Abstract
The structural properties of blends of waxy corn starch and potato starch at different proportions were evaluated at 20% solids content. The results from differential scanning calorimetry, wide-angle X-ray scattering, and small-angle X-ray scattering of the starch blends indicated that each starch gelatinized completely and independently. The setback viscosity values were higher in the blends compared with native starches, which suggested that the granular interactions had an important effect. The presence of waxy corn starch granules could have reinforced the network formed by amylose leached from potato starch granules. The intrinsic characteristics of waxy corn starch and potato starch determined the type of granular and molecular interactions that could be carried out during the processes of gelatinization and gel formation in starch blends.
Introduction
Native starches are not usually suitable for food applications due to their physicochemical and functional properties. Therefore, they are frequently modified using physical, chemical, and/or enzymatic methods to develop the desired properties.[Citation1,Citation2] An alternative to modifying starches is to mix different native starches to prepare blends with improved properties[Citation3–Citation6] through granular and molecular interactions that allow ingredients to be obtained that can be used as gelling agents, stabilizers, and thickeners, among other functions.
However, the granular or molecular interactions that take place when blending native starches can have non-additive effects when the properties (e.g., swelling, gelatinization, gelation, and retrogradation) of the starches in the blend are influenced by each other. On the other hand, when an additive effect takes place, the resulting properties of the blend can be predicted by the sum of the contribution of each starch.[Citation3,Citation6,Citation7]
With regard to thermal properties, it has been established[Citation6] that a difference of approximately 6°C in the gelatinization temperature of two starches at equal concentrations in a blend generates two endothermic peaks, indicating an additive effect and that each starch gelatinizes independently. However, quantifying the contribution of each starch to the endothermic signal is not an easy task. Also, the correlation with other techniques (i.e., X-ray diffraction) and effects of the disorganization achieved during gelatinization on the rheological properties is not clear.
According to previous studies, the starch gelatinization process is affected by the water content[Citation8,Citation9] due to the level of disorganization within the granules that can occur, and therefore, the swelling, solubilization, viscosity, and gel formation properties are impacted.[Citation8] For starches, a minimum water content of 66% is needed to be considered water-excess conditions.[Citation6,Citation10]
Fonseca-Florido et al.[Citation3] reported that the gelatinization properties of achira and amaranth starch blends depended on the water content (excess or limited), proportions of the blend, and intrinsic properties of each starch, including the granule size, amylose content, and crystalline pattern. Waterschoot et al.[Citation11] indicated that the pasting of starch blends not only depended on the individual starches and blend composition but also on the total starch concentration, so the water content plays an important role in the interactions between starches and changes in the thermal, structural, and rheological properties.
In studies of the pasting and gel conformation properties of starches, some authors have reported that the type of interactions between amylose and/or amylopectin of different starches depends on the botanical source because differences in swelling power result in more rigid granule remnants after the heating phase.[Citation7,Citation12–Citation14] This behavior affects the rheological properties of starches. Therefore, it is important to establish a relationship between the gelatinization process and rheological properties of starch blends.
The aim of this study was to evaluate the thermal, rheological, phase transitions, and crystalline properties of potato starch (PS) and waxy corn starch (WCS) blends at several proportions based on the intrinsic characteristics of the starches as well as the granular or molecular interactions between both starches. The starches for this study were chosen based on granule size, amylose content, crystalline pattern, and availability.
Materials and methods
Materials and blend formulations
WCS (Nifra Comercial S. A. de C. V., Cuautitlán Izcalli, México) and PS (Sigma-Aldrich, Bornem, Belgium) were mixed at different proportions (% wt/wt) to obtain four blends in addition to the two native starches: 20% WCS/80% PS (WCS20PS80); 40% WCS/60% PS (WCS40PS60); 60% WCS/40% PS (WCS60PS40); and 80% WCS/20% PS (WCS80PS20). Each blend was thoroughly shaken to achieve complete homogenization prior to each analysis.
Chemical analysis
The amylose and amylopectin percentages were measured following the method reported by Gibson et al.[Citation15] using an amylose/amylopectin assay kit (Megazyme K-AMYL 07/11, Megazyme International, Ireland Ltd., Ireland). All analyses were performed in triplicate. The phosphorous content was measured using a standard method (995.11; AOAC 1998). The sample was dried and incinerated to remove organic matter, and the inorganic residue was solubilized in hydrochloric acid. A color reaction was carried out with this solution based on the formation of a blue complex between phosphate and sodium molybdate [(MoO24MoO3)2H3PO4] in the presence of ascorbic acid as a reducing agent. Analyses were performed in triplicate for each starch, and calculations were carried out using Eq. 1.
where V1 is volume of the solution used in the color reaction (mL); V2 is volume of the volumetric flask containing the incinerated sample (mL); P is amount of phosphorous in the standard curve corresponding to the absorbance of the sample (mg); and W is amount of sample (mg).
