ABSTRACT
The finite element method was employed to model the simultaneous heat and mass transfer in cylindrical samples of banana during convective drying. The Arbitrary Lagrangian–Eulerian (ALE) approach was implemented to incorporate the axial and radial shrinkage effects in the FEM model. The results showed that the FEM model was satisfactorily able to predict the moisture content (R2 > 0.97) and core temperature (R2 > 0.98). Therefore, the proposed model can be used as a promising tool to optimise the drying process of banana from quality and efficiency points of view. Also, the model can be easily extended for drying of similar products with cylindrical shape.
Introduction
Banana due to high moisture content is very perishable and hence after harvesting it is subjected to fast deterioration. To reduce its moisture content and consequently prolong its shelf life, different drying techniques were used. Among them, the hot air drying under forced convection is the most common drying method for drying of different foodstuffs and vegetables (e.g. tomatoes,[Citation1] parsley,[Citation2] and bananas).[Citation3] However, agricultural products with a such high moisture content often crumble irregularly during drying. This phenomenon (stated as shrinkage) is frequently observed for some fruits such as banana, occurred when the moisture gradient within the products induce microstructural stresses to the grid structure of the tissue previously occupied by water which leads to contract and consequent collapse.[Citation4] Shrinkage affects the heat and mass transfer rate during the drying process due to reduction of product surface area[Citation5] and shorten the water diffusion path inside the products.[Citation6–Citation8] It also has some side effects on the final quality of the dried material and, in turn, its marketability.[Citation9] Therefore, shrinkage must be included into the mathematical model for accurate predictions of the product temperature and moisture content during drying.[Citation10,Citation11]
Based on our literature review, the early convective drying kinetics of ripe bananas were studied by empirical models and diffusion models as a whole fruit form,[Citation12–Citation14] and sliced form.[Citation15–Citation17] However, these models had the drawback of ignoring the effect of shrinkage. Besides, although the empirical models are easy to use in engineering applications, their use is restricted to particular process conditions which are used to identify the model parameters.[Citation18] Neglecting the shrinkage effect, a mathematical model was developed for prediction of moisture content and temperature of banana during vacuum and far infrared radiation drying.[Citation19] Also, Karunasena et al.[Citation20,Citation21] studied product shrinkage during drying based on microstructural analysis.
In general, the drying process can be studied by solving two main partial differential equations (PDEs) (Fourier law and Fick’s second law); which express the heat and mass transfer phenomena during drying. Both analytical and numerical approaches can be used to solve these equations depending on problem complexity.[Citation22] These equations are nonlinear and conjugated to each other since the thermophysical properties of the product are dependent on both the temperature and the moisture content. Incorporating the shrinkage in the drying models makes them even more complex as they cannot be solved by analytical methods. There are some studies on drying of bananas[Citation10,Citation23,Citation24] and other fruits[Citation25,Citation26] considering the product shrinkage in the drying model. However, they assumed that the moisture diffusion occurred under isothermal conditions. With this simplification, the heat transfer equation is removable and the solution of only mass transfer equation suffices to describe the drying process.
The finite element method (FEM) as a numerical technique is available to accurately solve complex engineering problems that are not solvable by analytical methods or require costly experiments to arrive at an acceptable solution.[Citation27] By accounting the shrinkage in the heat and mass transfer equations, the resulting systems of coupled nonlinear PDEs must be solved under the moving boundary conditions. This problem can be solved by FEM coupled to the Arbitrary Lagrangian–Eulerian (ALE) procedure. The ALE is developed based on the advantages of both the Lagrangian and the Eulerian approaches in which the nodes of computational mesh may be moved with the continuum in normal Lagrangian manner or be held fixed in the Eulerian fashion or be moved in some arbitrarily specified way to give a continuous rezoning capability.[Citation28] For more information and detailed discussion on the ALE approach, the reader is referred to the works of Taciroglu et al.[Citation29] and Anahid and Khoei.[Citation30]
The ALE was successfully implemented for simulation of the drying process of some shrinkable products. For example, Aprajeeta et al.[Citation31] developed a one-dimensional model to study the behaviour of potato slabs during convective drying. They carried out some experiments for their model validation. Curcio and Aversa[Citation32] formulated a model based on coupling of mass, heat and momentum transfers, and the ALE approach to estimate the product shrinkage during drying. Another numerical study was performed to describe convective drying of prunes.[Citation33] He also considered the prune as a composite of two different materials (flesh and stone) and used the FEM coupled to ALE to account the shrinkage of products in the model. These models, however, were developed by the assumption of one directional shrinkage effect.
