ABSTRACT
Polyphenolic and mineral profiles of berries and leaves of V. myrtillus (bilberry) and V. uliginosum (bog bilberry) collected from northwestern Macedonia were examined in this study. Overall, 70 polyphenolic compounds were identified from various classes. Laricitrin-3-O-pentoside, trans- and cis-piceid, hexahydroxydiphenoyl-galoyl-glucose, and feruloyl-acetyl glucoside and coumaroyl glucoside of kaempferol were identified for Vaccinium genus. Bilberries had higher total phenolic content than bog bilberry. Major differences in the polyphenolic pattern of berries were found in the flavonols content, whereas leaves differed mostly in the procyanidin content. Berries and leaves of bilberry had higher K and P content compared with bog bilberry and similar contents for Ca, Mg, Mn, and Al, which were comparable to the data for other regions, whereas the measured levels of Zn, Fe, and Cu were higher than the ones reported for bilberries from other regions.
Introduction
European bilberry (Vaccinium myrtillus L.) and bog bilberry (Vaccinium uliginosum L.) belong to the Vaccinium genus, Ericaceae family. Vaccinium species are low-growing, perennial herbs found in the temperate climate zones. They are the most important wild berry plants in the forests of the North Eurasia and North America Mountains. They thrive in damp acid soils, damp woods, and sandy and rocky soils, and cover vast areas. The popularity of bilberry can be ascribed to its balanced sweet–sour taste and well-known nutritional value.[Citation1] Bilberries collected from wild areas are mainly consumed locally as fresh or processed to different products (jams, syrups, juices, alcoholic liqueur, teas, and sweets). They contain high amounts of sugars (240–600 mmol/kg) and organic acids (58–143 mmol/kg)[Citation2] as well as an assortment of phenolic compounds, which have multiple biological effects. In vitro studies indicate the anti-carcinogenic, anti-inflammatory, and antimicrobial activities.[Citation3] Berries are also widely recognized for their various health-promoting properties including a reduced risk of cancer and cardiovascular disease.[Citation4,Citation5] Various biological activities of plant-derived products are associated with plant phenolic compounds, which is the main reason for the extensive research on their abundance and chemical structures nowadays. Phenolic compounds in berry extracts were shown to have a strong capacity to scavenge oxygen radical species and to inhibit the growth of pathogenic bacteria.[Citation6–Citation11]
Teas from bilberry leaves have traditional usage in European countries in diabetes as well as in urinary diseases due to their astringent and antiseptic properties. Antibacterial, anti-inflammatory, hypoglycaemic, and hypolipidemic activities are also associated with bilberry leaves.[Citation12] Many phenolic compounds have been identified in bilberries and differences in their phenolic profiles have been observed and linked to numerous factors. Anthocyanins represent the most important group in bilberries, contributing to their attractive purple-blue colour hues but also defining quality traits.[Citation13,Citation14] The level of anthocyanins varies with cultivar type, season, degree of ripeness, and growing conditions;[Citation15] therefore, reported literature data are quite diverse. Other groups of phenolics identified in bilberries are flavonols, flavan-3-ols, and hydroxycinnamic acids.[Citation8,Citation9,Citation13,Citation16]
A proper consumption of minerals is another very important part of the human diet.[Citation17] Some of them present as trace elements (microelements) and are essential for the physiological equilibrium of the organism. The human body does not make minerals and, to meet the daily needs, minerals must be obtained through the diet, which implies the need of having data on the mineral composition of food products, especially the ones considered as health beneficial.
As a part of the current interest in the nutritional and nutraceutical properties of locally produced berries, the aim of this article was to determine the nature and content of polyphenols and minerals (macro- and microelements) of wild bilberries (berries and leaves) grown in Macedonia and compare the composition of local bilberry and bog bilberry to the ones from other regions in the world. This is the first study on the polyphenolic composition of European bilberry and bog bilberry collected from high mountain pastures from the region of Macedonia and the first report of the polyphenolic profile of leaf extracts of bog bilberry. Since wild bilberry is considered a product with high health-promoting potential for consumers, a detailed insight into the nature and content of polyphenols and minerals is necessary for further studies of its potential exploitation.
Materials and methods
Reagents and chemicals
Formic acid, methanol, water, nitric acid (trace pure), hydrogen peroxide, p.a. were purchased from Merck (Darmstadt, Germany). Acetone and acetic acid of analytical grade were purchased from Alkaloid (Skopje, R. Macedonia). Standard of malvidin-3-glucoside chloride was purchased from Phytolab (Vestenbergsgreuth, Germany); (–)-epicatechin, rutin, quercetin, and resveratrol were from Sigma (Darmstadt, Germany); p-coumaric acid, ferulic acid, and caffeic acid were from Genay (Lyon, France). Trans-piceid was from Phytolab (Vestenbergsgreuth, Germany). Standard solutions of metals were prepared by dilution 11355-ICP multi Element Standard IV (Merck, Darmstadt, Germany).
Plant material
Fresh leaves and ripe berries of bilberry (V. myrtillus) and bog bilberry (V. uliginosum) were analysed for the polyphenols and mineral composition. The berries were harvested at commercial ripeness, specifically when 80% of the surface was red, which corresponds to stage 5 in terms of commercial criterion. They have been collected in August 2015 on Shara Mtn., Peak Popova Šapka (1780 m). Plant samples (approximately 100 mature berries of moderate size and 500 g leaf samples) of bilberry and bog bilberry were sampled according to their abundance and biomass (10–20 replicates from each sampling point). Plant identity (five replicates of both species) was verified by Academician Vlado MatevskiFootnote1 at the Department of botany (Institute of biology, Faculty of Natural Sciences and Mathematics, Skopje). Voucher specimens (VM-15 and VU-15) were deposited at the Institute of Chemistry, Faculty of Natural Sciences and Mathematics, Skopje, R. Macedonia.
For polyphenolic compounds analysis, berries were frozen and stored at –80 °C until analysis, whereas leaves were air dried to a constant weight at room temperature (25 °C) for 7–14 days. For mineral content analysis, all plant samples were washed carefully with redistilled water to remove soil particles. The plant material was also air dried to a constant weight (25 °C, for 7–14 days).
Extraction of fruit samples for polyphenols content analysis
Extracts of phenolic compounds were prepared by modifying the method described by Kajdžanoska et al.[Citation18] Precisely weight amount of around 5 g of frozen material was homogenized in 50 mL of ice-cold deoxygenated methanol that had previously been flushed for a few minutes with nitrogen and extracted with 20 mL of extraction solvent mixture containing acetone and acetic acid (99:1, v/v) (Ika Laborteknik, Staufen, Germany). The extracts were sonicated for 15 min (ultrasonic bath Branson 3510, USA) and centrifuged for 15 min at 3000 RCF at +4 °C. The residue was then re-extracted until the solution became colourless (total volume was approximately 100 mL). The supernatants were concentrated in a rotary evaporator (Ika, RV 10, Wilmington, USA) at low pressure (70 mbar) and temperature below 37 °C. The volume of the extract (aqueous residue) was made up to 10 mL with 20% methanol in water, and it was filtered through a 0.45 μm pore-size filter (CHROMAL Xtra RC-45/13, Germany) before analysis. Extracts were prepared in triplicate.
Extraction of leaf samples for polyphenols content analysis
Dried leaves were homogenized (Ika Laborteknik, Staufen, Germany) for 30 s. The powders obtained were collected for phenolics extraction. Precisely weighed amount of around 1 g of powdered material (three replicates from each sample) was processed. The extraction procedure for sample preparation was performed with 15 mL of 70% (v/v) methanol, using ultrasonic bath (Branson model 3510, USA) for 30 min and centrifuged for 15 min at 3000 RCF. The supernatant was filtered through a 0.45 mm pore-size filter (CHROMAL Xtra RC-45/13, Germany) before analysis.
Plant extract preparation for mineral content analysis
Plant samples – homogenized berries and dried leaves (0.5 g) – were placed in Teflon digestion vessels. Around 7 mL HNO3 (69%) and 2 mL H2O2 (30%) were added, and the vessels were capped closed, tightened, and placed in the rotor of the Mars microwave digestion system (CEM, Matthews, North Carolina, USA). The digestion was carried out following the digestion program: (1) temperature 180 °C, 10 min ramp time, 1400 W; (2) temperature 180 °C, 5 min hold time, 1400 W. Finally, the vessels were cooled, carefully opened, and digests quantitatively transferred to 25 mL calibrated flasks and filled to volume with redistilled water.
