ABSTRACT
This paper investigated the effects of l-lysine (Lys) and l-arginine (Arg) on the water-holding capacity (WHC) and hardness of myosin gel as well as the mechanism of these effects. The results showed that Lys, Arg, and KOH increased the WHC but decreased the hardness in all cases. At pH 6.32, the WHC increased in the order control < KOH < Lys ≈ Arg, while the hardness decreased in the order control > KOH > Lys > Arg. Lys shortened low field nuclear magnetic resonance spin-spin relaxation times (T2), while Arg and KOH slightly affected T2. Lys, Arg, and KOH had different influences on the molecular forces in myosin gels and the distribution of myosin size. Lys formed a gel with porous clusters, while Arg generated a gel with fine caves. Therefore, both Lys and Arg changed the microstructure of myosin gel by changing the distribution of the myosin size as well as the molecular forces that form and/or maintain myosin gel, ultimately contributing to the differences in the WHC, hardness, and T2.
Introduction
Water-holding capacity (WHC) and texture are two important properties of meat products.[Citation1] The current method to improve WHC and texture uses sodium chloride and phosphates.[Citation2,Citation3] However, excessive intake of sodium chloride and phosphates has been reported to have potential health risks.[Citation4,Citation5] The development of reduced-sodium and low-phosphate meat products is an important direction of research in the meat industry.[Citation6,Citation7] However, reducing sodium chloride and phosphates can decrease the water-binding capacity, texture, and juiciness of meat products.[Citation1,Citation5] Recently, l-lysine (Lys) and l-arginine (Arg) have been reported to decrease cooking loss (CL), but increase WHC and texture of low-phosphate pork sausage,[Citation8,Citation9] indicating that either Lys or Arg may improve the yield and textures of meat products. Further investigations are necessary to understand how Lys or Arg improves the yield and texture of meat products.
Myosin, a component of the myofibrillar protein matrix, is able to form a three-dimensional network gel and is substantially responsible for the WHC and texture of processed meat products.[Citation10] The factors that are responsible for the WHC and texture of meat and meat products are fundamentally summarized as the molecular forces and microstructure of salt-soluble meat protein (SSMP) gel, which depend on pH and other factors.[Citation10,Citation11]
Both Lys and Arg have been reported to increase the solubility of porcine myosin and chicken myosin[Citation12–Citation14] and increase the pH of pork sausage.[Citation8,Citation9] Lys reportedly decreased the α-helical content, but increased the surface hydrophobicity and reactive sulphydryl content of porcine myosin.[Citation12] Unlike Lys, Arg reportedly had slight influences on the secondary structure of myosin;[Citation13] moreover, Arg has been reported to suppress protein aggregation.[Citation15] The solubility, pH, secondary structure, and aggregation are related to the properties of heat-induced myosin gel. Theoretically, both Lys and Arg may have significant but different influences on chicken myosin gel. To date, a few papers have reported on the effects of histidine on the thermal gelling properties of the myosin system[Citation16,Citation17] and the effects of Arg on actomyosin[Citation18] or the SSMP system.[Citation19] However, information is lacking regarding the effects of Lys or Arg on the thermal gelling properties of the myosin system. Furthermore, the SSMP system and the actomyosin system contain a variety of proteins such as myosin, actin, and/or their complexes, and it is not clear whether any specific protein of the systems interacts with Lys or Arg. This study focused on the comparative effects of Lys and Arg on the WHC and hardness of chicken myosin gel. It also attempted to discern the possible mechanism from the molecular forces and microstructure of the myosin gel by using different instrumental techniques.
Materials and methods
Materials
Lys and Arg were purchased from Shanghai Juyuan Biotechnology Co., Ltd. (Shanghai, China). Three batches (approximately 10 kg) of chicken breasts obtained from birds slaughtered at the age of approximately 40 days were purchased from a local supermarket (the Carrefour Group).
Extraction of myosin
Immediately after the poultry was killed, myosin was extracted from chicken breast muscle according to the previous methods with slight modifications.[Citation1] Around 500 mL of cold solution (0.1 M KCl, 20 mM potassium phosphate, 2 mM EDTA-2Na, pH 7.0) containing 1 mM dithiothreitol was added to 150 g of the minced meat. The mixture was stirred with a DS-1 blender (Shanghai Yueci Electronic Technology Co., Ltd., China), treated with 1% Triton X-100, and then homogenized at 10,000 rpm using an FA25 Model homogenizer (Fluko, China). The homogenization was performed three times for 20 s each. The mixture was centrifuged for 10 min at 8,000 g using a GL-211M centrifuge (Xiangyi Laboratory Instrument Development Co., Ltd., Changsha, China). The sediment was obtained and then extracted for 15 min with three volumes of a solution (0.3 M KCl, 0.1 M KH2PO4, 50 mM K2HPO4, 1 mM EDTA-2Na, 4 mM sodium pyrophosphate, pH 6.5). The resulting extract was subsequently centrifuged for 12 min at 5,000 g. The supernatant was diluted with 14 volumes of cold 1 mM EDTA-2Na solution and stored for 2.5 h. The diluted supernatant was removed from the floating substance using a siphon, centrifuged at 10,000 g for 6 min and suspended with 1 volume of 0.6 M KCl buffer (0.6 M KCl, 10 mM potassium phosphate, pH 6.5). The precipitated protein was collected. Then, 5 mM MgCl2 · 6H2O, 5 mM sodium pyrophosphate (Na4P2O7 · 10H2O), and 2 mM ATP-2Na were added to the protein. The mixture was stirred for 30 min followed by a 20-min centrifugation at 10,000 g. The supernatant was diluted again with nine volumes of cold distilled water and then precipitated for approximately 12 h. The floating substance and precipitated protein were retreated according to the above-mentioned procedure. The resulting solution was dialysed against the 0.6 M KCl buffer (pH 6.5) using the same protocol mentioned above. The supernatant was considered as extracted myosin. All steps were carried out at 0–4°C in a cold chamber.
