH and the DPPH scavenging activity. However, the proper hydrolysis conditions to produce the highest antioxidant activity were different from the proper hydrolysis conditions to reach the highest DH. It can also be seen that low DH gave Douchi protein hydrolysate significant inhibition of DPPH scavenging activity. These results indicated that the final DH did not determine the antioxidant activity protein hydrolysate. A similar result showing that higher DH does not guarantee a higher antioxidant activity has been previously reported.[Citation27]
Predicted Model and Statistical Analysis
The extraction conditions of protein hydrolysate from Douchi were optimized by RSM. The effects of E/S (X1), extraction time (X2), and extraction temperature (X3) on DPPH scavenging activity of enzymatic hydrolysis were studied. The data from 17 experimental runs using BBD are presented. The results showed obvious changes in DPPH scavenging activity with changes in these experimental parameters. The minimum DPPH scavenging activity (190 μg/mL) was found with the combination of the E/S 1.5%, 2 h extraction time, and 60℃ extraction temperature.
By employing multiple regression analysis on the experimental data, the predicted response Y for the DPPH scavenging activity can be obtained by the following second-order polynomial equation:
where X1, X2, and X3 were the coded values of the test variables, E/S(%), extraction time (h), extraction temperature (°C), respectively. An analysis of variance (ANOVA) was performed to determine the stability of the model. The significance of each coefficient was analyzed by F-test and the p-value; the quadratic regression model demonstrated that the model was highly significant, as was evident from the F-test, with a very low probability value (p = 0.0001). The lack of fit measures the failure of the model to represent the data in the experimental domain at points that are not included in the regression. A model is considered adequate (significant) at the 95% confidence level, meaning the p-value given by the lack of fit test is higher than 0.05. The F-value (3.17) and p-value (0.14) attained by the lack of fit analysis implied that the lack of fit was not significant relative to pure error, thus indicating that the model was adequate for predicting the DPPH scavenging activity under any combination of values for the three variables. For the p-value of the three independent variables, X3 (0.0003) was lower than X1 and X2, indicating the extraction temperature had the most significant effect on the antioxidative activity within a 99% confidence interval. The quadratic terms, X12 (p =0.0002), X22 (p =0.0065), and X32 (p < 0.0001), as well as the interaction term X1 × X2 (0.01 < p < 0.05) were all significant within a 99% confidence interval. Therefore, the E/S (X1), the extraction time (X2), and the extraction temperature (X3) were important factors in the DPPH scavenging activity.
The coefficient of determination (RCitation2 = 0.97), the adjusted coefficient of determination (RCitation2Adj = 0.94) and the coefficient of variation (C.V. = 5.26%) are shown in . These values indicated that the accuracy and the general availability of the polynomial model were adequate. The very low value of 5.26% for the C.V. clearly indicates a very high degree of precision and a good deal of reliability in the experimental values. The adeq. precision measured the signal to noise ratio, and a ratio greater than 4 is normally desirable. The adeq. precision of 15.35 for this model indicated that it could be used to navigate the design space.
Table 1. Analysis of variance for the fitted quadratic polynomial model of the DPPH.IC50 value.
Optimization of the Procedure
The optimal values of the selected variables were obtained by solving the regression equation using the Design-Expert software. The 3D response surface and the 2D contour plots are provided as graphical representations of the regression equation (). They provided a method to visualize the relationship between responses and experimental levels of each variable and the type of interactions between two test variables. The shapes of the contour plots, circular or elliptical, indicated whether the mutual interactions between variables are significant or not. Each contour curve represents an infinite number of combinations of two test variables, while the other factors were fixed at zero. (A,a) shows the effect of the reciprocal interactions of E/S and extraction time on DPPH scavenging activity. The IC50 of the DPPH scavenging activity decreased at first and then increased, when the E/S reached a designed range from 1.3 to 1.7%, and it increased linearly with increase in extraction time. The contours were slightly inclined to the horizontal, showing that there was a significant interaction between the two parameters. The IC50 of the DPPH scavenging activity decreased at first and then increased with increasing E/S and extraction temperature (shown in [B,b]). From (C,c), the changes in IC50 values were negligible with an increase in the extraction time, and the IC50 values increased with an increase in the extraction temperature at first and then decreased with the increase of time.