LC/DAD/ESI-Msn analysis
Chromatographic separations were carried out on a 150 mm x 4.6 mm, 5 µm, ZORBAX SB-C18 column (Agilent Technologies, Germany). The mobile phase consisted of water–formic acid (5%, v/v) (A) and methanol (B) for the berry extracts and water–formic acid (1%, v/v) (A) and methanol (B) for the leaf extracts. Two gradient elution methods were optimized for separation: method 1 (0–5 min, 5% B; 5–45 min, 5–80% B; 45–60 min, 80–100% B and 60–65 min, 100% B) for berry extracts and method 2 (0–5 min, 20% B; 5–10 min, 20–35% B; 10–20 min, 35% B; 20–30 min, 35–50% B; 30–45 min, 50–80% B; 45–55 min, 80–100% B and 55–65 min, 100% B) for leaf extracts. The flow rate used in both methods was 0.4 mL/min. The injection volume was 10 µL and 5 µL for berry and leaf extracts, respectively.
The HPLC system was equipped with an Agilent 1100 series diode array detector and an ion trap mass detector in series (Agilent Technologies, Waldbronn, Germany). It consisted of a G1312A binary pump, a G1329A autosampler, a G1379B degasser, and a G1315D photodiode array detector, controlled by a ChemStation software (Agilent, v.08.03). Spectral data from all peaks were accumulated in the range 190–600 nm and chromatograms were recorded at 280 nm for procyanidins and cinchonais, at 300 nm for stilbenes, at 320 nm for hydroxycinnamic acid derivatives and coumaroyl iridoids, at 360 nm for flavonols, and at 520 nm for anthocyanins.
The mass detector was a G2449A Ion-Trap Mass Spectrometer equipped with an electrospray ionization (ESI) system and controlled by LCMSD software (Agilent, v.6.1.). Nitrogen was used as the nebulizing gas at a pressure of 65 psi and the flow was adjusted to 12 L/min. The heated capillary and the voltage were maintained at 325 °C and 4 kV, respectively. The parameters for capillary exit offset, skimmer 1, and skimmer 2 were 100 V, 40 V, and 6V, respectively, and compound stability was 100%. MS data were acquired in the positive and negative ionization modes. The full scan covered the mass range at m/z 50–1200.
Identification and quantification of polyphenolic compounds
Liquid chromatography with diode-array detection (LC–DAD) was used for separation and quantification. Peak assignment of the various classes of polyphenols in the chromatograms was based on the comparison of their retention behaviour and UV–Vis spectra to those of the authentic compounds and literature data. The conjugated forms of polyphenolic compounds were further characterized by electrospray ionization mass spectrometric detection in the positive ionization mode for anthocyanins and in the negative ionization mode for flavonols, phenolic acids, coumaroyl iridoids, flavan-3-ols, cinchonais, and stilbenes.
Quantification was performed directly by HPLC/DAD using five-point regression curves (R2 ≥ 0.999) of authentic standards. Flavonols were determined at 360 nm using quercetin 3-O-rutinoside (rutin); phenolic acids and coumaroyl iridoids were determined at 320 nm using caffeic acid; proanthocyanidin derivatives and cinchonains were determined at 280 nm using (–)-epicatehin; anthocyanins were quantified with malvidin 3-O-glucoside chloride at 520 nm; and piceid derivatives were quantified using piceid as an external standard at 300 nm. In case of overlapping peaks in the DAD-chromatograms, separate quantitation was possible with the help of the extracted ion chromatograms (EICs) at the m/z values of the corresponding molecular ions of each overlapping compound: the EIC integral value was used to estimate the contribution of each individual overlapping compound to the joint DAD peaks. Total content is the sum of contents of all identified phenolic compounds.
Analysis with inductively coupled plasma atomic emission spectroscopy
Bilberry samples were digested by the microwave digestion system Mars (CEM, Matthews, North Carolina, USA) using nitric acid and hydrogen peroxide. From the obtained solution Ag, Al, As, Ba, Ca, Cd, Co, Cr, Cu, Fe, K, Li, Mg, Mn, Mo, Na, Ni, P, Pb, Sr, Tl, V, and Zn were analysed by atomic emission spectrometry with inductively coupled plasma atomic emission spectroscopy ICP-AES (Varian, 715-ES, Pocasset, USA) using ultrasonic nebulizer CETAC (ICP/U-5000AT+) for better sensitivity. Out of the 23 analysed, the contents of seven elements (As, Cd, Co, Mo, Pb, V, and Tl) were below limit of detection (LOD).
Statistical analysis
Statistical treatment including calculations of means and standard deviations was performed applying Excel (Microsoft Office, 2010). Samples were analysed in triplicate, and one-way analysis of variance (ANOVA) was performed using STATISTICA, version 7. Differences at p < 0.5.
Results and discussion
The LC–DAD chromatograms obtained for berries of bilberry and bog bilberry are presented in (peak assignments as in ). The corresponding chromatograms obtained for leaves samples are presented in (peak assignments as in ). The peaks, i.e. polyphenolic compounds, detected in all studied samples were classified into the following groups: anthocyanins, phenolic acid and derivatives, flavonols, coumaroyl iridoids and stilbenes for fruit extracts, and phenolic acid and derivatives, flavonols, flavan-3-ols, coumaroyl iridoids, and cinchonains for leaf extracts.
Table 1. Retention times, UV–Vis, mass spectral data, and contents of phenolic compounds in bilberry and bog bilberry.
Table 2. Retention times, UV–Vis, mass spectral data, and contents of phenolic compounds (mg/100 g DW) in bilberry and bog bilberry leaf extracts.
Figure 1. HPLC/DAD chromatograms of berry extracts of (1) bilberry (in red) and (2) bog bilberry (in green): (a) 520 nm, (b) 360 nm, (c) 300 nm.
A1–A17 for anthocyanins, Ac1–Ac14 for hydroxycinnamic acid derivatives, F1–F15 for flavonols, S1–S2 for stilbenes, and I1 for iridoid. Peak assignments as in .
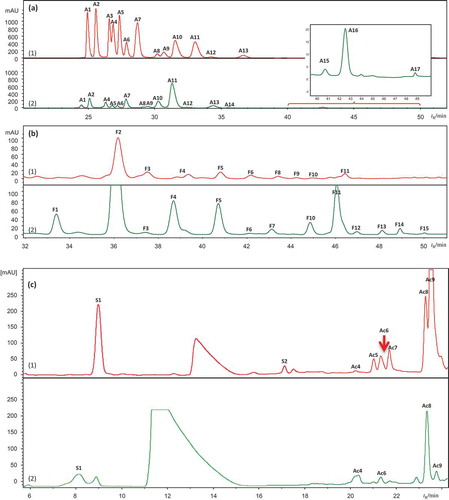
Figure 2. HPLC/DAD chromatograms of leaf extract of (1) bilberry (in red) and (2) bog bilberry (in green): (a) 280 nm, (b) 360 nm.
Ac1–Ac13 for hydroxycinnamic acid derivatives, F1–F15 for flavonols, FL1–FL5 for flavan-3-ols, S1–S2 for stilbenes, I1–I2 for iridoids, and C for cinchonain. Peak assignments as in
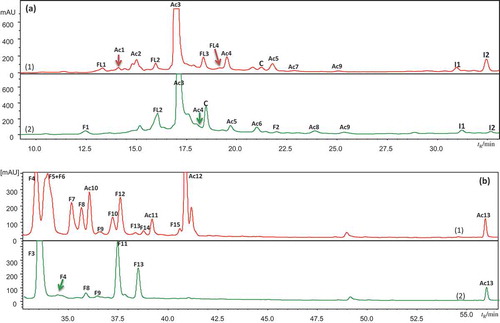
In total, 74 different compounds were identified and only seven compounds – 5-caffeoylquinic acid, feruloylquinic acid, coumaroyliridoid isomer, gallic acid derivative, and several flavonols (quercetin-3-O-glucoside, quercetin-3-O-rutinoside, kaempferol 3-O-glucoside) – were detected in berries and leaves of both species. The total phenolic content for berries (TPC) (mg/100 g FW) was 706 ± 1 for bilberry and 393 ± 1 for bog bilberry. Anthocyanins were the dominant phenolic group (72% for bilberry, 39% for bog bilberry), followed by phenolic acids and derivatives (20% for bilberry, 47% for bog bilberry), whereas flavonoids were a minor group (2% for bilberry, 13% for bog bilberry) ().
