ABSTRACT
The apple peel (i.e., outermost tissue) plays a crucial role in protecting and preserving apple life, as well as in reducing fruit injury. Apple peels of the Danxia and Starkrimson varieties were compared for their tensile properties, shear properties, and tensile-property variations after 0, 14, and 28 days of storage at room temperature. Apple peel microstructures were observed and imaged using scanning electron microscopy. Cubic polynomials proved superior for quantifying the stress–strain non-linear relationship of peels. With increased storage period, tensile strength and fracture strain gradually decreased but elastic modulus increased. The mechanical indices of Starkrimson peels were greater than those of Danxia peels after the same storage period. Significant differences between cultivars were observed in terms of tensile strength, elastic modulus, and shear strength; significant storage differences also existed for both cultivars. Mechanical properties were closely related to peel microstructures. Strengths and weaknesses mainly depended on the number and width of microcracks on the cuticle, cuticle form, cell aspect radio, and space between cells.
Introduction
In recent years, research on the mechanical properties of apples has mainly focused on two areas: (1) compression, stress relaxation, and creep characteristics of the fruits;[Citation1–Citation4] and (2) tension, compression, and rheology of pulp cut from the fruits.[Citation5–Citation8] Li and Wang[Citation1] measured and analyzed Fuji apples through compression tests and found that different loading rates and fruit shapes affected the bioyield rupture force and deformation values of the fruit. In order to discuss the mechanism and frequency of Qinguan apple damage, the stress relaxation character of the fruit was studied under various deformations, relaxation durations, and deformation rate conditions. It was discovered that fruits bruise when deformation values exceed the threshold value previously determined by research on the influence factor of damage volume.[Citation2] Guillermin et al.[Citation3] compared the technological and rheological properties of two cider apple fruit: Douce Coetligné and Avrolles, as well as the evolution of these properties during post-harvest storage (15 or 30 days) through compression and puncture tests. They found that Douce Coetligné revealed important visco-elastic mechanisms, whereas plastic irreversible deformations played an important role in the breakdown of cell layers in Avrolles. The micromechanical properties of two Iranian apple varieties (Shafi Abadi and Golab Kohanz) were investigated by applying creep and stress relaxation. For both varieties, the decay modulus, equilibrium modulus, time of relaxation, and specific viscosity were measured in the relaxation model, based on the generalized Maxwell model, instantaneous elastic modulus retardation time, and coefficient of viscosity.[Citation4]
Under different storage conditions, the micromechanical properties of two types of apple tissues were investigated by tensile and compression tests using a miniature tensile stage positioned underneath a microscope for simultaneous acquisition of force-displacement curves. It was found that micromechanical properties had no effect on fruit shelf life, and the mechanical properties of cultivars had no differences at the micro- and macro-level.[Citation5] The effect of turgor on the micromechanical properties of apple tissues was studied by applying tensile and compression tests. The stress–strain curves presented as sigmoid in both tests, and increasing turgor produced no significant difference on strain at maximum and failure stress.[Citation6] In order to determine the effects of fruit temperature on firmness during post-harvest handling, tensile and puncture experiments of three apple varieties were conducted. It was found that the relationship between fruit firmness readings and temperature ranging from 0 to 20°C was linear and positive for fruits at harvest, but linear and negative for stored fruits. Under various temperatures, firmness was not influenced by orchard or harvest maturity.[Citation7] To evaluate the efficacy of rheological and textural analyses of fruit tissue, dynamic rheology, compression, and penetration measurements, scanning electron microscopy (SEM) was used to study the macro- and microscopic changes in apple tissue structure during a storage period of 14 days at 20°C. The results showed that apple flesh microstructure had undergone many apparent macroscopic changes in texture and rheology.[Citation8]
As the outermost tissue of apples, the apple peel, has an important role in protecting and preserving apple life, and its character and microstructure are closely related to fruit storage life. The organization structure of 25 kinds of apple fruits was investigated with a light microscope, and results indicated that thicker apple skin with more cell layers could protect the fruit from crush injury, dehydration, and damage.[Citation9] A study on the anatomical structures of three apple varieties during storage showed high storability when the surface cuticle on the skin was thick, the skin had more layers of cells, and the skin cells were smaller and more densely arranged.[Citation10] Gong and Zhang[Citation11] discussed the relationship between the skin structure and storability of the four apple varieties to provide a theoretical basis for preservative storage. They concluded that the fruit had good ability to resist microorganism infection and mechanical injury when the wax coat on the apple skin was thick and epidermis cells were longer and thicker.