In this study, the ALE formulation was implemented to describe the shrinkage of bananas as well as the moisture content and the temperature during drying. The objective of this study was to simulate the convective drying of banana slabs including the effects of both radial and axial shrinkages using a coupled 2D axisymmetric heat conduction and moisture diffusion model with the nonlinear finite element method and ALE approach. Subsequently, the FEM model was verified through comparing its predictions with experimental data under different drying conditions. The outcome of this study can be used for accurate prediction of temperature and moisture content distributions inside bananas under different drying conditions.
Materials and methods
Sample preparation
Ripe bananas (var. Cavendish) were purchased from a local market in Mashhad (Khorasan Razavi Province, Iran). They were hand peeled, and then cut (in the radial orientation) into slabs of 5 mm thickness and approximate diameter of 30 mm, using a slicing machine. About 70 grams of banana (around 20 samples) were used for each experiment. The moisture content of samples before drying was determined by the oven drying method at 105°C for 48 h.[Citation34] The initial moisture content of banana was 79% wet basis.
Drying experiments
Drying experiments were carried out in order to obtain the shrinkage pattern and validation of the FEM model. A convective dryer was used to dry the banana samples from the initial moisture contents of 79% (wet basis) to the equilibrium moisture content in which there was not any net moisture exchange between the samples and the drying air. Then, the moisture ratio (MR) of banana samples was calculated using the following equation:[Citation35]
where MR, Mt, M0, and Me are the moisture ratio, the moisture content at specific time (t), the initial moisture content, and the equilibrium moisture content, respectively. The moisture content values are in dry basis.
A computer controlled dryer were used to perform drying experiments. The details of dryer can be found in the work of Nadian et al..[Citation36] Banana samples were dried in different drying air temperatures i.e. 80, 90, and 100°C. All experimental runs were performed in triplicate.
Moisture and temperature profiles
The moisture content evolution of banana samples during drying was obtained real time by the gravimetrical method using the A&D digital balance with accuracy of 0.01 g. A digital thermometer (TM-947SD, Lutron Electronics Co., Inc.) consists of a data logger with four K-type thermocouples were used to measure temperature changes inside the banana samples (three samples) and also the drying air within the dryer. The thermocouples were placed in the geometric centre of the banana samples. The thermometer was set to save temperature values during drying with 30 s intervals.
Shrinkage measurement
The image processing technique was used to determine area shrinkage of banana samples. The images of samples were taken with a Nikon Coolpix P510 digital camera (Nikon Inc., Japan) at time intervals of 30 s during drying. A program was written in MATLAB software to measure the surface area changes of banana samples. The Eq. (2) was used to calculate the radial shrinkage (Rsh) of banana samples. The axial shrinkage (Ash) was also determined experimentally by measuring the evolution of sample thickness using Eq. (3). The radial and axial shrinkages as a function of moisture ratio were used as the changes in domain boundaries in order to define the moving mesh in the FEM model.
where, At, A0, Zt, and Z0 are the surface area at time t (m2), initial surface area (m2), half of thickness at time t(m), and half of initial thickness (m), respectively.
Governing equations
Drying of banana samples accompanies with different transfer phenomena such as; moisture diffusion from the inner layers of banana to the outer surface, convection heat transfer between food and drying air, conductive heat transfer within the banana, evaporation of diffused water in the interface of samples and medium.[Citation33] If the shrinkage effect is considered, these transfer phenomena may affected with structural changes of banana samples simultaneously. The schematic view of above mentioned phenomena are shown in .
Heat and mass transfer equations
The general form of heat transfer equation for a cylindrical banana sample can be derived from Fourier’s law as follow:
where ρ, Cp, k, r, z, and t are density (kgm−3), specific heat (Jkg−1K−1), thermal conductivity (Wm−1K−1), banana radius (m), banana thickness (m), and drying time (s), respectively. It was assumed that the moisture transfer occurs only by diffusion inside the banana therefore, Fick’s law was used for expression of mass transfer as follow:
where ρs and Deff are the density of the solid product (kgm−3) and the moisture diffusivity (m2s−1), respectively.