Figure 3. Distribution of the main phenolic classes in (a) berries and (b) leaves of bilberry and bog bilberry.
Ac: acid derivatives; I: iridoids; S: stilbenes; F: flavonols; FL: flavan-3-ols; A: anthocyanins; Ci: cinchonain; D: delphinidin derivatives; C: cyanidin derivatives; Pt: petunidin derivatives; Pe: peonidin derivatives; M: malvidin derivatives; Q: quercetin derivatives; My: myricetin derivatives; L: laricitrin derivatives; Sy: syringetin derivatives; K: kaempferol derivatives; Is: isorhamnetin derivatives; Vm: Vaccinium myrtillus – bilberry; Vu: Vaccinium uliginosum – bog bilberryx axis – contents of phenolic compounds, anthocyanins, and flavonols expressed as mg/100 g FW in berries and as mg/100 g DW in leaves
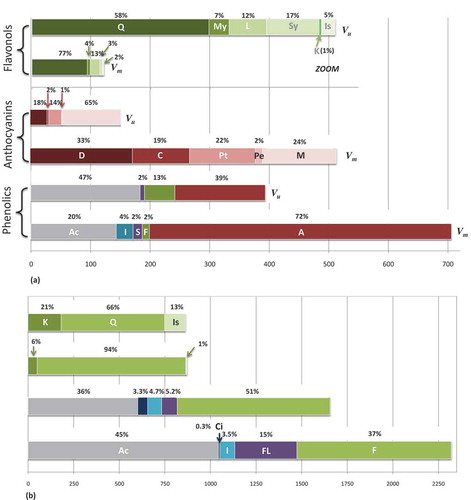
The results obtained for our samples were higher compared with those for bilberries from Montenegro,[Citation19] where the TPC (mgGAE/100 g FW) ranged from 392 to 520, but was lower compared with the TPC found in bilberries from Serbia (890 ± 9).[Citation20,Citation21] These three regions (Macedonia, Serbia, and Montenegro) are very close and we suppose that the difference in TPC may be due to the altitude and sunlight. It has been reported earlier that light intensity, photoperiod, and temperature influence the biosynthesis of many secondary metabolites.[Citation22] Jaakola et al.[Citation23] found that the concentration of anthocyanins, catechins, flavonols, and hydroxycinnamic acids were higher in bilberry leaves exposed to direct sunlight. It was also noticed that at altitude higher than 1500 m, higher amounts of total phenolics were observed.
TPC for bog bilberry is generally lower compared with those found in bilberry. The TPC (mgGAE/100 g FW) for bog bilberry from Finland[Citation24] was 161 ± 1 and from Turkey it was 679 ± 5.[Citation9]
The TPC (mg/100 g DW) of leaf extracts was 2320 ± 1 and 1656 ± 1 for bilberry and bog bilberry, respectively. The total content of phenolic acids and derivatives (mg/100 g DW) was higher and present 1044 ± 3 for bilberry and 601 ± 1 for bog bilberry and the relative amounts of phenolic acids and derivatives, flavonols, flavan-3-ols, iridoids, and cinchonain were 45%, 36.5%, 14.7%, 3.5%, and 0.3% for bilberry and 36%, 51%, 5.2%, 4.6%, and 3.3% for bog bilberry leaf extracts, respectively ().
Identification and quantification of polyphenolic compounds in berries
Anthocyanins
As previously reported,[Citation13] anthocyanins are the major polyphenolic class that characterizes genus Vaccinium. In our study, 13 and 16 different anthocyanins were found in the extracts of bilberry and bog bilberry, respectively. From the MS data, five anthocyanidins were detected. The [Y0]+ ions at m/z 303, 287, 317, 301, and 331 indicated delphinidin (aglycone of compounds A1, A2, A4, A14, and A15), cyanidin (A3 and A5), petunidin (A6, A7, A9, and A17), peonidin (A8 and A12), and malvidin (A10, A11, A13, and A16), respectively. Among these, compound A5 was identified as cyanidin 3-O-glucoside by comparison with an authentic reference standard.
L-galactose and D-glucose have been found as the most common sugars linked to flavonoids in wild-growing and cultivated species of the genus Vaccinium, whereas arabinose is the most common pentose.[Citation25] According to previous reports,[Citation26] xylose is the second most common pentose linked to flavonoids in Vaccinium plants, and its glycosides are eluted before the ones of arabinose. Therefore, compounds A4, A9, A12, and A13 were tentatively identified as delphinidin, petunidin, peonidin, and malvidin-3-O-arabinoside, and A15 and A16 as delphinidin and malvidin-3-O-xyloside, respectively. In general, glycosylation usually occurs at hydroxyl groups in positions 3- and 5- in anthocyanidins.[Citation27] Compound A17 with a molecular ion at m/z 625 and neutral loss of 308 giving the aglycone ion at m/z 317 indicated the presence of petunidin-3-O-rutinoside.
Compounds A1–A13 have previously been found in both bilberry and bog bilberry.[Citation13] In our samples, only cyanidin-3-O-galactoside (A3) was not found in bog bilberry. Malvidin-3-O-xyloside (A16) was found only in bog bilberry extracts, which is in accordance with published data,[Citation16,Citation28] and this compound is a marker for bog bilberry. Delphinidin (A14), delphinidin-3-O-xyloside (A16), and petunidin-3-O-rutinoside (A17) were found only in the extracts of bog bilberry. Only A14 has previously been found in bilberries from Slovenia,[Citation29] whereas A16 and A17 have not been previously reported in bilberry and bog bilberry. Delphinidin-3-O-xyloside (A16) previously was found in Caucasian blueberry (V. arctostaphylos) from Iran[Citation8] and petunidin-3-O-rutinoside (A17) was reported in berry extracts of Madeira blueberry (V. padifolium) from Finland.[Citation13]
The total amount of anthocyanins (mg/100 g FW) in berries was 507 ± 1 for bilberry and 152 ± 1 for bog bilberry. The total anthocyanins content in bilberries (507 ± 1) was lower compared with those found in bilberries from Slovenia (1210 ± 111),[Citation29] but in the same range with bilberries from Finland (average value of 411).[Citation30] The content of anthocyanins in bog bilberries (152 ± 1) is in the same range as the published values for bog bilberries from Finland[Citation6,Citation28] and slightly lower than those found in bog bilberries from Turkey.[Citation9] A previous study demonstrated that higher light intensity seemed to be a more important factor stimulating higher production of phenolics than the soil nutrient status.[Citation31]
Petunidin-3-O-glucoside (A7) was the most abundant anthocyanin for bilberry (16% of total anthocyanins), whereas malvidin-3-O-glucoside (A11) was the most abundant anthocyanin for bog bilberry (49% of total anthocyanins).The proportions of the different anthocyanins in bilberries were very similar for four of them: delphinidin (33%), malvidin (24%), cyanidin (22%), and petunidin (22%), and peonidin (2%) had a very low relative amount (). In published data for Slovenian bilberries,[Citation29] delphinidin and cyanidin glycosides were found as dominant (>70%), as also recently reported for Finnish bilberries,[Citation30] whereas glycosides of malvidin were dominant in blueberries cultivated in Slovenia.[Citation29] In blueberries from Canada and the United States, for instance, delphinidin glycosides were predominant.[Citation24] In both Slovenian berries,[Citation29] peonidin glycosides content was the lowest and the same was reported for blueberries grown in Finland.[Citation30]
The proportions of the major anthocyanins in bog bilberries were 65% malvidin, 18% delphinidin, 13% petunidin, and 2% and 1% for the less-abundant cyanidin and peonidin (). These results follow a similar distribution pattern as reported for bog bilberries from Nordic countries (41% malvidin, 28% delphinidin, 19% petunidin, 7% cyanidin, and 2% peonidin),[Citation32] but higher proportion of delphinidin (48%) and lower values of malvidin (15%) were reported for populations of the northwest United States.[Citation24] The proportions of malvidin, delphinidin, petunidin, cyanidin, and peonidin type in Turkish samples of bog bilberry were 46%, 13%, 13%, 21%, and 7%, respectively.[Citation9]
Phenolic acids and derivatives
Phenolic acids and derivatives were the second major phenolic class in berry extracts. Compounds Ac1 and Ac9 were identified as quinic acid and 5-caffeoylquinic acid, respectively, while Ac5 was identified as the feruloylquinic acid isomer.[Citation33,Citation34] Analogously, compounds Ac4, Ac6, and Ac8 were tentatively identified as caffeoyl derivatives due to their fragment ion at m/z 179 (caffeoyl moiety). Based on UV–Vis absorption and mass spectra, compounds Ac3 and Ac7 were identified as protocatechuic acid 4-O-β-hexoside with [M–H]— at m/z 315 and hydroxyferuloylglucose with [M–H]— at m/z 371. Ac2 was identified as gallic acid, compared to reference, whereas Ac10, Ac11, and Ac12, according to their UV–Vis spectra, were characterized as gallic acid derivatives. The phenolic acids content (mg/100 g FW) was 143 ± 1 for bilberry and 183.2 ± 0.3 for bog bilberry with no substantial differences in their content (, ). In both samples, gallic acid derivatives (Ac11 and Ac12) were the most abundant, ranging from 70–80% of the total phenolic acids and derivatives content.
