ABSTRACT
The application of hesperidin as bioactive compound is limited due to its low water solubility. The effect of natural products derived from black tea (theophylline, theaflavin, and theaflavin-3,3′-digallate) on the solubility of hesperidin was evaluated by liquid chromatography time-of-flight/mass spectrometry and the mechanism was investigated by NMR spectroscopy. As a result, theaflavin-3,3′-gallate was the most effective in improving hesperidin solubility, and had synergistic effect with theaflavin on complexing with hesperidin. The complexation with natural products caused changes in both the chemical shift (0.01–0.29 ppm) and the diffusion coefficient (0.39–1.19) of hesperidin, implying that the proximity of B ring in hesperidin may play an important role in forming a complex with natural products.
Introduction
Hesperidin is a flavanone glycoside (a subclass of flavonoids), which is found abundantly in citrus fruits.[Citation1] It exhibits variable biological properties such as antioxidant, radical scavenging, anti-inflammatory, analgesic, anticancer, and hypolipidemic activities.[Citation2–Citation4] However, hesperidin presents low water solubility and is poorly absorbed in the small intestine, which greatly reduces the drug bioavailability.[Citation5,Citation6] An improvement in the water solubility of hesperidin may enhance its bioavailability and subsequent metabolic stability and tissue distribution, and is becoming an increasingly important area of food research.[Citation7] Previous study found that the water solubility of hesperidin was improved after transglycosylating hesperidin to glycosyl hesperidin by cyclodextrin (CD) glucanotransferase.[Citation8] The inclusion formation of a β-CD and hesperidin complex could also enhance the water solubility of hesperidin.[Citation9] Besides, a fermented tea containing hesperidin, narirutin, catechins, and their oxidative products has also been reported to increase the water solubility of hesperidin, and the tea extract fractions including (−)-epigallocatechin-3-O-gallate (EGCG), theophylline (THP), theaflavins, and theasinensins showed the best solubilization effect on hesperidin (sixfold).[Citation10] The mechanism of solubilization of hesperidin by tea polyphenol theasinensin A (TSA) has successfully been demonstrated in our previous study; that hesperidin complexed with TSA and formed a stable 2:1 Hesp-TSA complex in water.[Citation11] It is thus presumed that hydrophilic natural products from fermented tea could interact with hesperidin and improve its water solubility.
Various natural products from tea are believed to exert protective effects against diseases and have been subjected to extensive investigation.[Citation12–Citation15] THP occurs naturally in tea and is clinical efficient in the treatment of asthma and chronic obstructive pulmonary disease. It also poses anti-inflammatory effects and has the potential to reverse corticosteroid resistance at lower doses.[Citation16,Citation17] Black tea is rich in theaflavins which have been shown to exhibit anti-inflammatory, cholesterol-lowering, and antiviral abilities.[Citation18,Citation19] Taking into account the wide range of beneficial health effects induced by natural products from tea, synergistic health advantages can be expected while these compounds are selected to interact with hesperidin in order to improve its solubility.
To determine the relevance between the water solubility of hesperidin and its interactions with natural products from tea, NMR spectroscopy is an efficient way to provide a comprehensive insight into the molecular structure of the complex.[Citation20–Citation23] NMR techniques including Citation1H NMR, DOSY (diffusion-ordered NMR spectroscopy), and ROESY (rotating frame nuclear overhauser effect spectroscopy) have been reported to characterize the inclusion complex of theaflavin-3,3′-digallate (TF33′G) and CDs (α-, β-, and γ-CD), and showed that the galloyl moiety of TF33′G was present in the β-CD cavity.[Citation24]
With limited studies to date, further studies are required to analyse natural products from tea which can contribute to the water solubility of hesperidin. Thus the aim of this study was to evaluate the solubility of hesperidin mixing with natural products from tea including THP, theaflavin (TF), and TF33′G using liquid chromatography time-of-flight/mass spectrometry (LC-TOF/MS), and to investigate the intermolecular interactions between hesperidin and these natural products related to solubility improvement by NMR techniques.