The composition and concentration of the extracted myosin were determined by SDS-PAGE with a gel imaging system (Universal Hood II, Bio-rad Co., USA) (Supplementary material 1) and a Biuret method,[Citation12] respectively. The final protein concentration was adjusted to 20 mg/mL of myosin using a 0.6 M KCl buffer (pH 6.5).
Preparation of myosin solutions
To each actomyosin solution prepared according to 2.1.2, either Lys or Arg (A, control), 0.01% Lys (m/v) (B), 0.05% Lys (m/v) (C), 0.10% Lys (m/v) (D), 0.01% Arg (m/v) (E), 0.05% Arg (m/v) (F), or 0.10% Arg (m/v) (G) was added. The pH of each myosin solution prepared above was then immediately measured;[Citation19] the pH for each myosin solution was 6.04 ± 0.03, 6.11 ± 0.01, 6.32 ± 0.02, 6.62 ± 0.01, 6.10 ± 0.01, 6.32 ± 0.01, and 6.62 ± 0.01, respectively. The pH of the myosin solution, which is important for myosin gel,[Citation10] was adjusted to 6.32 with a dilute KOH solution before heating (H). To ensure that Lys or Arg had fully interacted with myosin, the eight myosin solutions were stored for approximately 12 h at 4°C before use.
Preparation of myosin gels
Myosin gels were prepared according to the previous method.[Citation18] For each of the above myosin solutions, the process was as follows: 10 mL of the myosin solution was heated in a beaker (inner diameter: 25 mm; height: 35 mm). The temperature was increased from 20°C to 80°C at the rate of 4°C/min and then maintained at 80 ± 2°C for 30 min in a water bath. The sample was immediately cooled with flowing tap water and stored for approximately 12 h at 4°C for the next analysis.
Physicochemical properties of myosin gels
Water-holding capacity
The WHC of the gel was analysed according to the procedure described by Liu et al.[Citation20] with slight modifications. Specifically, gel (approximately 10 g) was centrifuged at 1,000 g at 4°C for 10 min. Each sample was measured in triplicate (n = 3). The WHC was expressed as follows:
WHC (%) = m2 ÷ m1 × 100
where m1 and m2 are the initial weight and the weight retained after centrifugation of myosin gel, respectively.
Hardness
Gel hardness was measured using a TA-XTplus Texture Analyzer (Stable Micro System Co., Surrey, UK) at room temperature (approximately 20°C) according to the protocol described by Qin et al.[Citation19] with some modifications. Gel was subjected to a compression test using a cylindrical probe (P/0.5 inch, aluminium). The test conditions were as follows: trigger type strain, pretest speed 1.5 mm/s, test speed 1.0 mm/s, post-test speed 1.0 mm/s, distance 4.0 mm, trigger force 0.05 N, and time lag 5 s between two compressions. Gel hardness was reported as the maximum sustained compression force. Each sample was carried out in triplicate (n = 3).
Low field nuclear magnetic resonance spin-spin relaxation times:
Low field nuclear magnetic resonance (LF-NMR) Citation1H spin-spin relaxation time (T2) was measured based on a previous method with slight modifications.[Citation21] One to three grams of each gel sample was placed in a glass tube (inner diameter: 60 mm). Data were collected using a Niumag Benchtop Pulsed NMR Analyzer MesoMR23-060H-I (Niumag Electric Corporation, Shanghai, China). The operation conditions were as follows: a proton resonance of 23.311 MHz, a magnetic field intensity of 0.5 T, and a τ-value (time between the 90° and 180° pulse) of 0.2 ms. Data from 16,000 echoes per scan were obtained from eight scan repetitions with 7.5 s between each scan. NMR T2 data were analysed using Multi-Exp Inv Analysis Software (Niumag Electric Corp., Shanghai, China). Each sample was measured in triplicate (n = 3).
Molecular forces in myosin gels
Using the procedure described by Li et al.[Citation22] with some modifications, of the myosin solutions mentioned in 2.3, four samples (without Lys or Arg, with 0.05% Lys, and with 0.05% Arg, all with their pH adjusted to 6.32 using a dilute KOH solution) were treated with destabilizing reagents as follows: no reagent (control), 0.5 M sodium thiocyanate (NaSCN), 10% (v/v) propylene glycol (PG), 0.2 M 2-mercaptoethanol (2-MeSH), and 0.5 M urea. Samples were fully stirred and homogenized for 30 s at medium speed using a blender. Gels were prepared according to the method described above. Their WHC and strength were determined in triplicate (n = 3) as described above.