The content of individual identified compounds (Ac1–Ac9) (mg/100 g FW) ranged from 19 to 50 for both species. As reported,[Citation29,Citation35] 5-caffeoylquinic acid (Ac9) was the most abundant hydroxycinnamic acid in bilberries (6.5% of the total phenolic acid content), whereas its content was only 0.3% for bog bilberry. In contrast, the content of quinic acid (Ac1) was much higher in bog bilberry (24% of the total phenolic acids content). The content of hydroxycinnamic acids (A4–A9), together with quinic acid, gallic acid, and protocatehuic acid 4-O-β-hexoside, was in accordance with previously published data.[Citation29,Citation35]
Flavonols
Fifteen different flavonols (nine in bilberry and 13 in bog bilberry) were identified according to UV–Vis absorption and MS data (). The [Y0]—ions at m/z 317, 301, 331, 315, 345, and 285 indicated six aglycones with the corresponding molecular ions: myricetin (aglycone of F1 and F6), quercetin (F2, F3, F5, F9, and F15), laricitrin (F4, F7, and F8), isorhamnetin (F10 and F14), syringetin (F11 and F12), and kaempferol (F13). F3 was identified as quercetin-3-O-rutinoside by comparison with a standard. The particular hexose moieties from these flavonol glycosides could not be confirmed based on MS information only, whereas, according to previous reports, arabinose was the main pentose linked to flavonoids in Vaccinium plants.[Citation28] Compounds F2, F4, F10, F11, F12, and F13 were tentatively identified by comparison to literature data.
Quercetin-3-O-glucoside (F2), quercetin-3-O-rutinoside (rutin) (F3), myricetin (F6), laricitrin (F8), isorhamnetin-3-O-glucoside (F10), syringetin-3-O-glucoside (F11), and quercetin (F15) were previously found in both analysed bilberries.[Citation13] In our extracts, laricitrin (F8) was found only in bilberry, whereas quercetin (F15) was found in bog bilberry. F1 was tentatively identified as myricetin pentoside and it was found only in bog bilberry. There are data for the presence of myricetin-3-O-xyloside in bilberry,[Citation33] whereas myricetin-3-O-arabinoside has been reported in bog bilberry.[Citation16]
Quercetin-3-O-arabinoside (F5), quercetin-3-O-rhamnoside (F9), syringetin-3-O-galactoside (F12), and kaempferol-3-О-glucoside (F13) have previously been found in bilberries.[Citation29,Citation33,Citation34,Citation36] In our study, F5 was found in both analysed bilberries, whereas F9 was found only in bilberry, and F12 and F13 only in bog bilberry.
Laricitrin-3-O-galactoside (F4) was found in both bilberry extracts and it has previously been reported only in bog bilberry from Finland.[Citation28] Laricitrin-3-O-pentoside (F7) was found only in the extracts of bog bilberry. This compound has previously been reported in bilberry and highbush blueberry (V. corymbosum) from Slovenia.[Citation32] Compound F14, tentatively identified as isorhamnetin pentoside, was detected only in the extracts of bog bilberry. There are literature data for the presence of isorhamnetin 3-O-xyloside only in bilberry extracts.[Citation36]
The content of flavonols (mg/100 g FW) measured in the studied species was 12.1 ± 0.4 for bilberry and 51.0 ± 0.4 for bog bilberry, representing only 1.7% and 13% of TPC for bilberry and bog bilberry, respectively (). The total flavonol contents of the studied berries were lower compared to reported data.[Citation6] The average reported flavonol content was 154 ± 40 for bog bilberries from Finland[Citation30] and 93 ± 4 for the ones from China.[Citation16] The average flavonol content for bilberries from Slovenia was 114 ± 6.[Citation32] On the other hand, this total flavonol content in wild species from Macedonia is significantly higher than those reported for cultivated berries (average content 4.2 ± 0.8).[Citation35] The main flavonol in bilberries was quercetin 3-O-rutinoside (F3) (18% of the total flavonols). Quercetin 3-O-glucoside (F2) was the most abundant in bog bilberries (48% of the total flavonols), which together with laricitrin-3-O-galactoside (F4) (11%), quercetin-3-O-arabinoside (F5) (9%), and syringetin-3-O-glucoside (F11) (17%) contributed to 85% of the total flavonols content.
The proportions of quercetin glycosides were 76% and 58% for bilberry and bog bilberry, respectively (). Quercetin glycosides have been reported to be more abundant than myricetin glycosides in Chinese bog bilberries[Citation16] and in Slovenian bilberries.[Citation32] In contrast, bog bilberries of the northwestern United States[Citation24] contained more myricetin than quercetin. In our samples, quercetin glycosides were the most abundant but they were not followed by myricetin glycosides. The proportions for laricitrin, syringetin, myricetin, and isorhamnetin glycosides were 13%, 3%, 4%, and 2% for bilberry and 12%, 17%, 6%, and 4% for bog bilberry, respectively.
Iridoids
According to its UV–Vis spectrum and fragmentation pattern with the typical ion at m/z 163 compound I1, previously reported,[Citation33] was tentatively identified as a coumaroyl iridoid isomer. It was found only in bilberry extracts, and it contributed with 4% to TPC.
Stilbenes
There are not many reports on stilbenes in bilberries and bog bilberries except the ones reporting the presence of trans-resveratrol in Slovenian bilberries,[Citation29] and in other berries such as cowberries, cranberries, strawberries, and red currants.[Citation37] Resveratrol, pterostilbene, and piceatannol have been found in other Vaccinium species.[Citation38] However, there are no available data for the presence of piceid (cis- and trans-) in Vaccinium representatives. Based on the characteristic UV–Vis and mass spectra, two compounds were identified as trans- and cis-piceid (S1 and S2) with [M–H]— at m/z 389 and MS2 spectra of these ions yielded a base peak [M–H–162]— corresponding to the loss of glucose. The structure of trans-piceid (S1) was confirmed using an authentic standard.
Trans-piceid was detected in both studied Vaccinium species and the contents (mg/100 g FW) were 14.3 ± 0.4 and 7 ± 2 for bilberry and bog bilberry, respectively. Cis-piceid was detected only in bilberry and its content was 0.9 ± 0.2. The total content of stilbenes (trans- and cis-piceid) was higher compared to previously reported stilbenes in other Vaccinium species, but lower than those found in grape samples, for example. The average content of trans-resveratrol in bilberries from Slovenia[Citation29] was 0.3 mg/100 g FW.
