ABSTRACT
The dried petals of Echium amoenum Fisch. & C.A. Mey. are used for the treatment of many diseases but there are not any reports about the effects of drying conditions on their bioactive compounds. A complete 32 factorial design was used, where the independent variables were drying temperature (40°C–60°C) and air flow rate (0.5–1.0 m/s). The bioactive compounds studied were total phenolic, total flavonoid and anthocyanin contents, and antioxidant activity. The desirability index was used to predict the optimal drying conditions. Both independent variables were statistically significant. The optimal drying conditions were air velocity of 0.86 m/s at 60°C. The results show that the drying conditions play an important role in determining the final quality of the product, content of its bioactive compounds, and minimum drying time.
Introduction
Echium amoenum (Boraginaceae) is a biennial or perennial herb indigenous to the narrow zone of northern part of Iran and Caucasus, where it grows at an altitude ranging from 60 to 2200 m.[Citation1] Four species of Echium L. can be found in Iran.[Citation2] It is an important medicinal plant in traditional Iranian medicine.[Citation3,Citation4] Dried violet-blue petals of E. amoenum have been known for medicinal properties such as demulcent, anti-inflammatory, analgesic, and sedative, and used especially for the treatment of common cold and sore throat in the Iranian folk medicine.[Citation3–Citation6] There are some reports about the anxiolytic effect of E. amoenum and some reports that it has the capacity to increase the cellular immune response.[Citation7] Many countries are registering their standardized herbal drugs with numerous post-harvest preservation and management methods, but many are not economically feasible. Perhaps one of the most widely used herb preservation methods is dehydration. Care must be taken to set the drying parameters so that the herbs are not exposed to excessively high temperatures, causing major losses of their medicinal, culinary, nutraceutical, and visual properties, which would negatively affect the product value. Under the right conditions, drying can produce a sufficiently shelf-stable product without major losses in herb value.[Citation8]
Polyphenolic compounds inhibit several oxidative enzymes and inflammatory agents and have been shown to have anti-allergenic, antiviral, antibacterial, antifungal, antitumor, atheroprotective and hypoglycemic properties, and antihaemorrhagic activity. The antioxidative activity of food-based polyphenolics is based on the cumulative effects of chemical and enzymatic processes that occur during processing and/or storage.[Citation9] During drying, enzymatic processes in fresh plant tissues can lead to significant changes in the composition of bioactive constituents of herbs. Especially phenolic compounds, ascorbic acid, and pigments are highly susceptible to degradation during processing, resulting in colour changes of the material and the loss of physicochemical properties. Fluidized beds are widely used in industries for drying of fertilizers, chemicals, pharmaceuticals, and minerals. The increasing application of fluidized bed drying for agricultural materials is due to the evolving designs of this method for fluidization of coarse material, which is rather difficult to fluidize. Compared with other drying methods, fluidized bed offers advantages such as high heat capacity, improved heat and mass transfer rates between the phases, and ease of handling and transport of fluidized solids.[Citation10]
Despite several reports that drying is generally unfavourable due to the possibility of inducing oxidative decomposition, either enzymatically or by thermal degradation of polyphenols, high antioxidant potential of dried fruits was reported by Kamiloglu and Capanoglu.[Citation11] Additionally, increases in phenolic and flavonoid contents, as well as antioxidant activity, due to drying have even been reported. In this sense, water plays an important role in biological reactions, including physical, chemical, microbiological, and enzyme reactions.[Citation9] Although there have been several investigations of the effects of processing on anthocyanin and polyphenolic content of various medicinal plants,[Citation12,Citation13] to the best of our knowledge, there is no published work on the impact of drying of E. amoenum petals on their bioactive compounds. Investigation of optimal thermal processes for the drying of E. amoenum petals will provide an opportunity for the drug industry to produce a highly bioactive dried product. Therefore, different drying conditions were applied, and their influence on physicochemical quality, total phenolic, total flavonoid, and anthocyanin contents, as well as the percentage of radical scavenging activity was investigated, to select the optimal drying conditions and provide an insight in the physicochemical properties and the concentration of bioactive compounds of this plant.
Material and methods
Drying process
Fresh E. amoenum petals used in the drying experiments were collected from Ali-Abad (Golestan Province, Iran) between March and May. The petals without blemishes were selected for drying. They were stored at (4 ± 0.5)°C prior to the beginning of the tests. In order to determine the effect of drying conditions, the E. amoenum petals were dried in a fluidized bed dryer at 40°C, 50°C, and 60°C and air velocities of 0.5, 0.75, and 1 m/s. Drying experiments in a laboratory-scale fluidized bed dryer were described by Nadi.[Citation14]
Experimental design
The conditions applied in the experimental setups used for the drying of petals are based on a factorial design nm, where n is the number of levels and m is the number of factors. The air drying temperature and velocity were the two factors under study (m = 2), each at three levels (n = 3). Thus, nine treatments were required (32). shows the decoded levels of the variables used in the experimental design to represent the experiments.
