ABSTRACT
Chicken bone protein hydrolysate (CBPH) and its peptide fractions (CBPH-I, molecular weight < 3 kDa; CBPH-II, molecular weight 3–10 kDa) were heated with galactose in aqueous solution at 100°C for 90 min. Antioxidant activities of CBPH, CBPH-I, CBPH-II, and their derived Maillard reaction products (MRPs) were evaluated. CBPH, peptide fractions, and their MRP exerted strong DPPH radical scavenging activity and reducing power, but low scavenging activity toward the hydroxyl radicals. All the tested antioxidant activities of CBPH and its peptide fractions were significantly increased after the Maillard reaction with galactose. The increment in the antioxidant activity of CBPH-II MRP was higher than that of CBPH-I MRP, although more glycation was found in CBPH-I MRP. The results indicated that the improvement of the antioxidant activity of peptide fraction via the Maillard reaction should be closely related to its molecular size.
Introduction
Peptides have a wide range of bioactivities including antioxidant, antimicrobial, antihypertensive, and immunomodulating activities.[Citation1,Citation2] They can be obtained by the enzymatic proteolysis of proteins, and the most active ones generally contain 3–20 amino acid residues. Antioxidant activity is commonly found in peptides from various food proteins, such as bean protein,[Citation3] peanut protein,[Citation4] meat protein,[Citation5] fish skin protein,[Citation6] and milk protein.[Citation7]
The Maillard reaction is a complex series of reactions between carbonyl and amino groups, which often occurred in the food system during process and storage. It is noted that the antioxidant activity of amino acids, peptides, or proteins can be enhanced through the Maillard reaction with reducing sugar.[Citation8–Citation11] Thus, Maillard reaction products (MRPs) might act as effective antioxidants in the food system. In recent years, the antioxidant potential of protein hydrolysates—sugar systems[Citation12,Citation13] and the related processing conditions such as temperature,[Citation5] pH,[Citation14] and sugar type[Citation10] have been widely reported. Owing to the complexity of their structure and composition, the major peptides in protein hydrolysates have different reactivity toward carbonyl groups in reducing sugars, and their corresponding MRPs may reduce radical chain reactions, scavenge the reactive oxygen, decompose hydrogen peroxide, and chelate metal ions.[Citation11,Citation15,Citation16] However, the relationship between the molecular size of peptides and the antioxidant activity of the corresponding MRP has not been clearly identified.
Chicken bone is an abundant by-product of chicken meat processing. Approximately 28 million tons of raw chicken were consumed in China in 2012, producing theoretically 5.6–14 million tons of chicken bone.[Citation17] It is generally used to produce chicken flavoring, especially after the Maillard reaction with reducing sugar. Our previous study showed that chicken bone protein hydrolysate (CBPH)-MRP derived from galactose had stronger antioxidant activity than those from other hexoses.[Citation18] The aim of this study was to prepare the MRP from CBPH and its peptide fractions, and evaluate the changes in their antioxidant activities via the Maillard reaction with galactose. Glycation extent and the formation of intermediate, brown and fluorescent compounds were also determined.
Materials and methods
Materials
Chicken bones were purchased from a local poultry factory (Hangzhou, Zhejiang, China), and kept at −18°C for not more than one month. Protein content in the bones was 18.72% by the Kjeldahl method.[Citation19] Papain (4.7 × 104 U/g), neutral protease (6.0 × 105 U/g), and trypsin (1.4 × 104 U/g) were supplied by Huasen Co. (Zhengzhou, Henan, China). o-phthaldialdehyde (OPA), 1,1-diphenyl-2-picryl-hydrazil (DPPH), and 1,10-phenanthroline were purchased from Sigma (St. Louis, MO, USA). All other reagents were of analytical grade.