Identification and quantification of polyphenolic compounds in leaf extracts
Phenolic acids and derivatives
Thirteen phenolic acids and derivatives were identified in leaf extracts of the analysed Vaccinium species. Based on its fragmentation pattern in negative ionization mode and comparison to authentic standard, compound (L)Ac3 was identified as chlorogenic acid (5-O-caffeoylquinic acid). (L)Ac5 was identified as 4-caffeoylquinic acid based on its retention order[Citation33] and relative intensities of diagnostic fragments in negative ionization mode.[Citation34] Analogously, (L)Ac4, (L)Ac6, and (L)Ac9 with molecular ions at m/z 337 and characteristic fragmentation patterns explained by Clifford et al.[Citation34] were identified as 3-, 5-, and 4-p-coumaroylquinic acid, respectively. Compounds (L)Ac1 and (L)Ac2 with [M–H]—at m/z 515 were identified as dicaffeoylquinic acid derivatives, whereas (L)Ac7, with [M–H]—at m/z 367 and fragment ion at m/z 191 (quinic acid moiety), was identified as feruloylquinic acid isomer. Besides caffeoylquinic acids (L(Ac3) and L(Ac6)), p-coumaroylquinic acids have been reported in the leaves of bilberry from Northern Finland[Citation33] and one isomer each of p-coumaroylquinic acid and feruloylquinic (L(Ac7)) acid were found in our previous study in the leaves of bilberry from Osogovo Mtn.[Citation39] Compounds (L)Ac10, (L)Ac11, and (L)Ac12 ([M–H]—at m/z 411 and MS2 at m/z 163) were p-coumaric acid derivatives (). (L)Ac13 was tentatively identified as gallic acid derivative and (L)Ac8 as hexahydroxydiphenoyl-galloyl-glucose (HHDP-galloyl-glucose). The latter derivative has not been previously detected in Vaccinium plants, but it was reported in strawberries.[Citation40]
Nowadays, bilberry leaves are considered an important source of chlorogenic acid (3-caffeoylquinic acid) that occurs in nature in other isomeric as 4-caffeoylquinic and 5-caffeoylquinic acid.[Citation34] 5-Caffeoylquinic acid was the dominant phenolic compound in bilberry leaf extracts contributing to 57% and 64% to the total phenolic acid derivatives for bilberry and bog bilberry, respectively, and 28% to TPC for both species. These results correspond well to published data where the content of chlorogenic acid in leaves of bilberry from Poland gave up to 35% of TPC.[Citation41] Compounds (L)Ac10, 11, and 12 were found only in the leaf extracts of bilberry (total content 243 ± 4 mg/100 g DW). Also, two isomers of dicaffeoylquinic acid were measured in the leaf extracts of bilberry contributing to 3% in TPC, which were not detected in bog bilberry.
Flavonols
Differences in leaf flavonol profiles between the two species were also found. In total, 15 flavonols were identified in the leaves, 12 in bilberry, and nine in bog bilberry that were glycosides or acyl-glycosides of quercetin, kaempferol, and isorhamnetin. Compound L(F5) (quercetin 3-O-rutinoside) was identified using a standard and MS data (). From UV and MS data and according to Hokkanen et al.,[Citation33] seven other quercetin derivatives were detected: quercetin-3-O-galactoside (L)F3, quercetin-3-O-glucoside (L)F4, another quercetin hexoside (L)F6, quercetin-3-(6”-acetylglucoside) (L)F7, quercetin-3-O-pentoside (L)F8, and quercetin-3-O and 7-O-rhamnoside, (L)F9 and (L)F12.
(L)F10, (L)F11, (L)F14, and (L)F15 with [M–H]at m/z 447, 461, 489, and 431, respectively, and fragment at m/z 285 corresponding to the aglycone of kaempferol, were identified as kaempferol-3-O-glucoside, kaempferol-3-O-arabinoside, kaempferol-3-(6”-acetylglucoside), and kaempferol-3-O-rhamnoside, respectively, whereas (L)F1 and (L)F2 were tentatively identified as kaempferol feruloyl-acetylglucoside ([M–H]–at m/z 665 and MS2 at m/z 489 and 285) and kaempferol coumaroylglucoside ([M–H]–at m/z 593 and MS2 at m/z 431 and 285), respectively.
Compounds (L)F3-L(F5), L(F8), and L(F9) have previously been reported in the leaves of bilberry and lowbush blueberry,[Citation33,Citation42] whereas L(F4), L(F7), L(F9), and L(F12) were found in our previous study.[Citation39] Kaempferol feruloyl-acetylglucoside (L)F1 and kaempferol coumaroylglucoside (L)F2 () have not been previously reported in Vaccinium species.
The proportion of flavonols in leaves of bilberry (846 ± 4 mg/100 g DW, 37% of TPC) was lower compared to leaves of bog bilberry (838 ± 2 mg/100 g DW, up to 50% of TPC) (). These flavonols contributions to TPC are in accordance with the reported data. The average flavonols content in leaves of bilberry from Finland was 44%[Citation33] and for the representatives from Osogovo Mtn., Macedonia 62%.[Citation39] However, the reported average proportion of flavonols in the wild species of lowbush blueberry leaves was significantly lower (19%).[Citation42]
Kaempferol feruloyl-acetylglucoside (L)F1, kaempferol coumaroylglucoside (L)F2, kaempferol-3-O-arabinoside (L)F11, and quercetin-3-O-galactoside (L)F3 were found only in bog bilberry leaf extract, the latter one contributing 63% to the total flavonols content, whereas kaempferol-3-O-arabinoside (L)F11 contributed 16% to the total flavonols content.
In contrast, quercetin-3-O-rutinoside (L)F5, quercetin-3-O-glucoside (L)F6, quercetin-3-(6”-acetylglucoside) (L)F1, and quercetin rhamnoside (L)F1 were found only in bilberry leaf extract. These four compounds together with quercetin-3-O-glucoside (L)F1 and quercetin-3-O-pentoside (L)F8 present 93% of the total flavonols content in this species.
The contribution of quercetin glycosides to leaf total flavonols was 94% for bilberry and 65% for bog bilberry. The fractions of kaempferol and isorhamnetin glycosides were higher in bog bilberry (21% and 13%, respectively) compared to bilberry (6% and 1%, respectively). These results are in accordance with the literature data, where quercetin glycosides have been reported to be more abundant.[Citation33]
Flavan-3-ols
Compounds (L)FL1, (L)FL3, and (L)FL4 were, respectively, identified as proanthocyanidin dimer B type, catechins, and (epi)catechin according to retention times, UV–Vis and MS data of standards (). (L)FL2 was identified as proanthocyanidin dimer with [M–H]— at m/z 577 and MS2 at m/z 289, whereas (L)FL5 was identified as epicatechin gallate.
All five flavan-3-ols were detected in the leaf extracts of bilberry, and only (L)FL2 was detected in bog bilberry. These compounds are usually found in the berries (even though we didn’t find them in our berry extracts), but there are not much data on their presence in the leaves. In our previous study, we detected only catechin in the leaves of bilberry from Osogovo Mtn.[Citation39] In contrast, Hokkanen et al.[Citation33] found seven different flavan-3-ols in the leaf extracts of bilberry. Catechin and procyanidin B2 have also been reported in the leaves of lowbush blueberry (V. angustifolium).[Citation42]
The biggest difference between the two studied species was found in the procyanidins content (340 ± 2 and 85.8 ± 0.7 mg/100 g DW for bilberry and bog bilberry, respectively), which present 15% and 5% of TPC for bilberry and bog bilberry, respectively. The proportion of flavan-3-ols in bilberry leaves was higher compared to the values found in bilberry leaves from Northern Finland,[Citation33] where the average content of flavan-3-ols was around 4% of TPC. Procyanidin dimers ((L)FL1 and (L)FL2) had the highest contribution of up to 65% of total flavan-3-ols content and 9% of TPC, followed by catechin ((L)FL3) (28% of total flavan-3-ols and 4% of TPC), and epicatechin ((L)FL4) and epicatechin gallate ((L)FL5) (2% and 3% of total flavan-3-ols, and 0.4% and 0.5% of TPC, respectively). Epicatechin has been reported as the dominant flavan-3-ol in leaves of bilberry from Finland (1.75% to TPC).[Citation33] The content of the detected one procyanidin, previously reported in bilberry leaves from Osogovo Mtn.,[Citation39] was 0.21 mg/g DW contributing to 3% to TPC.