Materials and methods
Materials
Hesperidin (≥97.0%, C28H34O15, MW 610.56, chemical structure in ), THP, TF, and TF33′G were purchased from Sigma (St. Louis, MO, USA). 3-Trimethylsilyl-1-propanesulfonic acid-d6 (DSS-d6, 98.0 atom% D) was obtained from Santa Cruz Biotechnology Inc. (Texas, USA). Deuterium oxide (D2O, 99.8 atom% D) and dimethyl sulfoxide-d6 (DMSO-d6, 99.9 atom% D) were acquired from Acros Organics (Fair Lawn, USA). Other reagents were of analytical grade and were used without further purification.
LC-TOF/MS measurement
To estimate the solubility changing of hesperidin, free hesperidin, hesperidin mixed with THP, TF, and TF33′G (Hesp-THP, Hesp-TF, Hesp-TF33′G) was dissolved in 10% DMSO at molar ratios of 1:1, 1:5, and 1:10, respectively. After incubation at 37°C for 24 h, the mixture was centrifuged at 10,000g at 20°C for 10 min. The supernatant was then 100-fold diluted by 10% DMSO. Sample of 20 μL were injected into the LC-TOF/MS system (An Agilent 1200 series HPLC (Agilent Technologies, Waldbronn, Germany)) with a micro degasser (G1379B), binary pump (G1312A), high performance autosampler SL (G1367B), and a thermostatically controlled oven apartment (G1316A) was used for chromatography. Separations were achieved on an Agilent TC-C18 column (4.6 mm × 150 mm) at 40°C. The mobile phase consisted of 0.1% aqueous formic acid (A) and methanol with 0.1% formic acid (B) using a linear gradient from 0% to 100% of solvent B with a flow rate of 0.2 mL/min. MS analysis was performed on a micro TOF-II mass spectrometer (Bruker Daltonics, Bremen, Germany). Electrospray ionization was applied and ionization was performed in the negative mode. Mass spectral data were collected within the range of 100–1000 m/z. Ionization conditions were as follows: nebulizer pressure, 1.6 bar; dry gas flow (nitrogen), 8.0 L/min; drying temperature, 200°C; and capillary voltage, 3800 V. Hesperidin was identified at m/z 609.2 using the above conditions.
1H NMR measurement
In order to investigate the mechanism of solubility improvement of hesperidin, free hesperidin and Hesp-THP, Hesp-TF, and Hesp-TF33′G at molar ratios 1:1, 1:5, and 1:10 and Hesp-THP-TF, Hesp-THP-TF33′G, and Hesp-TF-TF33′G at molar ratios 1:1:1, 1:5:5, and 1:10:10, respectively, in 10% DMSO-d6 were prepared. After incubation at 37°C for 1 h, samples volumes of 600 μL were placed in 5 mm NMR sample tubes and measured. All measurements were carried out on a Bruker 400 MHz spectrometer composed of fully digitized circuitry operating at 25°C. Citation1H NMR spectra were acquired by a single pulse sequence at the following conditions: acquisition time 2.18 s, X offset 4.7 ppm, acquisition point 16384, scans 16, relaxation delay 15 s. The 90° pulse width of hesperidin was determined using single pulse sequence under conditions described above at the pulse-widths setting from 41.0 to 44.0 μs linearly, with 0.1 μs intervals. The spin-lattice relaxation delay (T1) value was measured of 1 mM hesperidin in 10% DMSO-d6 by an arrayed experiment using an inversion-recovery pulse sequence[Citation25] with a relaxation delay of 30 s. All spectra were referenced to the signal of DSS-d6 at 0.0 ppm.
DOSY measurement
DOSY NMR measurements[Citation22] using a spin-echo method (pulse sequence beginning with a 90° pulse, after a time interval and followed by a 180° pulse) were performed by a diffusion time of 0.1 s, field gradient pulse width of 1.0 × 10−Citation3 s at 256 scans of 16,384 acquisition points, 90° pulse width of 10.5 μs, and a relaxation delay of 30 s at 25°C. Through changing the listed field gradient values and checking the resulting signal decay of hesperidin, the appropriate pulse field gradient was optimized: array type, logarithmic; range, 1.0 × 10−Citation4–5.0 × 10−Citation1 T/m; points, 16; base, 2. The D value was calculated according to Eq. (1)[Citation26]:
in which γ is the gyromagnetic ratio in the observation of nuclei; G is the pulse-field gradient strength; δ is the pulse width of the field gradient; Δ is the diffusion time, and I is the signal intensity. DSS-d6 was also used as an internal standard (IS) for D value. The D values of hesperidin were all normalized to that of the IS.