There is limited research on the combination of the mechanical properties and microstructure of apple peels. The objectives of this study were: (1) to study the variation in these properties of peel in two apple cultivars and establish the relationship between elastic modulus and strain, and (2) to evaluate the relationship between the mechanical properties and microstructure of apple peels. The results are expected to provide basic technical parameters for establishing a mechanical model of apple fruit and plentiful mechanical indexes of fruit properties. This study also provides some mechanical properties as a reference for selecting apple peels as a biomimetic material prototype for packaging which keeps food fresh.
Materials and methods
Materials
Two types of autumn-ripening apple cultivars, Danxia and Starkrimson, picked from the Shanxi Fruit Institute Academy of Agriculture Sciences in October, 2013, were used for this study. Fruits were selected according to regularity of shape, absence of blemishes and defects, as well as distinct shadowed and sunlit sides. Apples were stored at room temperature (21°C and 80% relative humidity). Fruit peel tension experiments were conducted on day 0, day 14, and day 28, and shear tests of fresh peels were carried out on harvest day. Sample fruits were selected based on their (1) longitudinal diameters: 62.5∼63.4 mm for Danxia and 62.5∼63.4 mm for Starkrimson, and (2) transverse diameters: 71.8∼76. 2 mm for Danxia and 74.1–76.8 mm for Starkrimson.
Samples
Tensile samples
Samples from the shadow side and sunlit side of the peridermis were tested. Longitudinal and transverse samples were simultaneously collected from each side. To ensure a uniform sample size, peels were cut from fruit in 40 × 15 mm strips using a razor blade at room temperature. They were placed on a flat, smooth rubber mat and the inner pulp was carefully removed. The samples were observed under a magnifier to ensure there were no scratches or bores (; left).
For each cultivar, six samples from the same side were analyzed. Tensile tests were carried out with an electronic testing machine (INSTRON-5544, USA). Appropriate samples were immediately mounted in the clamp of the testing machine to prevent water loss, and tested at a rate of 1 mm/min (; right). Sample thickness was 0.210–0.225 mm for Danxia and 0.213–0.220 mm for Starkrimson, as measured with a raster thickness gauge (JC010-1, China) with a measurement range of 0–10 mm and resolution of one thousandth of one millimeter. In tensile tests, the original gauge length of samples was measured as 9.98–10.05 mm for Danxia and 9.98–10.04 mm for Starkrimson, using vernier calipers with a resolution of 0.01 mm.
Shear samples
Shear samples measuring 24 × 24 × t mm (t denotes specimen thickness) were obtained from the sunlit and shadow sides of apples. Specimen thickness was 0.165–0.197 mm for Danxia and 0.174–0.198 mm for Starkrimson, as checked with a raster thickness gauge. A setting jig, formed by the film perforation and cylinder perforation composition, was used to ensure uniform force during testing. In the test, specimens were fixed between the upper and lower film, and the cylinder perforator, with 14 mm diameter, was fixed on the universal testing machine (as shown in ). In shear experiments, the test speed was 1 mm/min, and the average values were measured after 15 repetitions per cultivar.
As the outermost tissue, fruit peel bears the action of internal and external load in the process of natural growth of fruit, so it inevitably has the ability to withstand shear to prevent dislocation under load. Shear strength () is an important indicator of peel shear deformation, calculated as
, where
represents shear failure force;
represents the diameter of cylinder perforator; and
represents the peel specimen thickness.
SEM sample observation
Tissue blocks (fruit sections) were cut from the shadow and sunlit sides of apple periderm. The samples were then fixed with a 3% solution of glutaraldehyde at a low temperature (0~4°C) for 2 h. The segments were then washed three times with the same buffer for 15 min per session and dehydrated in ethanol series. They were displaced by tert-butyl alcohol and freeze-dried by a JEOL JFD-320 instrument. Finally, the samples were coated with platinum using a JEOL JFC-1600 Sputter Coater and observed with a JEOL JEM-6490 LV SEM. In order to determine the microstructure of the fracture surface, samples that broke were immediately examined by SEM following the steps previously outlined.