Initial and boundary conditions
It was assumed that the temperature and moisture concentrations of banana before drying were uniform and equal to T0 and C0, respectively. Hence, the initial conditions for Eqs. (4) and (5) can be written as:
There are no concentration and temperature gradients at the centreline of banana samples during drying. Hence, the symmetry boundary conditions were applied for Eqs. (4) and (5) as:
A part of the heating energy of the hot air during drying is consumed for evaporation of water at the banana surface while the remaining is conducted into the banana sample to raise its temperature. Therefore, the second boundary conditions for heat transfer equation can be written by heat balance at the air–sample interface as follow:
where n is the unit normal vector pointing outward from the boundaries. The last term in Eq. (8) refers to the heat for water evaporation at the surface, where λ (Jkg−1) is the water latent heat of evaporation, given as a function of temperature by the following equation:[Citation37]
The second boundary conditions relative to the mass transfer equation which applied to the external surfaces are based on the balance between the diffusive water coming from the core of banana and the moisture leaving the product through the airflow:
The effective mass diffusivity (Deff) was obtained from the moisture ratio data, using the slope method.[Citation38] An Arrhenius type function was used to relate this parameter and drying temperature using the following equation:[Citation39,Citation40]
where D0, Ea, Rg, and T are the pre-exponential Arrhenius factor (m2s−1), the activation energy (kJmol−1), universal gas constant (8.314 Jmol−1K−1), and the drying temperature (K), respectively.
Model parameters
The thermophysical properties of banana as a function of moisture content and temperature are needed for accurate solution of heat and mass transfer PDEs. These properties (i.e. density, specific heat, and thermal conductivity) were obtained using the equations based on the properties of product composition (i.e. water, carbohydrate, fibre, fat, protein, and ash). They were obtained experimentally as a function of their temperatures.[Citation37] This method was used by others for several products such as papaya,[Citation41] prune,[Citation33] and barely grain.[Citation42] The summary of this method and the calculated parameters are shown in . The convection heat transfer coefficient ht (Wm−2K−1) was obtained by the correlation considering the process parameters using the following equations:[Citation43,Citation44]
Table 1. Thermophysical properties used for simulation of banana drying.
where ρa, ν, µ, and d are the air density (kgm−3), air velocity (ms−1), air viscosity (kgm−1s−1), and banana sample diameter (m), respectively. The mass transfer coefficient, hm (kgm−2s−1), was determined based on the heat transfer equation according to:[Citation45]
where Cpa, αa, and Dw are the air specific heat (Jkg−1K−1), air thermal diffusivity (m2s−1), and water mass diffusivity in the air (m2s−1), respectively.
Development of the FEM model
The mentioned nonlinear partial differential equations (PDEs) describing coupled heat and mass transfer in convective drying of banana were solved by the finite elements method using a commercial software (COMSOL Multiphysics, version 5.1.). Duo to the axisymmetry of the banana slabs, only one quarter of planer intersection was considered in the simulation (). Therefore, a rectangle with length of 15 mm and width of 2.5 mm was created in COMSOL for numerical solution. In a 2D axisymmetric model (), the coordinate origin represents the centre of banana slab, the boundaries Г2 and Г3 represent the surface of banana, but the boundaries Г1 and Г4 represent the axisymmetric and symmetry lines inside the sample, respectively. The domain was discretised into 678 triangular elements leading to 4419 degrees of freedom. The mesh was finer close to the boundaries Г2 and Г3, which exposed to the drying air. Two modules of COMSOL i.e. Heat Transfer in Solids and Transport of Diluted Species were used to handle the heat and mass transfer problem, respectively. Besides, the Arbitrary Lagrangian–Eulerian (ALE) approach was incorporated in the model using the Moving Mesh module in order to account both radial and axial shrinkages that occurred during drying. The drying simulation was performed with time step of 30 s up to 32000 s.
Validation of the FEM analysis
The mean relative error (E%) between the predicted and experimental values was calculated for validation of the numerical analysis using the following equation:[Citation46,Citation47]
Results and discussion
shows the variations of radial shrinkage (Rsh) and axial shrinkage (Ash) of banana samples versus moisture ratio during drying. It can be found from the figure that banana samples experience different shrinkage in axial and radial directions. Therefore, the assumption of isotropic shrinkage is not anymore valid and hence the models based on this assumption are not trustworthy for predicting the behaviour of banana slabs during the drying process. It was also found that both shrinkages (radial and axial) take place almost in linear pattern for all drying air temperatures. Furthermore, less shrinkage occurs in both radial and axial directions, as the drying temperature increases. This finding is in agreement with the works of others for banana,[Citation23] hawthorn fruit,[Citation48] and pomegranate arils.[Citation49] Reducing the radius and thickness of banana during drying as a function of local moisture content are presented in . The fairly high coefficients of determination show the validity of the linear functions. The linear relation between product shrinkage and moisture content were reported by others for different products such as potato,[Citation31] apple,[Citation50] and carrot.[Citation51] To incorporate shrinkage in the FEM model, the functions presented in were used to define the moving mesh in the FEM model through the ALE approach.