Table 1. Matrix of the experimental design (32).
Preparation of extracts
All samples were extracted with 50% methanol (v/v) followed by extraction with 70% acetone (v/v) according to the method described by Rufino et al.[Citation15] to obtain the crude extract, which was later used in determining the phenolic content and in the antioxidant activity tests.
A mass of 2 g of the sample of dried petal powder was transferred to a 100 mL beaker and 4 mL of 50% methanol was added. The mixture was homogenized using a mixer with a steel shaft (ShimiFann Co., Tehran, Iran) and then left to rest for 1 h at room temperature. The supernatant was transferred to a 100 mL volumetric flask. A volume of 4 mL of 70% acetone was added to the residue from the first extraction, which was then homogenized and left to rest for 1 h at room temperature. The obtained supernatant was transferred to the same volumetric flask containing the first supernatant, and the volume was completed to 100 mL with distilled water. All extractions were performed in triplicate.
Determination of antioxidant activity
The DPPH method is based on the scavenging of the free DPPH (1,1-diphenyl-2-picrylhydrazyl) radical by antioxidants, measured as absorbance at 517 nm. The electrons are transferred from an antioxidant compound to the free radical, DPPH, which loses its purple colour when reduced. Briefly, 1 mL (0.1 mM) of DPPH solution in methanol was mixed with 3 mL of sample solution in water. Finally, after 30 min, the absorbance was measured at 517 nm. Decrease of the absorbance of DPPH solution indicates an increase of the DPPH radical scavenging activity. To assess the radical scavenging activity, the percentage of inhibition was obtained using the following equation:
where Acotrol is the absorbance of control reaction and Asample is the absorbance in the presence of test or standard sample.
Determination of total phenolic content
Total phenolic content was estimated using Folin-Ciocalteu’s assay.[Citation16,Citation17] A mass concentration of 10 mg/mL of extract was mixed with 1.0 mL of Folin-Ciocalteu’s reagent, 0.8 mL of Na2CO3 was added and the volume was then increased to 10 mL using water/methanol (4:6) mixture as diluting fluid. Absorbance was read at 740 nm after 30 min using a UV/Vis spectrophotometer (type 108, Systronics, Ahmedabad, Gujarat, India). Standard calibration curve was obtained using 0–800 mg/L of tannic acid, and total phenolic content was expressed in milligram of tannic acid equivalents (TAE) per 100 g of sample.[Citation18]
Estimation of total flavonoids
The total flavonoid content (TFC) was estimated using the Dowd method as adapted by Arvouet-Grand et al.[Citation19] A volume of 5.0 mL of 2% aluminium trichloride in methanol was mixed with the same volume of the extract solution (10 mg/mL). Absorbance readings at 415 nm using spectrophotometer (type 108, Systronics) were taken after 10 min against a blank sample consisting of the extract solution with 5.0 mL of methanol without AlCl3. The TFC was determined using a standard curve with quercetin and expressed in grams of quercetin equivalents per 100 g of sample.
Determination of anthocyanins
Determination of anthocyanin content was carried out using the method of Wagner.[Citation28] A mass of 0.1 g of sample was soaked in 10 mL of acidified methanol (methanol/HCl = 99:1, v/v). The tissue was crushed and kept at 25°C for 24 h in the dark. The extracts were then centrifuged at 4000g for 5 min at room temperature. The absorption rate of the supernatant was read by spectrophotometer (type 108, Systronics) at 550 nm. To calculate the amount of anthocyanins, the molar absorption coefficient of 33,000 mol–Citation1 cm–Citation1 was used and anthocyanin content was expressed in micromoles per gram of fresh mass.
Energy consumption
During the experiments, total energy needed for drying one charge of the heater and energy requirement for drying 1 kg of fresh E. amoenum petals were calculated for each experiment using the following equation:[Citation20]
where Et is total energy (kWh) needed for drying, A is tray area (mCitation2), v is air velocity (m/s), is air density (kg/mCitation3), tdrying is total drying time (h),
is temperature difference (°C), and ca is the specific heat (J/kg °C).
Results and discussion
Effect of drying conditions on antioxidant activity
Drying process generally results in a depletion of naturally occurring antioxidants in raw materials from plants.[Citation21] Intense and/or prolonged thermal treatment may be responsible for a significant loss of natural antioxidants, as most of these compounds are relatively unstable.
The antioxidant activity determined using DPPH radical was evaluated in the samples both before and after drying. The evaluation of different reaction kinetics of dried E. amoenum petal extracts depended on the nature of the tested antioxidants. As can be observed in , drying temperature and air velocity had a significant influence on the antioxidant activity (p < 0.01).
Table 2. Total antioxidant capacity (TAC) of fresh and dried Echium amoenum petals under different drying conditions.