Scanning electron microscopy
The WCS and PS were analyzed using a scanning electron microscope (JEOL JSM-6300 Akishima, Tokyo, Japan) operating at 15 A and 20 kV. Completely dry samples were placed on a slide with two-sided carbon conductive tape and then covered with gold. The granule size was determined using the software included with the equipment for 20 granules of both native starches.
X-ray diffraction
X-ray diffraction (XRD) analysis was carried out using a diffractometer (Equinox 2000, Inel, NH, USA) operating at a 35 kV power difference, 15 mA current density, CuKα copper monochromatic radiation line (λ = 1.5406 Å), 2θ data collection angle of 4–80°, 0.1 s–Citation1 rate, and 5 s count time per angle pass. Crystalline and amorphous areas were determined from the diffractogram using Fytik software (version 0.9.8), and the percentage of crystallinity was calculated according to the method reported by Hermans and Weidinger.[Citation16]
Thermal properties
The gelatinization onset (To), peak (Tp), and end-point (Te) temperatures as well as gelatinization enthalpy (ΔH) of native starches and their blends were measured using a differential scanning calorimeter (DSC) (Model 822E, Mettler Toledo, Griefensee, Zurich, Switzerland). Four milligrams were placed in standard 40 µL aluminum crucibles and distilled water was added at a 1:4 (starch/water) ratio using a Hamilton microsyringe. After being hermetically sealed, the samples were analyzed within a 25–95°C interval at a 5°C/min heating rate.
Deconvolution analysis
For deconvolution analysis, the method described by Fonseca-Florido et al.[Citation3] was followed. AAnalyzer® software, which is a peak-fitting program for simultaneously calculating the area under the curve of multiple datasets, was used. Maximum temperatures of gelatinization (Tp) of 62.44°C and 72.25°C were determined for PS and WCS, respectively.
Wide-angle X-ray scattering and small-angle X-ray scattering analysis
Starch slurries containing 20% solids (w/w) were prepared for wide-angle X-ray scattering (WAXS) and small-angle X-ray scattering (SAXS) experiments. Experiments were performed using a SAXSess mcCitation2 (Anton-Paar, Graz, Austria). An X-ray generator with a long fine focus sealed glass X-ray tube (GE) was operated at 40 kV and 50 mA. A focusing multilayer optics and block collimator provided an intense monochromatic primary beam (Cu-Ka, λ = 0.1542 nm). A semitransparent beam enabled the measurement of an attenuated primary beam at a zero scattering vector. The samples were filled into a paste cell sample holder and placed into a TCS 130 temperature-controlled sample holder unit (Anton-Paar) along the line shaped X-ray beam in the camera housing. The samples were heated from 25°C to 95°C at 2.0°C/min. The X-ray 2D patterns were collected at 46°C, 60°C, 70°C, 75°C, 85°C, and 90°C. The 2D scattered intensity distribution was recorded by an imaging-plate (IP) detector and read out by a Cyclone storage phosphor system (Perkin Elmer, USA). The 2D data were integrated into one-dimension using the SAXS Quant 2D 3.91 software. The background scattering contributions were corrected. A desmearing correction was also conducted because of the line collimation.
In practice, on SAXS measurements, a sample contained many stacks oriented over all directions. The intensities of the stacks at a certain q value were distributed in reciprocal space over the surface of a sphere of radius q. This meant that the intensity from the sample of non-oriented stacks was given by Eq. 2.
This is known as the Lorentz correction. The lamellar repeat distance (L) was determined as the Bragg (q/2π = 1/d) spacing of the scattering maximum after the desmeared signals.[Citation17]
Swelling power
Swelling power (SP) was measured in triplicate following the method reported by Anderson et al.[Citation18] with some modifications. One gram of each mixture was placed in 35 mL centrifuge tubes, and 10 mL of distilled water was added. Then, the tubes were agitated in a vortex for 30 s and heated to 60°C, 70°C, 80°C, and 90°C in a water bath for 30 min and then cooled in an ice water bath and centrifuged at 8000 rpm for 25 min (Sorvall Thermo Fischer Scientific, Germany). The supernatant was decanted, and the sediment was weighed. Calculation of SP, expressed in grams (g/g), was conducted using Eq. 3.