Preparation of CBPH and peptide fractions
Chicken bones were cooked in distilled water (1:1.5, w/v) in a YXQ-LS-50A autoclave (Shanghai Boxun Co., Shanghai, China) at 130°C for 20 min, followed by grinding into a bone slurry in a Model 24 mill (Buyang Co., Jinhua, China) through a 50-mesh sieve. The slurry was hydrolyzed using mixed enzymes (papain, 500 U/g bone; neutral protease, 500 U/g bone; trypsin, 1000 U/g bone) at 55°C for 3.5 h without pH control, as described by Xu et al.[Citation18] The hydrolysis was terminated by heating at 100°C for 15 min to inactivate the enzymes, followed by centrifugation (5,000 × g, 20 min) to remove the floating fat and insoluble residues. Hydrolysis degree was 34.01%. The obtained solution was termed as CBPH. Then, CBPH was separated using an Amicon 8400 ultrafiltration cell (Amicon Inc., Beverly, MA, USA) with 3 and 10 kDa cutoff membranes (Millipore Corp., Billerica, MA, USA). CBPH-I and CBPH-II represented the fractions with molecular weigh distribution of <3 and 3–10 kDa, respectively. CBPH, CBPH-I, and CBPH-II were collected, lyophilized, and stored at 4°C until used.
Preparation of MRPs
MRPs from CBPH, CBPH-I, and CBPH-II with galactose were prepared as described by Xu et al.[Citation18] Briefly, CBPH, CBPH-I, and CBPH-II were dissolved in distilled water at a content of 7.0 mg/mL, respectively. Galactose was added into the solutions at a weight ratio of 3:1 (galactose: CBPH or the peptide fraction). The Maillard reaction was carried out at 100°C for 90 min, followed by rapid cooling in an iced bath and centrifuging at 5,000 × g for 20 min. The resulting solutions were termed as CBPH MRP, CBPH-I MRP, and CBPH-II MRP, respectively. The unheated solution of the CBPH (or CBPH-I, CBPH-II)–galactose system was used as the control. After the determination of free amino group, browning, UV absorbance, and florescence, all the solutions were further lyophilized and stored at 4°C for antioxidant analysis.
Determination of free amino group content
The content of free amino group was determined according to a modified OPA method.[Citation20] Sample solution (200 μL) was added to 6 mL of sodium borate buffer (50 mM, pH 9.5) containing 80 g/L OPA, 10 g/L SDS, and 25 μM β-mercaptoethanol. After incubation at 25°C for 3 min, the absorbance was recorded at 340 nm using a UV-762 spectrophotometer (Meipuda Co., Shanghai, China). The content of free amino group was calculated from the standard curve using L-lysine and expressed as mmol NH2/L.
Determination of UV absorbance and browning
UV absorbance and browning were determined at 294 and 420 nm, respectively, as described by Laroque et al.,[Citation20] using a UV-762 spectrophotometer (Meipuda Co., Shanghai, China).
Determination of fluorescence spectra
Fluorescence spectra were determined according to the method of Lertittikul et al.,[Citation14] using an RF-1501 fluorescence spectrophotometer (Shimadzu Co., Kyoto, Japan). The excitation was set at 347 nm, and the emission was recorded from 300 to 500 nm at the scan rate of 120 nm/min. The constant slit was 5 nm.
Determination of DPPH radical scavenging activity
DPPH radical scavenging activity was determined according to the method of Sun et al.[Citation5] with slight modification. Each lyophilized sample was dissolved in distilled water at the contents of 2, 4, and 6 mg/mL. The sample solution (2 mL) was mixed with 2 mL of 0.15 mM DPPH in 95% ethanol, followed by incubation at 25°C for 30 min in the dark. The absorbance of the reaction was read at 517 nm. DPPH radical scavenging activity was calculated using the following formula:
where As is the absorbance of the sample, Ac is the absorbance of the control (95% ethanol was added instead of DPPH solution), and Ab is the absorbance of the blank (distilled water was added instead of sample solution).