Table 2. Linear relation for the variations of radius (R) and thickness (Z) of banana samples versus moisture content (d.b) during drying.
Figure 3. The radial and the axial shrinkages of banana samples versus the moisture ratio for different drying temperatures.
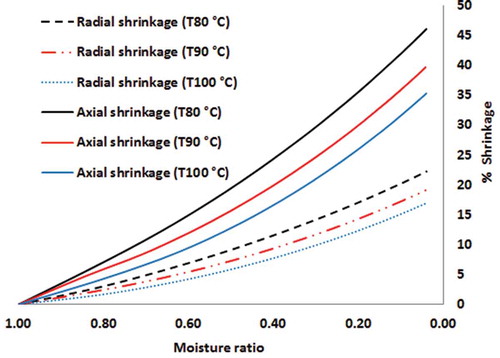
shows the experimental and predicted drying curves of banana with different drying air temperatures. As it can be seen in the figure, the water removal is expedited as the drying temperature increases. This behaviour is due to the strong dependence of the effective moisture diffusivity on temperature. Actually, increasing the difference between medium and product temperature leads to a higher heat flux and in turn increases the effective moisture diffusivity.[Citation52] The figure also demonstrates that the experimental data and the predicted values are in good agreements. The statistical comparisons of these curves were performed by fitting a line between the predicted and experimental moisture ratios in the form of Mrpred = A × Mrexp + B, where A and B are constants (). By plotting the mentioned equations, it could be concluded that the predicted and experimental values are closely around a straight line. However, to avoid prolongation of paper, these graphs were not presented. The suitability of the model for different air temperatures can be confirmed by the high coefficients of determination (R2 > 0.97). Also, the mean relative error (E%) ranged between 5.48–9.86% which is in the acceptable range (E% < 10) according to.[Citation53]
Table 3. The constants A, B and the coefficient of determination (R2) for the linear relations between experimental and predicted moisture ratios by FEM (Mrpred = A × Mrexp+B).
shows the temperature distributions of banana samples at different elapsed times. This figure also exhibits the progress of shrinkages of banana slabs during drying in axial and radial directions. In this figure, the evolution of temperature inside the banana slabs is reported only for the air temperature of 100°C; the other drying temperatures had similar trends. As it can be seen in the figure, the temperature inside the banana varies in both radial and axial directions. An obvious temperature difference between the surface of banana and the drying air temperature is seen, which is relatively high during early stages of drying. This might be due to the evaporation of water from the surface of banana slabs, as it is an endothermic process. In other words, at the early stage of the drying process, the moisture at the surface of banana consumes most of the receiving heat for evaporation and hence less heat conducted to the inner part of sample. As the drying proceeds, more heat transfers to the inside of banana and hence it gradually warms up and gets closer to the drying air temperature. It was found that the temperature gradient inside the banana decreases rather rapidly over the drying time. It can also be concluded that the right side of the domain (Γ3) has higher temperature than its top side (Γ2), while both of them are in touch with the medium. This can be explained by this fact that the width of domain is very smaller than its length. Therefore the temperature distribution in axial direction is more uniform than radial direction. This can also be confirmed with moisture distribution that was shown in .
Figure 5. The temperature distributions inside the banana sample during drying with an air temperature of 100°C at different elapsed times; (a) 0 s, (b) 200 s, (c) 1000 s, (d) 2000 s, (e) 4000 s, (f) 9000 s, (g) 14000 s, (h) 18000 s.