Dynamic viscoelastic measurement
Measurements were performed using a controlled force rheometer (AR2000, TA-Instruments, New Castle, UK) in oscillatory mode with a parallel plate geometry of a 60 mm diameter with rugose texture surfaces with a 1 mm gap and solvent trap. The starch suspensions at a 20% solids content were heated (5°C/min at 1 Hz) from 25°C to 95°C, kept at 95°C for 1 min, and then cooled (5°C/min at 1 Hz) to 25°C. The storage module (G'), loss module (G“), and loss angle tangent (δ) were evaluated. Water evaporation from samples during measurement was prevented by using a geometry cover that generated a humidity saturated environment (RH = 100%). Before viscoelastic measurements, the linear viscoelasticity zone was calculated for the evaluated temperature range (25–95°C) following the method reported by Toro-Vázquez et al.[Citation19] Once the starch blends were cooled, the viscoelastic properties of the formed gels were evaluated, including the storage module (G'), loss module (G“), and loss angle tangent (δ) at 25°C and 1% deformation as well as a 0.1–100 s–Citation1 sweep frequency.
Viscosity profiles
The viscosity profiles were obtained using a rheometer (AR1500, TA-Instruments) equipped with a starch cell (aluminum cell insulated to control temperature). Samples were prepared in suspensions of 8% starch (dry base, db) in 25 g of distilled water and were processed with the following settings. The paddle speed was set at 78.53 rad/s from 25°C to 50°C; then, samples were heated from 50°C to 90°C; for 2.5 min at 90°C, followed by cooling to 50°C; and finally, for 2 min at 50°C (12.2°C/min heating and cooling rates; 16.75 rad/s angle speed). The peak viscosity (PV), viscosity after 2.5 min at 90°C (T), viscosity after 2 min at 50°C (E), breakdown viscosity (PV–T), and setback viscosity (E–T) were calculated from the viscosity profiles.
Statistical analysis
The results were analyzed with random one-way ANOVA, and a Tukey test to compare means (p ≤ 0.05). Analyses were carried out using the SigmaPlot, version 11.0, statistics package.
Results and discussion
Native starches characterization
The amylose contents were 26.7% and 2.36% for PS and WCS, respectively. According to the amylose content, PS was classified as a normal starch and WCS as a waxy starch.[Citation20,Citation21] The phosphorous content in PS was 0.076 ± 0.002% (760 ppm), which was similar to the interval reported by other authors[Citation6,Citation22] and gave PS the highest phosphate monoester content among starches. Phosphorous was present in the form of phosphate groups covalently bonded to amylopectin.[Citation23]
The phosphorous content in WCS was 0.004 ± 0.001% (40 ppm), despite its low level of amylose content. In WCS, phosphorous can form inclusion complexes with amylopectin.[Citation20,Citation23] Phosphorous in WCS occurred as phospholipids anchored to amylose and long chains of amylopectin (DP > 60). PS showed a B-type XRD pattern, which is typical of tubers and roots. Meanwhile, WCS showed an A-type XRD, which is characteristic of cereals (diffractograms not shown). Relative to the crystallinity percentage, the values were 19.33% and 25% for PS and WCS, respectively.
The granule sizes () ranged from 8.6 to 42 µm (mean of 27.1 µm) in PS and from 4.5 to 20 µm (mean of 10.2 µm) in WCS. In PS, the granule shape was oval and irregular with a smooth surface. In WCS, the granule shape was polygonal and spherical with an irregular and rough surface. PS granules were about two times larger than WCS granules.
Thermal properties
Analysis of the thermal properties (To, Tp, and Te) showed that WCS (72.50°C) had a higher gelatinization temperature than PS (62.56°C) (). Variations in transition temperatures between these starches can be attributed to differences in the amylopectin content, crystallinity, X-ray pattern, and size distribution.
Table 1. Thermal properties of PS, WCS, and their blends (WCS/PS).
Two shoulders were observed in each endothermal peak (). The first shoulder corresponded to PS and the second shoulder was associated with WCS based on their transition temperatures. The intensity of the endothermal signals for each starch changed according to the proportion in the blend. The thermograms for the blends showed an additive behavior because each starch gelatinized independently, which was favored by the differences in gelatinization temperatures (approximately 10°C) and excess water content (20% solids). Under conditions where there was enough water, each starch gelatinized with no restrictions due to the presence of the other starch. A similar behavior has been reported in other studies that recorded two endotherms in starch blends of potato and maize,[Citation14] canna and maize,[Citation5] or yam and cassava.[Citation24]
Figure 2. (A) Thermograms and (B) deconvolution of endothermic peaks of PS, WCS, and their blends (WCS/PS).