Determination of hydroxyl radical scavenging activity
Hydroxyl radical scavenging activity was measured according to the method of Liu et al.[Citation12] Each lyophilized sample was dissolved in distilled water at the contents of 2, 6, and 10 mg/mL. The sample solution (2 mL) was mixed with a reaction solution containing 0.6 mL of phenanthroline (5 mM), 0.4 mL of sodium phosphate buffer (0.2 M, pH 7.4), and 0.6 mL of ferrous sulfate (5 mM). Then 0.4 mL of hydrogen peroxide (1 g/L, freshly prepared) was added to initiate the reaction, followed by incubation at 37°C for 60 min. The absorbance of the reaction was read at 536 nm. Hydroxyl radical scavenging activity was calculated using the following formula:
where As is the absorbance of the sample, Ac is the absorbance of the control (distilled water was added instead of hydrogen peroxide solution and sample solution), and Ab is the absorbance of the blank (distilled water was added instead of the sample solution).
Determination of reducing power
Reducing power was determined according to the method of Vhangani and Wyk.[Citation10] Each lyophilized sample was dissolved in distilled water at the contents of 2, 4, and 6 mg/mL. The sample solution (2 mL) was mixed with 2 mL of sodium phosphate buffer (0.2 M, pH 6.5) and 2 mL of potassium ferricyanide (10 g/L), followed by incubation at 50°C for 20 min. The reaction solution was mixed with 1 mL of trichloroacetic acid (100 g/L) and subsequently centrifuged at 2,000 × g for 10 min. Then, the supernatant (2 mL) was mixed with 2 mL of distilled water and 0.4 mL of ferric chloride (1 g/L). The blank was prepared by replacing the sample solution with distilled water. The absorbance of the reaction was read at 700 nm. Reducing power was expressed as A700nm.
Statistical analysis
All data were subjected to analysis of variance (ANOVA). Significant differences among means from triplicate analyses were determined using Duncan’s multiple range tests using SPSS 21.0 (IBM-SPSS Inc., Chicago, Il, USA).
Results and discussion
Glycation extent
The Maillard reaction occurs mainly through the interaction between carbonyl groups in reducing sugar and free amino groups in amino acids, peptides, or proteins. Thus, the loss of free amino groups is often used as an indicator of glycation extent during the Maillard reaction.[Citation11,Citation20] The contents of free amino groups in CBPH, peptide fractions, and their MRP are shown in . CBPH-II had much fewer free amino groups than CBPH and CBPH-I, due to its relatively high molecular weight. A significant reduction in free amino groups was found in the MRP solutions, indicating that α- or ε-NH2 groups were covalently attached to carbonyl groups in galactose to a great extent. Similar results were reported in shrimp hydrolysate-sugar model systems[Citation20] and bovine casein peptide-sugar model systems.[Citation11] The content of free amino groups in CBPH-I MRP was decreased by 26.81%, more than that in CBPH-II MRP (21.70%). That is, there was a higher glycation extent in CBPH-I MRP. It might be attributed not only to the higher number of free amino groups in low-molecular-weight peptides, but also to their greater reactivity toward reducing sugar. Su et al.[Citation15] considered that the peptides with low molecular weight were more likely to crosslink with carbonyl groups in reducing sugar.
Table 1. Free amino group in CBPH and its peptide fractions before and after the Maillard reaction with galactose.
Formation of intermediate, brown, and fluorescent compounds
Intermediate compounds are generated in the Maillard reaction and then polymerized into brown compounds. Intermediate and brown compounds are often determined by UV absorbance at 294 nm and browning at 420 nm, respectively. UV absorbance and browning of CBPH, peptide fractions, and their MRP are shown in . UV absorbance and browning of CBPH and its peptide fractions increased after heating with galactose. Similar results were also reported by Laroque et al.[Citation20] and Hwang et al.[Citation9] The increments in UV absorbance and the browning of CBPH-I MRP were much higher than those of CBPH-II MRP. That is, higher amounts of immediate and brown compounds were formed in the MRP derived from lower-molecular-weight peptides. It was well with the loss of free amino groups (). These results further clarified the greater glycated reactivity of the peptides with lower molecular weight.
Table 2. Browning and UV absorbance in CBPH and its peptide fractions before and after the Maillard reaction with galactose.