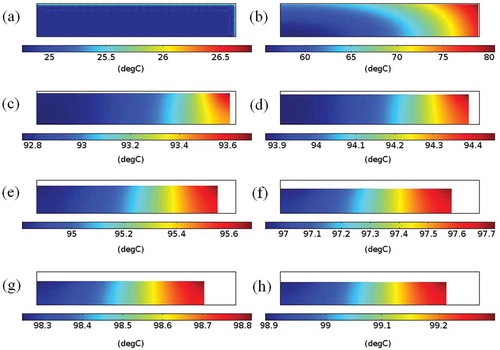
The variation of measured and predicted temperatures in the geometrical centre of banana sample is shown in . A good agreement between the simulated and measured data can be seen in the figure. However, a slight deviation between measured and predicted temperature is seen in the early stages of drying (t < 1000 s) which is shown in . It seems to be caused by assumption of a constant diffusion coefficient that is practically higher in the early stages of the drying process. The statistical comparisons of the measured and predicted temperatures were performed by a similar method for moisture ratio, which is shown in . It is seen that, the FEM could successfully predict the temperature at the centre of banana slabs during drying. The mean relative error (E%) for the temperature estimation was in the range of 1.21–1.96%. As the mean relative error for estimation of the temperature and the moisture ratio are in the acceptable range hence, it can be concluded that introducing the thermo physical properties of biomaterials e.g. banana as function of temperature and moisture content is an encouraging idea for accurate simulation of the drying process.
Table 4. The constants A, B and the coefficient of determination (R2) for the linear relation between experimental and predicted temperature at the centre of banana (Tpred = A×Texp+B).
Figure 6. The variations of predicted and experimental temperatures at the centre of the banana slab for different drying air temperatures; (a) whole drying time, (b) the early stages of drying (t < 5000 s).
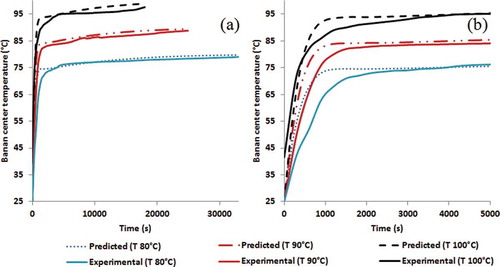
Figure 7. The moisture distributions (w.b) inside the banana sample during drying with the air temperature of 100°C at different elapsed times; (a) 0 s, (b) 2000 s, (c) 4000 s, (d) 6000 s, (e) 9000 s, (f) 12000 s, (g) 15000 s, (h) 18000 s.
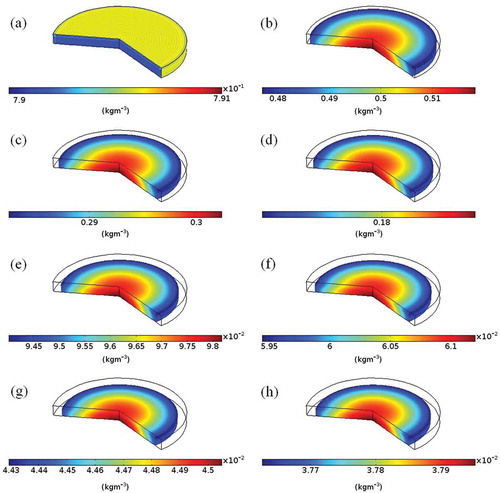
demonstrates the evolution of predicted moisture distribution inside the banana samples during drying. It shows the half top cross section of banana samples in a 3D view. This was obtained by rotation of the calculated domain () around the axisymmetric line. Changes in shrinkage of banana samples are also clear in this figure. As shown in the figure, the moisture content decreases gradually with elapse time, but at each step, there is only a very small amount of moisture at the surface of banana due to the rapid evaporation of surface water. While at the same time, the gradient of moisture concentration makes the migration of internal moisture to the outer surface. However the diffusion of the internal water to the outer surface is much slower due to the effect of internal mass transfer resistance.[Citation54,Citation55] Therefore, it can be concluded that the drying process of banana is controlled by the diffusion and takes place entirely in the falling rate period as shown previously in .
In general, our FEM model could successfully predict the temporal and spatial evolutions of moisture and temperature during drying of banana with various conditions. It is well known that the quality attributes of banana are affected by both temperature and moisture evolutions. Therefore, the proposed model can be a useful tool for design and optimisation of the banana drying process even in industrial scale to achieve dried banana with desired quality. Besides, the proposed model can be easily extend for various agricultural products with cylindrical shape or similar heat and mass transfer process such as cooking, roasting etc.