Validation of the Model
To validate the adequacy of the model equations, a verification experiment was carried out under the optimal conditions with 1.4% E/S, 1.7 h extraction time, and 63°C extraction temperature. Under these optimal conditions, the mean value of the DPPH scavenging activity was 188.12 ± 0.19 μg/mL (n = 3), corresponding well to the value predicted from the model equation, which confirmed that the model was adequate for the optimization. The IC50 of the Douchi protein hydrolysate was lower than those of ALPs (0.75 mg/mL),[Citation28] neutrase hydrolysates (2.3 mg/mL),[Citation29] DH-5 (0.56 mg/mL),[Citation30] and E(a) – 5 (0.22 mg/mL)[Citation31] from the protein hydrolysates of alfalfa leaf, Cannabis sativa L., buckwheat and Chinese cherry seeds, respectively, but higher than the control Trolox (0.045 mg/mL)[Citation30] and Vc (0.105 mg/mL).[Citation26] These results illustrated that Douchi protein hydrolysate showed a stronger DPPH scavenging activity than other plant resources.
Antioxidant Activity of the Fractions
Ultrafiltration technology has been successfully utilized to obtain powerful bioactive protein hydrolysates, which could avoid the loss of bioactive substances during treatment. From above, the protein hydrolysate showed higher antioxidant activity in the DPPH scavenging activity; therefore, it was chosen as sample for analyzing antioxidant activity in relation to molecular weight. The ultrafiltration membranes of different molecular weight cut-offs (50 kDa, 10 kDa and 5 kDa) were used successively to identify the main fractions of the Douchi protein hydrolysate. The IC50 of the DPPH scavenging activity of each fraction is shown in . It was found that the DPPH scavenging activity of protein hydrolysate was related to the molecular weight, and the 10–50 kDa fraction exhibited the highest scavenging activity. Similar to our findings, rice protein hydrolysates separated by sequential ultrafiltration showed that the high-MW hydrolysate fraction F1 (>10 kDa) had higher DPPH scavenging activities than low-MW fractions F2-F4 (3–10, 1–3, and <1 kDa).[Citation32] A similar study also showed that a protein from Zanthoxylum piperitum with a MW of 24 kDa possessed stronger DPPH radical scavenging activity.[Citation33] It has been suggested that the 10–50 kDa Douchi protein hydrolysate fraction may consist of potent bioactive peptides sequences, even though the fraction contains peptides relatively large in size.
Table 2. Antioxidant activities of protein hydrolysate fractions separated by ultrafiltration.
The amino acid compositions of the protein hydrolysate and ultrafiltration fractions are presented in . Asp and Glu were the most abundant amino acids in the fractions; these two amino acids have two carboxyl groups and one basic amino group and belong to the acidic amino acids. A previous report has indicated that acidic amino acids, such as Glu and Asp, have strong antioxidant effects due to the presence of excess electrons that can be donated during interactions with free radicals.[Citation34] A similar study has reported that the acidic fractions from protein hydrolysates showed stronger DPPH radical scavenging activity than basic fractions.[Citation35] Therefore, acidic amino acids might control the antioxidant potential of proteins and peptides.
Table 3. Amino acid of protein hydrolysate fractions separated by ultrafiltration.
The FT-IR spectra of the protein hydrolysate fractions are illustrated in . A hydroxyl stretch vibration was observed at 3400~3200 cm−Citation1, and a C-H stretch was observed at 3000~2800 cm−Citation1. Strong peaks in absorption from 1630 cm−Citation1~1539 cm−Citation1 were characteristic of amide I and amide II, which are typical of protein absorption. The absorption at 1398 cm−Citation1 should be assigned to the stretching vibration of C-N in the amide. The peaks at 1200~1000 cm−Citation1 suggested the presence of ring vibrations overlapped with stretching vibrations of C-OH and C-O-C.[Citation36] Based on this analysis of the FTIR spectra, there were no significant differences between any of the fractions in terms of secondary structure.
Conclusions
In this work, Douchi protein hydrolysate extraction and ultrafiltration were carried out. The hydrolysis of Douchi protein hydrolysate was investigated using signal factor and RSM. The optimal conditions that were determined are as follows: 1.4% E/S, 1.7 h extraction time, 63°C extraction temperature, pH 9, and a ratio of water to material of 7. The fraction of protein hydrolysate within the molecular weight range of 10–50 kDa displayed the highest antioxidant activity of any fraction. Acidic amino acids were the most abundant amino acids in all protein hydrolysate ultrafiltration fractions, and there were no significant differences between the fractions in terms of secondary structure.
References
- Halliwell, B.; Gutteridge, J.M. Oxygen Toxicity, Oxygen Radicals, Transition Metals and Disease. Journal Biochemistry 1984, 219, 1–14.
- Ashok, B.T.; Ali, R. The Aging Paradox: Free Radical Theory of Aging. Experimental Gerontology 1999, 34, 293–303.