The Maillard reaction is also related to the development of fluorescent compounds, which are also the precursors of brown pigments. The fluorescence emission spectra of CBPH, peptide fractions, and their MRP are shown in . There was a fluorescence peak at 347 nm found in CBPH and its peptide fractions, which might be induced by some endogenous fluorophores. This peak was not observed in the MRP, representing the loss of endogenous fluorophores in CBPH and its peptide fractions after the Maillard reaction with galactose. A fluorescence peak at 425 nm was observed in the MRP derived from CBPH and its peptide fractions. Previous studies have suggested that fluorescent compounds in the MRP have a maximum excitation at wavelengths between 340 and 370 nm, and the wavelengths of maximum emission are between 420 and 450 nm.[Citation21] CBPH-II MRP had a higher intensity for this peak than CBPH-I MRP. This indicated that more fluorescent compounds were formed in the MRP derived from high-molecular-weight peptides. The development of fluorescent compounds was contrary to the loss of free amino groups (), and the increments of browning and UV absorbance (). Benjakul et al.[Citation22] presented that there was no correlation between the changes of UV absorbance and fluorescence intensity in porcine plasma protein-sugar model systems. It seemed that more fluorescent compounds were generated in the MRP from the peptides with a higher molecular size.
Figure 1. Fluorescence emission spectra of CBPH and its fractions before and after the Maillard reaction with galactose. Excitation wavelength was 347 nm. CBPH (or CBPH-I, CBPH-II) is the CBPH (or CBPH-I, CBPH-II)–galactose system without heating (i.e. the control solution). MRP is the CBPH (or CBPH-I, CBPH-II)–galactose system after heating at 100°C for 90 min without pH control (i.e. MRP solution). The control and MRP solutions were diluted fivefold with distilled water.
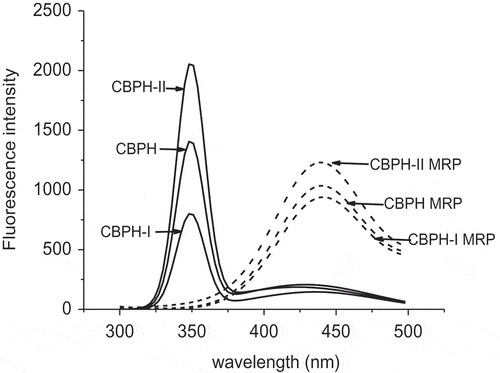
DPPH radical scavenging activity
DPPH radical is relatively stable and has been widely used to evaluate the antioxidant potentials of natural compounds as free radical scavengers or hydrogen donors. The DPPH radical scavenging activities of CBPH, peptide fractions, and their MRP are shown in . CBPH and the peptide fractions showed strong scavenging activities toward DPPH radicals. The activity of CBPH-I was higher than that of CBPH-II. This suggested that lower-molecular-weight peptides were more effective as DPPH radical scavengers than high-molecular-weight peptides. Similar results were also found in peptide fractions prepared from African yam bean seed protein[Citation23] and chicken skin protein hydrolysate.[Citation13]
Figure 2. DPPH radical scavenging activities of CBPH and its fractions before and after the Maillard reaction with galactose. CBPH (or CBPH-I, CBPH-II) is the lyophilized sample from CBPH (or CBPH-I, CBPH-II)–galactose system without heating. CBPH MRP (or CBPH-I MRP, CBPH-II MRP) is the lyophilized sample from CBPH (or CBPH-I, CBPH-II)–galactose system after heating at 100°C for 90 min without pH control.