Statistics
The LC-TOF/MS assays were carried out in triplicate and data were expressed as the mean ± standard deviation (SD). Statistical differences among different groups at each mole ratio were analysed by one-way analysis of variance (ANOVA), followed by post hoc Tukey-Kramer analysis. A p value of <0.05 was considered to be the statistically significant difference. All statistical analyses were performed using Stat View J 5.0 (SAS Institute, Cary, NC, USA).
Results and discussion
LC-TOF/MS analysis of the solubility of hesperidin
The effects of natural products from tea including THP, TF, and TF33′G on the solubility of hesperidin were estimated by LC-TOF/MS. shows the chemical structures of hesperidin and the natural products from tea used in this study. The 10% DMSO was used as the solvent for the LC-TOF/MS study. As shown in our previous study, the changing trend of solubility for hesperidin mixing with tea polyphenols at molar ratios of 1:1, 1:5, and 1:10 in 10% DMSO was in accordance with that in water. As a result, the solvent environment of 10% DMSO (dielectric constant ε: 46.8) is illustrated to be comparable to that of water (ε: 80) and is appropriate to be used as the solvent in this study.[Citation11,Citation27] shows the LC-TOF/MS chromatogram of hesperidin in 10% DMSO with retention time 24.4 min, and peak areas of hesperidin were used to calculate the solubility. shows the solubility of free hesperidin and Hesp-THP, Hesp-TF, and Hesp-TF33′G at molar ratios 1:1, 1:5, and 1:10, respectively, in 10% DMSO. As proven, the natural products in black tea could enhance the solubility of hesperidin. With the increasing TF33′G concentrations, the solubility of hesperidin was remarkably increased and reached 2.5-fold at Hesp-TF33′G with a mole ratio of 1:10, significantly higher than those of the others (p < 0.05), while it was 1.9-fold for Hesp-TF, and 1.6-fold for Hesp-THP at a molar ratio of 1:10. The solubility improvement of hesperidin mixing with different natural products indicated the following trend upon potential complex formation: Hesp-TF33′G > Hesp-TF > Hesp-THP. It has been reported that amongst galloyl esters, there is a dependence for strong binding on the free galloyl ester group content.[Citation28] Since TF33′G with a galloyl moiety () is highly hydrophilic, it is proposed that this molecular structure may account for the relative higher potential interaction with hesperidin than the others. However, in consequence of the fast crystallization tendency of hesperidin, some hesperidin interacting with natural products was unstable and could reform self-crystallization out of solution again.
1H NMR analysis of hesperidin complex
To achieve an adequate structure-specific understanding of the complex formation between hesperidin and natural products from tea, it is very helpful to use NMR techniques for molecular level resolution. Formation of the complex can initially attribute to the chemical shift changings (Δδ). For all of the NMR experiments, 10% DMSO-d6 was used as the solvent since it has been shown that the information on the complex formation of natural products in 10% DMSO-d6 is consistent with that in D2O by NMR experiments.[Citation11] shows the Citation1H NMR spectra of hesperidin with target peaks assigned according to the reported data.[Citation29] shows the ∆δ for protons in Hesp-THP, Hesp-TF, and Hesp-TF33′G at molar ratios of 1:1, 1:5, and 1:10 and Hesp-THP-TF, Hesp-THP-TF33′G, and Hesp-TF-TF33′G at molar ratios of 1:1:1, 1:5:5, and 1:10:10, respectively, in 10% DMSO-d6 at 25°C. As shown in the table, with an increased concentration of natural products, increasing up-field chemical shifts are clearly shown for protons H2′,5′,6′, H6, H8, and H2 in hesperidin with the following order: H2 (0.01–0.29 ppm) > H2′,5′,6′ (0.01–0.11 ppm) > H8 (0.01–0.08 ppm) > H6 (0.01–0.06 ppm), suggesting that intermolecular interaction between the aglycone moiety of hesperidin and natural products from tea might have occurred. Induced Δδ was not