Conclusion
The FEM were coupled to ALE in order to solve transit heat and mass transfer PDEs describing drying of banana slabs including the shrinkage effect. The model developed and verified in this study enables the prediction of temperature and moisture concentration inside the banana samples under different drying conditions. The predicted data was found to be in good agreement with experimental data. Therefore, this model can be used in order to analyse and optimise the drying process of banana. Also, the developed model can easily be extended for other agricultural products with cylindrical shape.
Acknowledgements
The authors would like to thank Dr. Mahmood Reza Golzarian and Dr. Mohammad Hossein Nadian for technical assistance.
Funding
This work was financially supported by Ferdowsi University of Mashhad (Project No. 38200).
Additional information
Funding
References
- Izli, N.; Isik, E. Color and Microstructure Properties of Tomatoes Dried by Microwave, Convective, and Microwave-Convective Methods. International Journal of Food Properties 2015, 18(2), 241–249.
- Akbudak, N.; Akbudak, B. Effect of Vacuum, Microwave, and Convective Drying on Selected Parsley Quality. International Journal of Food Properties 2013, 16(1), 205–215.
- Nguyen, M.-H.; Price, W.E. Air-Drying of banana: Influence of Experimental Parameters, Slab Thickness, Banana Maturity and Harvesting Season. Journal of Food Engineering 2007, 79(1), 200–207.
- Aguilera, J.M. Drying and Dried Products Under the Microscope. Food Science and Technology International 2003, 9(3), 137–143.
- May, B.; Perre, P. The Importance of Considering Exchange Surface Area Reduction to Exhibit a Constant Drying Flux Period in Foodstuffs. Journal of Food Engineering 2002, 54(4), 271–282.
- Milczarek, R.R.; Dai, A.A.; Otoni, C.G.; McHugh, T.H. Effect of Shrinkage on Isothermal Drying Behavior of 2-Phase Olive Mill Waste. Journal of Food Engineering 2011, 103(4), 434–441.
- Thuwapanichayanan, R.; Prachayawarakorn, S.; Kunwisawa, J.; Soponronnarit, S. Determination of Effective Moisture Diffusivity and Assessment of Quality Attributes of Banana Slices during Drying. LWT-Food Science and Technology 2011, 44(6), 1502–1510.
- Zielinska, M.; Markowski, M. Air Drying Characteristics and Moisture Diffusivity of Carrots. Chemical Engineering and Processing: Process Intensification 2010, 49(2), 212–218.
- Mayor, L.; Sereno, A. Modelling Shrinkage during Convective Drying of Food Materials: A Review. Journal of Food Engineering 2004, 61(3), 373–386.
- De Lima, A.; Queiroz, M.; Nebra, S. Simultaneous Moisture Transport and Shrinkage during Drying of Solids with Ellipsoidal Configuration. Chemical Engineering Journal 2002, 86(1), 85–93.
- Katekawa, M.; Silva, M. A Review of Drying Models Including Shrinkage Effects. Drying Technology 2006, 24(1), 5–20.
- Dandamrongrak, R.; Young, G.; Mason, R. Evaluation of Various Pre-Treatments for the Dehydration of Banana and Selection of Suitable Drying Models. Journal of Food Engineering 2002, 55(2), 139–146.
- Jannot, Y.; Talla, A.; Nganhou, J.; Puiggali, J.-R. Modeling of Banana Convective Drying by the Drying Characteristic Curve (DCC) Method. Drying Technology 2004, 22(8), 1949–1968.
- Queiroz, M.; Nebra, S. Theoretical and Experimental Analysis of the Drying Kinetics of Bananas. Journal of Food Engineering 2001, 47(2), 127–132.
- Boudhrioua, N.; Giampaoli, P.; Bonazzi, C. Changes in Aromatic Components of Banana during Ripening and Air-Drying. LWT-Food Science and Technology 2003, 36(6), 633–642.
- Chua, K.; Mujumdar, A.; Chou, S.; Hawlader, M.; Ho, J. Convective Drying of Banana, Guava and Potato Pieces: Effect of Cyclical Variations of Air Temperature on Drying Kinetics and Color Change. Drying Technology 2000, 18(4–5), 907–936.
- Demirel, D.; Turhan, M. Air-Drying Behavior of Dwarf Cavendish and Gros Michel banana Slices. Journal of Food Engineering 2003, 59(1), 1–11.
- Ah-Hen, K.; Zambra, C.E.; Aguëro, J.E.; Vega-Gálvez, A.; Lemus-Mondaca, R. Moisture Diffusivity Coefficient And Convective Drying Modelling of Murta (Ugni molinae Turcz): Influence of Temperature and Vacuum on Drying Kinetics. Food and Bioprocess Technology 2013, 6(4), 919–930.