Data analysis
Measurements, such as tensile strength, elastic modulus, fracture strain, and shear strength, were subjected to analysis of variance (ANOVA) in the procedure of Statistical Analysis System (Version 8, SAS Institute, Cary, NC, USA), with a 95% confidence interval. The microstructure indexes of peels were measured, using MATLAB software (Version 7, the Mathworks, Natick, USA).
Results and discussion
Tensile test of fresh peel
Relation between elastic modulus and strain
shows a typical stress–strain curve depicting a non-linear relationship. The curve presents a similar S-shape and has no obvious bioyield point. This was also observed by Wang et al.[Citation12] When the load reaches rupture force the curve begins to fracture, and the break-point lays at a point.
The apple peel is a biological tissue and the peel stress–strain relation does not obey Hooke’s Law. However, the mechanical property indexes of peels, such as strength and elasticity, determine the degree of fruit injury and destruction during harvest, packing, transport, and storage.[Citation13–Citation15] Meanwhile, the crack resistance and malleability of apple peels affect their tensile strength.[Citation16,Citation17] To determine the strength and elasticity of different deformations, the test data of fresh peels, collected by system and only contained the data before curve rupture and at curve break, based on the principle the least-square method of curve matching and was used the procedure of Statistical Analysis System for line regression. Results showed that the cubic curve satisfied the chosen function. The fitting polynomial was:
where is tensile stress at axial direction, MPa;
is fitting coefficients; and
is the tensile strain. On the basis of the fitting polynomial, the formula of elasticity was defined as:
Together, the two equations determine the strength and elasticity values of any peel deformation quantity. These equations provide a quantitative expression to establish a tensile non-linear equation of apple peel and contribute to non-linear research on peel material.
and , respectively, summarize the fitting coefficients and the tensile strength, elastic modulus, fracture strain, fitting error for peels from four parts of Danxia and Starkrimson cultivars: the sunlit longitudinal, the shadow longitudinal, the sunlit transverse, and the shadow transverse. The polynomial fitting curves of Danxia and Starkrimson stress–strain are shown in .
Table 1. Curve fitting coefficients of fresh peels under tensile loading.
Table 2. Experimental value and fitted error of fresh peels under tensile loading.
Tensile property analysis
shows that the mechanical parameters of fresh peel samples, including tensile strength, elastic modulus, and fracture strain, differed in different parts of each cultivar, as well as in the same parts of different cultivars. Experimental data showed that the mean and standard deviation of tensile strength for all peel samples were 2.19 and 0.25 MPa, respectively, for Danxia, and 2.46 and 0.18 MPa, respectively, for Starkrimson. The mean and standard deviation of elastic modulus were 18.81 and 2.50 MPa, respectively, for Danxia, and 22.81 and 3.01 MPa, respectively, for Starkrimson. It was found that the stiffness of the apple peel was much larger than that of apple pulp.[Citation5,Citation6] For mean values of tensile strength and elastic modulus, the longitudinal sample had greater tensile strength and elastic modulus than the transverse on the same side of each cultivar, and the sunlit samples had greater tensile strength than the shadow samples. Sunlit longitudinal samples of each cultivar had the highest tensile strength for all four peel parts. Starkrimson cultivars were unique in that tensile strength of the sunlit side was significantly different (p ≤ 0.05) from that of the shadow, and tensile strength of the longitudinal samples showed a significant difference (p ≤ 0.05) from that of the transverse samples. Another significant difference (p ≤ 0.05) between longitudinal and transverse samples showed that the former were more elastic than the latter. Starkrimson demonstrated higher tensile strength and elastic modulus than Danxia for the same peel parts. There was a significant difference (p ≤ 0.001) between the tensile strength of Starkrimson and that of Danxia, and the elastic modulus was more pronounced (p ≤ 0.01) for Starkrimson samples than for Danxia samples. The foregoing analysis shows that apple peel has an anisotropic heterogeneous behavior. Starkrimson also proved to have a greater ability to resist deformation and damage than Danxia peels.