Antioxidant capacity of fresh E. amoenum petals was 89.70% and it decreased progressively with drying due to heat damage and reduction in moisture content. The resulting decrease of antioxidant capacity when the product reached the final moisture level of 0.05 kg/kg of dry mass at air velocity of 0.5 m/s was 34.19%, 43.74%, and 82.16% when drying at 40°C, 50°C, and 60°C, respectively, compared with fresh samples. Corresponding values at air velocity of 0.75 m/s were 45.32%, 37.68%, and 24.38%, and at air velocity of 1.00 m/s were 49.94%, 31.82%, and 43.03% at the same respective temperatures. For the prediction of the antioxidant capacity as a function of the independent variables, the following equation was used:
where TAC is total antioxidant capacity, T is temperature, and v is air velocity. The data treatment shows that 88.52% of the antioxidant activity variability was explained by Eq. (3). The residuals were also appropriately distributed. From this equation, it is possible to construct the plot shown in , where the effect of temperature and air velocity on the antioxidant activity can be seen. At intermediate air velocity, the antioxidant activity was higher at lower temperature. Decrease in the antioxidant activity as a consequence of the increase of temperature has been reported by Wang and Lin,[Citation22] Wang et al.,[Citation23] Mori et al.,[Citation24] and Morales-Delgado et al.[Citation25] Petals dried at 50°C and 1 m/s had the highest DPPH values (61.16%), while petals dried under other conditions had lower antioxidant activity. The possible reason is that a lower decomposition of lycopene, α-tocopherol, and β-carotene at this temperature leads to a higher retention of antioxidant activity. Kha et al.[Citation26] and Auisakchaiyoung and Rojanakorn[Citation27] reported that the loss of antioxidant activity of gac aril increased when the air drying temperature was increased from 40°C to 80°C.
Effect of drying conditions on total phenolic content
Phenolic compounds structurally involve an aromatic ring that bears one or more hydroxyl substituents. They range from simple phenolic molecules to highly polymerized compounds.[Citation28] Scientific works that study the effects of drying on vegetable phenolic compounds concluded that they vary from having no effect at all,[Citation29] to significant losses[Citation30–Citation34] or enhancement of total phenolic content (TPC).[Citation35–Citation37] Different authors observed wide variations in the TPC in different medicinal plants, or even within the same medicinal plant. These differences may be attributed to the complexity of these groups of compounds as well as to extraction and analysis methods.[Citation45] Evaluation of TPC losses in dried E. amoenum petals allowed the determination of the effect of different drying conditions on TPC change.
Statistical analysis of variance (ANOVA) clearly shows the differences between the TPC values (p < 0.01) among the treatments. According to Nadi,[Citation14] the effect of air velocity and drying temperature on TPC losses is highly significant (p < 0.01), that is at 60°C, the highest drying temperature, degradation of total phenolics was the weakest (30.86%, 24.69%, and 23.46% at air velocity of 0.5, 0.75, and 1 m/s, respectively). High drying temperatures can cause inactivation of enzymes responsible for the oxidation of polyphenols, such as polyphenol oxidases and peroxidases, present in plant materials,[Citation31,Citation36] while the formation of phenolic compounds at high temperatures could be caused by the availability of phenolic precursor molecules through non-enzymatic interconversion between phenolic molecules,[Citation38] which is probably due to high convective forces acting at the air–solid interface, and retarding heat diffusion into the petals. Also, the formation of phenolic compounds at high temperatures can possibly be explained by their liberation during drying. Phenolic glycosides are localized in hydrophilic regions of cells such as vacuoles and apoplasts or as other soluble phenols in the cytoplasm and in the cell nuclei,[Citation39] where they seem to get a protective heat shield by the cell wall material. Internal resistance to heat diffusion is therefore an important parameter to be considered during heat treatment when regarding the quality of dried petals. Haard and Chism[Citation40] determined that the phenolic compounds in plants act as metabolic intermediates and normally accumulate in cellular vacuoles. Similarly, maximum TPC reduction was observed in onion dried at 50°C, and lower reduction at 70°C.[Citation41] Gupta et al.[Citation36] and Garau et al.[Citation42] concluded that longer drying times result in higher reduction of TPC in orange by-products, even at lower drying temperature. TPC reduction at lower temperature can also be caused by the binding of polyphenols with other compounds or by the modification of their chemical structure following heat treatment, which would prevent them from extraction and determination by the adopted methods.[Citation43,Citation44] The following equation was derived from the experimental data in order to express the relationship between the TPC and the independent variables. Statistical results show that 91.06% of TPC variability can be explained by this equation, with independent and identical distribution:
The effect of temperature and air velocity on TPC is shown in . A non-linear behaviour can be observed, with higher TPC values at higher temperatures (60°C).