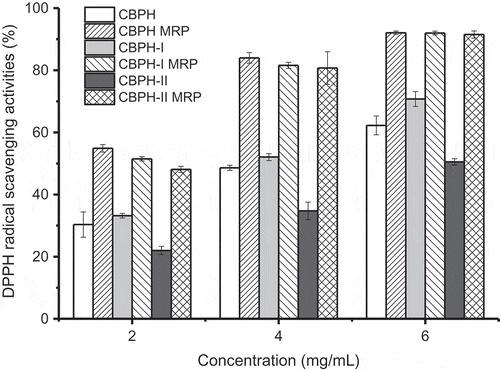
The DPPH radical scavenging activities of CBPH and its peptide fractions were significantly enhanced after the Maillard reaction with galactose. Similar results were reported by Su et al.[Citation15] and Liu et al.[Citation12] who considered that the Maillard reaction could improve the peptides’ scavenging ability against DPPH radicals. Jiang et al.[Citation11] pointed out that intermediate and brown compounds formed in the Maillard reaction could function as hydrogen donors to form stable DPPH-H molecules. The DPPH radical scavenging activity of CBPH-II MRP was similar to those of CBPH MRP and CBPH-I MRP. The increment in the DPPH radical scavenging activity of CBPH-II MRP was higher than that of CBPH-I MRP. It was inconsistent with the loss of free amino groups () and the increments in UV absorbance and browning (), but agreed with the change in fluorescence intensity (). The results suggested that the improvement in the DPPH radical scavenging activity of the MRP was positively correlated with the formation of fluorescent compounds. Morales and Jiménez-Pérez[Citation24] also stated that the fluorescence measurement of heated sugar/amino acid systems was more effective than browning to follow the formation of antioxidant MRP.
Hydroxyl radical scavenging activity
Hydroxyl radical is a highly reactive oxygen species that can hydroxylate proteins, DNA, and lipids. Therefore, the scavenging activity toward hydroxyl radicals is one of the most effective protections against various diseases caused by this radical-induced oxidative stress. The hydroxyl radical scavenging activities of CBPH, peptide fractions, and their MRP are shown in . CBPH and its peptide fractions had low hydroxyl radical scavenging activities, even at the content of 10.0 mg/mL. Yoshimura et al.[Citation25] considered that the reducing products formed in the Maillard reaction could reduce Fe3+ to Fe2+ and thus prolong the Fenton reaction. CBPH and its peptide fractions are poor scavengers toward hydroxyl radicals when compared with hoki frame peptides (activity >70% at the content of 0.5 mg/mL)[Citation16] and whey peptides (activity 23.33 ~ 65.67 at the content of 1 mg/mL).[Citation7] The activity of CBPH-II was higher than that of CBPH-I, which was contrary to the DPPH radical scavenging activity of peptide fractions (). It was similar to the results of peptide fractions from croaker skin protein hydrolysate (Kumar et al., 2012), but different from the reports of Jamdar et al.[Citation26] and Girgih et al.,[Citation27] who found that the peptides with a lower molecular weight had better hydroxyl radical scavenging activity.
Figure 3. Hydroxyl radical scavenging activity of CBPH and its fractions before and after the Maillard reaction with galactose. CBPH (or CBPH-I, CBPH-II) is the lyophilized sample from CBPH (or CBPH-I, CBPH-II)–galactose system without heating. CBPH MRP (or CBPH-I MRP, CBPH-II MRP) is the lyophilized sample from CBPH (or CBPH-I, CBPH-II)–galactose system after heating at 100°C for 90 min without pH control.
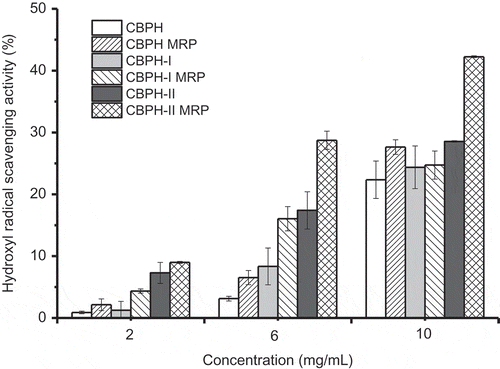
An increase in hydroxyl radical scavenging activity was found in the MRP derived from CBPH and its peptide fractions. Similar results were described in fructose-lysine and ribose-lysine model systems[Citation10] and D-xylose-glycine reaction systems.[Citation28] Hydroxyl radicals’ inhibition of the MRP might be due to their metal chelating capacity and hydrogen peroxide scavenging activity. Hydroxyl radical scavenging activity was found in the order of CBPH-II MRP > CBPH MRP ≈ CBPH-I MRP. The increment in the hydroxyl radical scavenging activity of CBPH-II MRP was higher than that of CBPH-I MRP, except at the content of 2 mg/ml. The improvement in the hydroxyl radical scavenging activity of CBPH and its peptide fraction after the Maillard reaction with galactose was similar to the change in DPPH radical scavenging activity (). It was also not consistent with the loss of free amino groups () and the increments in UV absorbance and browning (), but agreed with the change in fluorescence intensity (). That is, the improvement in the hydroxyl radical scavenging activity of the derived MRP depended largely on the formation of fluorescent intermediate compounds.