Test data also indicated that the mean and standard deviation of fracture strain for all peel samples were 0.19 and 0.06, respectively, for Danxia, and 0.20 and 0.03, respectively, for Starkrimson. For mean values of fracture strain, the values of longitudinal samples were significantly larger (p ≤ 0.05) than the transverse samples. This indicated that peel was horizontally more compressible and malleable than vertically. With two cultivars, no significant effect was observed for fracture strain. Note that expansibility may not vary much for both peels. From the fitting error of the tensile strength of peel, it was discovered that error was minimal at less than 10 per 1000. The fitting error of the elastic modulus was relatively large, but also less than 10%. Error analysis indicated that the fitting cubic polynomials had a superior description of the non-linear relation of apple peels under tension.
Shear test of fresh peel
Shear force-deformation curve
A typical result of shear load-displacement curve on peel is shown in . The shear curve of the peel presents three sections. The first section ranges from 0 to 0.6 mm, and in this section, loads are small and almost constant. This is because the sample which comes from a sphere fruit has a buckled state of its own, so when the cylinder perforator begins to contact the peel sample, the loads make the buckling states of cells extend gradually. In the second section, which ranges from 0.6 to 0.86 mm, the buckling states of the sample fade away. The tissue cells of the peel shift to fill the intercellular spaces between them when the cylinder perforator contacts and squeezes the sample. Cell turgidity then increases with the increase of cell deformation. The third section ranges from 0.86 to 1.0 mm, and cell turgidity increases sharply with further compression from the perforator. The osmotic balance of cell sap is disrupted, ultimately leading to cell rupture. Thus, when load increases sharply and reaches the maximum (b point), the sample suffers shear failure. B acts as the rupture point, at which significant peel shear failure occurs, and its corresponding strength values are considered as shear strength. There is only one peak on this curve, indicating that shear failure of all peel cells occurs at the same time. The peel fracture specimen is shown in .
Shear property analysis
Shear strength values of two types of peel sample are shown in . Shear strength ranged from 2.61 to 4.81 MPa for Danxia, and from 3.15 to 6.38 MPa for Starkrimson. Shear values changed more than tensile strength, and the ratio of ultimate to average shear strength of the sunlit side reached 1.4. Within the same cultivar, the mean shear strength of the sunlit side was greater than that of the shadow side, and there was only a significant difference (p ≤ 0.05) between them for the Daxia cultivar. Between the two cultivars, Starkrimson had higher shear strength than Danxia for the same peel part, and the shear strength of Starkrimson peel had a significant difference (p ≤ 0.001) from the Danxia peel. This shows that the apple peel also has heterogeneous behavior and the anti-shear capacity of sunlit peel was higher than that of shadow peel. It also shows the anti-shear capacity of Starkrimson was larger than that of Danxia.
Table 3. Means and standard errors of shear strength of apple peels.
Tensile properties during storage
For two peel cultivars, the changes of mechanical properties obtained from four parts, including sunlight longitudinal, shadow longitudinal, sunlight transverse, and shadow transverse (designated as P1, P2, P3, and P4, respectively) during storage, are shown in . With prolonged storage of the fruits, it was observed that average tensile strength was significantly less in both cultivars. The average fracture strain of each also gradually decreased. The effect of storage time was significant for the tensile strength and fracture strain of both cultivars (p ≤ 0.001). The average elastic modulus of peels gradually increased as the storage time increased. There were no significant differences in elastic modulus for the cultivars. For the same storage period, it was observed that average tensile strength, elastic modulus, and fracture strain of the Starkrimson samples were higher than those of Danxia samples from the same part. A markedly significant difference (p ≤ 0.001) in average tensile strength was found between cultivars at day 14 and day 28. The elastic modulus of peel samples also significantly differed (p ≤ 0.05). Meanwhile, for the peel samples, the average fracture strain of Starkrimson became significantly more extensible than that of Danxia at day 28 (p ≤ 0.05).