Effect of drying conditions on flavonoid content
Flavonoids constitute the largest group of plant phenols and account for over half of the 8000 naturally occurring phenolic compounds.[Citation45] The potential health benefits of flavonoids and other phenolic compounds are supported by epidemiological and in vitro evidence of antioxidant, cardioprotective, and anticarcinogenic activities. They also protect against other non-transmissible chronic diseases.[Citation46] shows that air velocity and drying temperature were significant factors that affect the variation of TFC during fluidized bed drying (p < 0.01). It can be seen that the concentration of total flavonoids in the extracts of dried petals was always lower than in those of fresh petals; however, the flavonoid content gradually increased as the drying temperature increased. The highest TFC was in the petals dried at 60°C. These compounds might form at high temperatures because of the availability of precursors of these molecules by non-enzymatic intermolecular interconversion.[Citation47] These findings are in agreement with previous investigations by Ben Haj Said et al.[Citation48] of dehydrated Allium roseum leaves and Duzzioni et al.[Citation49] of drying acerola (Malpighiaemarginata D.C.) residue. TFC loss in dried petals varied from 6.93% to 68.26% compared with fresh petals, and was relatively lower than the TPC loss. The loss of flavonoids was lower when drying in fluidized bed at 60°C (19.57%, 6.93%, and 23.91% at air velocity of 0.5, 0.75, and 1 m/s, respectively) than at other measured temperatures. The loss of flavonoids during drying might be due to the process conditions, in particular the temperatures and the duration.[Citation50] Davey et al.[Citation51] reported that thermal processing can cause thermal breakdown of the phytochemicals, affecting the integrity of the cell structure, which then results in the migration of components, and leads to losses by leakage or breakdown through various chemical reactions involving enzymes, light, and oxygen. According to Korus[Citation33] flavonoid loss can also be explained by their oxidation, which is promoted by hot air drying.
Table 3. Total flavonoids content (TFC) of fresh and dried Echium amoenum petals under different drying conditions.
From the experimental results an equation has been obtained to describe the variation in the TFC as a function of the independent variables (Eq. 5). The statistical results show that 97.15% of the TFC variability is explained by Eq. (5) within dependent distribution of the residuals.
The response surface plot of the TFC after drying is shown in . It can be observed that at 60°C and 0.8 m/s, the TFC was at its highest level (0.22 g/mg of dry matter).
Effect of drying conditions on anthocyanin content
Anthocyanins form the largest group of water-soluble natural pigments from plants that provide red, blue, and violet colours to flowers, fruits, vegetables, other food products. They are active compounds, sensitive to pH, temperature, light, oxygen, enzyme, and sulphur dioxide. Anthocyanins have been reported to be destroyed by high heat during processing and storage of food.[Citation52] Total anthocyanin content in fresh and dried E. amoenum petals is shown in . Fresh samples had an average anthocyanin content of 0.58 μmol/g (dry mass basis), while drying decreased it. The effect of different drying conditions was found to be significantly different at p ≤ 0.01. The mechanism of thermal degradation of anthocyanins is not understood well, although a number of pathways have been proposed. Their degradation depends on the temperature and duration of treatment.
Table 4. Anthocyanin content of fresh and dried Echium amoenum petals under different drying conditions.
Although the anthocyanin content was reported to decrease at higher temperature, in this work the drying air temperature of 60°C proved to be the optimum to retain the highest amount of phenolic compounds. This result is similar to that reported by Wiriya et al.,[Citation53] Garba et al.,[Citation54] and Katsube et al.[Citation55] and is in agreement with the previous finding that processing involving high temperature and short treatment time is recommended for maximum anthocyanin retention in food.[Citation56] If heating is not extreme or prolonged, the process of degradation is partially reversible, which is why at high temperature anthocyanin content is high.
Anthocyanin content in samples treated at 60°C was significantly higher than in those treated at 40°C and 50°C. The lower anthocyanin content at 50°C might be due to longer exposure to heat. In the work by Nadi and Abdanan,[Citation57] the duration of drying decreased from 7 to 1 h with the increase of temperature from 40°C to 60°C. The observations are in agreement with the statement that heat is the most destructive factor for anthocyanins and, consequently, long exposure to drying accelerates degradation of these compounds.[Citation13] To minimize thermal destruction of anthocyanins, short-time processes at high temperature are recommended.[Citation58] According to the results, the stability of E. amoenum petal anthocyanins gradually increased with the increase of heating temperature and shorter heating time. The following empirical equation was used to predict the anthocyanin content as a function of the independent variables. The data treatment shows that 77.34% of the anthocyanin content is explained by following equation, with appropriate distribution of residuals:
shows the variation in the anthocyanin content as a function of temperature and air velocity. A strong non-linear behaviour was observed, and the best drying results were achieved at intermediate air velocities.