Reducing power
The reducing capacity of a compound often serves as an indicator of its potential antioxidant activity. The reducing powers of CBPH, peptide fractions, and their MRP are shown in . CBPH and its peptide fractions showed moderate reducing powers, and the activity of CBPH-I was higher than that of CBPH-II. This result suggested that low-molecular-weight peptides had better reducing power in relation to high-molecular-weight peptides, which is similar to the findings in the peptides prepared from African yam bean seed protein.[Citation23] On the contrary, Girgih et al.[Citation26] reported an increase in the reducing power of hemp seed protein hydrolysate with increasing molecular weight.
Figure 4. Reducing power of CBPH and its fractions before and after the Maillard reaction with galactose. CBPH/CBPH-I/CBPH-II is the lyophilized sample from the CBPH/CBPH-I/CBPH-II–galactose system without heating. CBPH (or CBPH-I, CBPH-II) is the lyophilized sample from the CBPH (or CBPH-I, CBPH-II)–galactose system without heating. CBPH MRP (or CBPH-I MRP, CBPH-II MRP) is the lyophilized sample from the CBPH (or CBPH-I, CBPH-II)–galactose system after heating at 100°C for 90 min without pH control.
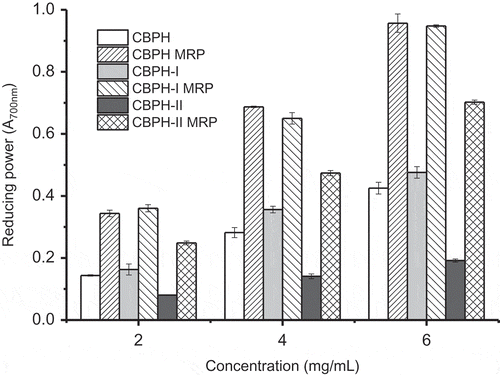
The reducing powers of CBPH and the peptide fractions were significantly improved by the Maillard reaction with galactose. Hydroxyl and pyrrole groups of brown compounds in the MRP can donate electrons to form stable products, thereby terminating the free radical chain reactions.[Citation8,Citation9] Intermediate compounds in the MRP may break the radical chain reaction by donating hydrogen atoms.[Citation29] Reducing power was found in the order of CBPH MRP ≈ CBPH-I MRP > CBPH-II MRP. The increment in the reducing power of CBPH-II MRP was higher than that of CBPH-I MRP, similar to the change in the radical scavenging activity of the MRP derived from the peptide fractions ( and ). The change in the reducing power of CBPH and its peptide fractions following the Maillard reaction with galactose was in agreement with the change in fluorescence intensity (). These results further confirmed that fluorescent compounds were responsible for the reducing power of the derived MRP.
Conclusion
This study evaluated the antioxidant activities of CBPH and its peptide fractions (CBPH-I, <3 kDa and CBPH-II, 3–10 kDa) as affected by the Maillard reaction with galactose. The antioxidant actions of CBPH and its peptide fractions were markedly improved by the Maillard reaction, including DPPH radical scavenging activity, hydroxyl radical scavenging activity, and reducing power. The MRP derived from low-molecular-weight peptides had stronger antioxidant actions (e.g. DPPH radical scavenging activity and reducing power) than that from high-molecular-weight peptides. However, the activities in the MRP derived from high-molecular-weight peptides were improved more than those from low-molecular-weight peptides. It was consistent with the formation of fluorescent compounds, but did not correlate with the glycation extent (i.e. the loss of free amino group) and browning. More fluorescent compounds were generated in the MRP from higher-molecular-weight peptides. Thus, molecular size of the peptides might have an important role in the corresponding MRP antioxidant activity.