Scanning electron microscopy (SEM)
Gel microstructure was determined according to a previous protocol with some modifications.[Citation1] First, the gel (5 × 5 × 1 mm) was dried for nearly 24 h in an FD-1A-50 lyophilizer (Beijing, Beijing Boyikang Experimental Instrument Co. Ltd., China). Second, scanning electron microscopy (SEM) observations were performed with a scanning electron microscope (Jeol, JSM 6490LV, Tokyo, Japan). At least two fields from each sample were examined, and one was illustrated.
Distribution of myosin size
Among the myosin solutions mentioned in 2.3, four samples (without Lys or Arg, with 0.05% Lys, and with 0.05% Arg, all with their pH adjusted to 6.32 using a dilute KOH solution) were measured with an MS-2000 particle size analyser (Malvern Instruments Ltd, Great Malvern, UK) equipped with a 4 mW He-Ne laser (k = 633 nm).[Citation23] The myosin solutions were placed in a 1-cm path length quartz cuvette and measured with a detection angle of 90° at 25 ± 0.1°C. The hydrodynamic diameters of myosin particles were estimated from the autocorrelation function, using the Cumulants method,[Citation23] and the distributions of scattering particle size were monitored based on the scattering intensity. Each sample was measured in triplicate (n = 3).
Statistical analysis
Analyses of variances (ANOVAs), means, and standard errors were determined using Excel 2003 (Microsoft Office Excel 2003 for Windows). All data were presented as the mean ± SD. ANOVA was introduced to determine the significance of the samples at the p < 0.05 level by the t-test.
Results and discussion
WHC and texture
Water-holding capacity
WHC is an important property of meat protein gel that reflects the physical entrapment of water in myosin gel. As shown in , the samples treated with Lys or Arg had a higher WHC than the control in all cases. Moreover, the WHC increased with increasing Lys or Arg (p < 0.05). The WHC was not obviously different between the samples treated with the same concentrations of Lys and Arg (p > 0.05). The present results suggest that the application of Lys or Arg is favourable for improving the WHC. Arg was also reported to increase the WHC of chicken actomyosin[Citation18] and salt-soluble protein gel.[Citation19] The sample treated with KOH had a higher WHC than the control. This was in accordance with previous findings.[Citation11,Citation20]
Figure 1. Effects of l-lysine (Lys), l-arginine (Arg), or pH on the WHC (A) and hardness (B) of myosin gel (n = 3). A: Lys or Arg 0%, pH 6.04; B: Lys 0.01%, pH 6.11; C: Lys 0.05%, pH 6.32; D: Lys 0.10%, pH 6.62; E: Arg 0.01%, pH 6.10; F: Arg 0.05%, pH 6.32; G: Arg 0.10%, pH 6.62; and H: Lys or Arg 0%, pH 6.32. a–f mean that the same index is significant different (p < 0.05).
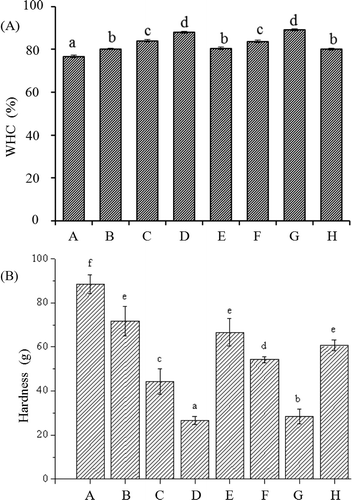
The WHC was mainly influenced by the molecular forces and gel microstructure. As mentioned above, the addition of Lys or Arg induced a pH in the myosin solution, which further altered the isoelectric points of the salt-soluble proteins, which could theoretically strengthen the electrostatic repulsion between protein molecules and expose more sites to bind water and ultimately increase the WHC.[Citation11] This was further confirmed by the fact that the sample treated with KOH had a higher WHC than the control. However, the sample treated with KOH had a lower WHC than the samples treated with 0.05% Lys or 0.05% Arg (p < 0.05), indicating that in addition to pH, there may be other factors that also affect WHC. The antioxidant properties of Lys and Arg may affect the formation of disulphide bonds in myosin gel,[Citation24] and the amino group and carboxyl group in Lys and Arg molecules may affect the hydrogen bonding of myosin gel. These may be important for the WHC. On the other hand,[Citation10] showed that small pores were more favourable for holding the majority of water in a gel network, compared to large ones. The previous work showed that Arg resulted in gels with fine caves,[Citation18,Citation19] which may synergistically influence the WHC of the myosin gel.
Instrumental texture
Texture is another important characteristic of meat protein gel and is associated with chewiness. As shown in , the hardness decreased with increased Lys or Arg in all cases (p < 0.05). The present results showed that the introduction of Lys or Arg to the myosin solution favoured the formation of a softer gel. However, previous findings showed that Arg increased the hardness of chicken actomyosin[Citation18] and salt-soluble proteins.[Citation19] In addition to myosin, actin exists in chicken salt-soluble proteins and actomyosin.[Citation10] Although actin cannot form a gel by itself, the presence of actin can enhance the gelling ability of myosin when induced by heat treatment.[Citation10,Citation25] This may contribute to the differences between the present and previous results.
Molecular forces and gel microstructure are the two main factors that affect the hardness of myosin gels. As mentioned above, the addition of Lys or Arg changed the pH of the myosin solution and deviated from the optimum pH for gel formation,[Citation10] which may contribute to the decreased gel hardness. This was also supported by the fact that the sample treated with KOH had lower hardness than the control. However, the sample treated with KOH had higher hardness than the samples treated with 0.05% Lys or 0.05% Arg (p < 0.05), although these samples had the almost same pH. This difference could not be entirely attributed to the changes in pH. Both Lys and Arg have antioxidant properties, which may prevent active sulfhydryl groups from forming disulphide bonds.[Citation24] This may provide a plausible explanation for the fact that the samples treated with 0.05% Lys or 0.05% Arg had lower hardness than the sample treated with KOH.