Energy consumption under different drying conditions
Performance evaluation of an industrial inclined bed dryer is needed to determine the influence of temperature and air flow on the energy consumption and quality of the dried product. The effect of two independent variables on the energy consumption was examined using the coefficients of the second-order polynomial regression equations. A three-dimensional surface plot shows clearly the effect of independent variables (air velocity and drying temperature) on the energy consumption during drying of E. amoenum petals (). The energy consumption decreased with an increase in the drying temperature and air velocity. This behaviour may be caused by excitation of molecules, when molecules are highly excitated at increased temperature, and the greater distance weakens the attractive force between them.[Citation59] A linear term was fitted for predicting the energy consumption (EC) using the following equation:
Figure 2. Response surface plot of the effects of drying conditions (air velocity and temperature) on the energy consumption during drying dried Echium amoenum petals.
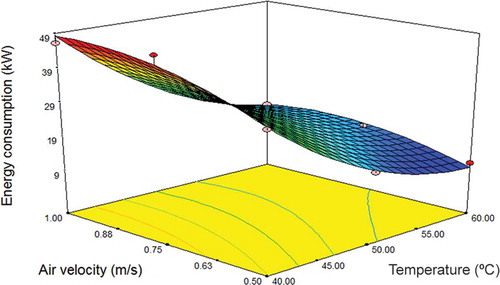
The optimum energy consumption (i.e. the lowest EC value of 1.05 kW/h) was predicted to be obtained at the drying temperature of 57°C and air velocity of 0.5 m/s using response surface plot and response optimizer. These results are in agreement with Aghbashlo[Citation60] for barberry fruit and Amiri Chayjan[Citation61] for potato. Statistical analysis shows that 91.06% of energy consumption variability is explained by Eq. (7), with independent and identical distribution.
Optimization of response
Complex chemical interactions that affect functional properties of food and medicinal plants during drying are still under investigation. Therefore, an optimum combination of drying conditions should be established for each product to minimize the degradation of bioactive compounds during dehydration. In order to obtain dried E. amoenum petals with the minimum energy consumption and maximum total phenolic, flavonoid and anthocyanin content, and antioxidant capacity, the optimal fluidized bed drying conditions were determined based on the combination of both responses (highest retention of bioactive compounds at minimum energy consumption). Multiple graphical and numerical optimizations were carried out to determine an optimum level of independent variables with desirable response results. The following optimal conditions for the responses were obtained: drying temperature of 60°C and air velocity of 0.86 m/s, with a desirability value of 0.91. Using the optimum conditions, the corresponding predicted response values of TPC, TFC, anthocyanin content, antioxidant capacity, and energy consumption were 0.63 mg/g of dry matter, 0.22 mg/g of dry matter, 0.31 µmol/g, 53.70%, and 1.72 kWh, respectively.
Conclusion
Dried E. amoenum petals can be considered as an important source of biologically active compounds with high antioxidant capacity. The stability of the compounds highly depends on drying conditions. Controlled fluidized bed drying conditions (temperature and air velocity) can lead to high-quality medicinal plant product with high phytochemical content and antioxidant capacity. The highest content of bioactive compounds, with minimum energy consumption, was obtained after drying at 60°C and air velocity of 0.86 m/s. The physiochemical changes in the samples during dehydration can significantly affect the physiological activities of this plant. High temperature and intermediate air velocity should be used in order to guarantee the best preservation of bioactive compounds that characterize this plant and to protect its biological activities. The results of this research are essential for the improvement of industrial dehydration of E. amoenum petals in order to maintain their high content of bioactive compounds.
References
- Rechinger, K.H. Flora Iranica; Akademische Druck- Und Verlagsanstalt: Graz, Austria. 1967; 281 pp.
- Mozaffarian, V. Encyclopedia of Iranian Plant Names; Farhang Moaser: Tehran, Iran, 1996; 574 pp.
- Hooper, D. Useful Plants and Drugs of Iran and Iraq; Field Museum of Natural History: Chicago, USA. 1937; 44 pp.
- Zargari, A. Medicinal Plants; Tehran University Publication: Tehran: Tehran, Iran, 1996; 538 pp.
- Amin, Gh.R. Popular Medicinal Plants of Iran. Press; Iranian Research Institute of Medicinal Plants: Tehran, Iran, 1991; 230 pp.
- Shafaghi, B.; Naderi, N.; Tahmasb, L.; Kamalinejad, M. Anxiolytic Effect of Echium Amoenum L. in Mice. Iranian Journal of Pharmaceutical Research 2002, 1, 37–41.
- Amirghofran, Z.; Azadbakht, M.; Keshavarzi, F. Echium Amoenum Stimulate of Lymphocyte Proliferation and Inhibit of Humoral Antibody Synthesis. Iranian Journal of Medical Sciences 2000, 25, 119–124.
- Jambor, J.; Czosnowska, E. Herbal Medicines from Fresh Plants. Postêpy Fitoterapii 2002, 8, 2–5.