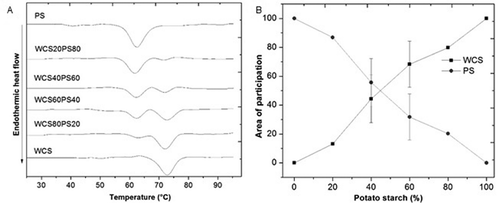
In the blends, To and Te () did not show significant differences with respect to PS and WCS, respectively. The Tp values were divided between both starches. Based on the DSC transition temperatures of the blends, it was difficult to establish whether there were interactions between the starches. In these cases, the deconvolution method applied to blend thermograms was useful for evaluating whether there was an additive or non-additive effect on the gelatinization enthalpy.
The deconvolution of the area of each shoulder of the endothermic peak of the blends allowed us to determinate the participation of each starch in the gelatinization enthalpy. shows the contribution of each starch. In all of the evaluated blends, the starches gelatinized independently because the calculated areas corresponded to the proportion of each starch in the blend, for example, in the blend WCS80PS20, WCS and PS had a participation area of 79.77% and 20.23%, respectively. Participation in the area of the endothermal peaks suggested that neither of the two starches had restrictions or modifications in their thermal properties. These results differed from the results from other starch blends, such as the non-additive effects reported by Fonseca-Florido et al.[Citation3] regarding the contribution of the area depending on the amount of solids content (5% and 40%) for achira and amaranth starch blends, which affected the gelatinization process of both starches. However, in this study, blends of WCS and PS showed an additive effect, which was supported by the participation of each starch in the endothermic peaks corresponding to the gelatinization process. This behavior could be explained by the differences in the packing of the double helices of amylopectin and differences in gelatinization temperatures between both starches (>10°C), which resulted in independent gelatinization promoted by excess water. This outcome has been clearly demonstrated for blends of starches that have large differences in their gelatinization temperatures and thus no overlap in their individual gelatinization endotherms, such as for blends of sweet potato and mung bean starches,[Citation7] waxy maize and barley starches,[Citation1] and rice starches with different amylose contents.[Citation13]
Wide-angle X-ray scattering and small-angle X-ray scattering analysis
The 1D WAXS patterns obtained during in situ heating of native starches and blends are shown in . A complete rupture of the structure was observed above 70°C and 75°C for PS () and WCS (Fig. 3D), respectively, which indicated that complete gelatinization occurred. This behavior was related to the gelatinization properties of each starch. The WCS60PS40 and WCS80PS20 blends showed crystalline structure changes as a function of temperature. The WCS60PS40 sample showed that the crystalline plane signals (200, 201, 300, 211), and (311) were associated with the A-type starch (WCS) and the crystalline planes (100), (110), (101), and (401) were associated with the B-type starch (PS) at 46°C. However, when the sample was heated (at approximately 60°C), the crystalline planes of PS and WCS began to disappear, although the signals for WCS (201, 300, 211) remained, which showed the same behavior as the DSC thermograms where PS gelatinized first. At 70°C, the WAXS pattern showed a complete disorganization of starch granules associated with the complete gelatinization of both starches in the blend. The WCS80PS20 blend showed the same behavior. Similar results were reported for PS blended with maize, waxy maize, rice, and waxy rice starches, in which a complete gelatinization pattern was achieved in starch blends as measured by synchrotron WAXD.[Citation25]
Figure 3. Wide-angle X-ray scattering (WAXS) patterns of (A) PS, (B) WCS60PS40, (C) WCS80PS20, and (D) WCS during in situ heating.
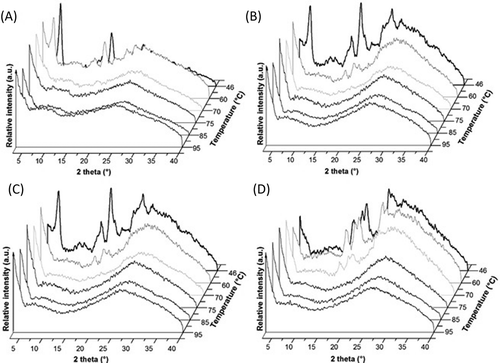
The morphological study was complemented by SAXS analysis. The PS, WCS, WCS60PS40, and WCS80PS20 samples showed a scattering maxima at q values of 0.66 nm–Citation1 at 46°C (), which corresponded to a lamellar thickness (L) of 9.5 nm. This value agreed with previously reported values for WCS and/or PS starches.[Citation26,Citation27] The SAXS profiles revealed a coherent melting of the lamellar structure. Nevertheless, the starch samples presented different behaviors related to the PS weight content. For example, WCS60PS40 maintained the SAXS maximal position even above the PS gelatinization temperature (60°C), although the scattering intensity decreased. The same behavior was shown by WCS. This behavior could be explained in terms of delays in the laminar disorganization of WCS during the hydrothermal process due to its internal organization.[Citation28] On the other hand, the WCS80PS20 blend showed a scattering maxima at 46°C. However, this signal decreased at 60°C and disappeared at higher temperatures, which was an outcome similar to the PS behavior. This result indicated that the lamellar order was completely lost at temperatures above the PS gelatinization temperature. By studying starch blends, some authors have reported that, in many cases, each starch gelatinizes independently of the other starch when there is high water content (>65%),[Citation1,Citation6,Citation7] which could facilitate a complete gelatinization of WCS and PS. For this reason, no changes in the gelatinization properties of blends compared with pure starches were observed in this study because the disruption of amylopectin double helices and the lamellar order were not affected by possible water competition between starches.