Image analysis
Cross-section microstructure of fresh peels
Based on the cross-section images obtained, the apple peels are composed of cuticle, epidermis, several layers of hypodermis, and the cuticle covers on the fruit surface.[Citation12] The structure of fruit cuticle and epidermis was found to be different on different sides of each cultivar. Danxia peel cuticle had an uneven and inconsistent arrangement, and was characterized by a deep V-shaped depression which was deeper on the shadow side. In contrast with Danxia peel, Starkrimson cuticle had a uniform and consistent arrangement, and the V-shaped depression on the cuticle was relatively shallow, but also comparatively deep on the shadow side. Both cultivars had epidermis that was a single layer of circular or ellipse cells, but the Danxia epidermis cells were loosely arranged and showed “fragmentation” characteristics ().
Figure 8. Cross-section microstructure of fresh peels observed using SEM: A: Danxia sunlit side; B: Danxia shadow side; C: Starkrimson sunlit side; D: Starkrimson shadow side.
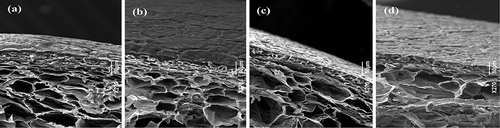
The microstructure indices of Danxia and Starkrimson[Citation12] peels were measured with MATLAB software (). The Starkrimson cuticle was 4.6% thicker than Danxia. It varied from 10.37 to 18.52 μm, with an average of 12.87 μm. Epidermal cells were 8% shorter, and width was 0.8% greater than Danxia. Average length and width were 16.3 and 8.48 μm, respectively. Note that the aspect ratio of Danxia epidermal cells was more than 9.3% larger than Starkrimson cells, and two adjacent cell spaces of the Danxia epidermis were larger than that of Starkrimson. There was a greater variation in measurement data for Danxia than for Starkrimson, and the two cultivars had significant differences in cuticle layer thickness and cell aspect ratio.
Table 4. The parameters of microstructure of peel.
Certain differences presented when studying the relationship of mechanical properties and microstructure indices. The aspect ratio of epidermal cells was smaller for Starkrimson peel, whose mechanical properties were larger, and it may be considered that, when a rounded cell is deformed under load, its stress concentration is relatively lower, subjecting it to great tension or compression force. This result agrees with Allende et al.,[Citation14] who discovered that round cells were probably stronger than elongated cells, and “Tradiro” hypodermal cells had lower susceptibility to puncture injury in the round hypodermis and parenchyma layers. Wang et al.[Citation12] also discovered that rounder cells may resist higher rupture stress than elongated cells. The larger space of epidermal cells may allow larger movements between cells under tensile loading, creating stronger peel tensile properties. However, it was observed that despite the larger spaces between Danxia epidermal cells, the tensile strength of Danxia peel was lower. This may be because Danxia cuticle is embedded deeper in the epidermal layer, which could lead to less sliding between cells. Alamar et al.[Citation5] reported that deformation of air spaces in tissue represents an important portion of total tissue volume, and the difference of the deformation could explain the high strain values obtained for compression tests compared to tensile tests.
The fracture morphology of fresh peels
The fracture morphology of tensile had an irregular and obviously undulated surface (), and the fracture process of samples showed the viscoelastic behavior of resisting brittle fracture. These results confirmed the stress–strain curve that tensile stress never rapidly decreased to zero but rather had smaller fluctuations when the stress–strain curves began to tear away. The fracture mechanism of the peel was not the tearing between cells but rather, direct rupture of cells, and tensile strength was relatively larger.
Surface microstructure of peels for storage
According to scanning microscopy observations, the microstructures of fruit surfaces changed much during the storage period for both cultivars (). At day 0, microcracks (average = 12.8 μm) were observed on Danxia peels from the shadow side but not on Danxia peels from the sunlit side. The microcrack numbers in shadow and sunlit peels clearly grew, and their widths in shadow and sunlit aspects averaged 14.87 and 8.07 μm at day 14, respectively. At day 28, components of the cuticle layer began to crack and separate, and microcracks were clearly distinguishable. However, for the Starkrimson cultivar, it was found that there were hardly any microcracks on peels from the sunlit side, and the microcracks on shadow side peels had an average width of 5.68 μm on day 0. On day 14, microcracks appeared on the sunlit side peel with a width of 5.13 μm. On the shadow side peel, microcracks increased, and their width increased to 9.11 μm. On day 28, the cuticle structure suffered more serious crushing, which led to difficulties identifying boundaries of the microcracks.