Iridoids
The fragmentation spectra of compounds I1 and I2 were identical. The presence of a fragment ion at m/z 163 in negative ion mode suggested a coumaroyl unit, whereas a fragment corresponding to a loss of 44 amu implied carboxylic group in the structure. Loss of a coumaroyl unit from the parent ion produced a fragment ion at m/z 371 and a further loss of 162 amu resulted in the ion at m/z 209 suggesting the presence of hexose moiety in the structure. The ion at m/z 373 corresponds to the loss of a hexose unit directly from the parent ion, implying that the sugar and coumaroyl unit are not linked, but directly attached to the aglycone. Two different iridoid glycosides with the same molecular ions have been detected by Hokkanen et al.[Citation33] in leaves of bilberry and lingonberry. Coumaroyl iridoids have also been reported in cranberry juice.[Citation43] The presence of monotropein in lingonberry and bilberry juice suggests, together with the MS data obtained and interpreted here, that compounds I1 and I2 might be 10-p-trans- and 10-p-cis-coumaroyl-1S-monotropein. This is also supported by the fact that coumaroyl iridoids from cranberry have been identified as 10-p-trans- and 10-p-cis-coumaroyl-1S-dihydromonotropein.[Citation43] 10-p-trans-coumaroyl-1S-monotropein (vaccinoside) has been reported in V. bracteatum and 10-p-cis-coumaroyl-1S-monotropein (andromedoside) has been reported in Andromeda polifolia,[Citation43] but not in bilberry.
The total content of coumaroyl iridoid isomers (mg/100 g DW) was 82.2 ± 0.4 and 76.5 ± 0.8 for bilberry and bog bilberry, respectively, which present 3.5% and 4.6% of TPC. The contribution of these compounds found in this study is higher compared with the literature data for Vaccinium (0.98% of TPC).[Citation33] It was interesting that compound I2 is dominant in bilberry (84% of the total iridoid content and 3% of TPC), whereas compound I1 is dominant in the leaves of bog bilberry (73% of the total iridoid content and 3% of TPC).
Cinchonains
Compound (L)C had molecular ion at m/z 451 and fragment ions at m/z 341, 321, 289, 231, 217, 189, and 177. Its fragmentation pattern has been described by Hokkanen et al.[Citation33] in leaf extracts of bilberry and identified as cinchonain Ix (x = a, b, c or d).
The content of (L)C was 7.0 ± 0.2 and 54 ± 2 mg/100 g DW contributing to 0.3% and 3.3% to TPC for bilberry and bog bilberry, respectively. These results are much lower compared with those of Hokkanen et al.,[Citation33] where the content of cinchonains (six different compounds) constituted up to 18.5% of TPC.
Mineral content of berries and leaves of bilberry and bog bilberry
The mineral content of fruits and vegetables contributes to augment their nutritional value, but at the same time, trace elements can be toxic at concentrations beyond those necessary for their biological functions. Information on the mineral composition is important to assess the benefits of consumption of wild species such as bilberry.[Citation44]
In this investigation, a comprehensive study was made on the content of a certain number of elements in wild bilberry. Besides the phenolic compounds in bilberry, it is also essential to determine the content of minerals, particularly in the berries and leaves that are used and consumed. Four macroelements (Ca, K, P, and Mg) and 12 microelements (Al, Fe, Na, Ag, Ba, Cr, Cu, Li, Mn, Ni, Sr, and Zn) were analysed in this study, performed on dried berry and leaf samples of bilberry and bog bilberry ().
Table 3. Content of macro- and microelements in berries and leaves of bilberry and bog bilberry.
The berries and leaves of bilberry had a higher potassium content (mg/100 g DW) (898 and 657, respectively) compared with the berries and leaves of bog bilberry (614 and 563, respectively). This level is comparable to the K level in bilberry consumed in Finland (680),[Citation45] and higher than the one reported for bilberry from Latvia and Italy (482 and 547, respectively).[Citation46,Citation47]
Berry samples from bilberry also contained a higher level of phosphorus (80.8 mg/100 g DW) compared with bog bilberry (59.9 mg/100 g DW), whereas its content in the leaves of both species was around 63 mg/100 g DW. These values are lower than the ones found in berries of bilberry from Finland, Latvia, and Italy (130, 95, and 117 mg/100 g DW, respectively),[Citation45–Citation47]but comparable to the ones in UK and Mexico (40–150 and 60–190 mg/100 g DW).[Citation48,Citation49]
The content of Ca (mg/100 g DW) in the berry samples of bilberry and bog bilberry was 200 and 174, respectively. These berries contained similar levels of Ca as European berries such as raspberry (160), bilberry, lingonberry, and cranberry (140, 100 and 110 mg/100 g DW, respectively) from Finland,[Citation45] bilberry from Italy (140 mg/100 g DW) (39) and blueberry, bilberry, and red berry (57, 160, and 241 mg/100 g DW, respectively) from Latvia.[Citation47] The leaves of bilberry and bog bilberry were found to be a very rich source of Ca containing above 1300 mg/100 g DW.
The levels of Mg were 80 and 60 mg/100 g DW for bilberry and bog bilberry, respectively (). These levels were similar to or slightly higher than those in bilberries consumed in Finland and Italy (60 and 59.7 mg/100 g DW, respectively).[Citation45,Citation46] Lower levels of Mg were reported for berries consumed in UK and Mexico (20–120 and 30–170 mg/100 g DW, respectively).[Citation48,Citation49] The content of Mg in leaves was very high (266 and 355 mg/100 g DW for bilberry and bog bilberry, respectively), indicating that wild bilberries from Macedonia are a rich source of Mg.
With regard to the Zn content, leaves of bog bilberry (3.27 mg/100 g DW) contained approximately twice as much Zn as raspberry leaves (2.0 mg/100 g DW), identified as the richest source of Zn among berries grown in Finland.[Citation45] High levels of Fe of 12 and 14 mg/100 g DW were found in the analysed bilberry and bog bilberry, respectively (). The leaves of both species contained around 9 mg/100 g DW and in this respect were comparable to fruits and vegetables consumed in Finland[Citation45] and richer in Fe than bilberry samples from Finland, Latvia, and Italy (3.1, 1.69 and 1.8 mg/100 g DW, respectively)[Citation45–Citation47] and other selected fruits consumed in UK and Mexico (0.4–4.4 and 1.1–4.1 mg/100 g DW, respectively).[Citation48,Citation49]
Bilberry and bog bilberry, with 3.8 and 3.2 mg/100 g DW, for berry and leaf extracts, respectively, contained very high levels of Cu that were approximately twice the level of Cu in European fruits and vegetables[Citation45] and those found in bilberry samples from Finland,[Citation45] Italy,[Citation46] and Latvia[Citation47] (around 0.4 mg/100 g DW).
Relatively high level of Mn was found in the studied bilberries and bog bilberries (22.2 and 23 mg/100 g DW, respectively) (). Similar Mn levels were reported for bilberry consumed in Finland and Latvia (28.9 and 18.8 mg/100 g DW, respectively)[Citation45,Citation47] and lower in berries from Italy (11.8 mg/100 g DW).[Citation46] The content of Mn in leaves was 10 times higher compared to berries (241 and 198 mg/100 g DW for bilberry and bog bilberry, respectively). The contents of Al in berries of bilberry and bog bilberry (2.7 and 4.8 mg/100 g DW, respectively) were lower compared to those of leaves of both species (24 and 15 mg/100 g DW, respectively). These values are in accordance with those found in bilberries from Finland, Italy, and Latvia (2.3, 2.2, and 3.06 mg/100 g DW, respectively).[Citation45–Citation47] Cobalt (Co) and nickel (Ni) are required by humans in minute amounts and are toxic at high levels and lead (Pb) is well known for its toxicological properties. The contents of these and other elements (As, Cd, Co, Mo, and Pb) in all analysed berry and leaf samples were below the limit of detection (for As and Pb it was <0.01 mg/100 g, and for Cd, Co, Mo, and Tl, <0.001 mg/100 g) and comply with the food safety regulations.