LF-NMR spin-spin relaxation times
The dynamics of water, cooperatively determined by both the molecular forces and microstructure of the myosin gel, were investigated using NMR measurement.[Citation21] As illustrated in , four obvious relaxation populations in the NMR T2 signal for the control, centred at approximately 6.03–10.72 ms (T2b), 75.61–132.69 ms (T21), 199.58–930.93 ms (T22), and 1417.60–3272.53 ms (T23), were assigned to the bound, partially immobilized, immobilized, and free water, respectively. Similarly, four relaxation populations were also found in a typical NMR T2 signal for porcine salt-soluble protein gel[Citation21] and chicken salt-soluble protein gel.[Citation19] The immobilized water reflects the capacity of processed meat products to hold the added water;[Citation21] therefore, the T22 fraction was emphasized in discussion.
Figure 2. Effects of l-lysine (Lys), l-arginine (Arg), or pH on the distribution of T2 relaxation times of myosin gels (n = 3). A: Lys or Arg 0%, pH 6.04; B: Lys 0.01%, pH 6.11; C: Lys 0.05%, pH 6.32; D: Lys 0.10%, pH 6.62; E: Arg 0.01%, pH 6.10; F: Arg 0.05%, pH 6.32; G: Arg 0.10%, pH 6.62; and H: Lys or Arg 0%, pH 6.32.
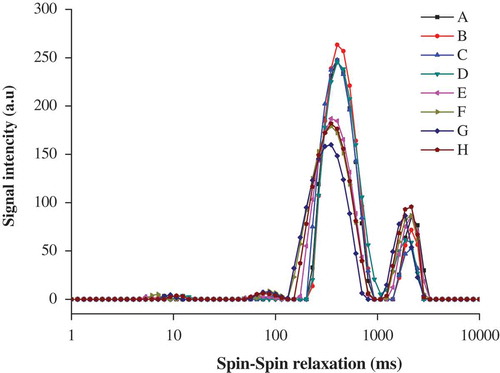
Compared with the control, the addition of Arg caused shorter T2 relaxation times in all cases. The T2 relaxation times tended to become shorter with increased Arg (p < 0.05). Similarly, the addition of k-carrageenan also decreased the T2 relaxation times in myosin gel.[Citation1] The present results suggest that the water mobility was somehow decreased in the presence of Arg. As mentioned above, Arg caused the pH of the myosin solution to further deviate from the isoelectric point of myosin; theoretically, myosin gained more net negative charge, which may strengthen the interaction between myosin and water,[Citation11] ultimately contributing to the shorter T2 relaxation times. This view was also confirmed by the fact that the T2 signal shifted in the shorter direction in the sample treated with KOH (p < 0.05), compared with the control. In addition, the amino group and the carboxyl group may also lead to the shorter T2 relaxation times via hydrogen bonding. However, Arg reportedly prolonged the T2 relaxation times in the chicken salt-soluble protein gel[Citation19] and chicken actomyosin.[Citation18] Further investigations are necessary to understand the difference. In contrast to Arg, Lys had only slight influences on the T2 relaxation times in all cases (p > 0.05). The results suggested that the T2 relaxation times were more dependent on the amino acid type than on its levels. This could not be entirely explained by the higher pH and the enhanced hydrogen bonding. The pore size of myosin gel was negatively correlated with water mobility[Citation21] and had important influences on T2 relaxation times. SEM indicated that the gel with Lys had overall bigger caves than the one with Arg (to be discussed below), which may offset the electrostatic interactions. This may be responsible for the Lys-treatment causing slight changes in T2 relaxation times.
As summarized in , the addition of 0.01–0.1% Lys or 0.05–0.1% Arg increased the proportion of the T22 fraction (PT22) (p > 0.05), suggesting that there may be a positive relationship between the fraction of T22 distribution and WHC. This was consistent with a previous description.[Citation21,Citation26] An increase in PT22 was also observed in chicken salt-soluble protein gel[Citation19] and chicken actomyosin in the presence of Arg.[Citation18] Increases in the solubility of myosin[Citation12,Citation13] and formation of the gel with fine pores may contribute to the increased PT22. The sample treated with KOH had a slightly higher fraction of T22 distribution (p > 0.05) than the control, but was obviously lower than the sample treated with 0.05% Lys or 0.05% Arg (p < 0.05), respectively. This was not fully explained by the changes in pH. On the other hand, Han et al.[Citation21] noted that the water in large pores had better mobility than that in small pores. The caves in the sample treated with KOH were bigger than those in the sample treated with 0.05% Lys or 0.05% Arg, respectively. This was a possible reason that the sample treated with KOH had a significantly lower fraction of T22 distribution than the samples treated with 0.05% Lys or 0.05% Arg.
Table 1. Effects of l-lysine (Lys), l-arginine (Arg), or pH the proportion (PT22) and width for T22 relaxation time of myosin heat-induced gelation (n = 3).