Figure 10. The surface microstructure of peels during storage: A: Danxia sunlit side; B: Danxia shadow side; C: Starkrimson sunlit side; D: Starkrimson shadow side.
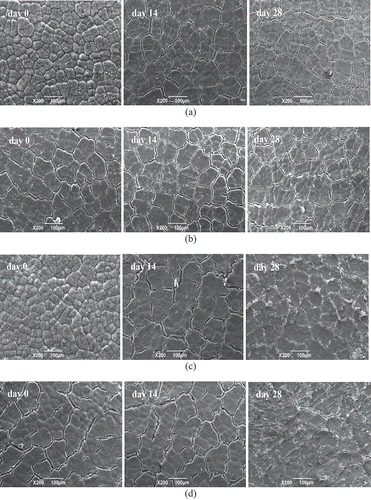
Clearly, for any cultivar, there are more microcracks, which are of greater width, on shadow side peels. This may be because mechanical properties of the shadow side samples were below those of sunlit samples. Wang et al.[Citation12] also discovered a negative effect for the width and quantity of microcracks on peel mechanical properties. Excessive water loss and nutrient runoff from peel tissues, together with increasing number width of microcracks during storage, may result in peel tensile strength trending down over time. By prolonging storage time, peel elastic modulus increased constantly, possibly because cuticle fragmentation had less locking force among epidermis cells, resulting in increased length and sliding distance among epidermis cells.
Conclusions
In order to determine the strength and elasticity among different deformations of apple peel, the fitting cubic polynomials were obtained. They provided a superior description of the non-linear relation of apple peels under tension and contributed to nonlinear research on peel material. On harvest days, the average tensile strength, elastic modulus, and fracture strain for Starkrimson samples were significantly larger than those of Danxia samples. With increased storage time, the tensile strength and fracture strain were respectively reduced by 13 and 10% in Danxia peel, and 10 and 17% in Starkrimson peel, but the elastic modulus was increased by 3% in Danxia and 7% for Starkrimson. With the same storage period, average tensile strength and elastic modulus of Starkrimson samples were significantly higher than those of Danxia samples (p ≤ 0.05), and fracture strain of Starkrimson became significantly larger than that of Danxia at day 28 (p ≤ 0.05). The ability of Starkrimson peels to resist deformation appeared higher than that of Danxia peels, and the expansibility of Starkrimson cracks was higher than that of Danxia cracks. For fresh peel samples, shear strength changed greatly, particularly the ratio of ultimate and average shear strength of sunlit sides, which reached 1.4 for both types of peel. The highest average shear strength was measured on the samples of sunlit side peel. Average shear strength was significantly higher for Starkrimson samples than for Danxia samples (p ≤ 0.001). It was indicated that the apple peel behaves in a heterogeneous manner; anti-shear capacity is higher for the sunlit side than the shadow side, and the anti-shear capacity is greater for Starkrimson than Danxia.
Relating the mechanical properties and microstructure of peel, it was found that the tension properties and anti-shear capacity of peel were relatively larger when epidermal cells are rounded. Moreover, the fracture morphology of fresh peels reflected a viscoelasticity behavior. As storage time was prolonged, cuticle components were lost. Nonetheless, the tensile strength and elastic modulus of Starkrimson was superior to that of Danxia (p ≤ 0.05). This shows that cuticle form influenced the mechanical properties of the peel, and Starkrimson peel tissue structure has a higher density. This may also explain why Starkrimson had better resistance to cracks during the fruit development. This may have also caused the core browning of Starkrimson fruit during the storage period. In summary, this research provides knowledge for better evaluation of apple peel. It also provides basic technical parameters and mechanical indexes to fruit properties for establishing a mechanical model of the apple fruit.
References
- Li, X.Y.; Wang, W. A Study on Compressive Properties of Apple. Acta University Agriculture Boreali-occidentalis 1998, 26(2), 107–110.