Swelling power
The SP is shown in . PS swelled to 6 g/g beginning at 60°C, exhibited no changes at 70°C and 80°C, and swelled only slightly more at 90°C. The PS behavior is a consequence of the phosphate groups esterifying the amylopectin fraction as well as the granule size and its B-type pattern, which contributed to the higher SP.[Citation7,Citation12] By contrast, WCS consistently responded to higher temperatures with higher SP values, with a high value (7.22 g/g) at 90°C that was barely above the value of PS. This behavior could be due to its closed internal structure (A-type pattern) and higher amylopectin content. Therefore, it requires higher temperatures to achieve maximum swelling and gelatinization.[Citation23] The SP difference between both starches was also related to the transition temperatures observed in DSC, internal structure and composition differences, and internal disorganization due to gelatinization that increased water absorption in granules.
The WCS20PS80 blend showed lower SP values than PS at 60°C and 70°C due to the presence of WSC interfering with swelling for PS and as a consequence of the differences in granule size, which limited water absorption by PS. However, at 80°C and 90°C, the SP increased, as WCS showed a better SP at higher temperatures than PS.
The blends containing high proportions of WCS showed higher SP values at higher temperatures compared with the blends containing higher PS proportions. The highest values (7.71–8.70 g/g) were exhibited by the WCS60PS40 blend at 70°C, 80°C, and 90°C, followed by the WCS80PS20 blend (7.32–7.53 g/g) at 80°C and 90°C. These blends likely had better SP at high temperatures compared with the other blends and native starches because WCS had delayed swelling compared with the swelling of WCS alone at the same temperature, as PS first absorbed the surrounding water and caused a delay in the internal disorganization of WCS granules. This behavior could be explained by the contact surface between granules, granule size, and structural characteristics of both starches, and effects on the SP of starches.[Citation3,Citation12] This behavior was not shown by DSC, in which each starch apparently gelatinized independently.
The swelling restrictions of PS due to the presence of WCS and water competition generated between the two starches allowed PS to attain characteristics similar to those achieved through other modifications, such as annealing and heat–moisture treatment. Similar results were reported by other authors[Citation3,Citation5,Citation12] in which the starch with the larger granule size showed a greater restriction in SP.
According to the results obtained in this study, the proportion at which PS and WCS were blended allowed the blends to reach lower or higher values of SP. Thus, depending on the final use, such as when starch is used as a filler or thickening agent, the most suitable proportion of each starch in the blend could be established.
Rheological properties
The temperature results from the dynamic oscillation test are shown in . The initial rise in G' (storage modulus) is attributed to the degree of granular swelling that fills the available volume in the system and intergranular contact between swollen granules.[Citation13,Citation21] The G' values for all samples rose progressively at certain temperatures until they reached a maximum (G'max) and then fell as heating continued, which indicated that there was a disruption in the starch granules during prolonged heating. This disruption was caused by a loss of the crystalline remnant in the swollen starch granule, which then deformed and lost its structure. As the PS content decreased, so did the G'max values in the blends due to delays in the swelling of starches, as a consequence of water competition and granules surrounding each other, creating a physical barrier that affected the behavior of the starches in the blend as well as the rheological properties. PS, unlike WCS, showed the highest G' values, which were favored by a high phosphate monoester content, larger granule size, and organization of the internal structure. A smaller decrease in G' values (breakdown) was observed during heating in WCS, which reflected granule rigidity and a more stable structure.
Figure 6. (A) Gelatinization and gelation kinetics; (B) tan δ; and (C) viscosity curves of PS, WCS, and their blends (WCS/PS) during heating and cooling stages.