- Namiki, M. Antioxidants/Antimutagens in Foods. Reviews Food Science and Nutrition 1990, 29, 273–300.
- Georgettia, S.R.; Casagrande, R.; Souza, C.R.F.; Oliveira, W.P.; Fonseca, M.J.V. Spray Drying of the Soybean Extract: Effects on Chemical Properties and Antioxidant Activity. LWT–Food Science and Technology 2008, 41, 1521–1527.
- Zhu, K.X.; Zhou, H.M.; Qian, H.F. Antioxidant and Free Radical-Scavenging Activities of Wheat Germ Protein Hydrolysates (WGPH) Prepared with Alcalase. Process Biochemistry 2006, 6, 1296–1302.
- Li, X.X.; Han, L.J.; Chen, L.J. In Vitro Antioxidant Activity of Protein Hydrolysates Prepared from Corn Gluten Meal. Journal of the Science of Food and Agriculture 2008, 88, 1660–1666.
- He, R.; Girgih, A.T.; Rozoy, E.; Bazinet, L.; Ju, X.R.; Aluko, R.E. Selective Separation and Concentration of Antihypertensive Peptides from Rapeseed Protein Hydrolysate by Electro Dialysis with Ultrafiltration Membranes. Food Chemistry 2016, 197, 1008–1014.
- Mirzaei, M.; Mirdamadi, S.; Ehsani, M.R.; Aminlari, M.; Hosseini, E. Purification and Identification of Antioxidant and ACE-Inhibitory Peptide from Saccharomyces Cerevisiae Protein Hydrolysate. Journal of Functional Foods 2015, 19, 259–268.
- Saidi, S.; Deratani, A.; Belleville, M.P.; Amar, R.B. Antioxidant Properties of Peptide Fractions from Tuna Dark Muscle Protein by-Product Hydrolysate Produced by Membrane Fractionation Process. Food Research International 2014, 65, 329–336.
- Mohammad, A.W.; Ng, C.Y.; Ying, P.L.; Ng, G.H. Ultrafiltration in Food Processing Industry: Review on Application, Membrane Fouling, and Fouling Control. Food and Bioprocess Technology 2012, 5, 1143–1156.
- Zhang, J.H; Tatsumi, E.; Ding, C.H.; Li, L.T. Angiotensin I-Converting Enzyme Inhibitory Peptides in Douchi: A Chinese Traditional Fermented Soybean Product. Food Chemistry 2006, 98, 551–557.
- Sun, P.; Wang, J.Q.; Zhang, H.T. Effects of Bacillus Subtilis Natto on Performance and Immune Function of Preweaning Calves. Journal Dairy Science 2010, 93, 5851–5855.
- Nout, M.J.R.; Beernink, G.; Bonants-Lan Laarhoven, T.M.G. Growth of Bacillus Cereus in Soyabean Tempeh. International Journal of Microbiology 1987, 4, 293–301.
- Kim, N.Y.; Song, E.J.; Kwon, D.Y.; Kim, H.P.; Heo, M.Y. Antioxidant and Antigenotoxic Activities of Korean Fermented Soybean. Food and Chemical Toxicology 2008, 46, 1184–1189.
- Gibbs, B.F.; Zougman, A.; Masse, R.; Mulligan, C. Production and Characterization of Bioactive Peptides from Soy Hydrolysate and Soy-Fermented Food. Food Research International 2004, 37, 123–131.
- Zhang, L.; Liu, Y.Z.; Lu, D.; Han, J.; Lu, X.M.; Tian, Z.H.; Wang, Z. Angiotensin Converting Enzyme Inhibitory, Antioxidant Activities, and Antihyperlipidaemic Activities of Protein Hydrolysates from Scallop Mantle (Chlamys Farreri). International Journal of Food Properties 2015, 18, 33–42.
- Alashi, A.M.; Blanchard, C.L.; Mailer, R.J. Antioxidant Properties of Australian Canola Meal Protein Hydrolysates. Food Chemistry 2014, 146, 500–506.
- Box, G.E.; Wilson, K.B. On the Experimental Attainment of Optimum Conditions. Journal of the Royal Statistical Society, Series B (Methodological) 1951, 13, 41–45.
- Nayik, G.A.; Dar, B.N.; Nanda, V. Optimization of the Process Parameters to Establish the Quality Attributes of DPPH Radical Scavenging Activity, Total Phenolic Content, and Total Flavonoid Content of Apple (Malus Domestica) Honey Using Response Surface Methodology. International Journal of Food Properties 2016, 19, 1738–1748.