Funding
The research was financially supported by National Natural Science Foundation of China (No. 31271887).
Additional information
Funding
References
- Hartmann, R.; Meisel, H. Food-derived Peptides with Biological Activity: From Research to Food Applications. Current Opinion in Biotechnology 2007, 18, 163–169.
- Sarmadi, B.H.; Ismail, A. Antioxidative Peptides from Food Proteins: A Review. Peptides 2010, 31, 1949–1956.
- Carrasco-Castilla, J.; Hernández-Álvarez, A.J.; Jiménez-Martínez, C.; Jacinto-Hernández, C.; Alaiz, M.; Girón-Calle, J; Vioque, J; Dávila-Ortiz, G. Antioxidant and Metal Chelating Activities of Peptide Fractions from Phaseolin and Bean Protein Hydrolysates. Food Chemistry 2012, 135, 1789–1795.
- Jamdar, S.N.; Rajalakshmi, V.; Pednekar, M.D.; Juan, F.; Yardi, V.; Sharma, A. Influence of Degree of Hydrolysis on Functional Properties, Antioxidant Activity and ACE Inhibitory Activity of Peanut Protein Hydrolysate. Food Chemistry 2010, 121, 178–184.
- Sun, Y.; Pan, D.; Guo, Y.; Li, J. Purification of Chicken Breast Protein Hydrolysate and Analysis of its Antioxidant Activity. Food and Chemical Toxicology 2010, 50, 3397–3404.
- Kumar, N.S.S.; Nazeer, R.A.; Jaiganesh, R. Purification and Identification of Antioxidant Peptides from the Skin Protein Hydrolysate of Two Marine fishes, Horse Mackerel (Magalaspis cordyla) and Croaker (Otolithes ruber). Amino Acids 2012, 42, 1641–1649.
- Peng, X.; Xiong, Y.L.; Kong, B. Antioxidant Activity of Peptide Fractions from Whey Protein Hydrolysates as Measured by Electron Spin Resonance. Food Chemistry 2009, 113, 196–201.
- Gu, F.L.; Kim, J.M.; Abbas, S.; Zhang, X.M.; Xia, S.Q.; Chen, Z.X. Structure and Antioxidant Activity of High Molecular Weight Maillard Reaction Products from Casein-glucose. Food Chemistry 2010, 120, 505–511.
- Hwang, G.; Kim, H.Y.; Woo, K.S.; Lee, J.; Jeong, H.S. Biological Activities of Maillard Reaction Products (MRP) in a Sugar-amino Acid Model System. Food Chemistry 2011, 126, 221–227.
- Vhangani, L.N.; Wyk, J.V. Antioxidant Activity of Maillard Reaction Products (MRP) Derived from Fructose–lysine and Ribose–lysine Model Systems. Food Chemistry 2013, 137, 92–98.
- Jiang, Z.; Wang, L.; Wu, W.; Wang, Y. Biological Activities and Physicochemical Properties of Maillard Reaction Products in Sugar–bovine Casein Peptide Model Systems. Food Chemistry 2013, 141, 3837–3845.
- Liu, P.; Huang, M.; Song, S.; Hayat, K.; Zhang, X.; Xia, S.; Jia, C. Sensory Characteristics and Antioxidant Activities of Maillard Reaction Products from Soy Protein Hydrolysates with Different Molecular Weight Distribution. Food Bioprocess Technology 2012, 5, 1775–1789.
- Onuh, J.O.; Girgih, A.T.; Aluko, R.E.; Aliani, M. In Vitro Antioxidant Properties of Chicken Skin Enzymatic Protein Hydrolysates and Membrane Fractions. Food Chemistry 2014, 150, 366–373.