observed for the other signals of hesperidin. For the B ring (H2′,5′,6′) in hesperidin, Δδ of Hesp-TF-TF33ʹG at a mole ratio of 1:10:10 shows the highest value (0.11 ppm), followed by that of Hesp-TF33ʹG (0.09 ppm), and Hesp-THP-TF33ʹG (0.07 ppm). For both A (H6, H8) and C (H2) rings in hesperidin, Hesp-TF-TF33ʹG affected Δδ the most, indicating that TF and TF33ʹG had a synergistic effect on complexing with hesperidin. The gradual splitting of the signals from H6 and H8 suggested a binding angle of the A ring in hesperidin with natural products since the shielding effect of aromatic rings increases with a smaller distance.[Citation30] The outstanding Δδ of H2 might be caused by a direct strong interaction, or by the reshaped C ring resulting from the interacting behaviours of the A and B rings in hesperidin. The Citation1H NMR results illustrated that protons belonging to the aromatic A, B rings and the heterocyclic C ring in hesperidin, whose hydrophobic property mainly accounts for the poor solubility of hesperidin, participated in the interaction. The strong up-field shift for H2′,5′,6′ and the smaller up-field shift for H8 and H6 indicated that B ring in hesperidin was in closer proximity to the natural products than A ring. The similar Δδ of H2′,5′,6′, H8, and H6 in hesperidin were also reported for hesperidin interacting with β-CD.[Citation31] Since the galloyl ester in gallate-type catechins is the preferred site for complex formation,[Citation28] it is presumed that pi–pi interactions and/or hydrophobic effects between the B ring in hesperidin and galloyl moiety in TF33′G may play an important role in stabilizing the complex. As previously reported, the heterocyclic moiety of nicotinamide improved the solubility of an insoluble drug, diazepam, by promoting stacking interactions; a similar enhancement for improved drug solubility was observed owing to pi–pi interactions.[Citation32] The Δδ of protons of the A, B rings in hesperidin were thus suggested as a signature with which aromatic units from both hesperidin and natural products come into close proximity, resulting in improved solubility of hesperidin.
Table 1. Citation1H NMR chemical shift changes (∆δ, ppm) of signals of hesperidin complexed with theophylline (Hesp-THP), theaflavin (Hesp-TF), and theaflavin-3-3ʹ-digallate (Hesp-TF33ʹG) at molar ratios 1:1, 1:5, and 1:10 and their mixture Hesp-THP-TF, Hesp-THP-TF33ʹG, and Hesp-TF-TF33ʹG at molar ratios 1:1:1, 1:5:5, and 1:10:10, respectively, in 10% DMSO-d6 at 25°C. The ∆δ was obtained by the δ of signals of free hesperidin minus that of hesperidin complex.
Figure 4 Citation1H NMR spectra (δ, 2.5–7.5 ppm) of hesperidin in 10% DMSO-d6 at 25°C. Target protons in hesperidin are H2′,5′,6′, H6, H8, H2.
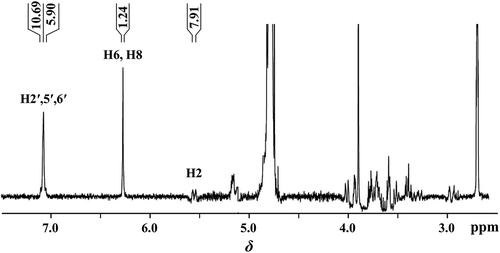
DOSY analysis of hesperidin complex
The determination of D values by DOSY NMR analysis provides binding evidence between differently sized species and has been used in the study of complex phenomenon in solution.[Citation22,Citation33,Citation34] In this experiment, the D value of hesperidin is independent of viscosity by using DSS-d6 as IS[Citation35] and the D values of hesperidin were calculated from the resonance signals of H2′,5′,6′ (7.06 ppm) normalized to that of DSS-d6 (0.00 ppm). The D values of hesperidin complexed with the natural products at molar ratios 1:1, 1:5, and 1:10 (or 1:1:1; 1:5:5; 1:10:10) in 10% DMSO-d6 are presented in . The data show that in the presence of natural products, the D value of hesperidin decreases (ΔD: 0.39–1.19) as a reflection of the increase in its hydrodynamic radii in the gradient magnetic field. The ΔD of Hesp-TF-TF33ʹG shows the highest value, indicating the synergistic effect of TF and TF33ʹG on complexing with hesperidin, in accordance with results of the Citation1H-NMR. The D value variations undergone by hesperidin indicate an increase in its effective size due to intermolecular activities, further confirming the complex formation between hesperidin and natural products.