As for Lys-myosin composite gels, the width of the populations centred at T22 decreased with elevated Lys, and a similar tendency was also found in Arg-myosin composite gels (). The results indicated that the increases in Lys or Arg caused the small variations in the chemical and physical properties of the myosin gels. This was most likely due to the increased pH. However, the samples treated with 0.01% Lys or 0.01% Arg had a broader population centred at T22 compared with the control (p < 0.05). Additionally, the sample treated with KOH had a broader population centred at T22 than either the control or the samples treated with 0.01–0.10% Lys or Arg (p < 0.05). The results could not be completely ascribed to pH. Han et al.[Citation21] noted that the structural heterogeneity of myosin gel was also important for the width of the population centred at T22. The sample treated with KOH had a more uneven microstructure than the samples treated with 0.05% Lys or Arg, which may contribute to the broader population centred at T22.
The present results showed that molecular forces and microstructure may have collaborative effects on the WHC, texture, and T2 of myosin gel. Therefore, the main molecular forces and microstructure were further investigated to clarify the possible role of Lys or Arg in the characteristics of myosin gel.
Molecular force
Various stabilizing/destabilizing reagents have important influences on the formation, strength, and syneresis of gels, which may reflect the main molecular forces that form or maintain the three-dimensional network structure.[Citation27] Therefore, NaSCN, PG, 2-MeSH, and urea were examined to gain insight into the effects of Lys or Arg on the main molecular forces in myosin gel.
As shown in , the addition of NaSCN increased the WHC, but decreased the hardness, in all cases (p < 0.05). Considering that NaSCN is able to neutralize charges,[Citation28] it could be deduced that electrostatic interactions were the major forces cooperatively forming and/or maintaining the myosin gel networks in all cases. Compared with the control, the decreases in hardness were more significant in the samples treated with Lys, Arg, or KOH, indicating that electrostatic interactions may be more important in those three samples. Similarly, some literature has also showed that the addition of NaSCN decreased the hardness of composite meat protein gel with flaxseed gum.[Citation29] The samples treated with Lys, Arg, or KOH had higher pH values than the control, which caused further deviation from the isoelectric point of myosin. Consequently, myosin gained a net negative charge, which may affect the formation or maintenance of the three-dimensional network structure.
Figure 3. Effects of NaSCN, PG, 2-MeSH, or urea on the WHC (A) and hardness (B) of various myosin gel (n = 3). A: Lys or Arg 0%, pH 6.04; C: Lys 0.05%, pH 6.32; F: Arg 0.05%, pH 6.32; and H: Lys or Arg 0%, pH 6.32. a–d mean that the same index is significant different (p < 0.05).
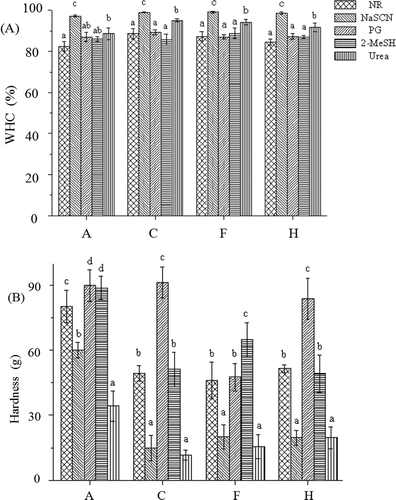
It is well known that PG can disrupt hydrophobic forces and enhance hydrogen bonds and electrostatic interactions.[Citation30] PG increased the hardness of the control and the samples treated with Lys or KOH (p < 0.05), suggesting that hydrogen bonds and electrostatic interactions were more important than hydrophobic forces in those three samples. Furthermore, the hardness increased by approximately 12%, 85%, and 62% in the control, the sample treated with Lys, and the sample treated with KOH, respectively, indicating that the importance of hydrogen bonds and electrostatic interactions in the three samples decreased in the following order: the sample treated with Lys > the sample treated with KOH > the control. The amino group and the carboxyl group of Lys have a strong capacity to form hydrogen bonds, which may enforce the hydrogen bonding of the myosin gel. In contrast, PG slightly varied the hardness of the sample treated with Arg (p > 0.05), showing that hydrogen bonds and electrostatic interactions were rarely more important than hydrophobic forces in the Arg-myosin composite gel. In addition to the amino group and carboxyl group, Arg has a guanidinium group that interacts with the aromatic residues of protein by cation interactions,[Citation31] which may reinforce hydrophobic forces. Therefore, hydrophobic forces became more important in the Arg-myosin composite gel than in the other myosin gels.
2-MeSH is usually considered to cleave inter- and intramolecular disulphide bonds to facilitate protein unfolding and increase the exposure of reactive groups that could be involved in hydrogen bonding and hydrophobic interactions.[Citation30] 2-MeSH caused an obvious increase in the hardness of the control and the sample treated with Arg (p < 0.05), suggesting that disulphide bonds were important for the control and the sample treated with Arg. Additionally, the hardness increased by approximately 10% and 41% in the control and sample treated with Arg, respectively, showing that disulphide bonds in the control were less important than those in the sample treated with Arg. Arg was reported to have an antioxidative activity,[Citation24] which may inhibit the formation of disulphide bonds. In contrast, 2-MeSH caused only slight changes in the hardness of the samples treated with Lys or KOH (p > 0.05), suggesting that disulphide bonds were rarely important for the samples treated with Lys or KOH.