- López-Vidaña, E.C.; Figueroa, I.P.; Cortés, F.B.; Rojano, B.A.; Ocañ, A.N. Effect Of Temperature On Antioxidant Capacity During Drying Process Of Mortiño (Vaccinium meridionale Swartz). International Journal of Food Properties 2016, In press.
- Strumillo, C.; Kudra, T. Drying: Principles, Applications and Design; Gordon & Breach Science Pub, New York, USA, 1987; 466 pp.
- Kamiloglu, S.; Capanoglu, E. Polyphenol Content in Figs (Ficus carica L.): Effect of Sun-Drying. International Journal of Food Properties 2015, 18, 521–535.
- Kalt, W.; Mcdonald, J.E.; Donner, H. Anthocyanins, Phenolics, and Antioxidant Capacity of Processed Lowbush Blueberry Products. Journal of Food Science 2000, 65, 390–393.
- Skrede, G.; Wrolstad, R.E.; Durst, R.W. Changes in Anthocyanins and Polyphenolics during Juice Processing of Highbush Blueberries (Vaccinium Corymbosum L.). Journal of Food Science 2000, 65, 357–364.
- Nadi, F. Development of a New Model for Mass Transfer Kinetic of Petals of Echium Amoenum Fisch & Mey under Fluidized Bed Conditions. Food Technology and Biotechnology 2016, 54, 217–227.
- Rufino, M.S.M.; Alves, R.E.; Brito, E.S.; Morais, S.M.; Sampaio, C.G.; Pérez-Jiménez J.; Saura-Calixto, F.D. Metodologia Científica: Determinação Da Atividade Antioxidante Total Emfrutas Pela Captura Do Radical Livre ABTS; Embrapa Agroindústria Tropical: Fortaleza, Brazil, 2007; 1–4 pp.
- Wright, L.P.; Mphangwe, N.I.K.; Nyirenda, H.E.; Apostolides, Z. Analysis of Caffeine and Flavan-3-Ol Composition in the Fresh Leaf of Camellia Sinesis for Predicting the Quality of the Black Tea Produced in Central and Southern Africa. Journal of the Science of Food and Agriculture 2000, 80, 1823–1830.
- Atoui, A.K.; Mansouri, A.; Boskou, G.; Kefalas, P. Tea and Herbal Infusions: Their Antioxidant Activity and Phenolic Profile. Food Chemistry 2005, 89, 27–36.
- Matthäus, B. Antioxidant Activity of Extracts Obtained from Residues of Different Oilseeds. Journal of Agricultural and Food Chemistry 2002, 50, 3444–3452.
- Arvouet-Grand, A.; Vennat, B.; Pourrat, A.; Legret P. Standardization of Propolis Extract and Identification of Principal Constituents. Journal De Pharmacie De Belgique 1994; 49, 462–468.
- Koyuncu, T.; Pinar, Y.; Lule, F. Convective Drying Characteristics of Azarole Red(Crataegus Monogyna Jacq.) and Yellow (Crataegus Aronia Bosc.) Fruits. Journal of Food Engineering 2007, 78, 1471–5.
- Tomaino, A.; Cimino, F.; Zimbalatti, V.; Venuti, V.; Sulfaro, V.; Pasquale, A.; Saija, A. Influence of Heating on Antioxidant Activity and the Chemical Composition of Some Spice Essential Oils. Food Chemistry 2004, 89, 549–554.
- Wang, S.Y.; Lin H.S. Antioxidant Activity in Fruits and Leaves of Blackberry, Raspberry, and Strawberry Varies with Cultivar and Developmental Stage. Journal of Agricultural and Food Chemistry 2000, 48, 140–146.
- Wang, S.Y.; Zheng, W.; Galletta G.J. Cultural System Affects Quality and Antioxidant Capacity in Strawberries. Journal of Agricultural and Food Chemistry 2002, 50, 6534–6542.
- Mori, K.; Goto-Yamamoto, N.; Kitayama, H.K. Loss of Anthocyanins in Redwine Grape under High Temperature. Journal of Experimental Botany 2007, 58, 1935–1945.
- Morales-Delgado, D.Y.; T´Ellez-Medina, D.I.; Rivero-Ram´Irez, N.L.; Arellano-C´Ardenas, S.; L´Opez-Cortez, S.; Hern´Andez-S´Anchez, H.; Guti´Errez-L´Opez G.; Cornejo-Maz´On, M. Effect of Convective Drying on Total Anthocyanin Content, Antioxidant Activity and Cell Morphometric Parameters of Strawberry Parenchymal Tissue (Fragaria X Ananassa Dutch). Revista Mexicana De Ingeniería Química Journal 2014, 13, 179–187.
- Kha, T. C.; Tuyen, C.; Nguyen, M.H.; Roach P.D. Effects of Pre-Treatments and Air Drying Temperature on Colour and Antioxidant Properties of Gac Fruit Powder. International Journal of Food Engineering 2011, 7, 1556–3758.