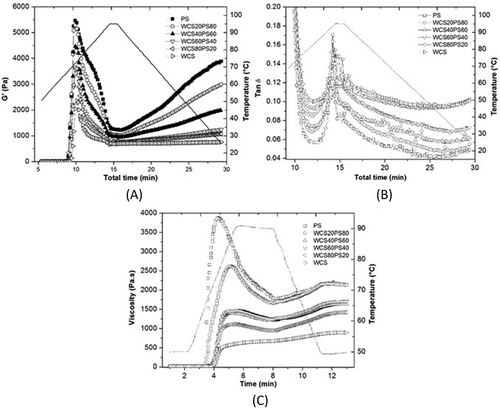
In the cooling stage, the increase in G' (Pa) was caused by the transition of amylose during heating from a disordered (unraveling) to an ordered (raveling) state. Amylose formed double helices as well as a three-dimensional network in which it saturated swollen granules and amylopectin fragments (). In the case of PS, the three-dimensional networks that were formed consisted of swollen granules and/or fragments in an amylose matrix, whereas in WCS, the low amylose content (<3%) could have been responsible for its very low G' values and the weakness of the network, which formed only with swollen granules[Citation7,Citation13] (). For this reason, as the PS content in the blends decreased, so did the G' values, which suggested that there was a transition from a strong network with amylose–amylopectin interactions to a weaker network containing only amylopectin. The starch components, granule shapes, and size distribution affected the packing characteristics.[Citation29,Citation30]
The blends showed a more stable behavior compared with PS in breakdown but also higher values compared with the elastic modulus of WCS during the cooling stage. This behavior could be due to molecular and granular interactions between starches and water competition in which each starch presented delayed swelling. The characteristics of the network formed by each starch led to the creation of a three-dimensional network of double helices of amylose in PS, which was reinforced by fragmented and swollen WCS granules in the blend. Depending on the proportion of the starches in the blends, the G' values were generally between the values of the individual starches.
Tan δ described the viscosity/elasticity ratio in the viscoelastic region of the materials. Initially, in the samples that were studied, tan δ decreased and then increased to reach a maximum value at 13 min and 88°C during the temperature scan (). The observed peak could have been due to the disintegration of starch granules followed by lixiviation, which resulted in system fluidity[Citation19] and a consequential decrease in elasticity. As WCS increased in the blends, the maximum value of tan δ increased, which led to a loss of modulus due to the high amylopectin content of WCS. In the cooling stage, G' increased as the molecules reorganized, which resulted in the lowest tan δ values and indicated that a gel formed.
Viscosity profiles
Viscosity was the highest in PS during heating. The peak viscosity (PV) value in the blends decreased as the proportion of PS decreased (Fig. 6C). The starch viscosity depends on the granule size and rigidity, degree of swelling, stability under heat, and shearing of swollen granules, as well as the structural differences of amylose and amylopectin.[Citation31] In PS with a high phosphate monoester content,[Citation12] PV was reached at 75°C (temperature peak (TP)) as the large granules swelled rapidly. Meanwhile, blends showed an increase in TP (86–91°C) due to the stability of WCS granules, water competition between starches and SP limitation.
The breakdown viscosity (BV) is related to the granule disintegration level. The BV values decreased as the proportion of WCS (128 Pa.s) increased in each blend, which could be attributed to the granule rigidity, heat stability, and shear forces from WCS. The results indicated that there was less susceptibility to disintegration by shear when the WCS proportion increased in the blends, which modified the resistance of the granules in the blends. This behavior could be observed in the BV values of WCS20PS80 (941 Pa.s), WCS40PS60 (190 Pa.s), WCS60PS640 (226 Pa.s), and WCS80PS620 (163 Pa.s).
The setback viscosity (SB) increased slightly as the PS (328 Pa.s) content decreased in the blends, for example, WCS20PS80 (452 Pa.s), WCS40PS60 (423 Pa.s), WCS60PS640 (463 Pa.s), WCS80PS620 (472 Pa.s), and WCS (220 Pa.s). This non-additive effect occurred due to interactions between the components of both starches that were leached out after granule disruption, which resulted in a gel structure that was reinforced with amylose and contributed to the high setback value. This behavior was also reported by Waterschoot et al.,[Citation11] Wu et al.,[Citation7] and Fonseca-Florido et al.[Citation14] for starch blends.
The breakdown viscosity is also related to the peak time. The PS value was 4.3 min, and this value increased to 5.1 min in the WCS20PS80 and WCS40PS60 blends. The highest value was 5.7 min for WCS60PS40 and then 5.4 min for WCS80PS20 and WCS. The blends showed higher values than PS due to the presence of WCS granules surrounding PS, which limited its swelling power.
Conclusion
An additive effect was observed during the gelatinization of WCS and PS blends, but once the disruption of granules occurred and the components of the starches were solubilized, the blends showed granular and molecular interactions that modified the rheological and viscosity properties of the blends.