Urea increased the WHC, but decreased the hardness, in all cases (p < 0.05). Urea at high concentrations is well known to diminish hydrogen bonds and weaken hydrophobic interactions by altering the water structure and increasing the solubility of hydrophobic amino acid side chains.[Citation22] Therefore, the results suggested that hydrogen bonds and/or hydrophobic interactions were involved in the gel networks in all cases. However, the hardness decreased by approximately 57%, 76%, 66%, and 62% in the control and the samples treated with Lys, Arg, and KOH, respectively, showing that the contribution of hydrogen bonds and electrostatic interactions in the four samples were different and decreased in the following order: the sample treated with Lys > the sample treated with Arg > the sample treated with KOH > the control.
Microstructure (SEM)
Microstructure is also correlated with the WHC and texture of gels.[Citation1,Citation19,Citation21] In the control, many clusters were found (). This was basically in accordance with previous findings.[Citation18,Citation19] Compared with the control, the protein aggregates tended to become smaller in the presence of 0.01% and 0.05% Lys. Furthermore, there were many fine pores in the protein aggregates ( and ). Compared with the control, the addition of 0.1% Lys also resulted in smaller clusters in the myosin gel (). However, the addition of 0.1% Lys could have induced a more concave–convex microstructure with larger aggregation, which resulted in more severe phase separation than did the addition of 0.01% or 0.05% Lys. The fine pores distributed in the clusters also disappeared. The caves in the Arg-myosin composite gel were smaller than those in the control, but bigger than those in the Lys-myosin composite gel (–). Protein aggregates were not observed in the Arg-myosin composite gel. Arg has weak preferential exclusion from the protein surface, which prevents the proteins from aggregating.[Citation32] The caves in the sample treated with KOH were bigger and more irregular than those in the Lys-myosin composite gel (). It was remarkable that the microstructures were distinctly different between the myosin gel, the Lys-myosin composite gel, the Arg-myosin composite gel, and the KOH-myosin composite gel. The changes in the gel microstructure might have contributed to the increased WHC and the decreased hardness in the presence of Lys or Arg. The present results indicate that the amino acid type is more important for the microstructures of the myosin gel than its level, which could not entirely be explained by the change of pH. Yamamoto[Citation33] and Yamamoto et al.[Citation34] showed that the microstructure of the thermal myosin gel was related to myosin’s form in solution. Therefore, myosin’s form in solution was further investigated.
Figure 4. Effects of l-lysine (Lys), l-arginine (Arg), or pH on the SEM images (left: A1–H1, × 500; and right: A2–H2, × 2000) of myosin gel. A: Lys or Arg 0%, pH 6.04; B: Lys 0.01%, pH 6.11; C: Lys 0.05%, pH 6.32; D: Lys 0.10%, pH 6.62; E: Arg 0.01%, pH 6.10; F: Arg 0.05%, pH 6.32; G: Arg 0.10%, pH 6.62; and H: Lys or Arg 0%, pH 6.32.
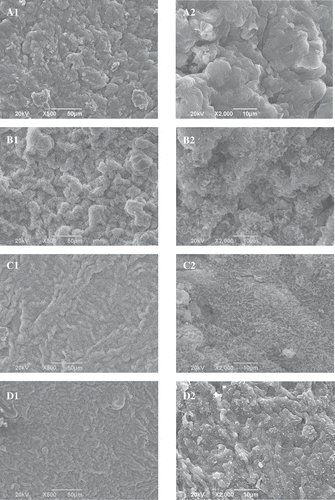
Distribution of myosin size
Myosin form in solution can be analysed by particle size distribution.[Citation23] In the control solution, two peaks at 11.5 and 85.3 µm along with an unresolved “shoulder” at 362.1 nm were observed (). Based on transmission electron microscopy observations, the peak at 11.5 µm was assigned to myosin monomers, and the other peak and unresolved “shoulder” were attributed to soluble filaments.[Citation23] These results indicated that soluble myosin solution is not homogeneous but contains monomeric species and soluble filaments.
Figure 5. Effects of l-lysine (Lys), l-arginine (Arg), or pH on the size distribution of myosin (n = 3). A: Lys or Arg 0%, pH 6.04; C: Lys 0.05%, pH 6.32; F: Arg 0.05%, pH 6.32; and H: Lys or Arg 0%, pH 6.32.
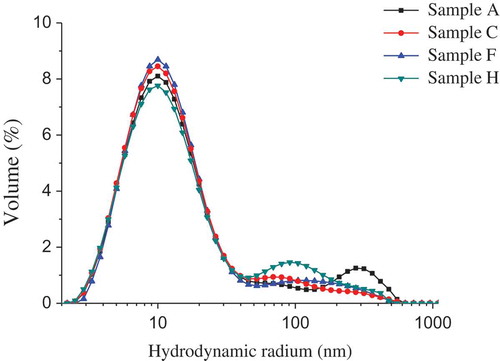
Lys, Arg, and KOH had significant but different influences on the size distribution of soluble myosin filaments. Among the various treatments, the proportion of the first peak increased in the following order: KOH < control < Lys < Arg. The results showed that the equilibrium between myosin filament and its monomer in solution could be varied by Lys, Arg, and KOH adjustment: both Lys and Arg increased the proportion of myosin monomers; moreover, Arg was more effective than Lys. Unlike Lys and Arg, KOH caused a decrease in the proportion of myosin monomers. Takai et al.[Citation13] found that Arg was more effective in increasing the solubility of porcine myosin in a physiological salt solution than Lys was. The authors also proposed that the solubility of porcine myosin may be due to the dissociation of myosin filaments. Schneider and Trout[Citation32] showed that Arg prevented protein from aggregation due to the weak preferential exclusion of Arg from the protein surface.