- Li, X.Y.; Wang, W.; Zhu, J.P.; Chen, H. A Study on Stress Relaxation Properties of Mechanical Damage of Apple. Transaction of the Chinese Society for Agricultural Machinery 1997, 28, 70–74.
- Guillermin, P.; Dupont, N.; Le Morvan, C.; Le Quéré, J.-M.; Langlais, C.; Mauget, J.C. Rheological and Technological Properties of Two Cider Apple Cultivars. LWT–Food Science and Technology 2006, 39(9), 995–1000.
- Chakespari, A.G.; Rajabipour, A.; Mobli, H. Anisotropic Relaxation and Creep Properties of Apple (cv. Shafi Abadi and Golab Kohanz). Advance Journal of Food Science and Technology 2010, 2(4), 200–205.
- Alamar, M.C.; Vanstreels, E.; Oey, M.L.; Moltó, E.; Nicolaï, B.M. Micromechanical Behaviour of Apple Tissue in Tensile and Compression Tests: Storage Conditions and Cultivar Effect. Journal of Food Engineering 2008, 86(3), 324–333.
- Oey, M.L.; Vanstreels, E.; De Baerdemaeker, J.; Tijskens, E.; Ramon, H.; Hertog, M.L.A.T.M.; Nicolaï, B. Effect of Turgor on Micromechanical and Structural Properties of Apple Tissue: A Quantitative Analysis. Postharvest Biology and Technology 2007, 44(3), 240–247.
- Johnston, J.W.; Hewett, E.W.; Harker, F.R.; Hertog, M.L.A.T.M. Physical Change in Apple Texture with Fruit Temperature: Effects of Cultivar and Time in Storage. Postharvest Biology and Technology 2001, 23(1), 13–21.
- Varela, P.; Salvador, A.; Fiszman, S. Changes in Apple Tissue with Storage Time: Rheological, Textural and Microstructural Analyses. Journal of Food Engineering 2007, 78(2), 622–629.
- Deng, J.G.; Liu, G.C.; Yin, G.C. Investigation on the Organization Structure of Apple Fruits. Journal of Fruit Science 1995, 12(2), 71–74.
- Liu, G.C.; Ma, H.Y.; Lu, D.G.; Qin, S.J.; Du, G.D.; Wang, H. Research on the Change of “Hanfu” Apple Fruit Anatomic Structure and Quality During Storage. Northern Horticulture 2012, 15, 1–4.
- Gong, Y.M.; Zhang, F.M. A Study on a Link Between Apple Peel Structure and Storability. Shan Xi Fruits 1998, 2, 4–5.
- Wang, J.X.; Cui, Q.L.; Li, H.B.; Liu, Y.P. Experimental Research on Mechanical Properties of Apple Peels. Journal of Engineering and Technology Science 2015, 47(6), 688–705.
- Desmet, M.; Lammertyn, J.; Verlinden, B.E.; Nicolaï, B.M. Mechanical Properties of Tomatoes as Related to Puncture Injury Susceptibility. Journal of Texture Studies 2002, 33(33), 415–429.
- Allende, A.; Desmet, M.; Vanstreels, E.; Bert, E.; Verlinden, B.E.; Nicolaï, B.M. Micromechanical and Geometrical Properties of Tomato Skin Related to Differences in Puncture Injury Susceptibility. Postharvest Biology and Technology 2004, 34(2),131–141.
- Krishna, K.S.; Sreenivasula, R.B. Post-Harvest Physico-Mechanical Properties of Orange Peel and Fruit. Journal of Food Engineering 2006, 73(2), 112–120.
- Li, K.Z.; Gao, Z.S. Preliminary Studies on the Mechanism of Fruit Cracking in Chinese Jujube. Journal of Fruit Science 1990, 4, 221–226.
- Cline, J.A.; Sekse, L.; Meland, M.; Webster, A.D. Rain-Induced Fruit Cracking of Sweet Cherries: I. Influence of Cultivar and Rootstock on Fruit Water Absorption, Cracking and Quality. Acta Agriculturae Scandinavica, Section B—Plant Soil Science 1995, 45(3), 213–223.