Figure 5 Diffusion coefficient changes (ΔD) of hesperidin complexed with theophylline (Hesp-THP), theaflavin (Hesp-TF), and theaflavin-3-3ʹ-digallate (Hesp-TF33ʹG) at molar ratios 1:1; 1:5, and 1:10 and their mixtures Hesp-THP-TF, Hesp-THP-TF33’G, and Hesp-TF-TF33ʹG at molar ratios 1:1:1, 1:5:5, and 1:10:10, respectively, in 10% DMSO-d6. The D values were all normalized to that of DSS-d6. The ∆D was obtained by the D value of free hesperidin minus that of hesperidin complex.
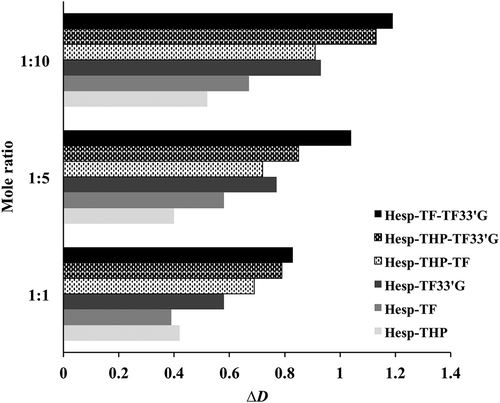
The NMR analysis suggests that the nonpolar regions of natural products paired against the hydrophobic faces of hesperidin can displace the unfavourably structured water molecules over hesperidin, which minimizes the exposition of the aromatic rings in hesperidin to water hence contributing to its increased solubility. The complex of hesperidin and the natural products was sustained through pi–pi interactions and/or hydrophobic effects[Citation36]. As hesperidin is known to convert to its aglycone hesperetin in small intestine and hesperetin has the same aromatic rings, similar strategy could be applied to the enhancement of the solubility of hesperetin. However, to determine a more detailed picture of the geometry of the complex, further ROESY and computational analyses of the hesperidin complex are needed.
Conclusion
This study illustrates structural information on the intermolecular interactions obtained from NMR spectroscopy, elucidating the mechanism of improved solubility of hesperidin through complexing with natural products from tea in terms of pi–pi interactions and/or hydrophobic effects. The use of this general strategy will contribute to the development of new complexes from functional natural food materials for water-insoluble food applications with synergistic combinations of biological properties. Furthermore, enhanced bioavailability of hesperidin through interacting with natural products could be expected and designed based on this work.
Funding
This study was financially supported by the Chinese National Natural Science Foundation (31601477), Tianjin Research Program of Application Foundation and Advanced Technology (15JCYBJC55200), Starting Foundation for Talent Introduction supported by Tianjin University of Science and Technology (1185-10263), Agricultural science and Technology Achievements Transformation Fund (2014GB2A100527), and China-European research collaboration program (SQ2013ZOA100001).
Additional information
Funding
References
- Garg, A.; Garg, S.; Zaneveld, L.J.D.; Singla, A.K. Chemistry and Pharmacology of the Citrus Bioflavonoid Hesperidin. Phytotherapy Research 2001, 15, 655–669.
- Ho, S.; Su, M.; Lin, C. Comparison of Peroxynitrite-Scavenging Capacities of Several Citrus Fruit Peels. International Journal of Food Properties 2014, 17, 111–124.
- Galati, E.M.; Monforte, M.T.; Kirjavainen, S.; Forestieri, A.M.; Trovato, A.; Tripodo, M.M. Biological Effects of Hesperidin, a Citrus Flavonoid. (Note I): Antiinflammatory and Analgesic Activity. Farmaco 1994, 40, 709–712.
- Roohbakhsh, A.; Parhiz, H.; Soltani, F.; Iranshahi, R.; Iranshahi, M. Molecular Mechanisms behind the Biological Effects of Hesperidin and Hesperetin for the Prevention of Cancer and Cardiovascular Diseases. Life Sciences 2015, 124, 64–74.
- Pinho-Ribeiro, F.A.; Hohmann, M.S.N.; Borghi, S.M.; Zarpelon, A.C.; Guazelli, C.F.S.; Manchope, M.F.; Casagrande, R.; Waldiceu, A.V.J. Protective Effects of the Flavonoid Hesperidin Methyl Chalcone in Inflammation and Pain in Mice: Role of TRPV1, Oxidative Stress, Cytokines and NF-κB. Chemico-Biological Interactions 2015, 228, 88–99.