- Auisakchaiyoung, T.; Rojanakorn, T. Effect of Foam-Mat Drying Conditions on Quality of Dried Gac Fruit (Momordica Cochinchinensis) Aril. International Food Research Journal 2015, 22, 2025–2031.
- Wagner, G.J. Content and vacuole/extra vacuole distribution of neutral sugars free amino acids, and anthocyanins in protoplast. Plant Physiology 1979, 64, 88–93.
- Huber, L.S.; Hoffmann-Ribani, R.; Rodriguez-Amaya, D.B. Quantitative Variation in Brazilian Vegetable Sources of Flavonols and Flavones. Food Chemistry 2009, 113, 1278–1282.
- Parka, Y.S.; Jung, S.T.; Kang, S.G.; Delgado-Licon, E.; Ayala, A.L.M.; Tapia, M.S.; Martín-Belloso, O.; Trakhtenberg, S.; Gorinstein S. Drying of Persimmons (Diospyros Kaki L.) and the Following Changes in the Studied Bioactive Compounds and the Total Radical Scavenging Activities. LWT- Food Science and Technology 2006, 39, 748–755.
- Lim, Y.Y.; Murtijaya, J. Antioxidant Properties of Phyllanthusamarus Extracts as Affected by Different Drying Methods. LWT- Food Science and Technology 2007, 40, 1664–1669.
- Chan, E.W.C.; Lim, Y.Y.; Wong, S.K.; Lim, K.K.; Tan, S.P.; Lianto, F.S.; Yong, M.Y. Effects of Different Drying Methods on the Antioxidant Properties of Leaves and Tea of Ginger Species. Food Chemistry 2009, 113, 166–172.
- Korus, A. Effect of Preliminary Processing, Method of Drying and Storage Temperature on the Level of Antioxidants in Kale (Brassica Oleracea L. Var. Acephala) Leaves. LWT- Food Science and Technology 2011, 44, 1711–1716.
- Miranda, M.; Vega-Galvez, A.; Lopez, J.; Parada, G.; Sanders, M.; Aranda, M.; Uribe, E.; Di Scala, K. Impact of Air-Drying Temperature on Nutritional Properties, Total Phenolic Content and Antioxidant Capacity of Quinoa Seeds (Chenopodium Quinoawilld.). Industrial Crops and Products 2010, 32, 258–263.
- Dewanto, V.; Wu, X.; Adom, K.K.; Liu, R.H. Thermal Processing Enhances the Nutritional Value of Tomatoes by Increasing Total Antioxidant Activity. Journal of Agricultural and Food Chemistry 2002, 50, 3010–3014.
- Gupta, S.; Cox, S.; Abu-Ghannam, N. Effect of Different Drying Temperatures on the Moisture and Phytochemical Constituents of Edible Irish Brown Seaweed. LWT- Food Science and Technology 2011, 44, 1266–1272.
- Hossain, M.B.; Barry-Ryan, C.; Martin-Diana, A.B.; Brunton, N.P. Effect of Drying Method on the Antioxidant Capacity of Six Lamiaceae Herbs. Food Chemistry 2010, 123, 85–91.
- Vega-Gálvez A.; Di Scala, K.; Rodríguez, K.; Lemus-Mondaca, R.; Miranda, M.; López, J.; Perez-Wona, M. Effect of Air-Drying Temperature on Physico-Chemical Properties, Antioxidant Capacity, Colour and Total Phenolic Content of Red Pepper (Capsicum Annuum L. Var. Hungarian). Food Chemistry 2009, 1174, 647–653.
- Sakihama, Y.; Cohen, M.; Grace, S.; Yamasaki, H. Plant Phenolic Antioxidant and Prooxidant Activities: Phenolics-Induced Oxidative Damage Mediated by Metals in Plants. Toxicology 2002, 117, 67–80.
- Haard, N.F.; Chism, G.W. Characteristics of Edible Plant Tissues. In Food Chimistry; Fennema, O.R.; Eds.; Marcel Dekker: New York, USA, 1996; 943–1011.
- Arslan, D.; Musa Özcan, M. Study the Effect of Sun, Oven and Microwave Drying on Quality of Onion Slices. LWT- Food Science and Technology 2010, 43, 1121–1127.
- Garau, M.C.; Simal, S.; Rossello, C.; Femenia, A. Effect of Air-Drying Temperature on Physico-Chemical Properties of Dietary Fibre and Antioxidant Capacity of Orange (Citrus Aurantium V. Canoneta) By- Products. Food Chemistry 2007, 104, 1014–1024.
- Mrad, N.D.; Boudhrioua, N.; Kechaou, N.; Courtois, F.; Bonazzi, C. Influence of Air Drying Temperature on Kinetics, Physicochemical Properties, Total Phenolic Content and Ascorbic Acid of Pears. Food and Bioproducts Processing 2012, 90, 433–441.