- Lertittikul, W.; Benjakul, S.; Tanaka, M. Characteristics and Antioxidative Activity of Maillard Reaction Products from A Porcine Plasma Protein–glucose Model System as Influenced by Ph. Food Chemistry 2007, 100, 669–677.
- Su, G.; Zheng, L.; Cui, C.; Yang, B.; Ren, J.; Zhao, J. Characterization of Antioxidant Activity and Volatile Compounds of Maillard Reaction Products Derived from Different Peptide Fractions of Peanut Hydrolysate. Food Research International 2011, 44, 3250–3258.
- Kim, S.Y.; Je, J.Y.; Kim, S.K. Purification and Characterization of Antioxidant Peptide from Hoki (Johnius belengerii) Frame Protein by Gastrointestinal Digestion. The Journal of Nutritional Biochemistry 2007, 18, 31–38.
- Wang, J.Z.; Yue, J.; Zhang, C.; Jia, W.; Li, X.; Sun, Z. Preparation of Peptone from Chicken Bone Residue by Using Natural Pancreas as Catalyst. Journal of Chemical Technology and Biotechnology 2016, 91, 2852–2861.
- Xu, D.; Gong, Y.D.; Shen, Y.F.; Nie, X.H. Effect of Temperature on Spectral Characteristics and Antioxidation of Maillard Reaction Products Derived from Chicken Bone Protein Hydrolysate. Food and Fermentation Industries (Chinese) 2015, 41, 126–129.
- AOAC. Official Methods of Analysis of AOAC International (17th); Chemists AOAC: Gaithersburg (MD), 2000.
- Laroque, D.; Inisan, C.; Berger, C.; Vouland, É.; Dufossé, L.; Guérard, F. Kinetic Study on the Maillard Reaction. Consideration of Sugar Reactivity. Food Chemistry 2008, 111, 1032–1042.
- Matiacevich, S.B.; Buera, M.P. A Critical Evaluation of Fluorescence as A Potential Marker for the Maillard Reaction. Food Chemistry 2006, 95, 423–430.
- Benjakul, S.; Lertittikul, W.; Bauer, F. Antioxidant Activity of Maillard Reaction Products from A Porcine Plasma Protein–sugar Model System. Food Chemistry 2005, 93, 189–196.
- Ajibola, C.F.; Fashakin, J.B.; Fagbemi, T.N.; Aluko, R.E. Effect of Peptide Size on Antioxidant Properties of African Yam Bean Seed (Sphenostylis stenocarpa) Protein Hydrolysate Fractions. International Journal of Molecular Sciences 2011, 12, 6685–6702.
- Morales, F.J.; Jiménez-Pérez, S. Free Radical Scavenging Capacity of Maillard Reaction Products as Related to Colour and Fluorescence. Food Chemistry 2001, 72, 119–125.
- Yoshimura, Y.; Iijima, T.; Watanabe, T.; Nakazawa, H. Antioxidative Effect of Maillard Reaction Products Using Glucose-glycine Model System. Journal of Agricultural and Food Chemistry 1997, 45, 4106–4109.
- Jamdar, S.N.; Rajalakshmi, V.; Sharma, A. Antioxidant and ACE Inhibitory Properties of Poultry Viscera Protein Hydrolysate and its Peptide Fractions. Journal of Food Biochemistry 2012, 36, 494–501.
- Girgih, A.T.; Udenigwe, C.C.; Aluko, R.E. In Vitro Antioxidant Properties of Hemp Seed (Cannabis satival) Protein Hydrolysate Fractions. Journal of the American Oil Chemists’ Society 2011, 88, 381–389.
- Shizuuchi, S.; Hayase, F. Antioxidative Activity of the Blue Pigment Formed in A D- Xylose- glycine Reaction System. Bioscience. Biotechnology, and Biochemistry 2003, 67, 54–59.
- Eichner, K.; Antioxidant Effect of Maillard Reaction Intermediates. Progress in Food and Nutrition Science 1981, 5, 441–451.