- Vallejo, F.; Larrosa, M.; Escudero, E.; Zafrilla, M.P.; Cerda, B.; Boza, J.; et al. Concentration and Solubility of Flavanones in Orange Beverages Affect Their Bioavailability in Humans. Journal of Agricultural and Food Chemistry 2010, 58, 6516–6524.
- Walle, T. Methylation of Dietary Flavones Greatly Improves Their Hepatic Metabolic Stability and Intestinal Absorption. Molecular Pharmacology 2007, 4, 826–832.
- Kometani, T.; Terada, Y.; Nishimura, T.; Takii, H.; Okada, S. Transglycosylation to Hesperidin by Cyclodextrin Glucanotransferase from an Alkalophilic Bacillus Species in Alkaline pH and Properties of Hesperidin Glycosides. Bioscience, Biotechnology, and Biochemistry 1994, 58, 1990–1994.
- Majumdar, S.; Srirangam, R. Solubility, Stability, Physicochemical Characteristics and in Vitro Ocular Tissue Permeability of Hesperidin: A Natural Bioflavonoid. Pharmaceutical Research 2009, 26, 1217–1225.
- Nakayama, H.; Tanaka, T.; Miyata, Y.; Saito, Y.; Matsui, T.; Aramaki, S.; et al. Development of Soluble Hesperidin-Containing Fermented Tea Made from Unripe Mandarin Orange Fruits and Third Crop Green Tea Leaves. Journal of Japan Society of Nutrition and Food Sciences 2014, 67, 95−103.
- Cao, R.; Kobayashi, Y.; Nonaka, A.; Miyata, Y.; Tanaka, K.; Tanaka, T.; Matsui, T. NMR Spectroscopic and Quantum Mechanical Analyses of Enhanced Solubilization of Hesperidin by Theasinensin A. Pharmaceutical Research 2015, 32, 2301–2309.
- Afaq, F.; Adhami, V.M.; Ahmad, N.; Mukhtar, H. Health Benefits of Tea Consumption. Tropical Journal of Pharmaceutical Research 2007, 6, 785–792.
- Yang, C.S.; Chen, G.; Wu, Q. Recent Scientific Studies of a Traditional Chinese Medicine, Tea, on Prevention of Chronic Diseases. Journal of Traditional and Complementary Medicine 2014, 4:17–23.
- Kobayashi, M.; Kawano, T.; Ukawa, Y.; Sagesaka, Y.M.; Fukuhara, I. Green Tea Beverages Enriched with Catechins with a Galloyl Moiety Reduce Body Fat in Moderately Obese Adults: A Randomized Double-Blind Placebo-Controlled Trial. Food & Function 2016, 7, 498–507.
- Fujiki, H.; Imai, K.; Nakachi, K.; Sueoka, E.; Watanabe, T.; Suganuma, M. Innovative Strategy of Cancer Treatment with the Combination of Green Tea Catechins and Anticancer Compounds. Cancer Cell & Microenvironment 2015, 2, e886.
- Barnes, P.J. Theophylline. American Journal of Respiratory and Critical Care Medicine 2013, 188, 901–906.
- Kanehara, M.; Yokoyama, A.; Tomoda, Y.; Shiota, N.; Iwamoto, H.; Ishikawa, N.; Taooka, Y.; Haruta, Y.; Hattori, N.; Kohno, N. Anti-Inflammatory Effects and Clinical Efficacy of Theophylline and Tulobuterol in Mild-to-Moderate Chronic Obstructive Pulmonary Disease. Pulmonary Pharmacology and Therapeutics 2008, 21, 874–878.
- Zu, M.; Yang, F.; Zhou, W.; Liu, A.; Du, G.; Zheng, L. In Vitro Anti-Influenza Virus and Anti-Inflammatory Activities of Theaflavin Derivatives. Antiviral Research 2012, 94, 217–224.
- de Oliveira, A.; Prince, D.; Lo, C.Y.; Lee, L.H.; Chu, T.C. Antiviral Activity of Theaflavin Digallate against Herpes Simplex Virus Type 1. Antiviral Research 2015, 118, 56–67.
- Teng. Q. Structural Biology: Practical NMR Applications; Springer: New York, 2005.