- Swasdisevi, T.; Devahastin, S.; Sa-Adchom, P.; Soponronnarit, S. Mathematical Modeling of Combined Far-Infrared and Vacuum Drying Banana Slice. Journal of Food Engineering 2009, 92(1), 100–106.
- Karunasena, H.; Hesami, P.; Senadeera, W.; Gu, Y.; Brown, R.J.; Oloyede, A. Scanning Electron Microscopic Study of Microstructure of Gala Apples during Hot Air Drying. Drying Technology 2014, 32(4), 455–468.
- Karunasena, H.; Senadeera, W.; Brown, R.J.; Gu, Y. A Particle Based Model to Simulate Microscale Morphological Changes of Plant Tissues during Drying. Soft matter 2014, 10(29), 5249–5268.
- Ruiz-Lopez, I.; Garcia-Alvarado, M. Analytical Solution for Food-Drying Kinetics Considering Shrinkage and Variable Diffusivity. Journal of Food Engineering 2007, 79(1), 208–216.
- Prachayawarakorn, S.; Tia, W.; Plyto, N.; Soponronnarit, S. Drying Kinetics and Quality Attributes of Low-Fat Banana Slices Dried at High Temperature. Journal of Food Engineering 2008, 85(4), 509–517.
- Talla, A.; Puiggali, J.-R.; Jomaa, W.; Jannot, Y. Shrinkage and Density Evolution during Drying of Tropical Fruits: Application to Banana. Journal of Food Engineering 2004, 64(1), 103–109.
- Brasiello, A.; Adiletta, G.; Russo, P.; Crescitelli, S.; Albanese, D.; Di Matteo, M. Mathematical Modeling of Eggplant Drying: Shrinkage Effect. Journal of Food Engineering 2013, 114(1), 99–105.
- Hernandez, J.; Pavon, G.; Garcıa, M. Analytical Solution of Mass Transfer Equation Considering Shrinkage for Modeling Food-Drying Kinetics. Journal of Food Engineering 2000, 45(1), 1–10.
- Seyedabadi, E.; Khojastehpour, M.; Sadrnia, H. Predicting Cantaloupe Bruising Using Non-Linear Finite Element Method. International Journal of Food Properties 2015, 18(9), 2015–2025.
- Donea, J.; Huerta, A.; Ponthot, J.; Rodríguez-Ferran, A. Arbitrary Lagrangian–Eulerian Methods. Encyclopedia of Computational Mechanics. In Stein, E.; De Borst, R.; Hughes, T.J.R., eds.; vol. 1. Wiley: Chichester, 2014.
- Taciroglu, E.; Acharya, A.; Namazifard, A.; Parsons, I. Arbitrary Lagrangian–Eulerian Methods for Analysis of Regressing Solid Domains and Interface Tracking. Computers & Structures 2009, 87(5), 355–367.
- Anahid, M.; Khoei, A. Modeling of Moving Boundaries in Large Plasticity Deformations Via an Enriched Arbitrary Lagrangian–Eulerian FE method. Scientia Iranica. Transaction A Journal of Civil Engineering 2010, 17, 141–160.
- Aprajeeta, J.; Gopirajah, R.; Anandharamakrishnan, C. Shrinkage and Porosity Effects on Heat and Mass Transfer during Potato Drying. Journal of Food Engineering 2015, 144, 119–128.
- Curcio, S.; Aversa, M. Influence of Shrinkage on Convective Drying of Fresh Vegetables: A Theoretical Model. Journal of Food Engineering 2014, 123, 36–49.
- Sabarez, H.T. Computational Modelling of the Transport Phenomena Occurring during Convective Drying of Prunes. Journal of Food Engineering 2012, 111(2), 279–288.
- AOAC. Official Methods of Analysis, 15th edn.; Association of Official Analytical Chemists: Arlington, VA, 1990.
- Seyedabadi, E. Drying Kinetics Modelling of Basil in Microwave Dryer. Agricultural Communications 2015, 3(4), 37–44.
- Nadian, M.H.; Abbaspour‐Fard, M.H.; Sadrnia, H.; Golzarian, M.R.; Tabasizadeh, M. Optimal Pretreatment Determination of Kiwifruit Drying Via Online Monitoring. Journal of the Science of Food and Agriculture 2016, 96(14), 4785–4796.