During the swelling process of starch blends, competition for water absorption took place because the PS showed a delay in swelling power, which slowed the release of the amylose and resulted in lower G' values for the blends. A non-additive effect was observed in the SB for all blends, which was associated with the interactions between the starch components. The granules of WCS reinforced the network that was formed from amylose that leached out from PS, resulting in higher setback values compared with those obtained with only one of the native starches. The intrinsic characteristics of each starch, such as the amylose content, granule size, and crystalline pattern, determined the type of interactions that occurred between the starches and affected the modification of the physicochemical and functional properties.
References
- Ortega-Ojeda, F.; Eliasson, A. Gelatinization and Retrogradation Behavior of some Starch Blends. Starch/Starke 2001, 53, 520–529. 10.1002/1521-379X(200110)53:10<520::AID-STAR520>3.0.CO;2-D
- Park, E.; Kim, H.; Kim, J.; Lim, S. Pasting Properties of Potato Starch and Waxy Maize Starch Blends. Starch/Starke 2009, 61, 352–357. 10.1002/star.200800029
- Fonseca-Florido, H.A.; Méndez-Montealvo, G.; Velazquez, G.; Gómez-Aldapa, C.A. Thermal Study in the Interactions of Starches Blends: Amaranth and Achira. Food Hydrocolloids 2016, 61, 640–648. 10.1016/j.foodhyd.2016.06.027
- Obanni, M.; BeMiller, J. Properties of some Starch Blends. Cereal Chemistry 1997, 74, 431–436. 10.1094/CCHEM.1997.74.4.431
- Puncha-Arnon, S.; Pathipanawat, W.; Puttanlek, C.; Rungsardthong, V.; Uttapap, D. Effects of Relative Granule Size and Gelatinization Temperature on Paste and Gel Properties of Starch Blends. Food Research International 2008, 41, 552–561. 10.1016/j.foodres.2008.03.012
- Waterschoot, J.; Gomand, S.; Fierens, E.; Delcour, J. Starch Blends and their Physicochemical Properties. Starch/Stärke 2014, 66, 1–13.
- Wu, K.; Dai, S.; Gan, R.; Corke, H.; Zhu, F. Thermal and Rheological Properties of Mung Bean Starch Blends with Potato, Sweet Potato, Rice, and Sorghum Starches. Food Bioprocess Technology 2016, 9, 1408–1421. 10.1007/s11947-016-1730-1
- Wang, S.; Copeland, L. Molecular Disassembly of Starch Granules during Gelatinization and its Effect on Starch Digestibility: A Review. Food & Function 2013, 4, 1564–1580. 10.1039/c3fo60258c
- Waigh, T.; Gidley, M.; Komanshek, B.; Donald, A. The Phase Transformations in Starch during Gelatinisation: A Liquid Crystalline Approach. Carbohydrate Research 2000, 328, 165–176. 10.1016/S0008-6215(00)00098-7
- Vermeylen, R.; Derycke, V.; Delcour, J.; Goderis, B.; Reynaers, H.; Koch, M. Gelatinization of Starch in Excess Water: Beyond the Melting of Lamellar Crystallites. A Combined wide-and Small-angle X-ray Scattering Study. Biomacromolecules 2006, 7, 2624–2630. 10.1021/bm060252d
- Waterschoot, J.; Gomand, S.V.; Willebrords, J.K.; Fierens, E.; Delcour, J.A. Pasting Properties of Blends of Potato, Rice and Maize Starches. Food Hydrocolloids 2014, 41, 298–308. 10.1016/j.foodhyd.2014.04.033
- Waterschoot, J.; Gomand, S.; Delcour, J. Impact of Swelling Power and Granule Size on Pasting of Blends of Potato, Waxy Rice and Maize Starches. Food Hydrocolloids 2016, 52, 69–77. 10.1016/j.foodhyd.2015.06.012
- Hagenimana, A.; Pu, P.; Ding, X. Study on Thermal and Rheological Properties of Native Rice Starches and their Corresponding Mixtures. Food Research International 2005, 38, 257–266. 10.1016/j.foodres.2004.05.009
- Fonseca-Florido, H.; Hernández, J.; Rodríguez, A.; Castro, J.; Acevedo, O.; Chavarria, N.; Gómez, C. Thermal, Rheological and Mechanical Properties of Normal Corn and Potato Starch Blends. International Journal of Food Properties. 2017, 20, 611–622. 10.1080/10942912.2016.1171779.2016
- Gibson, T.; Solah, V.; McCleary, B. A procedure to Measure Amylose in Cereal Starches and Flours with Con A. Journal of Cereal Science 1997, 25, 111–119. 10.1006/jcrs.1996.0086
- Hermans, P.; Weidinger, A. Quantitative X‐Ray Investigations on the Crystallinity of Cellulose Fibers. A Background Analysis. Journal of Applied Physics 1948, 19, 491–506. 10.1063/1.1698162
- Cameron, R.; Donald, A. A Small-angle X-ray Scattering Study of the Annealing and Gelatinization of Starch. Polymers 1992, 33, 2628–2635. 10.1016/0032-3861(92)91147-T
- Anderson, A.; Conway, H.; Pfeifer, V.; Griffin, E. Gelatinization of Corn Grits by Roll and extrusion Cooking. Journal of Cereal Science 1969, 14, 4–12.