Conclusion
Polyphenolic and mineral profiles of berries and leaves of bilberry and bog bilberry collected from northwest Macedonia were examined for the first time. Overall, 70 different phenolic compounds were identified, including anthocyanins, flavan-3-ols, proanthocyanidins, flavonols, phenolic acids, stilbenes, coumaroyl iridoids, and cinchonain. In both studied berry species, anthocyanins were the major polyphenolic class, which is in accordance with previously published data for Vaccinium berries from the other regions. Malvidin 3-O-xyloside (A16) was found only in bog bilberry extracts and it can be used as a their marker. Delphinidin (A14), delphinidin 3-O-xyloside (A16), and petunidin3-O-rutinoside (A17) were also found only in the extracts of bog bilberry. Delphinidin (A14) has been reported in bilberry previously, whereas delphinidin 3-O-xyloside (A16) and petunidin 3-O-rutinoside (A17) are here reported for the first time in bilberry or bog bilberry.
Trans- and cis-piceid are also here reported for the first time in Vaccinium berries. Trans-piceid was detected in both studied Vaccinium species, whereas cis-piceid was detected only in bilberry. The total content of stilbenes (trans- and cis-piceid) was higher compared to that reported for other Vaccinium species, but lower than that found in grapes. The polyphenolic profile of leaves of bilberries and bog bilberries revealed five classes of compounds: flavan-3-ols, flavonols, phenolic acid derivatives, iridoids, and cinchonains. The biggest difference between species was found in the procyanidins content, which present 15% and 5% of TPC for bilberry and bog bilberry, respectively. Differences in flavonol profiles were also found and, most importantly, kaempferol feruloyl-acetyl glucoside and kaempferol coumaroyl glucoside were for the first time detected in Vaccinium species. The mineral content of the leaves and berries of bilberry and bog bilberry is found comparable to samples from other regions except for the levels of Zn, Fe, and Cu that were found to be higher than those reported from other regions.
Notes
1 Research Center for Environment and Materials, Macedonian Academy of Sciences and Arts, Bul. Krste Misirkov, 2, P.O. Box 428, 1000, Skopje, Republic of Macedonia
References
- Hariram Nile, S.; Won Park, S. Edible Berries: Review on Bioactive Components and Their Effect on Human Health. Journal of Nutrition 2013, 30, 134–144.
- Mikulic-Petkovsek, M.; Schmitzer, V.; Slatnar, A.; Stampar, F.; Veberic, R. Composition of Sugars, Organic Acids, and Total Phenolics in 25 Wild or Cultivated Berry Species. Journal of Food Science 2012, 77, C1064–C1070.
- Szajdek, A.; Borowska, E.J. Bioactive Compounds and Health-promoting Properties of Berry Fruits: A Review. Plant Foods for Human Nutrition 2008, 63, 147–156.
- Steinmetz, K.A.; Potter, J.D. Vegetables, Fruit, and Cancer Prevention: A Review. Journal of the American Dietetic Association 1996, 96, 1027–1039.
- Ness, A.R.; Powles, J.W. Fruit and Vegetables, and Cardiovascular Disease: A Review. International Journal of Epidemiology 1997, 26, 1–13.
- Kähkönen, M.P.; Hopia, A.I.; Heinonen, M. Berry Phenolics and Their Antioxidant Activity. Journal of Agricultural and Food Chemistry 2001, 49, 4076–4082.
- Puupponen-Pimiä, R.; Nohynek, L.; Meier, C.; Kähkönen, M.; Heinonen, M.; Hopia, A.; Oksman-Caldentey, K.M. Antimicrobial Properties of Phenolic Compounds from Berries. Journal of Applied Microbiology 2001, 90, 494–507.
- Colak, N.; Torun, H.; Gruz, J.; Strnad, M.; Subrtova, M.; Inceer, H.; Ayaz, F. A. Comparison of Phenolics and Phenolic Acid Profiles in Conjunction with Oxygen Radical Absorbing Capacity (ORAC) in Berries of Vaccinium arctostaphylos L. and V. myrtillus L. Polish. Journal of Food and Nutrition Science 2016, 66, 85–91.
- Colak, N.; Torun, H.; Gruz, J.; Strnad, M.; Hermosín-Gutiérrez, I.; Hayirlioglu-Ayaz, S.; Ayaz, F.A. Bog Bilberry Phenolics, Antioxidant Capacity and Nutrient Profile. Food Chemistry 2016, 201, 339–349.
- Nowak, D.; Gośliński, M.; Wojtowicz, E. Comparative Analysis of the Antioxidant Capacity of Selected Fruit Juices and Nectars: Chokeberry Juice as a Rich Source of Polyphenols. International Journal of Food Properties 2016, 19, 1317–1324.
- Singh, H.; Lily, M.K.; Dangwal, K. Viburnum Mullaha D.DON Fruit (Indian Cranberry): A Potential Source of Polyphenol with Rich Antioxidant, Anti-elastase, Anti-collagenase and Anti-tyrosinase Activities. International Journal of Food Properties 2016, doi:10.1080/10942912.2016.1217878
- Ieri, F.; Martini, S.; Innocenti, M.; Mulinacci, N. Phenolic Distribution in Liquid Preparations of Vaccinium myrtillus L. and Vaccinium vitis Idaea L. Phytochemical Analysis 2013, 24, 467−475.
- Su, Z.;. Anthocyanins and Flavonoids of Vaccinium L. Pharmaceutical Crops 2012, 3, 7–37.
- Khattab, R.; Brooks, M.S.L.; Ghanem, A. Phenolic Analyses of Haskap Berries (Lonicera caerulea L.): Spectrophotometry versus High Performance Liquid Chromatography. International Journal of Food Properties 2016, 19, 1708–1725.
- Prior, R.L.; Cao, G.; Martin, A.; Sofic, E.; McEwen, J.; Obrien, C.; Lischner, N.; Ehlenfeldt, M.; Kalt, W.; Krewer, G.; Mainland, C.M. Antioxidant Capacity as Influenced by Total Phenolic and Anthocyanin Content, Maturity, and Variety of Vaccinium Species. Journal of Agricultural and Food Chemistry 1998, 46, 2686–2693.
- Wang, L.; Su, S.; Wu, J.; Du, H.; Li, S.; Huo, J.; Zhang, Y.; Wanga, L. Variation of Anthocyanins and Flavonols in Vaccinium uliginosum Berry in Lesser Khingan Mountains and Its Antioxidant Activity. Food Chemistry 2014, 160, 357–364.
- Soetan, K.O.; Olaiya, C.O.; Oyewole, O.E. The Importance of Mineral Elements for Humans, Domestic Animals and Plants: A Review. African Journal of Food Science 2010, 4, 200–222.
- Kajdžanoska, M.; Petreska, J.; Stefova, M. Comparison of Different Extraction Solvent Mixtures for Characterization of Phenolic Compounds in Strawberries. Journal of Agricultural and Food Chemistry 2011, 59, 5272–5278.
- Jovančević, M.; Balijagić, J.; Menković, N.; Šavikin, K.; Zdunić, G.; Janković, T.; Dekić-Ivanković, M. Analysis of Phenolic Compounds in Wild Populations of Bilberry (Vaccinium myrtillus L.) from Montenegro. Journal of Medicinal Plants Research 2011, 5, 910–914.
- Šavikin, K.; Zdunić, G.; Janković, T.; Tasić, S.; Menković, N.; Stević, T.; Đorđević, B. Phenolic Content and Radical Scavenging Capacity of Berries and Related Jams from Certificated Area in Serbia. Plant Foods for Human Nutrition 2009, 64, 212–217.
- Šavikin, K.; Zdunić, G.; Janković, T.; Gođevac, D.; Stanojković, T.; Pljevljakušić, D. Berry Fruit Teas: Phenolic Composition and Cytotoxic Activity. Food Research International 2014, 62, 677–683.
- Hohtola, A. Northern Plants as a Source of Bioactive Products. In Physiology of Northern Plants under Changing Environment; Taulavuori, E.; Tauravuori, K.; Eds.; Res. Signpost: India, 2007; 291–307.