- Kou, X.H.; Gao, J.; Xue, Z.H.; Zhang, Z.J.; Wang, H.; Wang, X. Purification and Identification of Antioxidant Peptides from Chickpea (Cicer Arietinum L.) Albumin Hydrolysates. LWT Food Science and Technology 2013, 50, 591–598.
- Guerard, F.; Guimas, L.; Binet, A. Production of Tuna Waste Hydrolysates by a Commercial Neutral Protease Preparation. Journal of Molecular Catalysis B: Enzymatic 2002, 19–20, 489–498.
- Blois, M.S. Antioxidant Determinations by the Use of a Stable Free Radical. Nature 1958, 26, 1199–1200.
- Dasa, A.J.; Dasa, G.; Miyaji, T.; et al. In vitro antioxidant activities of polyphenols purified from four plant species used in rice beer preparation in Assam India. International Journal of Food Properties. 2016, 19, 636–651.
- Chen, N.; Yang, H.M.; Sun, Y.; Niu, J.; Liu, S.Y. Purification and Identification of Antioxidant Peptides from Walnut (Juglans Regia L.) Protein Hydrolysates. Peptides 2012, 38, 344–349.
- Ye, M.; Ren, L.; Wu, Y.N. Quality Characteristics and Antioxidant Activity of Hickory-Black Soybean Yogurt. LWT–Food Science and Technology 2013, 51, 314–318.
- Jing, Y.S.; Cui, X.L.; Chen, Z.Y.; Huang, L.J.; Song, L.Y.; Liu, T.; Lv, W.J.; Yu, R.M. Elucidation and Biological Activities of a New Polysaccharide from Cultured Cordyceps Militaris. Carbohydrate Polymers 2014, 102, 288–296.
- Guo, Y.X.; Pan, D.D.; Tanokura, M. Optimisation of Hydrolysis Conditions for the Production of the Angiotensin-I Converting Enzyme (ACE) Inhibitory Peptides from Whey Protein Using Response Surface Methodology. Food Chemistry 2009, 114, 328–333.
- Xie, Z.J.; Huang, J.R.; Xu, X.M.; Jin, Z.Y. Antioxidant Activity of Peptides Isolated from Alfalfa Leaf Protein Hydrolysate. Food Chemistry 2008, 111, 370–376.
- Tang, C.H.; Wang, X.S.; Yang, X.Q. Enzymatic Hydrolysis of Hemp (Cannabis Sativa L.) Protein Isolate by Various Proteases and Antioxidant Properties of the Resulting Hydrolysates. Food Chemistry 2009, 114, 1484–1490.
- Tang, C.H.; Peng, J.; Zhen, D.W.; Chen, Z. Physicochemical and Antioxidant Properties of Buckwheat (Fagopyrum Esculentum Moench) Protein Hydrolysates. Food Chemistry 2009, 115, 672–678.
- Guo, P.; Qi, Y.J.; Zhu, C.H.; Wang, Q. Purification and Identification of Antioxidant Peptides from Chinese Cherry (Prunus Pseudocerasus Lindl.) Seeds. Journal of Functional Foods 2015, 19, 394–403.
- Zhou, K.Q.; Canning, C.; Sun, S. Effects of Rice Protein Hydrolysates Prepared by Microbial Proteases and Ultrafiltration on Free Radicals and Meat Lipid Oxidation. LWT–Food Science and Technology 2013, 50, 331–335.
- Lee, S.J.; Lim, K.T. Glycoprotein of Zanthoxylum Piperitum DC Has a Hepatoprotective Effect Via Anti-Oxidative Character in Vivo and in Vitro. Toxicology in Vitro 2008, 22, 376–385.
- Udenigwe, C.C.; Aluko, R.E. Chemometric Analysis of the Amino Acid Requirements of Antioxidant Food Protein Hydrolysates. International Journal of Molecular Sciences 2011, 12, 3148–3161.
- Eun, Y.P.; Morimae, M.; Matsumura, Y.; Nakamura, Y.; Sato, K. Antioxidant Activity of Some Protein Hydrolysates and Their Fractions with Different Isoelectric Points. Journal of Agricultural and Food Chemistry 2008, 56, 9246–9251.
- Xu, J.; Chen, Z.Y.; Song, L.Y.; Chen, L.L.; Zhu, J.H.; Lv, S.S.; Yu, R.M. A New in Vitro Anti-Tumor Polypeptide Isolated from Arca Inflata. Marine Drugs 2013, 11(12), 4773–4787.