- Zhang, J.; Tozuka, Y.; Uchiyama, H.; Higashi, K.; Moribe, K.; Takeuchi, H.; Yamamoto, K. NMR Investigation of a Novel Excipient, α-Glucosylhesperidin, as a Suitable Solubilizing Agent for Poorly Water-Soluble Drugs. Journal of Pharmaceutical Sciences 2011, 100, 4421–4431.
- Johnson, J.C.S. Diffusion Ordered Nuclear Magnetic Resonance Spectroscopy: Principles and Applications. Progress in Nuclear Magnetic Resonance Spectroscopy 1999, 34, 203–256.
- Brand, T.; Cabrita, E.J.; Berger, S. Intermolecular Interaction as Investigated by NOE and Diffusion Studies. Progress in Nuclear Magnetic Resonance Spectroscopy 2005, 46, 159–196.
- Nishizawa, M.; Hosoya, T.; Hirokawa, T.; Shin-ya, K.; Kumazawa, S. NMR Spectroscopic Characterization of Inclusion Complexes of Theaflavin Digallate and Cyclodextrins. Food Science and Technology Research 2014, 20, 663–670.
- Caligiani, A.; Acquotti, D.; Palla, G.; Bocchi, V. Identification and Quantification of the Main Organic Components of Vinegars by High Resolution 1H NMR Spectroscopy. Analytica Chimica Acta 2007, 585, 110−119.
- Lucas, L.H.; Otto, W.H.; Larive, C.K. The 2D-J-DOSY Experiment: Resolving Diffusion Coefficients in Mixtures. Journal of Magnetic Resonance 2002, 156:138−145.
- Divakar, S.; Agric, J. Structure of a Beta-Cyclodextrin-Vanillin Inclusion Complex. Food Chemistry 1990, 38, 940−944.
- Cai, Y.; Gaffney, S.H.; Lilley, T.H.; Magnolato, D.; Martin, R.; Spencer, C.M.; et al. Polyphenol Interactions. Part 4. Model Studies with Caffeine and Cyclodextrins. Journal of the Chemical Society, Perkin Transactions 1990, 2, 2197−2209.
- Maltese, F.; Erkelens, C.; der. Kooy, F.V.; Choi, Y.H.; Verpoorte, R. Identification of Natural Epimeric Flavanone Glycosides by NMR Spectroscopy. Food Chemistry 2009, 116, 575–579.
- Baranac-Stojanović, M.; Koch, A.; Kleinpeter, E. Is the Conventional Interpretation of the Anisotropic Effects of C=C Double Bonds and Aromatic Rings in NMR Spectra in Terms of the π-Electron Shielding/Deshielding Contributions Correct? Chemistry - A European Journal 2012, 18, 370−376.
- Ficarra, R.; Tommasini, S.; Raneri, D.; Calabro, M.L.; Di Bella, M.R.; Rustichelli, C.; et al. Study of Flavonoids/β-Cyclodextrins Inclusion Complexes by NMR, FT-IR, DSC, X-ray Investigation. Journal of Pharmaceutical and Biomedical Analysis 2002, 29, 1005–1014.
- Rasool, A.A.; Hussain, A.A.; Dittert, L.W. Solubility Enhancement of Some Water-Insoluble Drugs in the Presence of Nicotinamide and Related Compounds. Journal of Pharmaceutical Sciences 1991, 80, 387–393.
- Cao, R.; Nonaka, A.; Komura, F.; Matsui, T. Application of Diffusion Ordered-1H-Nuclear Magnetic Resonance Spectroscopy to Quantify Sucrose in Beverages. Food Chemistry 2015, 171, 8–12.
- Cao, R,; Komura, F.; Nonaka, A.; Kato, T.; Fukumashi, J.; Matsui, T. Quantitative Analysis of D-(+)-Glucose in Fruit Juices Using Diffusion Ordered-1H Nuclear Magnetic Resonance Spectroscopy. Analytical Sciences 2014, 30, 383–388.
- Cabrita, E.J.; Berger, S. DOSY Studies of Hydrogen Bond Association: Tetramethylsilane as a Reference Compound for Diffusion Studies. Magnetic Resonance in Chemistry 2001, 39, S142–148.
- Sherrill, C.D. Energy Component Analysis of π Interactions. Accounts of Chemical Research 2013, 46, 1020–1028.