- ASHRAE. ASHRAE Handbook-Fundamentals; American Society of Heating, Refrigiration and Air-Conditioning Engineers Inc.: Atlanta, USA. 2010
- Tzempelikos, D.A.; Vouros, A.P.; Bardakas, A.V.; Filios, A.E.; Margaris, D.P. Case Studies on the Effect of the Air Drying Conditions on the Convective Drying of Quinces. Case Studies in Thermal Engineering 2014, 3, 79–85.
- Da Silva, W.P.; E Silva, C.M.; Gomes, J.P. Drying Description of Cylindrical Pieces of Bananas in Different Temperatures Using Diffusion Models. Journal of Food Engineering 2013, 117(3), 417–424.
- Rodriguez, O.; Eim, V.S.; Simal, S.; Femenia, A.; Rossello, C. Validation of a Difussion Model Using Moisture Profiles Measured by Means of TD-NMR in Apples (Malus domestica). Food and Bioprocess Technology 2013, 6(2), 542–552.
- Lemus-Mondaca, R.A.; Zambra, C.E.; Vega-Gálvez, A.; Moraga, N.O. Coupled 3D Heat and Mass Transfer Model for Numerical Analysis of Drying Process in Papaya Slices. Journal of Food Engineering 2013, 116(1), 109–117.
- Montanuci, F.D.; Perussello, C.A.; De Matos Jorge, L.M.; Jorge, R.M.M. Experimental Analysis and Finite Element Simulation of the Hydration Process of Barley Grains. Journal of Food Engineering 2014, 131, 44–49.
- Earle, R.L. Unit Operations in Food Processing. (Second Edition). Elsevier Ltd, 2013.
- Singh, R.P.; Heldman, D.R. Introduction to Food Engineering, 5th edn.; Boston, USA: Elsevier-Academic Press; 2009.
- Cengel, Y.A. Heat Transfer: A Practical Approach; MacGraw Hill: New York, USA, 2003.
- Carcel, J.; Garcia-Perez, J.; Riera, E.; Mulet, A. Improvement of Convective Drying of Carrot by Applying Power Ultrasound—Influence of Mass Load Density. Drying Technology 2011, 29(2), 174–182.
- Garau, M.; Simal, S.; Femenia, A.; Rosselló, C. Drying of Orange Skin: Drying Kinetics Modelling and Functional Properties. Journal of Food Engineering 2006, 75(2), 288–295.
- Aral, S.; Bese, A.V. Convective Drying of Hawthorn Fruit (Crataegus spp.): Effect of Experimental Parameters on Drying Kinetics, Color, Shrinkage, and Rehydration Capacity. Food Chemistry 2016, 210, 577–584.
- Horuz, E.; Maskan, M. Hot Air and Microwave Drying of Pomegranate (Punica granatum L.). Arils. Journal of Food Science and Technology. 2015, 52(1), 285–293.
- Sturm, B.; Vega, A.-M.N.; Hofacker, W.C. Influence of Process Control Strategies on Drying Kinetics, Colour and Shrinkage of Air Dried Apples. Applied Thermal Engineering 2014, 62(2), 455–460.
- Hatamipour, M.; Mowla, D. Shrinkage of Carrots during Drying in an Inert Medium Fluidized Bed. Journal of Food Engineering 2002, 55(3), 247–252.
- Tzempelikos, D.A.; Mitrakos, D.; Vouros, A.P.; Bardakas, A.V.; Filios, A.E.; Margaris, D.P. Numerical Modeling of heat and Mass Transfer during Convective Drying of Cylindrical Quince Slices. Journal of Food Engineering 2015, 156, 10–21.
- Kaymak-Ertekin, F.; Gedik, A. Kinetic Modelling of Quality Deterioration in Onions during Drying and Storage. Journal of Food Engineering 2005, 68(4), 443–453.
- Ateeque, M.; Mishra, R.K.; Chandramohan, V.; Talukdar, P. Numerical Modeling of Convective Drying of Food with Spatially Dependent Transfer Coefficient in a Turbulent Flow Field. International Journal of Thermal Sciences 2014, 78, 145–157.
- Mohan, V.C.; Talukdar, P. Three Dimensional Numerical Modeling of Simultaneous Heat and Moisture Transfer in a Moist Object Subjected to Convective Drying. International Journal of Heat and Mass Transfer 2010, 53(21), 4638–4650.