- Martín-Cabrejasa, M.A.; Aguileraa, Y.; Pedrosab, M.M.; Cuadradob, C.; Hernándezc, T.; Díazc, S.; Estebana, R.M. The Impact of Dehydration Process on Antinutrients and Protein Digestibility of Some Legume Flours. Food Chemistry 2009, 114, 1063–1068.
- Balasundram, N.; Sundram K.; Samman. S. Phenolic Compounds in Plants and Agri-Industrial by-Products: Antioxidant Activity, Occurrence, and Potential Uses. Food Chemistry 2006, 99, 191–203.
- Celli, G.; Pereira-Netto, A.; Beta, T. Comparative Analysis of Total Phenolic Content, Antioxidant Activity, and Flavonoids Profile of Fruits from Two Varieties of Brazilian Cherry (Eugenia Uniflora L.) throughout the Fruit Developmental Stages. Food Research International 2011, 44, 2442–2451.
- Que, F.; Mao, L.; Fang, X.; Wu, T. Comparison of Hot Air-Drying and Freeze-Drying on the Physicochemical Properties and Antioxidant Activities of Pumpkin (Cucurbitamoschataduch.) Flours. International Journal of Food Science & Technology 2008, 43, 1195–1201.
- Ben Haj Said, L.; Neffati, H.N.M.; Bellagha S. Color, Phenolic and Antioxidant Characteristic Changes of Allium Roseum Leaves during Drying. Journal of Food Quality 2013, 36, 403–410.
- Duzzioni, A.G.; Diogo, V.M.L.; Silva, I.S.; Barrozo, M.A.S. Effect of Drying Kinetics on Main Bioactive Compounds and Antioxidant Activity of Acerola (Malpighia Emarginata D.C.) Residue. International Journal of Food Science & Technology 2013, 48, 1041–1047.
- Michalczyk, M.; Macura, R.; Matuszak I. The Effect of Air-Drying, Freeze-Drying and Storage on the Quality and Antioxidant Activity of Some Selected Berries. Journal of Food Processing and Preservation 2009, 33, 11–21.
- Davey, M.W.; Van-Montagu, M.; Inze, D.; Sanmartin, M.; Kanellis, A.; Smirnoff, N.; Benzie, I.J.J.; Strain, J.; Favell, D.; Fletcher, J. Plant L-Ascorbic Acid: Chemistry, Function, Metabolism, Bioavailability and Effects of Processing. Journal of the Science of Food and Agriculture 2002, 80, 825–860.
- Wang, H. Agriculture Research Service. The Properties of Roselle; United States Department of Agriculture, Maryland, 1998.
- Wiriya, P.; Paiboon, T.; Somchart, S. Effect of Drying Air Temperature and Chemical Pretreatments on Quality of Dried Chilli. International Food Research Journal 2009, 16, 441–454.
- Garba, U.; Kaur, S.; Gurumayum, S.; Rasane, P. Effect of Hot Water Blanching Time and Drying Temperature on the Thin Layer Drying Kinetics of and Anthocyanin Degradation in Black Carrot (Daucus Carota L.) Shreds. Food Technology and Biotechnology 2015, 53, 324–330.
- Katsube, T.; Tsurunaga, Y.; Sugiyama, M.; Furuno, T.; Yamazaki. Y. Effects of Air-Drying Temperature on Antioxidant Capacity and Stability of Polyphenolic Compounds in Mulberry (Morus Alba L.) Leaves. Food Chemistry 2009, 113, 964–969.
- Jackman, R.L.; Smith, J.L. Anthocyanins and Betalains. In Natural Food Colorants; Hendry, G.A.F., Houghton, J.D., Eds.; Blackie Academic & Professional: Glasgow, UK, 1996; 244–309 pp.
- Nadi, F.; Abdanan, S. An Investigation into the Effect of Drying Conditions of Kinetic Drying of Medicinal Plant of Echium Amoenum. International Medical Journal In Press.
- Shahidi, F.; Naczk, M. Phenolics in Food and Nutraceuticals; CRC Press: Florida, US, 1995; 576 pp.
- Jamali, A.; Kouhila, M.; Mohamed, I.; Idliman, A.; Lamharrar, A. Moisture Adsorption-Desorption Isotherms of Citrus Reticulate Leaves at Three Temperatures. Journal of Food Engineering 2006, 77, 71–78.
- Aghbashlo, M.; Kianmehr, M.; Samimi-Akhijahani, H. Influence of Drying Conditions on The Effective Moisture Diffusivity, Energy of Activation and Energy Consumption During the Thin-Layer Drying of Berberis Fruit (Berberidaceae). Energy Conversion and Management 2008, 49, 2865–2871.
- Amiri Chayjan, R. Modeling Some Drying Characteristics of High Moisture Potato Slices in Fixed, Semi Fluidized and Fluidized Bed Conditions. Journal of Agricultural Science and Technology 2012, 14, 1229–1241.