- Toro-Vásquez, J.; Gómez-Aldapa, C.; Aragon-Piña, A.; Brito- De La Fuente, E.; Dibildox-Alvarado, E.; Charó-Alonso, M. Interaction of Granular Maize Starch with Lysophosphatidylcholine Evaluated by Calorimetry, Mechanical and Microscopy Analysis. Journal of Cereal Science 2001, 38, 269–279. 10.1016/S0733-5210(03)00026-2
- BeMiller, J.; Whistler, R. Starch: Chemistry and Technology; 3rd. Elsevier: Oxford, UK, 2009.
- Singh, N.; Singh, J.; Lovedeep, K.; Singh, N.; Singh, B. Morphological, Thermal and Rheological Properties of Starches from Different Botanical Sources. Food Chemistry 2003, 81, 219–231. 10.1016/S0308-8146(02)00416-8
- Thitipraphunkul, K.; Uttapap, D.; Piyachomkwan, K.; Takeda, Y. A Comparative Study of Edible Canna (Canna edulis) Starch from Different Cultivars. Part II. Molecular Structure of Amylose and Amylopectin. Carbohydrate Polymers 2003, 54, 489–498. 10.1016/j.carbpol.2003.08.003
- Pérez, S.; Bertoft, E. The Molecular Structures of Starch Components and their Contribution to the Architecture of Starch Granules: A Comprehensive Review. Starch/Stärke 2010, 62, 389–420. 10.1002/star.201000013
- Karam, L.; Grossmann, M.; Silva, R.; Ferrero, C.; Zaritzky, N. Gel Textural Characteristics of Corn, Cassava and Yam Starch Blends: A Mixture Surface Response Methodology Approach. Starch/Starke 2005, 57, 62–70. 10.1002/star.200400328
- Waterschoot, J.; Gomand, S.; Delcour, J.; Goderis, B. Direct Evidence for the Non-additive Gelatinization in Binary Starch Blends: A Case Study on Potato Starch Mixed with Rice or Maize Starches. Food Hydrocolloids 2015, 50, 137–144. 10.1016/j.foodhyd.2015.04.020
- Hernández-Hernández, E.; Ávila-Orta, C.; Hsiao, B.; Castro-Rosas, J.; Gallegos-Infante, J.A.; Morales-Castro, J.; Gómez-Aldapa, C. Synchrotron X-ray Scattering Analysis of the Interaction between Corn Starch and an Exogenous Lipid during Hydrothermal Treatment. Journal of Cereal Science 2011, 54, 69–75. 10.1016/j.jcs.2011.03.001
- Vermeylen, R.; Goderis, B.; Delcour, J.A. An X-ray Study of Hydrothermally Treated Potato Starch. Carbohydrate polymers 2006, 64, 364–375. 10.1016/j.carbpol.2005.12.024
- Vermeylen, R.; Derycke, V.; Delcour, J.; Goderis, B.; Reynaers, H.; Koch, M. Structural Transformations during Gelatinization of Starches in Limited Water: Combined Wide-and Small-angle X-ray Scattering Study. Biomacromolecules 2006, 7, 1231–1238. 10.1021/bm050651t
- Méndez-Montealvo, G.; García-Suárez, F.J.; Paredes-López, O.; Bello-Pérez, L.A. Effect of Nixtamalization on Morphological and Rheological Characteristics of Maize Starch. Journal of Cereal Science 2008, 48, 420–425. 10.1016/j.jcs.2007.10.007
- Gupta, M.; Bawa, A.; Semwal, A. Morphological, Thermal, Pasting, and Rheological Properties of Barley Starch and their Blends. International Journal of Food Properties 2009, 12, 587–604. 10.1080/10942910801947763
- Crosbie, G.; Ross, A. The RVA Handbook; AACC International: 3340 Pilot Knob Road, St. Paul, MN, 2007; 152 pp.