- Jaakola, L.; Maatta-Riihinen, K.; Karenlampi, S.; Hohtola, A. Activation of Flavonoid Biosynthesis by Solar Radiation in Bilberry (Vaccinium myrtillus L.) Leaves. Planta 2004, 218, 721–728.
- Taruscio, T.G.; Barney, D.L.; Exon, J. Content and Profile of Flavanoid and Phenolic Acid Compounds in Conjunction with the Antioxidant Capacity for a Variety of Northwest Vaccinium Berries. Journal of Agricultural and Food Chemistry 2004, 52, 3169–3176.
- Barnes, J.S.; Nguyen, H.P.; Shen, S.; Schug, K.A. General Method for Extraction of Blueberry Anthocyanins and Identification Using High Performance Liquid Chromatography–Electrospray Ionization-ion Trap-time of Flight-mass Spectrometry. Journal of Chromatography A 2009, 1216, 4728–4735.
- Häkkinen, S.H.; Kärenlampi, S.O.; Heinonen, I.M.; Mykkänen, H.M.; Törrönen, A.R. HPLC Method for Screening of Flavonoids and Phenolic Acids in Berries. Journal of the Science of Food and Agriculture 1998, 77, 543–551.
- Abad-Garcia, B.; Berrueta, L.A.; Garmon-Lobato, S.; Gallo, B.; Vicente, F. A General Analytical Strategy for the Characterization of Phenolic Compounds in Fruit Juices by High-performance Liquid Chromatography with Diode Array Detection Coupled to Electrospray Ionization and Triple Quadrupole Mass Spectrometry. Journal of Chromatogrraphy A 2009, 1216, 5398–5415.
- Lätti, A.K.; Jaakola, L.; Riihinen, K.R.; Kainulainen, P.S. Anthocyanin and Flavonol Variation in Bog Bilberries (Vaccinium uliginosum L.) In Finland. Journal of Agricultural and Food Chemistry 2010, 58, 427–433.
- Može, Š.; Polak, T.; Gašperlin, L.; Koron, D.; Vanzo, A.; Poklar Ulrih, N.; Abram, V. Phenolics in Slovenian Bilberries (Vaccinium myrtillus L.) and Blueberries (Vaccinium corymbosum L.). Journal of Agricultural and Food Chemistry 2011, 59, 6998–7004.
- Lätti, A.K.; Riihinen, K.R.; Kainulainen, P.S. Analysis of Anthocyanin Variation in Wild Populations of Bilberry (Vaccinium myrtillus L.) in Finland. Journal of Agricultural and Food Chemistry 2008, 56, 190–196.
- Martz, F.; Jaakola, L.; Julkunen-Tiitto, R.; Stark, S. Phenolic Composition and Antioxidant Capacity of Bilberry (Vaccinium myrtillus) Leaves in Northern Europe following Foliar Development and along Environmental Gradients. Journal of Chemical Ecology 2010, 36, 1017–1028.
- Mikulic-Petkovsek, M.; Slatnar, A.; Stampar, F.; Veberic, R. Hplc-Msn Identification and Quantification of Flavonol Glycosides in 28 Wild and Cultivated Berry Species. Food Chemistry 2012, 135, 2138–2146.
- Hokkanen, J.; Mattila, S.; Jaakola, L.; Pirttila, A.M.; Tolonen, A. Identification of Phenolic Compounds from Lingonberry (Vacciniumvitis-Idaea L.), Bilberry (Vaccinium myrtillus L.) and Hybridbilberry (Vaccinium Intermedium Ruthe L.) Leaves. Journal of Agricultural and Food Chemistry 2009, 57, 9437–9447.
- Clifford, M.N.; Johnston, K.L.; Knight, S.; Kuhnert, N. Hierarchical Schemefor LC–Msn Identification of Chlorogenic Acids. Journal of Agricultural and Food Chemistry 2003, 51, 2900–2911.
- Gavrilova, V.; Kajdžanoska, M.; Gjamovski, V.; Stefova, M. Separation, Characterization and Quantification of Phenolic Compounds in Blueberries and Red and Black Currants by HPLC-DAD-ESI-Msn. Journal of Agricultural and Food Chemistry 2011, 59, 4009–4018.
- Laaksonen, O.; Sandell, M.; Kallio, H. Chemical Factors Contributing to Orosensory Profiles of Bilberry (Vaccinium myrtillus) Fractions. European Food Research and Technology 2010, 231, 271–285.
- Ehala, S.; Vaher, M.; Kaljurand, M. Characterization of Phenolic Profiles of Northern European Berries by Capillary Electrophoresis and Determination of Their Antioxidant Activity. Journal of Agricultural and Food Chemistry 2005, 53, 6484–6490.
- Rimando, A.M.; Kalt, W.; Magee, J.B.; Dewey, J.; Ballington, J.R. Resveratrol, Pterostilbene, and Piceatannol in Vaccinium Berries. Journal of Agricultural and Food Chemistry 2004, 52, 4713–4719.
- Stefkov,, G.; Hristovski, S.; Petreska Stanoeva, J.; Stefova, M.; Melovski,, L.; Kulevanova, S. Resource Assessment and Economic Potential of Bilberries (Vaccinium myrtillus and Vaccinium uliginosum) on Osogovo Mtn., R. Macedonia. Industrial Crops and Products 2014, 61, 145–150.
- Kajdžanoska, M.; Gjamovski, V.; Stefova, M. HPLC-DAD-ESI-Msn Identification of Phenolic Compounds in Cultivated Strawberries from Macedonia. Macedonian Journal of Chemistry and Chemical Engineering. 2010, 29, 181–194.
- Oszmiański, J.; Wojdyło, A.; Gorzelany, J.; Kapusta, I. Identification and Characterization Of low Molecular Weight Polyphenols in Berry Beaf Extracts by HPLC-DAD and LC-ESI/MS. Journal of Agricultural and Food Chemistry 2011, 59, 12830–12835.
- Harris, C.S.; Burt, A.J.; Saleem, A.; Le, P.M.; Martineau, L.C.; Haddad, P.; Bennett, S.A.L.; Arnason, J.T. A Single HPLC-PADAPCI/MS Method for the Quantitative Comparison of Phenolic Compounds Found in Leaf, Stem, Root and Fruit Extracts of Vaccinium angustifolium. Phytochemical Analysis 2007, 18, 161–169.
- Turner, A.; Chen, S.N.; Nikolic, D.; van Breemer, R.; Farnsworth, N.R.; Pauli, G.F. Coumaroyl Iridoids and a Depside from Cranberry (Vaccinium macrocarpon). Journal of Natural Products 2007, 70, 253–258.
- Damascos, M.; Arribere, M.; Svriz, M.; Bran, D. Fruit Mineral Contents of Six Wild Species of the North Andean Patagonia, Argentina. Biological Trace Element Research 2008, 125, 72–80.
- Ekholm, P.; Reinivuo, H.; Mattila, P.; Pakkala, H.; Koponen, J.; Happonen, A.; Hellström, J.; Ovaskainen, M.L. Changes in the Mineral and Trace Element Content of Cereals, Fruits and Vegetablesin Finland. Journal of Food Composition and Analysis 2007, 20, 487−495.
- Barizza, E.; Guzzo, F.; Fanton, P.; Lucchini, G.; Sacchi, G.A.; Lo Schiavo, F.; Nascimbene, J. Nutritional Profile and Productivity of Bilberry (Vaccinium myrtillus L.) in Different Habitats of Aprotected Area of the Eastern Italian Alps. Journal of Food Science 2013, 78, C673–C678.
- Skesters, A.; Kleiner, D.; Blázovics, A.; May, Z.; Kurucz, D.; Silova, A.; Szentmihályi, K. Mineral Element Content and Antioxidant Capacity of Some Latvian Berries. European Chemical Bulletin 2014, 3, 98–101.
- Sanchez-Castillo, C.; Dewey, P.J.S.; Aguirre, A.; Lara, J.J.; Vaca, R.; de la Barra, P.L.; Ortiz, M.; Escamilla, I.; James, W.P.T. The Mineral Content of Mexican Fruits and Vegetables. Journal of Food Composition and Analysis 1998, 11, 340−356.
- Davis, D.R. Declining Fruit and Vegetable Nutrient Composition. What Is the Evidence? Hortscience 2009, 44, 15–19.