Usually, two classical thermal myosin gels, aggregate-type gel and strand-type gel, are formed under two extreme conditions, myosin monomers and myosin filaments,[Citation33] respectively. In actuality, both myosin monomers and myosin filaments coexist in any given solution, and their ratio depends on the conditions. As mentioned above, both Lys and Arg caused an increase in the proportion of myosin monomers, which may favour the formation of strand-type gel, while KOH decreased the proportion of myosin monomers, which, theoretically, tends to form aggregate-type gel. This was consistent with the above-mentioned SEM observations.
Conclusion
At pH 6.32, Lys and Arg had similar influences on the WHC, but different influences on the hardness, the T2, the importance of the main molecular forces, the microstructure of the myosin gel, and the size distribution of myosin. It could be speculated that Lys and Arg had similar influences on the WHC, but different influences on the hardness and T2 of myosin gel by varying both the size distribution of myosin, which may cause the different microstructure of the myosin gel, and the molecular forces forming and/or maintaining the myosin gel.
LJFP_A_1315593_Supplementary_material.doc
Download MS Word (265.5 KB)Funding
This work was financially supported by the National Natural Science Foundation of China (No. 21542008). There is no conflict of interest.
Supplemental data
Supplemental data for this article can be accessed on the publisher’s website.
Additional information
Funding
References
- Chen, X.; Chen, C.G.; Zhou, Y.Z.; Li, P.J.; Ma, F. Effects of High Pressure Processing on the Thermal Gelling Properties of Chicken Breast Myosin Containing K-Carrageenan. Food Hydrocolloids 2014, 40, 262–272.
- Hong, G.P.; Chin, K.B. Effects of Microbial Transglutaminase and Sodium Alginate on Cold-Set Gelation of Porcine Myofibrillar Protein with Various Salt Levels. Food Hydrocolloids 2010, 24 (4), 444–451.
- Yapar, A.; Atay, S.; Kayacier, A.; Yetim, H. Effects of Different Levels of Salt and Phosphate on Some Emulsion Attributes of the Common Carp (Cyprinus carpio L., 1758). Food Hydrocolloids 2006, 20 (6), 825–830.
- Martelo-Vidal, M.J.; Fernandsz-No, I.C.; Guerra-Rodriguez, E.; Vazquez, M. Obtaining Reduced-Salt Restructured White Tuna (Thunnus alalunga) Mediated by Microbial Transglutaminase. LWT-Food Science and Technology 2016, 65 (1), 341–348.
- Hurtado, S.; Saguer, E.; Toldra, M.; Pares, D.; Carrtero, C. Porcine Plasma as Polyphosphate and Caseinate Replacer in Frankfurters. Meat Science 2012, 90 (3), 624–628.
- Cooper, M.; Klutka, R.; Randall, J. Assessing Sodium Intakes: Are Reduced Sodium Food Choices Being Made? Journal of Nutrition Education and Behavior 2015, 47 (4Suppl.), S44–S45.
- Haroldsen, P.E.; Musson, D.G.; Hanson, B.; Quartel, A.; O’neill, C.A. Effects of Food Intake on the Relative Bioavailability of Amifampridine Phosphate Salt in Healthy Adults. Clinical Therapeutics 2015, 37 (7), 1555–1563.
- Zhou, C.L.; Li, J.; Tan, S.J. Effect of L-lysine on the Physicochemical Properties of Pork Sausage. Food Science and Biotechnology 2014a, 23 (3), 775–780.
- Zhou, C.L.; Li, J.; Tan, S.J.; Sun, G.J. Effects of L-arginine on Physicochemical and Sensory Characteristics of Pork Sausage. Advance Journal of Food Science and Technology 2014b, 6 (5), 660–667.
- Sun, X.D.; Holley, R.A. Factors Influencing Gel Formation by Myofibrillar Proteins in Muscle Foods. Comprehensive Reviews in Food Science and Food Safety 2011b, 10 (1), 33–51.
- Wu, Y.; Xu, K.; Xu, X.L.; Niu, L. Effect of PH on Gelation Properties of Rabbit Myosin. Food Science 2010, 31 (9), 6–11. (in Chinese).
- Guo, X.Y.; Peng, Z.Q.; Zhang, Y.W.; Liu, B.; Cui, Y.Q. The Solubility and Conformational Characteristics of Porcine Myosin as Affected by the Presence of L-lysine and L-histidine. Food Chemistry 2015, 170, 212–217.
- Takai, E.; Yoshizawa, S.; Ejima, D.; Arakawa, T.; Shiraki, K. Synergistic Solubilization of Porcine Myosin in Physiologicalsalt Solution by Arginine. International Journal of Biological Macromolecules 2013, 62 (11), 647–651.
- Hayakawa, T.; Iyo, T.; Wakamatsu, J.; Nishimura, T.; Hattori, A. Myosin Is Solubilized in a Neutral and Low Ionic Strength Solution Containing L-histidine. Meat Science 2009, 82 (4), 151–154.
- Matsuoka, T.; Hamada, H.; Matsumoto, K.; Shiraki, K. Indispensable Structure of Solution Additives to Prevent Inactivation of Lysozyme for Heating and Refolding. Biotechnology Progress 2009, 25 (5), 1515–1524.
- Hayakawa, T.; Yoshida, Y.; Yasui, M.; Ito, T.; Iwaskai, T.; Wakamatsu, J.; Hattori, A.; Nishimura, T. Heat-induced Gelation of Myosin in a Low Ionic Strength Solution Containing L-Histidine. Meat Science 2012, 90 (1), 77–80.
- Chen, X.; Li, Y.; Zhou, R.; Liu, Z.M.; Lu, F.Z.; Lin, H.; Xu, X.L.; Zhou, G.H. L-histidine Improves Water Retention of Heat-induced Gel of Chicken Breast Myofibrillar Proteins in Low Ionic Strength Solution. International Journal of Food Science & Technology 2016, 51 (5), 1195–1203.
- Lei, Z.; Fu, Y.; Xu, P.; Zheng, Y.D.; Zhou, C.L. Effects of L-arginine on the Physicochemical and Gel Properties of Chicken Actomyosin. International Journal of Biological Macromolecules 2016, 92, 1258–1265.
- Qin, H.; Xu, P.; Zhou, C.L.; Wang, Y.J. Effects of L-arginine on Water Holding Capacity and Texture of Heat-Induced Gel of Salt-Soluble Proteins from Breast Muscle. LWT-Food Science and Technology 2015, 63 (2), 912–918.
- Liu, R.; Zhao, S.M.; Liu, Y.M.; Yang, H.; Xiong, S.B.; Xie, B.J.; Qin, L.H. Effect of Ph on the Gel Properties and Secondary Structure of Fish Myosin. Food Chemistry 2010, 121 (1), 196–202.
- Han, M.Y.; Wang, P.; Xu, X.L.; Zhou, G.H. Low-Field NMR Study of Heat-induced Gelation of Pork Myofibrillar Proteins and Its Relationship with Microstructural Characteristics. Food Research International 2014, 62, 1175–1182.
- Li, X.K.; Xia, W.S. Effects of Chitosan on the Gel Properties of Salt-soluble Meat Proteins from Silver Carp. Carbohydrate Polymers 2010, 82 (3), 958–964.
- Shimada, M.; Takai, E.; Ejima, D.; Arakawa, T.; Shiraki, K. Heat-induced Formation of Myosin Oligomer-Soluble Filament Complex in High-Salt Solution. International Journal of Biological Macromolecules 2015, 73, 17–22.
- Wachirasiri, K.; Wlnlapa, S.; Uttapap, D.; Rungsardthong, V. Use of Amino Acids as a Phosphate Alternative and Their Effects on Quality of Frozen White Shrimps (Penaeus vanamei). LWT-Food Science and Technology 2016, 69, 303–311.
- Samejima, K.; Ishioroshi, M.; Yasui, T. Relative Roles of the Head and Tail Portions of the Molecule in Heat-Induced Gelation of Myosin. Journal of Food Science 1981, 46 (5), 1412–1418.
- Wu, Z.; Bertaam, H.C.; Kohler, A.; Bocker, U.; Ofastad, R.; Andseren, H.J. Influence of Aging and Salting on Protein Secondary Structures and Water Distribution in Uncooked and Cooked Pork. A Combined FT-IR Microspectroscopy and 1H NMR Relaxometry Study. Journal of Agriculture and Food Chemistry 2006, 54 (22), 8589–8597.
- Bernal, V.M.; S, C.H.; Smith, J.L.; Stanley, D.W. Interactions in Protein/Polysaccharide/Calcium Gels. Journal of Food Science 1987, 52 (5), 1121–1136.
- Chen, H.H.; Xu, S.Y.; Wang, Z. Interaction between Flaxseed Gum and Meat Protein. Journal of Food Engineering 2007, 80 (4), 1051–1059.
- Sun, J.X.; Li, X.; Xu, X.L.; Zhou, G.H. Influence of Various Levels of Flaxseed Gum Addition on the Water-Holding Capacities of Heat-induced Porcine Myofibrillar Protein. Journal of Food Science 2011a, 76 (3), C472–C478.
- Utsumi, S.; Kinsella, J.E. Forces Involved in Soy Protein Gelation: Effects of Various Reagents on the Formation, Hardness and Solubility of Heat-induced Gels Meade from 7S, 11S and Soy Isolate. Journal of Food Science 1985, 50 (5), 1278–1282.
- Mason, P.E.; Dempsey, C.E.; Neilson, G.W.; Kline, S.R.; Brady, J.W. Preferential Interactions of Guanidinum Ions with Aromatic Groups over Aliphatic Groups. Journal of the American Chemical Society 2009, 131 (46), 16689–16696.
- Schneider, C.P.; Trout, B.L. Investigation of Cosolute-protein Preferential Interaction Coefficients: New Insight into the Mechanism by Which Arginine Inhibits Aggregation. Journal of Physical Chemistry. B 2009, 113 (7), 2050–2058.
- Yamamoto, K.; Samejima, K.; Yasui, T. Heat-Induced Gelation of Myosin Filaments. Agricultural and Biological Chemistry 1988, 52 (7), 1803–1811.
- Yamamoto, K.;. Electron Microscopy of Thermal Aggregation of Myosin. Journal of Biochemistry 1990, 108 (6), 896–898.